- 1College of Advanced Agriculture and Ecological Environment, Heilongjiang University, Harbin, China
- 2State Key Laboratory of Tree Genetics and Breeding, College of Forestry, Northeast Forestry University, Harbin, China
- 3Laboratory of Genetic Breeding in Tomato, College of Horticulture and Landscape Architecture, Northeast Agricultural University, Harbin, China
WRKY transcription factors (WRKYs) are one of the largest plant gene families in plants involved in various biotic and abiotic stress responses. Based on the conservation of WRKY proteins, we identified a total of 642 WRKYs in Amborella trichopoda (33), Vitis vinifera (64), Arabidopsis thaliana (48), Solanum lycopersicoides (88), S. pennellii (77), S. pimpinellifolium (80), S. lycopersicum var. cerasiforme (85), S. lycopersicum cv. Heinz1706 (85), and S. lycopersicum cv. M82 (82) genomes. Phylogenetic analysis clustered WRKYs from nine genomes above into two clusters (Cluster1 and Cluster2). Evolutionary analysis revealed that most of the WRKYs in tomato and its wild relatives were expanded after the whole genome triplication (WGT) event of Solanum ancestor. Effects of tandem duplication (TD) event for WRKYs revealed that several WRKYs have experienced TD event and drove the expansion of the WRKY gene family in tomato and its wild relatives. Comparative analysis of WRKYs derived from WGT and TD events indicated that the WGT event performed a stronger influence on the expansion of the WRKY gene family than the effects of the TD event. Transcriptome profiling of WRKYs in S. lycopersicum cv. Heinz1706 under the biotic stress condition relative to the control condition uncovered a number of up-regulated WRKYs in response to biotic stress. The diversified expression pattern among paralogs derived from TD and WGT implied the impact of gene duplication events on gene functional divergence and diversity in tomato. We hope that this project will supply novel knowledge for studying the evolutionary history and functional characteristics of WRKYs involved in biotic stress in tomato.
Background
The WRKY gene family is one of the largest transcription factor families modulating plant development and growth, in particular the responses to biotic and abiotic stresses. The primary characteristic of WRKY proteins is the DNA-binding domain, which contains the WRKYGQK sequence and a zinc-binding motif (Bakshi and Oelmüller, 2014). So far, WRKYs were found in a wide range of higher plants families using the domain conservation, including the 74 WRKYs identified in Arabidopsis, the total of 197, 119, and 100 WRKYs in soybean, maize, and rice, as well as a number of WRKYs in horticultural crops including strawberry (62), apple (127), citrus (50), and etc (Rushton et al., 2010; Wei et al., 2012; Ayadi et al., 2016; He et al., 2016; Meng et al., 2016; Wei et al., 2016). These WRKYs fell into three distinct groups (group I, group II, and group III) (Li et al., 2011). In addition to the genome-wide structural characterization of WRKYs in these different species, WRKYs have also been functionally characterized regarding their important roles involving the defense response to several pathogens and abiotic stresses (heat, drought, salinity, and oxidative stresses) (Joshi et al., 2016). For instance, WRKY27 in pepper, WRKY39, and WRKY40 in upland cotton modulate the resistance to R. solanacearum and wounding-induced response (Shi et al., 2014; Wang et al., 2014; Hussain et al., 2019). In Arabidopsis, WRKY25, WRKY33, and WRKY34 were up-regulated in response to cold and salt treatment respectively along with enhanced stress tolerance in the overexpression line, indicating their roles in conferring tolerance to abiotic stresses (Li et al., 2011). Thus, genomic analysis and functional studies regarding the roles of WRKYs in responses to abiotic and biotic stresses are critical to improving plant resilience and enhancing plant production in face of the changing environments.
Tomato (Solanum lycopersicum) is a worldwide economically important vegetable crop due to its high-level nutrients of fleshy fruit. In 2012, the first tomato high-quality genome, inbred cultivar “Heinz 1706,” was sequenced and released (Sato et al., 2012). After that, genomes of three tomato wild relatives, S.lycopersicoides, S. pennellii, and S. pimpinellifolium, as well as two different phenotypes, S. lycopersicum var. cerasiforme and S. lycopersicum cv. M82 were sequenced, which provided an extensive genomic resource for comparative genomic studies in the Solanum lineage (Bolger et al., 2014; Wang et al., 2020; Takei et al., 2021; Powell et al., 2022). Comparison between S. lycopersicum cv. Heinz1706 and V. vinifera genomes revealed that S. lycopersicum cv. Heinz1706 and V. vinifera shared the whole genome triplication (WGT) with common eudicot ancestor, followed by a recent WGT in S. lycopersicum cv. Heinz1706 (Sato et al., 2012). These comparative frameworks provided an invaluable opportunity for scientists to study the evolutionary relationship of the impact of gene duplications to gene function diversification and evolution.
