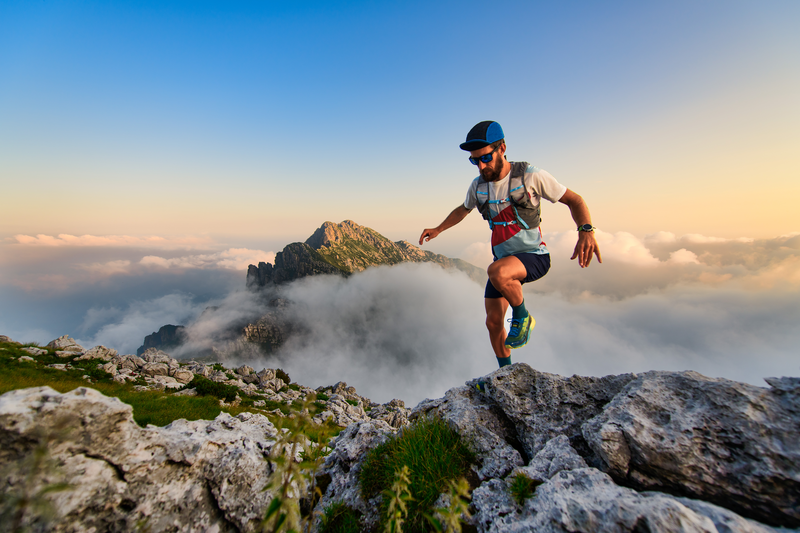
94% of researchers rate our articles as excellent or good
Learn more about the work of our research integrity team to safeguard the quality of each article we publish.
Find out more
ORIGINAL RESEARCH article
Front. Genet. , 03 March 2023
Sec. Computational Genomics
Volume 14 - 2023 | https://doi.org/10.3389/fgene.2023.1086368
This article is part of the Research Topic 21st International Conference on Bioinformatics (InCoB 2022) - Accelerating Innovation to Meet Biological Challenges: The Role of Bioinformatics View all 6 articles
Objective: Screening out potential herbal medicines and herbal ingredients for the treatment of gastric cancer based on transcriptomic analysis of immune infiltration and ferroptosis.
Methods: Gene expression profiles of gastric tumour tissues and normal tissue samples were obtained from the GEO database and the samples were analysed for immune cell infiltration condition and differential expressed genes of ferroptosis. Key genes were screened by protein-protein interaction (PPI) and enrichment analysis, and molecular docking was used to predict and preliminary validate potential herbal and traditional Chinese medicine components for gastric cancer based on the key genes. Finally, RT-QPCR was used to validate the prediction results.
Results: Immune cell infiltration analysis revealed high levels of infiltration of activated CD4 memory T cells, monocytes, M0 macrophages in gastric tumor tissues, while plasma cells and resting mast cells had higher levels of infiltration in the paraneoplastic tissues. Differential gene expression analysis identified 1,012 upregulated genes and 880 downregulated genes, of which 84 immune related differentially expressed genes such as CTSB, PGF and PLAU and 10 ferroptosis-related differentially expressed genes such as HSF1, NOX4 and NF2 were highly expressed in gastric cancer tissues. The results of enrichment analysis showed that they mainly involve 343 biological processes such as extracellular matrix organization and extracellular structural organization; 37 cellular components such as complexes of collagen trimer and basement membrane; 35 molecular functions such as signal receptor activator activity and receptor ligand activity; 19 regulatory pathways such as cytokine-cytokine receptor interactions and retinol metabolism. Finally, two key genes, TLR4 and KRAS, were selected and 12 herbal medicines such as Radix Salviae liguliobae, Rhizoma Coptidis, Rhizoma Polygoni cuspidati and 27 herbal ingredients such as resveratrol, salvianolic acid b were predicted on the basis of key genes. Molecular docking results showed that KRAS binds tightly to coumarin and magnolol, while TLR4 can bind tightly to resveratrol, curcumin, salvianolic acid b, shikonin. Subsequently, the effect of resveratrol and magnolol was experimentally verified.
Conclusion: Herbal medicines such as S. liguliobae, Rhizoma Coptidis, Rhizoma P. cuspidati and herbal ingredients such as resveratrol, curcumin, salvianolic acid b may provide research directions and alternative therapeutic approaches for immunomodulation of TME and ferroptosis of tumour cells in gastric cancer.
Gastric cancer is the fifth most common cancer and the third most common cause of cancer death worldwide (Thrift and El-Serag, 2020), is a global health problem with more than one million new diagnoses each year (Smyth et al., 2020). Although the incidence and mortality rate of gastric cancer has declined over the past half century, it remains the third leading cause of cancer death worldwide (Sexton et al., 2020). Although the 5-year survival rate for patients who undergo surgery at an early stage can be as high as 95%, the recurrence rate after surgery is high (Song et al., 2017). Clinical problems are prominent due to the low rate of early diagnosis of gastric cancer, the large number of patients with advanced stages and the fact that survival time for patients with advanced metastases is usually less than 1 year (Johnston and Beckman, 2019; Tan, 2019; Patel and Cecchini, 2020). A significantly higher proportion of microsatellite instability (MSI) features are present in gastrointestinal adenocarcinomas compared to all other forms of cancer (Liu et al., 2018). According to the Cancer Genome Atlas Research Network, gastric adenocarcinomas can be divided into four distinct subtypes based on the molecular markers that distinguish them: Epstein–Barr virus (EBV) positive tumors, chromosomal instability (CIN), genomically stability, and MSI-H (Liu et al., 2018; Pietrantonio et al., 2019). MSI-H tumors constitute approximately 23 percent of all GC.
Systemic immunotherapy, surgery, targeted therapy, radiotherapy and chemotherapy have all proven effective in the treatment of gastric adenocarcinoma. However, their clinical efficacy has been disappointing, making the pursuit of better treatment strategies a natural choice. Recently, immunotherapy has emerged as a promising approach for the treatment of gastric cancers (GC) (Coutzac et al., 2019). Inhibition of the programmed death-1 (PD-1)/programmed death ligand 1 (PD-L1) axis with immune checkpoint inhibitors (ICIs) such as nivolumab and pembrolizumab has emerged as a novel therapeutic strategy for advanced GC (Abozeid et al., 2017; Bizzaro et al., 2018; Kono et al., 2020). One of the main causes of uncontrolled proliferation of cancer cells is the disturbance of the tumour microenvironment (TME), which contains a large number of negative immune cells that can directly or indirectly create a survival environment favorable to tumor cell proliferation by modulating the immune system (Oya et al., 2020). Cumulative alterations in genes leading to the transformation of normal cells into cancer cells are reflected in next-generation sequencing technologies. Accurate and timely DNA repair is essential to maintain gene stability. Among the many DNA repair pathways, the mismatch repair (MMR) pathway is critical. Lack of MMR contributes to the molecular signature of MSIs, which in turn increases the risk of cancer. Biomarkers, in particular MSI, PD-L1, tumor mutation burden, Epstein-Barr virus, and human epidermal growth factor receptor 2 (HER2) are becoming an increasingly important factors in systemic therapeutic methods and in determining which patient groups are most likely to benefit from immunotherapy and targeted therapies (Joshi and Badgwell, 2021). Ferroptosis is a form of controlled cell death that is dependent on the presence of iron. It is caused by the accumulation of harmful lipid peroxides on cellular membranes (Dixon et al., 2012). This unique form of cell death that does not dependent on programmed regulation and poses a great threat to tumour cells. In recent years, significant progress has been made in understanding the role of ferroptosis in tumour biology and cancer therapy (Hassannia et al., 2019; Mou et al., 2019; Su et al., 2020). As ferroptosis has been increasingly studied, a growing number of findings suggest that ferroptosis frequently interferes with the immune response, leading to inflammation-associated immunosuppression and thus promoting tumour cell growth (Friedmann Angeli et al., 2019; Chen et al., 2021a).