Here, we collected three tomato species including S. lycopersicum var. cerasiforme, S. lycopersicum cv. Heinz1706, and S. lycopersicum cv. M82, and three tomato wild relatives including S. lycopersicoides, S. pennellii, S. pimpinellifolium to perform genome-wide comparative genomics analysis of WRKY gene family among six Solanum genomes. Then, we collected one basal angiosperm, A. trichopoda, one diploid species after WGT of Eudicot ancestor, V. vinifera, and one model plant, A. thaliana as control, to dissect the evolutionary history and gene family expansion of WRKYs in Solanum lineage. From comparison between V. vinifera and six Solanum genomes respectively, we analyzed the influence of a WGT event on the WRKY gene family to investigate the ancient loci or gene orders retained in six Solanum genomes. Further, we performed the analysis of tandem duplication (TD) events to detect the influence on the expansion of the WRKY gene family in six Solanum genomes. Finally, we investigated the expression patterns of WRKY TFs in S. lycopersicum cv. Heinz1706, in particular the WRKYs involved in the recent WGT and TD events.
Materials and methods
Data resource
V. vinifera (PN40024.v4) and A. trichopoda (AMTR1.0) genomic data were downloaded from Ensembl Genomes 53 (https://plants.ensembl.org/) (Howe et al., 2020). A. thaliana (TAIR11) genomic data were downloaded from TAIR (https://www.arabidopsis.org/) (Cheng et al., 2017). S. lycopersicoides, S. pennellii, S. pimpinellifolium, S. lycopersicum var. cerasiforme, S. lycopersicum cv. Heinz1706, and S. lycopersicum cv. M82 genomic data were downloaded from Sol Genomics Network (https://solgenomics.net/) (Fernandez-Pozo et al., 2015). The profile Hidden Markov Models (HMMs) of the WRKY domain (PF03106.18) was downloaded from Pfam 33.1 (May 2020, 18,259 entries) (http://pfam.xfam.org/) (Mistry et al., 2021). The RNA-seq data of S. lycopersicum cv. Heinz1706 was downloaded from SRA-NCBI with accession number: PRJNA378182 (Huot et al., 2018).
Identification of WRKYs
The “hmmsearch” module with “trusted cutoff” as threshold in HMMER v3.2.1 (http://hmmer.org/) was employed to identify the putative WRKY transcription factors in V. vinifera, A. trichopoda, A. thaliana, and six Solanum genomes. The highly conserved WRKY proteins with E-value =<1e−05 in the results from HMMER software were selected to construct the species-specific profile HMMs for nine target genomes with “hmmbuild” module in HMMER v3.2.1. The new species-specific profile HMMs of nine target genomes were used to search their corresponding genome protein sequences to get the WRKY transcription factor candidates among nine target genomes. The InterProScan was used to validate the WRKY domain among nine target genomes (Jones et al., 2014).
Reconstruction of phylogenetics among different species
All protein sequences of WRKY transcription factors among nine genomes retrieved from respective genome annotations were performed with multiple sequence alignments (MSA) with the “Clustal-W” module in MEGA version 10.2.5 (Kumar et al., 2018). The MSA file was used to construct a phylogenetic tree with the maximum likelihood (ML) statistical method and Jones-Taylor-Thornton (JTT) substitution model. The phylogenetic tree of WRKY transcription factors among V. vinifera and six Solanum genomes was performed with identical procedures and parameters.
Analysis of tandem duplicated WRKYs
For each genome among the nine target genomes, the bidirectional BLAST of protein sequences with diamond blastp was employed to identify paralogous gene pairs with E-value cutoff =< 1e−20 (Buchfink et al., 2021). The paralogous gene pairs with high similarity were detected in their location on pseudo-molecular chromosomes. The closer paralogous protein-coding genes within the identical genomic region was a tandem array, and the members of the tandem array were tandem duplicated genes in one corresponding genome. Any two tandem duplicated WRKYs within one tandem array were calculated as the ratio of the rates of non-synonymous to synonymous substitutions (Ka/Ks) using KaKs_Calculator 2.0 (Wang et al., 2010).
Analysis of whole-genome duplication in tomato relative to V. vinifera
The MCscanX toolkit was used to detect the orthologous genomic regions between V. vinifera and six Solanum genomes respectively (Wang et al., 2012). Firstly, the BLAST of protein sequences with diamond blastp was employed to identify orthologous gene pairs with E-value cutoff =< 1e−20 between the different genomes (Buchfink et al., 2021). Secondly, the MCscanX toolkit was employed to identify orthologous regions with the parameters (MATCH_SIZE = 5 and E_VALUE = 1e-20) between V. vinifera and six Solanum genomes, respectively. Based on the identified WRKY transcription factors in V. vinifera and six Solanum genomes, the orthologous genomic regions between V. vinifera and six Solanum genomes were parsed to get the WRKY orthologous gene pairs, which might be generated by a WGT event.
Analysis of transcriptomic expression
Transcriptomic data in this study was derived from the NCBI sequence read archive (SRA, bio-project No: PRJNA378182) (Kodama et al., 2012). A total of nine samples with three replicates for each from an experiment set up with three conditions (control, Lso-positive psyllids, Lso-negative psyllids) over three timepoints (Week1, Week2, and Week4) after treatment was adopted to study the roles of WRKYs in response to biotic stresses (CITE) (Huot et al., 2018). Based on released information, all samples were sequenced by the Illumina HiSeq 2500 platform with an average of 24.5 million reads per sample. For each sample, we quantified the count of reads based on gene annotations by salmon pipeline (lib-type: single-end). Reads count from each sample were further normalized using DESeq2 with default settings to eliminate effects of different library sizes before quantification of gene expression (Love et al., 2014). We quantified the relative transcripts abundance by transcripts per million (TPM) and performed the Pearson-correlation analysis over replicates for each sample to confirm the reproducibility of our experiments (Patro et al., 2017). Replicates with low correlation (r < 0.8, p < 0.05) was discarded for analysis. Average TPMs over replicates with high correlation were calculated as respective expression values. To compare the relative expression of WRKYs over time points and treatments, TPMs were further transformed into Z-score for heatmap visualization by the Pheatmap function in R (RRID:SCR_016418).
Results
The larger WRKY gene family in Solanum genomes
High-throughput genome sequencing technology in plants facilitates the analyses of genome evolution and genome-wide identifications of gene families. A. trichopoda was one species of the basal angiosperm without the recent and lineage-specific genome duplications, which provided the basis for studying the evolution of plant polyploidy (DePamphilis et al., 2013). V. vinifera was one species of the rosids have not undergone recent genome duplication after the Eudicot WGT (Jaillon et al., 2007). A. thaliana was the diploid model plant, and its genomic data was interpreted by researchers comprehensively (The Arabidopsis Genome Initiative, 2000). The evolutionary relationship among genomes of S. lycopersicum var. cerasiforme, S. lycopersicum cv. Heinz1706, and S. lycopersicum cv. M82 and their wild relatives, S. lycopersicoides, S. pennellii, S. pimpinellifolium are shown in (Figure 1). Based on the conservation of the DNA binding domain from WRKYs, we identified 33, 64, 48, 88, 77, 80, 85, 85, and 82 WRKYs in A. trichopoda, V. vinifera, A. thaliana, S. lycopersicoides, S. pennellii, S. pimpinellifolium, S. lycopersicum var. cerasiforme, S. lycopersicum cv. Heinz1706, and S. lycopersicum cv. M82, respectively. So, from basal angiosperm to Solanum lineage, the copy numbers of WRKYs increased.