Research models for predicting alternative candidates of Chinese medicine or herbs for the treatment of myocardial infarction (Jiang et al., 2022a), atrial fibrillation (Jiang et al., 2022b) and sports injury (Jiang et al., 2022c) with herbs or Chinese herbal medicines have been developed by us. This research aims to provide a new research mode to predict alternative candidates for the treatment of gastric cancer on the basis of immune infiltration and ferroptosis so that developing new alternative treatment strategies.
In order to obtain gene expression profiles of gastric cancer and normal tissue, the dataset (GSE13911) was searched in GEO database (Table 1), and gene IDs were converted into gene symbols.
An analysis of immune cell infiltration was performed using the CIBERSORT deconvolution method (perm = 1,000). Based on the cut-off criteria |logFC| ≥ 1 and adjP ≤ 0.05, gene expression profiles were filtrated for differentially expressed genes (DEGs).
Additionally, we investigated the differential expression of immune- and ferroptosis-related genes among gastric cancer tissue. From the ImmPort and FerrDb databases (Table 1), we identified genes associated with immunity and ferroptosis. Then, by intersecting them with DEGs, we were able to identify Immune-related DEGs (ImmDEGs) as well as ferroptosis-related.
In our study, DEGs were subjected from the STRING database (Table 1). We applied Protein-protein interaction (PPI) analysis to DEGs and screened the top 100 genes using the Cytohubba plugin in Cytoscape. These genes were identified as hub genes. In addition, DEGs were analyzed using the R package clusterprofiler (cutoff: p ≤ 0.05 and q ≤ 0.05) for pathway enrichment using Gene Ontology (GO) and Kyoto Encyclopedia of Genes and Genomes (KEGG).
By intersecting ImmDEG, FerDEG, and hub genes, key genes were identified. A reverse prediction algorithm was used to predict target herbal medicines and ingredients from the HERB database (Table 1) on the basis of key genes.
We downloaded the protein structure of key genes encoded from PDB database (Table 1), and the structure of predicted herbal ingredients from Pubchem database. Molecular docking of key genes with herbal ingredients method was utilizing Autodock vina tools, which displayed the best bond way for docking the key genes with herbal ingredients with the lowest binding free energy. The lowest bound free energy model was considered to be the best bond path.
The THP-1 cell used to construct the immune infiltration model, The THP-1 cell was purchased from Wuhan (Procell, China), used for in vitro research and cultivated in RPMI-1640 medium containing 10% foetal bovine serum (Gibco, MA, United States) and 0.05 mM β- Mercaptoethanol in a 37°C cell incubator with 5% CO2. Prior to each experiment, 1.5*106/mL THP-1 cells were inoculated in six-well plates and treated with 100 ng/mL Phorbol-12-myristate-13-acetate (PMA) for 24 h to induce differentiation into macrophages. PMA-induced THP-1 cells were treated as described below. Normal control group (NC): PMA-induced THP-1 cells were cultured in RPMI-1640 medium for 48 h. LPS + INF-Y group: PMA-induced THP-1 cells were incubated with 100 ng/mL lipopolysaccharide (LPS) and 20 ng/mL IFN-γ for 48 h. LPS + INF-Y + Resveratrol: THP-1 cell was first incubated with 2.5 uM Resveratrol for 2 h. LPS + INF-Y+ magnolol: HL-1 was first incubated with 15 uM magnolol for 2 h.
TRIzol reagent was used to extract the total RNA from THP-1 cells. With the use of the EvoM-MLV kits, cDNA was created. On a CFX96 Real-Time PCR Detection System (Bio-Rad Laboratories), quantitative reverse transcription-PCR (RT-qPCR) was carried out using 2X SYBR Green qPCR Master Mix (K1070500, APExBIO, US) as directed by the manufacturer and evaluated using the 2−ΔΔCT method. The ideal temperature conditions were 95°C for 30 s, 95°C for 5 s, and 40 cycles at 60°C for 5 s. The expression of the target genes was examined using the 2−ΔΔCT technique after the amounts of mRNA were adjusted to endogenous GAPDH.
From the GEO database, the targeted gene expression matrix (GSE13911) attained by our team. In the data matrix, gene expression levels are compared between gastric tumor tissue and normal tissue. The gastric cancer group included 38 samples, and the normal tissue group included 31 samples.
Differential immune cell infiltrations between normal tissue and gastric cancer tissues was shown in Figure 1A. We then used Wilcoxon test to find that resting CD4 memory T cells (p = 0.002), activated CD4 memory T cells (p = 0.01), M0 macrophages (p < 0.001), M1 macrophages (p < 0.001), M2 macrophages (p = 0.011), activated mast cells (p = 0.004) and neutrophils (p = 0.043) showed high levels of infiltration in gastric cancer tissues, while plasma cells (p < 0.001), monocytes (p = 0.015) and rested mast cells (p = 0.001) showed relatively higher levels of infiltration in normal tissues (see Figures 1B, C). Immunocell correlation analysis showed a high correlation between activation phase CD4 memory T cells and M1 macrophages (see Figure 2A). Differential gene expression analysis showed that 1,892 DEGs were obtained, including 1,012 upregulated genes and 880 downregulated genes (see Figure 2B).
FIGURE 1. (A) Each bar represents a sample, and each color represents a type of immune cell. Area of the color represents the percentage of immune cell infiltration responsible for total immune cell infiltration; (B). Red and green violin columns represent patients with tumor and normal tissues, respectively. The vertical axis represents the ratio of immune cell infiltration responsible for total immune cell infiltration. p-value, obtained using the Wilcoxon test, represents the difference between the immune cell infiltration level in patients with VSR and VAF; (C). Each column represents a sample, and each row represents a type of immune cell. Color transition from green to red represents an increase in immune cell infiltration level. Contrast group = normal tissue; trial group = tumor tissue.