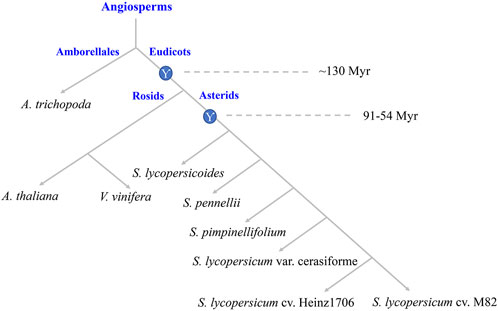
FIGURE 1. Evolutionary relationship and ancestral polyploid events. The circles with Ƴ represent the WGT event of Eudicot ancestor and Solanum lineage.
To better understand the distribution of WRKYs in genome, we characterized the genomic locations of these WRKYs. Among six Solanum genomes, the Chr05 pseudo-molecular chromosome in S. pennellii, S. pimpinellifolium, S. lycopersicum cv. Heinz1706, and S. lycopersicum cv. M82 contained the most WRKY transcription factors compared to the rest pseudo-molecular chromosomes (Table 1). The chr08 contained the most WRKY transcription factors in S. lycopersicoides genome, with chr10 in S. lycopersicum var. cerasiforme genome. Notably, None WRKY was identified in Chr11 pseudo-molecular chromosome for the six Solanum genomes. This is consistent with the previous report. Except that, the previous report identified 83 WRKYs in tomato with complex sequence retrieval from public databases, which had a slight difference in numbers of WRKYs with our analysis. This might be attributed to the update of tomato genome annotation.
Phylogenetic analysis of WRKYs in different genomes
All 642 protein sequences of the identified WRKYs among nine genomes were used to reconstruct the phylogenetic tree. From the phylogeny, all the identified WRKYs were grouped into two different clusters including Cluster1 and Cluster2 (Figure 2). Cluster1 contained Solanum-specific WRKYs group and part of group II of Arabidopsis WRKYs. In Solanum specific WRKY group, there are 4, 9, 15, 16, 15, and 13 WRKYs distributed into S. lycopersicoides, S. pennellii, S. pimpinellifolium, S. lycopersicum var. cerasiforme, S. lycopersicum cv. Heinz1706, and S. lycopersicum cv. M82 genomes, respectively, indicating that these WRKYs might be the products accompanied after the evolution of the Solanum ancestor (Table 2). In total, 72 Solanum specific WRKYs were obtained, which represented 14.5% of total WRKYs (497) in six Solanum genomes. Out of Arabidopsis WRKY Group I, II, and III, Group III contained the most WRKYs (286), representing 44.5% of total WRKYs in A. trichopoda, V. vinifera, A. thaliana, and six Solanum genomes. Interestingly, there is a group mixed with the members of Arabidopsis WRKY Group I, II, and III. This mixed group contained 78 WRKYs distributed into the analyzed nine genomes, representing 11.2% of total WRKYs in A. trichopoda, V. vinifera, A. thaliana, and six Solanum genomes.
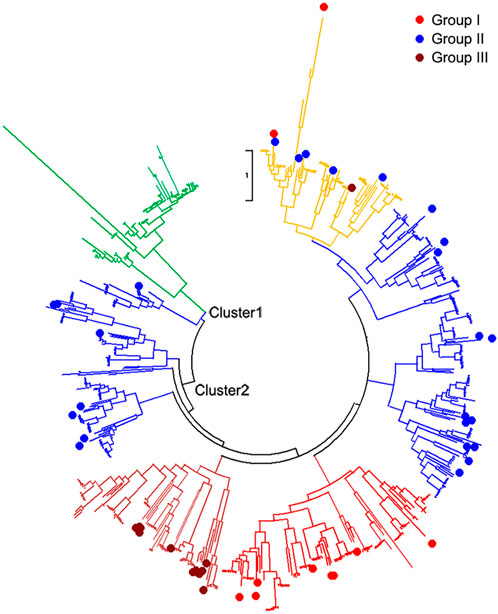
FIGURE 2. Phylogenetic tree of WRKYs in A. trichopoda, V. vinifera, A. thaliana, and six Solanum genomes. Red, blue, and blown colors of tree branches, as well as the corresponding solid circles, represent the group I, group II, and group III of A. thaliana WRKYs group. The green color of tree branches represents the Solanum specific WRKY group. The yellow color of tree branches represents the mixed group of the group I, group II, and group III of A. thaliana WRKYs group. The Cluster1 and Cluster2 within phylogenetic tree represent different clusters.