FIGURE 2. (A) the vertical axis and horizontal axis both represent the type of immune cells, and the value in each cell represents the correlation degree of the two immune cells. The higher the correlation, the redder the color; (B). Upregulated DEGs are highlighted in red and downregulated DEGs in blue. Criteria: |logFC| ≥ 1 and adjP value ≤ 0.05; (C). Expression levels of 167 ImmDEGs are shown, the darker the red color, the higher the expression level, and the darker the green color, the lower the expression level. Con group = normal tissue; trial group = tumor tissue; (D). Same as (C), expression levels of 19 FerDEGs are shown; (E). The PPI network was shown.
167 ImmDEGs was identified by the analysis of immune related gene expression profiles. 84 of these ImmDEGs (e.g., TLR4, CTSB, PGF) were highly expressed and 83 ImmDEGs (e.g., ESRRG, GHRL, CHGA) were lowly expressed in tumor tissues (see Figure 2C). At the same time, analysis of ferroptosis-related genes associated normal tissue and gastric cancer tissues screened out 19 FerDEGs: ten of them, i.e., TLR4, KRAS, HSF1, was highly expressed and nine of them, i.e., MUC1, PROM2, MT1G, were lowly expressed in tumor tissues (see Figure 2D).
PPI network analysis was conducted on DEGs using string database, with medium confidence ≥ 0.4, and then the top 100 genes were identified as hub genes (see Figure 2E). GO enrichment analysis indicated that DEGs were mainly enriched in 343 biological processes (GO-BP) such as extracellular matrix organization, extracellular structure organization, digestion, retinoic acid metabolic process, hormone metabolic process. The DEGs were enriched in 37 cellular components (GO-CC) including complex of collagen trimer, basement membrane, endoplasmic reticulum lume, and were enriched in 35 molecular functions (GO-MF) such as signal receptor activator activity, receptor ligand activity, chemokine activity, chemokine receptor binding (see Figure 3A). Furthermore, by analyzing co-enriched DEGs, some relationships between immune-related BPs, and some common DEGs between immune-related BPs were screened out by us such as BGN and MFAP2. These DEGs were co-enriched in several different BPs and get involved in external encapsulating structure organization, extracellular matrix organization as well as extracellular structure organization (Figure 3B). In fact, many biological processes are mutually interacting such as cellular response to chemokine, neutrophil chemotaxis and neutrophil migration (see Figure 3C). In terms of KEGG pathway enrichment analysis, 19 pathways such as cytokine-cytokine receptor interactions, retinol metabolism, and protein digestion and absorption were enriched (Figure 3D).
FIGURE 3. (A) Top 10 GO enrichment results were displayed, the horizontal axis represents the gene ratio, i.e., the ratio of the number of DEGs to number of total genes. Dot size is proportional to the gene ratio, and dot color from blue to red that the adjusted p-value is smaller; (B). Association among the top 5 immune-related biological processes was identified by analyzing co-enriched DEGs; (C). The relation of interaction between enriched biological process was shown, the circular node represents the biological process, the larger the node size and color are consistent with the enrichment results, the higher the gene ratio is, the larger the node is, and the redder the node color is, the higher the significance is; (D). Same as Figure 2A, KEGG enrichment results were displayed; (E). TLR4 and KRAS at the intersection of 167 ImmDEGs, 19 FerDEGs, and 100 hub genes.
By taking the intersection of ImmDEGs, FerDEGs and hub genes, two common key genes, TLR4 and KRAS were identified (see Figure 3E). 12 herbs (e.g., Rhizoma Coptidis, Folium Isatidis, Acidum Citricum, Radix Stellariae, Trifolium fragiferum) and 27 ingredients (e.g., resveratrol, cinnamaldehyde, oleuropein, shikonin, magnolol) were predicted the HERB database base on this two key genes (Tables 2, 3).
Among the molecular docking results of these herbal ingredients with key genes (Figures 4A–F), we found that TLR4 could bind closely to resveratrol (−6.2 kcal/mol), curcumin (−5.9 kcal/mol), salvianolic acid b (−7.6 kcal/mol), and shikonin (−6.9 kcal/mol), while KRAS bound closely to coumarin (−6.7 kcal/mol) and magnolol (−6.9 kcal/mol).
FIGURE 4. (A) shows TLR4 docked with resveratrol (−6.2 kcal/mol); (B) shows TLR4 docked with curcumin (−5.9 kcal/mol); (C) shows TLR4 docked with salvianolic acid b (−7.6 kcal/mol); (D) shows TLR4 docked with shikonin (−6.9 kcal/mol); (E) shows KRAS docked with coumarin (−6.7 kcal/mol); (F) shows KRAS docked with magnolol (−6.9 kcal/mol).
The results revealed that stimulation with LPS + IFN-γ substantially elevated the mRNA levels of iNOS, IL-1, KRAS, and TLR4 compared to NC group (Figure 5). Both resveratrol and magnolol administration markedly decreased the amount of key gene expression. Resveratrol and magnolol significantly reduced the expression of IL-1, iNOS, KRAS, and TLR4 as compared to the LPS + IFN-γ group.
FIGURE 5. RT-QPCR results for validation over three independent experiments. Data are shown as mean ± standard error of the mean. *p < 0.05, **p < 0.01, ***p < 0.001.
Gastric cancer is one of the leading causes of cancer deaths worldwide, with over one million new cases diagnosed each year (Smyth et al., 2020). The rate of early diagnosis of gastric cancer is low and survival time for patients with advanced metastases is usually less than 1 year (Patel and Cecchini, 2020). Surgical resection of the cancer is the accepted first line of treatment. However, although the 5-year survival rate for patients who undergo surgery at an early stage can be as high as 95% (Song et al., 2017), the recurrence rate after surgery is high, even if the cancer site is completely removed (Johnston and Beckman, 2019). Hence, there is an urgent demand to find novel mechanisms involved in gastric cancer, which may provide promising perspectives on treatment of gastric cancer patients. Ferroptosis and tumor immune micro-environment are one of the key mechanisms of gastric cancer.
Ferroptosis is a regulated form of cell death characterized by the iron-dependent accumulation of lipid hydroperoxides to lethal levels. Ferroptosis is associated with cellular vital metabolism in many diseases, including neoplastic diseases such as gastric cancer and non-neoplastic degenerative diseases such as Alzheimer’s disease, Parkinson’s disease, and ischemia-reperfusion injury (Stockwell et al., 2017; Chen et al., 2021b). Because of the specificity and breadth of this regulated cell death, interventions employing ferroptosis gene target agonists to induce ferroptosis in cancer cells are thought to have great anti-cancer potential (Lee et al., 2020). In this study, we screened 19 genes associated with ferroptosis, of which 10 FerDEGs such as TLR4, KRAS and HSF1 were highly expressed and 9 FerDEGs such as MUC1, PROM2 and MT1G were lowly expressed in tumour tissue. All of these targets have great potential for further study. However, among these genes, TLR4, a member of the TOLL-like receptor family, is the most interesting target among these FerDEGs. TLR4 is the target of intervention by multiple mechanisms, including ferritinases, inflammation and autophagic pathways. Thus, intervention on TLR4 may simultaneously produce therapeutic effects from multiple parallel pathways, which has more potential than other single pathway targets of intervention.
Disturbances in the immune system are one of the key causes of tumour development. When tumour cells develop, immune evasion due to immune imbalance causes tumour cells to become unrestrained and uncontrolled. In this study, immune infiltration analysis showed that resting CD4 memory T cells, activated CD4 memory T cells, monocytes, M0 macrophages, M1 macrophages, M2 macrophages, activated mast cells and neutrophils exhibited high levels of infiltration in gastric cancer tissues. There is growing evidence that aberrant behaviour of innate and adaptive immune cells in the tumour microenvironment (TME) contributes to tumour progression (Gajewski et al., 2013). Thus, as shown by the results of immune cell correlation analysis, the significant correlation between activated CD4 memory T cells (adaptive immune cells) and M1 macrophages (innate immune cells) is a manifestation of innate and adaptive immune interactions. Indeed, immune cells themselves are an important component of the tumour stroma and cancer cells themselves can interact with proximal immune cells by secreting various cytokines and chemokines, resulting in TME that is more conducive to tumour growth and metastasis (Li et al., 2020).
Interestingly, there is crosstalk between the immune response and ferritin formation, a property that has been applied in tumour therapy, for example, using CD8+ activation to induce ferritin formation in tumour cells (Tang et al., 2020). This crosstalk can occur in 2 ways: the immune cells themselves undergo ferroptosis in immune-disordered TME; immune cells recognise the tumour cells undergoing ferroptosis and generate an inflammatory clearance response (Hsu et al., 2021). It is clear that we expect a second metabolic modality to occur in tumour cells in TME, and although the exact details of the crosstalk between ferroptosis and immune disorders are currently poorly understood in humans, targeting and modulating this metabolic modality in tumour cells in TME is a very promising therapeutic approach. We therefore hope to predict some of the drugs that regulate this complex metabolic pathway by searching for genes common to both immunity and ferroptosis.
This study identified TLR4 and KRAS as the key genes through a comprehensive analysis of immune infiltration-related and ferroptosis-related genes. TLR4 has been associated with cancer in multiple ways. Various cell lines and tissue samples from patients with head and neck, esophageal, gastric, colorectal, liver, pancreatic, skin, breast, ovarian, cervical, and breast cancer were found to express high levels of TLR4 (Awasthi, 2014). TLR4 has been shown to be involved in immune responses to inflammatory signaling pathways that promote gastric carcinogenesis and to inhibit the immune response by mediating macrophage M2-type polarization, promoting tumor cell metastasis and being strongly associated with the outlook for those diagnosed with gastric cancer (He et al., 2018; Li et al., 2021). Activation of TLR4-NF-B under inflammatory conditions induces cancer cell motility and invasion (Liao et al., 2012; Huang et al., 2020a). Inhibition of TLR4 by siRNA and NF-B inhibitors can reduce cancer cell invasiveness. TLR4 induces an effective cytotoxic T cell immune response to tumour antigens (Fang et al., 2014). Ultimately, cytotoxic T cells will eliminate cancer cells. Numerous studies have demonstrated that TLR4 agonists do not significantly induce ferroptosis in cancer cells, but rather cause a negative immune response in in vivo against tumour population cells due to increased ferroptosis, indirectly creating a TME that is more favourable to cancer cell proliferation (Shetab Boushehri and Lamprecht, 2018; Kashani et al., 2021). Inhibition of TLR4 significantly attenuates the proliferation of inflammatory tumour cells, a phenomenon that has been demonstrated in several studies, such as the inhibition of the expression of this gene impedes the growth of colitis-associated colon and ovarian cancer cells (Earl et al., 2009; Kashani et al., 2020). Inhibition of TLR4 has been shown to reduce tumour burden in mouse models of hepatic steatosis and colorectal metastasis (Timar and Kashofer, 2020). Therefore, we suggest that TLR4 may be an important target in gastric cancer TME, where immune responses and ferritinase interactions are probably based on this key genes.
RAS mutation represents the most common oncogenic changes in human tumours. KRAS is the subtype of RAS that mutates at the highest rate (Quinlan et al., 2008; Fan et al., 2021). The proliferation of endothelium stem and progenitor cells is controlled by activated KRAS, which also plays a role in promoting the development of endodermally derived cancers. Oncogenic KRAS is responsible for cancer cell proliferation, energy metabolism, chemoresistance, invasion and metastasis (Fisher et al., 2001). KRAS extinction has been shown to cause tumour regression in genetically produced KRAS-mutant cancer (Boutin et al., 2017; Ayatollahi et al., 2018). Approximately to 11% of gastric cancer (GC) patients have KRAS mutations, with the most prevalent mutations occurring at codons 12 and 13 (Hewitt et al., 2019). Furthermore, according to a large multicenter investigation, KRAS activation occurs in the early phases of GC before developing a phenotype (Qian et al., 2014). KRAS activation is associated with EMT, MSI status and poor patient outcomes (Fu et al., 2019; Polom et al., 2019). Within malignancies, KRAS may be responsible for generating an inflammatory environment. Cancer cells driven by KRAS release cytokines and other substances to regulate stromal cells, such as fibroblasts and innate and adaptive immune cells, in their vicinity. Activation of the KRAS signaling pathway may stimulate the synthesis of chemokines, such as interleukin-8 (CXCL-8/IL-8), LIX, MIP2, KC and MCP-1 (Sparmann and Bar-Sagi, 2004; Ji et al., 2006). Positive feedback loops stimulate inflammatory mediators Src, EGR1, STAT3, NF-kB, and Cox-2 to maintain the oncogenic activity of KRAS (di Magliano and Logsdon, 2013). The mutant KRAS secretes IL-10 and TGF-b1 via the MEK-ERK-AP1 pathway, therefore inhibiting the activation of T cells (Zdanov et al., 2016). Immune evasion is a defining feature of KRAS-driven cancer, whereas KRAS produces immunosuppressive effects in tumour tissue by promoting macrophage M2 polarisation (suppressing-inflammatory phenotype), thereby creating a more hospitable TME ecological niche for cell proliferation and metastasis (Ischenko et al., 2021). It has been shown that KRAS-G12D protein released from tumour cells undergoes ferrogenesis and is taken up by macrophages, leading to the conversion of macrophages to an M2-like suppressing-inflammatory pro-tumour phenotype (Janes et al., 2018). Not surprisingly, KRAS acts as a dual regulator of the immune response and ferroptosis, similar to the role of TLR4 described above. Although KRAS was previously thought to be untargetable, studies coming in have found that targeted inhibitors of KRAS work satisfactorily (Fang et al., 2021). Therefore, herbal medicines and herbal ingredients predicted on the basis of KRAS also have great potential.