Evolution of WRKYs in tomato and its wild relatives
A previous report revealed that the Solanum lineage experienced the recent WGT event after the WGT event of Eudicots ancestor shared with rosids, but V. vinifera genome did not experience a WGT event after that (Jaillon et al., 2007). This result demonstrated that six Solanum genomes had three copies of orthologous genomic regions compared with V. vinifera genome, which could detect the ancient gene loci or gene orders in the genomes of tomato and its wild relatives. Thus, we performed the detection of WRKYs derived from the recent WGTs to study the copy-number variations of WRKY in Solanum genomes compared with V. vinifera. We collected 561 identified WRKYs among V. vinifera and six Solanum genomes to detect their evolutionary relationship (Figure 3). The V. vinifera WRKYs exhibited irregular distributions within different WRKY groups, and even grouped together. We compared V. vinifera genome to six Solanum genomes respectively to investigate the effects of WGT for the WRKYs in tomato and its wild relatives. After the implementation of syntenic analysis between the V. vinifera and six Solanum genomes, we obtained 50 orthologous gene pairs between V. vinifera and S. lycopersicoides genomes covering 40 and 50 WRKYs in V. vinifera and S. lycopersicoides, respectively. This result indicated that three copy paralogous WRKYs in S. lycopersicoides genome experienced genes loss after WGT event, along with two three-copies retained, six two-copies retained, and 32 one-copies retained in S. lycopersicoides genome (Table 3). For the comparison between V. vinifera and S. pennellii genomes, we obtained 46 orthologous gene pairs containing 39 and 46 WRKYs in V. vinifera and S. pennellii genomes, which included seven two-copies retained and 33 one-copies retained in S. pennellii genomes. For the comparison between V. vinifera and S. pimpinellifolium genomes, we obtained 39 orthologous gene pairs containing 33 and 39 WRKYs in V. vinifera and S. pennellii genomes, which included one three-copies retained, four two-copies retained, and 28 one-copies retained in S. pennellii genomes. For the three tomatoes, 38, 39, and 38 V. vinifera WRKYs were detected 45, 48, and 45 orthologous WRKYs in S. lycopersicum var. cerasiforme, S. lycopersicum cv. Heinz1706, and S. lycopersicum cv. M82 genomes, respectively.
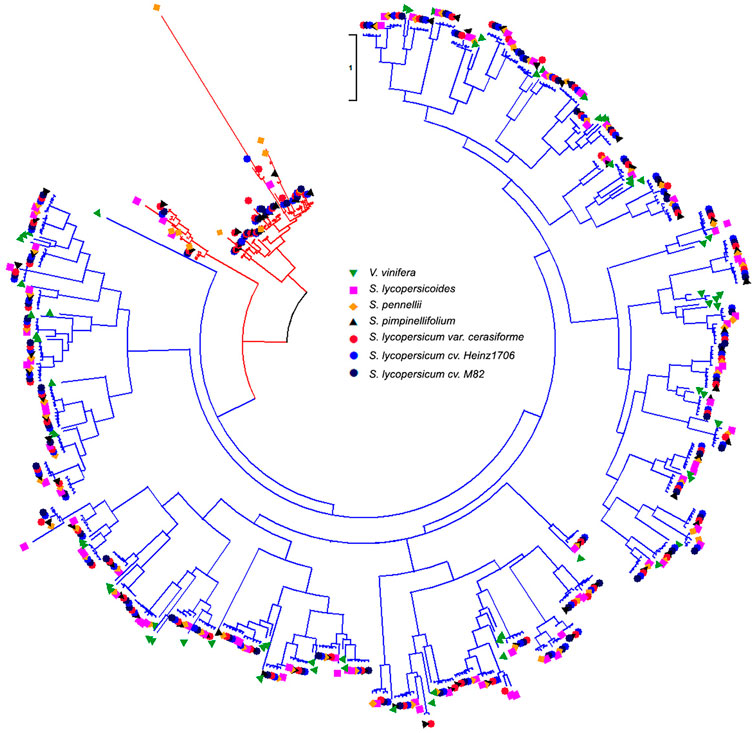
FIGURE 3. Phylogenetic tree of WRKYs in V. vinifera and six Solanum genomes. Red and blue colors of tree branches represent the Solanum specific WRKYs group and the Solanum shared group with V. vinifera. Green lower solid triangle, orange solid square, yellow solid diamond, black upper solid triangle, red solid circle, blue solid circle, and black solid circle represent the WRKYs in V. vinifera, Solanum lycopersicoides, S. pennellii, S. pimpinellifolium, S. lycopersicum var. cerasiforme, S. lycopersicum cv. Heinz1706, and S. lycopersicum cv. M82 genomes.