The HERB database is the world’s largest and most advanced database for the modernisation of Chinese medicine. The data in the database are derived from high-throughput experiments, so the database predicts results with high reliability (Fu et al., 2022). In this study, a total of 12 herbal medicines such as Rhizoma Coptidis, Folium Isatidis, Acidum Citricum, Radix Stellariae, T. fragiferum and 27 herbal ingredients such as resveratrol, cinnamaldehyde, oleuropein, shikonin and magnolol, were directly predicted based on the key genes TLR4 and KRAS. In fact, some of the predicted herbs such as Rhizoma Coptidis (Chai et al., 2018; Kim et al., 2019), Rhizoma Polygoni cuspidati (Jiang et al., 2017) and Radix Salviae liguliobae (Wilke et al., 2014) have been shown to have significant anti-gastric cancer effects. Predicted anti-gastric cancer effects of herbal ingredients such as taxol (Huang et al., 2020b; Zhang et al., 2021), coumarin (Kaur et al., 2015; Xu et al., 2017), resveratrol (Yan et al., 2020; Ren et al., 2021) and magnolol (Zhao et al., 2022) have also been demonstrated. Among the molecular docking results of these herbal ingredients with key genes, we found that TLR4 could bind closely to salvianolic acid b (−7.6 kcal/mol), and shikonin (−6.9 kcal/mol), while KRAS bound closely to coumarin (−6.7 kcal/mol) and magnolol (−6.9 kcal/mol). Salvianolic acid B have potent efficacy on cancer in vitro and in vivo. The mechanisms mainly include induction of apoptosis, autophagy and cell cycle arrest, anti-metastasis, formation of cancer stem cells, and potentiation of antitumor immunity (Boulos et al., 2019). At low doses, Shikonin produced apoptotic cell death and at high amounts, necroptosis (Xuan and Hu, 2009; Yang et al., 2014). Shikonin triggered dose-dependent apoptosis in cancer cells by raising intracellular levels of reactive oxygen species (ROS), which are primarily formed by lipoxygenase, NADPH oxidase, and mitochondrial complex II (Zhao et al., 2022).
Finally, Experiments in vitro were used to verify the modulatory effect of resveratrol and magnolol on immune imbalance after THP-1 cell stimulating with LPS + IFN-γ. We examined the expression levels of key genes (iNOS, IL-1, KRAS and TLR4) in each macrophage group by quantitative reverse transcription PCR and found that the expression levels of these genes in the resveratrol and magnolol groups were significantly lower than those in the LPS + IFN-γ group (see Figure 5).
Our study’s findings indicated that resveratrol and magnolol, which have both therapeutic and preventative benefits for patients with gastric cancer, have significant clinical implications. Resveratrol, magnolol, and our study model offer considerable potential in this field, especially in light of the apparent underdevelopment of treatments for gastric cancer. With the use of immune infiltration and ferroptosis, this study introduced a new research approach for predicting potential therapy options for gastric cancer, paving the way for the development of novel therapeutic approaches.
Therefore, these predicted herbs and herbal ingredients have great potential to improve the prognosis of gastric cancer patients by acting on the targets TLR4 and KRAS, and provide alternative options and direction for anti-gastric cancer herbal research.
Immune evasion is one of the major causes of loss of restraint in tumour cells, and iron death is an important modality regulating tumour cell death. TLR4 and KRAS, as common genes for immune infiltration and ferroptosis, are involved in gastric carcinogenesis and play an important role in gastric cancer progression. Based on the prediction of these two key genes, herbal ingredients such as resveratrol, magnolol, curcumin, salvianolic acid b, as well as herbal medicines such as S. liguliobae, Rhizoma Coptidis and Rhizoma Polygoni cuspidate provide research directions and alternative therapies for immunomodulation of TME and ferroptosis of tumour cells in gastric cancer.
The datasets presented in this study can be found in online repositories. The names of the repository/repositories and accession number(s) can be found in the article/Supplementary Material.
ML and CC designed and planned the study and identified and selected original studies. JT and FJ drafted the manuscript. ZZ and YS revised the final manuscript. RQ and JT performed all the bioinformatics analyses. ML and RQ contributed to data analysis. CC and ZZ provided advice during the study and manuscript preparation. All authors read and approved the final manuscript.
This work was supported by grants from the Natural Science Foundation of Guangdong Province of China (2021A1515011095), the Bao’an Science and Technology Innovation department of Shenzhen city of China (Grant Nos. 2020JD567, 2021JD068) and the Bao’an Chinese Medicine Development Foundation of China (Grant No. 2020KJCK-KTYJ-39).
The authors declare that the research was conducted in the absence of any commercial or financial relationships that could be construed as a potential conflict of interest.
All claims expressed in this article are solely those of the authors and do not necessarily represent those of their affiliated organizations, or those of the publisher, the editors and the reviewers. Any product that may be evaluated in this article, or claim that may be made by its manufacturer, is not guaranteed or endorsed by the publisher.
The Supplementary Material for this article can be found online at: https://www.frontiersin.org/articles/10.3389/fgene.2023.1086368/full#supplementary-material
Abozeid, M., Rosato, A., and Sommaggio, R. (2017). Immunotherapeutic strategies for gastric carcinoma: A review of preclinical and clinical recent development. BioMed Res. Int. 2017, 5791262. doi:10.1155/2017/5791262
Awasthi, S. (2014). Toll-like receptor-4 modulation for cancer immunotherapy. Front. Immunol. 5, 328. PMID:25120541. doi:10.3389/fimmu.2014.00328
Ayatollahi, H., Tavassoli, A., Jafarian, A. H., Alavi, A., Shakeri, S., Shams, S. F., et al. (2018). KRAS codon 12 and 13 mutations in gastric cancer in the northeast Iran. Iran. J. Pathol. 13 (2), 167–172. PMID:30697286. doi:10.30699/ijp.13.2.167
Bizzaro, N., Antico, A., and Villalta, D. (2018). Autoimmunity and gastric cancer. IJMS 19 (2), 377. doi:10.3390/ijms19020377
Boulos, J. C., Rahama, M., Hegazy, M-E. F., and Efferth, T. (2019). Shikonin derivatives for cancer prevention and therapy. Cancer Lett. 459, 248–267. PMID:31132429. doi:10.1016/j.canlet.2019.04.033
Boutin, A. T., Liao, W-T., Wang, M., Hwang, S. S., Karpinets, T. V., Cheung, H., et al. (2017). Oncogenic kras drives invasion and maintains metastases in colorectal cancer. Genes. Dev. 31 (4), 370–382. doi:10.1101/gad.293449.116
Chai, F-N., Ma, W-Y., Zhang, J., Xu, H. S., Li, Y. F., Zhou, Q. D., et al. (2018). Coptisine from rhizoma Coptidis exerts an anti-cancer effect on hepatocellular carcinoma by up-regulating MiR-122. Biomed. Pharmacother. 103, 1002–1011. doi:10.1016/j.biopha.2018.04.052
Chen, X., Kang, R., Kroemer, G., and Tang, D. (2021). Broadening horizons: The role of ferroptosis in cancer. Nat. Rev. Clin. Oncol. 18 (5), 280–296. doi:10.1038/s41571-020-00462-0
Chen, X., Kang, R., Kroemer, G., and Tang, D. (2021). Ferroptosis in infection, inflammation, and immunity. J. Exp. Med. 218 (6), e20210518. PMID:33978684. doi:10.1084/jem.20210518
Coutzac, C., Pernot, S., Chaput, N., and Zaanan, A. (2019). Immunotherapy in advanced gastric cancer, is it the future? Crit. Rev. Oncology/Hematology 133, 25–32. doi:10.1016/j.critrevonc.2018.10.007
di Magliano, M. P., and Logsdon, C. D. (2013). Roles for KRAS in pancreatic tumor development and progression. Gastroenterology 144 (6), 1220–1229. PMID:23622131. doi:10.1053/j.gastro.2013.01.071
Dixon, S. J., Lemberg, K. M., Lamprecht, M. R., Skouta, R., Zaitsev, E. M., Gleason, C. E., et al. (2012). Ferroptosis: An iron-dependent form of nonapoptotic cell death. Cell. 149 (5), 1060–1072. PMID:22632970. doi:10.1016/j.cell.2012.03.042
Earl, T. M., Nicoud, I. B., Pierce, J. M., Wright, J. P., Majoras, N. E., Rubin, J. E., et al. (2009). Silencing of TLR4 decreases liver tumor burden in a murine model of colorectal metastasis and hepatic steatosis. Ann. Surg. Oncol. 16 (4), 1043–1050. doi:10.1245/s10434-009-0325-8
Fan, G., Lou, L., Song, Z., Zhang, X., and Xiong, X. F. (2021). Targeting mutated GTPase KRAS in tumor therapies. Eur. J. Med. Chem. 226, 113816. doi:10.1016/j.ejmech.2021.113816
Fang, H., Ang, B., Xu, X., Huang, X., Wu, Y., Sun, Y., et al. (2014). TLR4 is essential for dendritic cell activation and anti-tumor T-cell response enhancement by DAMPs released from chemically stressed cancer cells. Cell. Mol. Immunol. 11 (2), 150–159. doi:10.1038/cmi.2013.59
Fang, S., Dong, L., Liu, L., Guo, J., Zhao, L., Zhang, J., et al. (2021). Herb: A high-throughput experiment- and reference-guided database of traditional Chinese medicine. Nucleic Acids Res. 49 (D1), D1197–D1206. doi:10.1093/nar/gkaa1063
Fisher, G. H., Wellen, S. L., Klimstra, D., Lenczowski, J. M., Tichelaar, J. W., Lizak, M. J., et al. (2001). Induction and apoptotic regression of lung adenocarcinomas by regulation of a K-ras transgene in the presence and absence of tumor suppressor genes. Genes. Dev. 15 (24), 3249–3262. doi:10.1101/gad.947701
Friedmann Angeli, J. P., Krysko, D. V., and Conrad, M. (2019). Ferroptosis at the crossroads of cancer-acquired drug resistance and immune evasion. Nat. Rev. Cancer 19 (7), 405–414. doi:10.1038/s41568-019-0149-1
Fu, X-H., Chen, Z-T., Wang, W-H., Fan, X. J., Huang, Y., Wu, X. B., et al. (2019). KRAS G12V mutation is an adverse prognostic factor of Chinese gastric cancer patients. J. Cancer 10 (4), 821–828. doi:10.7150/jca.27899
Fu, M., Liu, Y., Cheng, H., Xu, K., and Wang, G. (2022). Coptis chinensis and dried ginger herb combination inhibits gastric tumor growth by interfering with glucose metabolism via LDHA and SLC2A1. J. Ethnopharmacol. 284, 114771. doi:10.1016/j.jep.2021.114771
Gajewski, T. F., Schreiber, H., and Fu, Y. X. (2013). Innate and adaptive immune cells in the tumor microenvironment. Nat. Immunol. 14 (10), 1014–1022. PMID: 24048123. doi:10.1038/ni.2703
Hassannia, B., Vandenabeele, P., and Vanden Berghe, T. (2019). Targeting ferroptosis to iron out cancer. Cancer Cell. 35 (6), 830–849. doi:10.1016/j.ccell.2019.04.002
He, B., Xu, T., Pan, B., Pan, Y., Wang, X., Dong, J., et al. (2018). Polymorphisms of TGFBR1, TLR4 are associated with prognosis of gastric cancer in a Chinese population. Cancer Cell. Int. 18, 191. PMID:30479570. doi:10.1186/s12935-018-0682-0
Hewitt, L. C., Saito, Y., Wang, T., Matsuda, Y., Oosting, J., Silva, A. N. S., et al. (2019). KRAS status is related to histological phenotype in gastric cancer: Results from a large multicentre study. Gastric Cancer 22 (6), 1193–1203. doi:10.1007/s10120-019-00972-6
Hsu, S. K., Li, C. Y., Lin, I. L., Syue, W. J., Chen, Y. F., Cheng, K. C., et al. (2021). Inflammation-related pyroptosis, a novel programmed cell death pathway, and its crosstalk with immune therapy in cancer treatment. Theranostics 11 (18), 8813–8835. doi:10.7150/thno.62521
Huang, H. C., Cai, B. H., Suen, C. S., Lee, H. Y., Hwang, M. J., Liu, F. T., et al. (2020a). BGN/TLR4/NF-B mediates epigenetic silencing of immunosuppressive siglec ligands in colon cancer cells. Cells 9 (2), 397. doi:10.3390/cells9020397
Huang, S-L., Chang, T-C., Chao, C. C. K., and Sun, N. K. (2020b). Role of the TLR4-androgen receptor Axis and genistein in taxol-resistant ovarian cancer cells. Biochem. Pharmacol. 177, 113965. doi:10.1016/j.bcp.2020.113965
Ischenko, I., D’Amico, S., Rao, M., Li, J., Hayman, M. J., Powers, S., et al. (2021). KRAS drives immune evasion in a genetic model of pancreatic cancer. Nat. Commun. 12 (1), 1482. doi:10.1038/s41467-021-21736-w