Effects of TD event for WRKYs in tomato and its relatives
TD event will increase the gene copy number and further lead to the expansion of gene family in plants (Jaillon et al., 2007). To address the expansion of WRKY gene family in tomato and its relatives, we analyzed the influence of TD events on the WRKY gene family in six Solanum species. Combining sequence similarity and gene location, we got 4, 3, 4, 3, 5, and 4 WRKY tandem arrays containing 9, 6, 8, 6, 10, and 8 WRKYs which represented 10.2%, 7.8%, 10%, 7%, 11.8%, and 9.8% of corresponding total WRKYs in S. lycopersicoides, S. pennellii, S. pimpinellifolium, S. lycopersicum var. cerasiforme, S. lycopersicum cv. Heinz1706, and S. lycopersicum cv. M82 genomes, respectively (Table 4). Out of six Solanum genomes, S. pimpinellifolium, S. lycopersicum cv. Heinz1706, and S. lycopersicum cv. M82 genomes only contained two-copies of tandem arrays.
To further investigate the evolution of WRKY tandem arrays, we combined the analyses between the WRKYs from WGT and TD events. In S. lycopersicoides genome, V. vinifera WRKY (Vitvi07g00026) corresponded to a single orthologous gene (Solyd09g065590), which meant that this WRKY only had one copy retained after WGT event (Supplementary Table S1). But this gene (Solyd09g065590) in S. lycopersicoides genome experienced TD event and retained three-copies in a tandem array (Solyd09g065590, Solyd09g065600, and Solyd09g065610) (Supplementary Table S2). Out of the three members of this tandem array, Solyd09g065590 and Solyd09g065610 were all WRKYs, but Solyd09g065600 was a short protein with126 amino-acids and an incomplete WRKY domain. In S. lycopersicum var. cerasiforme genome, V. vinifera WRKY (Vitvi02g00114) had three orthologous genes (SLYcer08g00549, SLYcer08g06424, and SLYcer10g00730), which meant that the orthologous genes of V. vinifera WRKY (Vitvi02g00114) had three copies retained after WGT event. In S. lycopersicum cv. M82 genome, three orthologous genes (Solyc01g095630.3, Solyc05g050330.3, and Solyc10g009550.3) retained after WGT event relative to their ortholog V. vinifera WRKY (Vitvi15g01003). Also, the gene (Solyc05g050330.3) experienced TD event and generated a two-copies tandem array (Solyc05g050330.3 and Solyc05g050340.4).
Through the comparison of tandem duplicated WRKY gene pairs between V. vinifera and six Solanum genomes, we investigated that the tandem duplicated WRKYs in six Solanum genomes did not have the corresponding tandem duplicated genes in V. vinifera genome, which meant that the tandem duplicated WRKYs in six Solanum genomes were generated after the divergence between V. vinifera and Solanum ancestor. To investigate the selection pressures among 47 tandem duplicated WRKYs, we obtained 34 tandem duplicated WRKY gene pairs, and 12 WRKY gene pairs indicated to experience positive selection, with 21 WRKY gene pairs undergoing negative selection (Supplementary Table S3).