Janes, M. R., Zhang, J., Li, L-S., Hansen, R., Peters, U., Guo, X., et al. (2018). Targeting KRAS mutant cancers with a covalent G12C-specific inhibitor. Cell. 172 (3), 578–589.e17. PMID:29373830. doi:10.1016/j.cell.2018.01.006
Ji, H., Houghton, A. M., Mariani, T. J., Perera, S., Kim, C. B., Padera, R., et al. (2006). K-ras activation generates an inflammatory response in lung tumors. Oncogene 25 (14), 2105–2112. doi:10.1038/sj.onc.1209237
Jiang, G., Liu, J., Ren, B., Zhang, L., Owusu, L., Liu, L., et al. (2017). Anti-tumor and chemosensitization effects of cryptotanshinone extracted from salvia miltiorrhiza bge. On ovarian cancer cells in vitro. J. Ethnopharmacol. 205, 33–40. doi:10.1016/j.jep.2017.04.026
Jiang, F., Zeng, Z., Zhou, X., Tan, M., Zhang, W., Li, M., et al. (2022). Transcriptomic analysis uncovers immunogenic characteristics of ferroptosis for myocardial infarction and potential therapeutic prediction of Chinese herbs. Evid. Based Complement. Altern. Med. 25 (5), 4918343. doi:10.1155/2022/4918343
Jiang, F., Zhang, W., Lu, H., Tan, M., Zeng, Z., Song, Y., et al. (2022). Prediction of herbal medicines based on immune cell infiltration and immune- and ferroptosis-related gene expression levels to treat valvular atrial fibrillation. Front. Genet. 28 (9), 886860. doi:10.3389/fgene.2022.886860
Jiang, F., Yang, R., Xue, D., Li, R., Tan, M., Zeng, Z., et al. (2022). Effects of a natural nutritional supplement on immune cell infiltration and immune gene expression in exercise-induced injury. Front. Nutr. 16 (9), 987545. doi:10.3389/fnut.2022.987545
Johnston, F. M., and Beckman, M. (2019). Updates on management of gastric cancer. Curr. Oncol. Rep. 21 (8), 67. doi:10.1007/s11912-019-0820-4
Joshi, S. S., and Badgwell, B. D. (2021). Current treatment and recent progress in gastric cancer. CA Cancer J. Clin. 71 (3), 264–279. PMID:33592120. doi:10.3322/caac.21657
Kashani, B., Zandi, Z., Bashash, D., Zaghal, A., Momeny, M., Poursani, E. M., et al. (2020). Small molecule inhibitor of TLR4 inhibits ovarian cancer cell proliferation: New insight into the anticancer effect of TAK-242 (resatorvid). Cancer Chemother. Pharmacol. 85 (1), 47–59. doi:10.1007/s00280-019-03988-y
Kashani, B., Zandi, Z., Pourbagheri-Sigaroodi, A., Bashash, D., and Ghaffari, S. H. (2021). The role of toll-like receptor 4 (TLR4) in cancer progression: A possible therapeutic target? J. Cell. Physiol. 236 (6), 4121–4137. doi:10.1002/jcp.30166
Kaur, M., Kohli, S., Sandhu, S., Bansal, Y., and Bansal, G. (2015). Coumarin: A promising scaffold for anticancer agents. ACAMC 15 (8), 1032–1048. doi:10.2174/1871520615666150101125503
Kim, B. R., Ha, J., Lee, S., Park, J., and Cho, S. (2019). Anti-cancer effects of ethanol extract of reynoutria japonica houtt. Radix in human hepatocellular carcinoma cells via inhibition of MAPK and PI3K/akt signaling pathways. J. Ethnopharmacol. 245, 112179. doi:10.1016/j.jep.2019.112179
Kono, K., Nakajima, S., and Mimura, K. (2020). Current status of immune checkpoint inhibitors for gastric cancer. Gastric Cancer 23 (4), 565–578. doi:10.1007/s10120-020-01090-4
Lee, J-Y., Nam, M., Son, H. Y., Hyun, K., Jang, S. Y., Kim, J. W., et al. (2020). Polyunsaturated fatty acid biosynthesis pathway determines ferroptosis sensitivity in gastric cancer. Proc. Natl. Acad. Sci. U. S. A. 117 (51), 32433–32442. doi:10.1073/pnas.2006828117
Li, C., Jiang, P., Wei, S., Xu, X., and Wang, J. (2020). Regulatory T cells in tumor microenvironment: New mechanisms, potential therapeutic strategies and future prospects. Mol. Cancer 19 (1), 116. doi:10.1186/s12943-020-01234-1
Li, Q., Wu, W., Gong, D., Shang, R., Wang, J., and Yu, H. (2021). Propionibacterium acnes overabundance in gastric cancer promote M2 polarization of macrophages via a TLR4/PI3K/akt signaling. Gastric Cancer 24 (6), 1242–1253. doi:10.1007/s10120-021-01202-8
Liao, S-J., Zhou, Y-H., Yuan, Y., Li, D., Wu, F. H., Wang, Q., et al. (2012). Triggering of toll-like receptor 4 on metastatic breast cancer cells promotes avβ3-mediated adhesion and invasive migration. Breast Cancer Res. Tr. 133 (3), 853–863. doi:10.1007/s10549-011-1844-0
Liu, Y., Sethi, N. S., Hinoue, T., Schneider, B. G., Cherniack, A. D., Sanchez-Vega, F., et al. (2018). Comparative molecular analysis of gastrointestinal adenocarcinomas. Cancer Cell. 33 (4), 721–735.e8. PMID:29622466. doi:10.1016/j.ccell.2018.03.010
Mou, Y., Wang, J., Wu, J., He, D., Zhang, C., Duan, C., et al. (2019). Ferroptosis, a new form of cell death: Opportunities and challenges in cancer. J. Hematol. Oncol. 12 (1), 34. doi:10.1186/s13045-019-0720-y
Oya, Y., Hayakawa, Y., and Koike, K. (2020). Tumor microenvironment in gastric cancers. Cancer Sci. 111 (8), 2696–2707. doi:10.1111/cas.14521
Patel, T. H., and Cecchini, M. (2020). Targeted therapies in advanced gastric cancer. Curr. Treat. Options Oncol. 21 (9), 70. doi:10.1007/s11864-020-00774-4
Pietrantonio, F., Miceli, R., Raimondi, A., Kim, Y. W., Kang, W. K., Langley, R. E., et al. (2019). Individual patient data meta-analysis of the value of microsatellite instability as a biomarker in gastric cancer. J. Clin. Oncol. 37 (35), 3392–3400. doi:10.1200/JCO.19.01124
Polom, K., Das, K., Marrelli, D., Roviello, G., Pascale, V., Voglino, C., et al. (2019). KRAS mutation in gastric cancer and prognostication associated with microsatellite instability status. Pathol. Oncol. Res. 25 (1), 333–340. PMID:29116623. doi:10.1007/s12253-017-0348-6
Qian, Z., Zhu, G., Tang, L., Wang, M., Zhang, L., Fu, J., et al. (2014). Whole Genome gene copy number profiling of gastric cancer identifies PAK1 and KRAS gene amplification as therapy targets. Genes. Chromosom. Cancer 53 (11), 883–894. doi:10.1002/gcc.22196
Quinlan, M. P., Quatela, S. E., Philips, M. R., and Settleman, J. (2008). Activated kras, but not hras or nras, may initiate tumors of endodermal origin via stem cell expansion. Mol. Cell. Biol. 28 (8), 2659–2674. PMID:18268007. doi:10.1128/MCB.01661-07
Ren, B., Kwah, M. X-Y., Liu, C., Ma, Z., Shanmugam, M. K., Ding, L., et al. (2021). Resveratrol for cancer therapy: Challenges and future perspectives. Cancer Lett. 515, 63–72. doi:10.1016/j.canlet.2021.05.001
Sexton, R. E., Al Hallak, M. N., Diab, M., and Azmi, A. S. (2020). Gastric cancer: A comprehensive review of current and future treatment strategies. Cancer Metastasis Rev. 39 (4), 1179–1203. doi:10.1007/s10555-020-09925-3
Shetab Boushehri, M. A., and Lamprecht, A. (2018). TLR4-Based immunotherapeutics in cancer: A review of the achievements and shortcomings. Mol. Pharm. 15 (11), 4777–4800. doi:10.1021/acs.molpharmaceut.8b00691
Smyth, E. C., Nilsson, M., Grabsch, H. I., van Grieken, N. C., and Lordick, F. (2020). Gastric cancer. Lancet 396 (10251), 635–648. doi:10.1016/S0140-6736(20)31288-5
Song, Z., Wu, Y., Yang, J., Yang, D., and Fang, X. (2017). Progress in the treatment of advanced gastric cancer. Tumour Biol. 39 (7), 1010428317714626. doi:10.1177/1010428317714626
Sparmann, A., and Bar-Sagi, D. (2004). Ras-induced interleukin-8 expression plays a critical role in tumor growth and angiogenesis. Cancer Cell. 6 (5), 447–458. doi:10.1016/j.ccr.2004.09.028
Stockwell, B. R., Friedmann Angeli, J. P., Bayir, H., Bush, A. I., Conrad, M., Dixon, S. J., et al. (2017). Ferroptosis: A regulated cell death nexus linking metabolism, redox biology, and disease. Cell. 171 (2), 273–285. PMID:28985560. doi:10.1016/j.cell.2017.09.021
Su, Y., Zhao, B., Zhou, L., Zhang, Z., Shen, Y., Lv, H., et al. (2020). Ferroptosis, a novel pharmacological mechanism of anti-cancer drugs. Cancer Lett. 483, 127–136. doi:10.1016/j.canlet.2020.02.015
Tan, Z. (2019). Recent advances in the surgical treatment of advanced gastric cancer: A review. Med. Sci. Monit. 25, 3537–3541. doi:10.12659/MSM.916475
Tang, R., Xu, J., Zhang, B., Liu, J., Liang, C., Hua, J., et al. (2020). Ferroptosis, necroptosis, and pyroptosis in anticancer immunity. Hematol. Oncol. 13 (1), 110. PMID:32778143. doi:10.1186/s13045-020-00946-7
Thrift, A. P., and El-Serag, H. B. (2020). Burden of gastric cancer. Clin. Gastroenterology Hepatology 18 (3), 534–542. doi:10.1016/j.cgh.2019.07.045
Timar, J., and Kashofer, K. (2020). Molecular epidemiology and diagnostics of KRAS mutations in human cancer. Cancer Metastasis Rev. 39 (4), 1029–1038. doi:10.1007/s10555-020-09915-5
Wilke, H., Muro, K., Van Cutsem, E., Oh, S. C., Bodoky, G., Shimada, Y., et al. (2014). Ramucirumab plus paclitaxel versus placebo plus paclitaxel in patients with previously treated advanced gastric or gastro-oesophageal junction adenocarcinoma (rainbow): A double-blind, randomised phase 3 trial. Lancet Oncol. 15 (11), 1224–1235. doi:10.1016/S1470-2045(14)70420-6
Xu, J., Liu, D., Niu, H., Zhu, G., Xu, Y., Ye, D., et al. (2017). Resveratrol reverses doxorubicin resistance by inhibiting epithelial-mesenchymal transition (EMT) through modulating PTEN/akt signaling pathway in gastric cancer. J. Exp. Clin. Cancer Res. 36 (1), 19. doi:10.1186/s13046-016-0487-8
Xuan, Y., and Hu, X. (2009). Naturally-occurring shikonin analogues--a class of necroptotic inducers that circumvent cancer drug resistance. Cancer Lett. 274 (2), 233–242. doi:10.1016/j.canlet.2008.09.029
Yan, Y., Xu, J., and Mao, G. (2020). Honokiol suppression of human epidermal growth factor receptor 2 (HER2)-Positive gastric cancer cell biological activity and its mechanism. Med. Sci. Monit. 26, e923962. doi:10.12659/MSM.923962
Yang, J-T., Li, Z-L., Wu, J-Y., Lu, F. J., and Chen, C. H. (2014). An oxidative stress mechanism of shikonin in human glioma cells. PLoS One 9 (4), e94180. PMID:24714453. doi:10.1371/journal.pone.0094180
Zdanov, S., Mandapathil, M., Abu Eid, R., Adamson-Fadeyi, S., Wilson, W., Qian, J., et al. (2016). Mutant KRAS conversion of conventional T cells into regulatory T cells. Cancer Immunol. Res. 4 (4), 354–365. PMID:26880715. doi:10.1158/2326-6066.CIR-15-0241
Zhang, J., Feng, M., and Guan, W. (2021). Naturally occurring aesculetin coumarin exerts antiproliferative effects in gastric cancer cells mediated via apoptotic cell death, cell cycle arrest and targeting PI3K/AKT/M-TOR signalling pathway. Acta Biochim. Pol. 68, 109–113. doi:10.18388/abp.2020_5463
Keywords: immune infiltration, ferroptosis, gastric cancer, herbal medicine, herbal ingredient
Citation: Li M, Tao J, Qian R, Jiang F, Song Y, Zeng Z and Cai C (2023) Development of alternative herbals remedy for gastric cancer based on transcriptomic analysis of immune infiltration and ferroptosis. Front. Genet. 14:1086368. doi: 10.3389/fgene.2023.1086368
Received: 01 November 2022; Accepted: 14 February 2023;
Published: 03 March 2023.
Edited by:
Harpreet Singh, Hans Raj Mahila Maha Vidyalaya (HRMMV), IndiaReviewed by:
Chen Yang, Fudan University, ChinaCopyright © 2023 Li, Tao, Qian, Jiang, Song, Zeng and Cai. This is an open-access article distributed under the terms of the Creative Commons Attribution License (CC BY). The use, distribution or reproduction in other forums is permitted, provided the original author(s) and the copyright owner(s) are credited and that the original publication in this journal is cited, in accordance with accepted academic practice. No use, distribution or reproduction is permitted which does not comply with these terms.
*Correspondence: Zhicong Zeng, enpjb25nMjNAMTI2LmNvbQ==; Changlong Cai, NTg0NTQ1MzI4QHFxLmNvbQ==
Disclaimer: All claims expressed in this article are solely those of the authors and do not necessarily represent those of their affiliated organizations, or those of the publisher, the editors and the reviewers. Any product that may be evaluated in this article or claim that may be made by its manufacturer is not guaranteed or endorsed by the publisher.
Research integrity at Frontiers
Learn more about the work of our research integrity team to safeguard the quality of each article we publish.