Global transcriptome profiling of WRKYs in response to biotic stress
To identify biotic stress-responsive WRKY members in S. lycopersicum cv. Heinz1706, we referred to the publicly available RNAseq datasets including experiments under both biotic stress treatment and control conditions. After data processing, an average of 67.84% of reads were mapped to tomato transcripts using the salmon pipeline (Patro et al., 2017). Filtering of transcripts (counts >0) left 26,391 out of 33,950 transcripts for normalizations which include 63 out of 82 WRKY transcripts from S. lycopersicum cv. Heinz1706 reference genome. Both (variance stabilizing transformation (VSD) and transcripts per million (TPM) quantification based on normalized read counts per library were conducted to estimate the replicability of the experiment, and all samples are in high confidence correlation (r < 0.8) (Supplementary Figure S1). A hierarchical-clustering heatmap based on Z-score transformed TPMs clearly clustered WRKYs with eminent variations of stress responses over timepoints and two treatment types. Particularly, a great portion of WRKYs from Group II up-regulated at the 2 weeks after treatment of Lso-positive psyllids. A small cluster of WRKYs which contains six genes (as shown from the first six rows of heatmap) were identified with pronounced elevated expressions after the first week of Lso-negative psyllids treatment and these small subsets of genes might involve the early response to biotic stresses. And five WRKYs were uniquely up-regulated at the 4 weeks after Lso-positive psyllids treatment, including three WRKYs from the mixed group (Solyc05g015850.4, Solyc08g081630.2, and Solyc02g094270.2), one specific group (Solyc05g050040.3), and one form Group III (Solyc06g048870.3) (Figure 4A). Lastly, a great number of WRKYs exhibited high-level responses both the 2 and 4 weeks after experiments which might be explained by their functional redundancy.
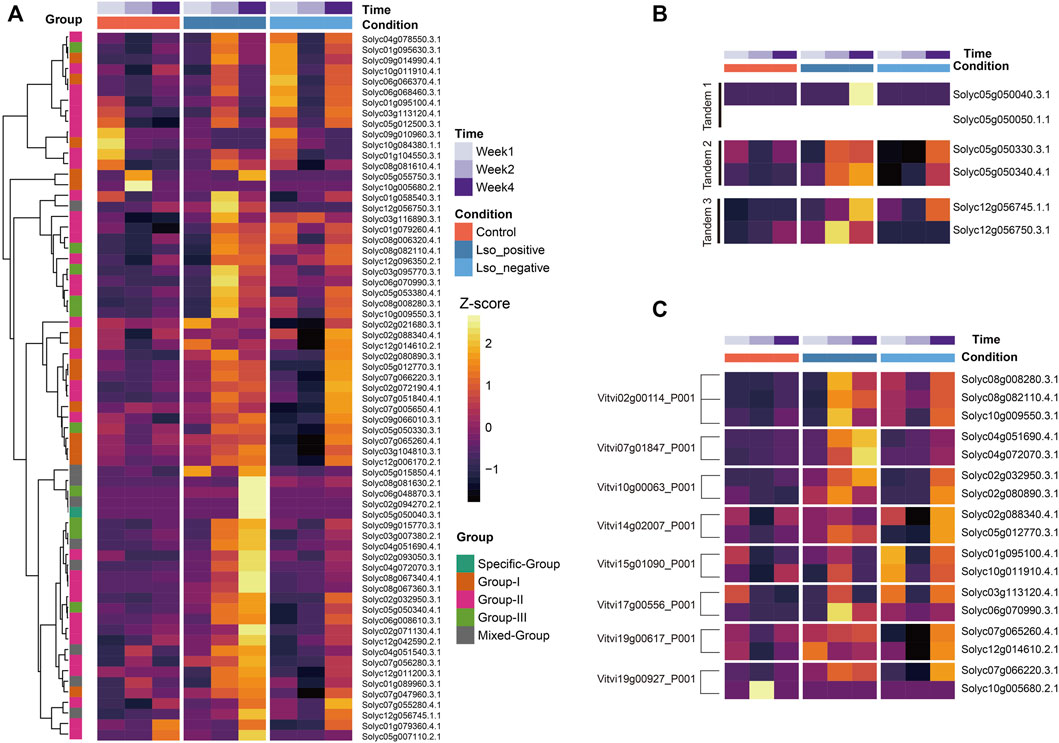
FIGURE 4. Expression profile of WRKYs identified in S. lycopersicum cv. Heinz1706 genome. (A) Hierarchical-Clustering of 62 WRKYs using Z-score transformed TPM data. The meta feature including time, condition, group of WRKYs were marked in legend for reference. (B) Five WRKYs derived from TD event were displayed to compare expression among samples under control and treatment condition. (C) Expression of eight groups of WRKYs paralogs derived from the recent WGT event were displayed in heatmap.
Transcriptional divergence of WRKY paralogs derived from gene duplications
To uncover the evolutionary impacts of gene duplications on gene expression of WRKYs in tomato, we examined the expression profiles of tandem duplicated gene pairs (TD) and genes derived from a WGT event. As especially shown in our selected transcriptome dataset, there were five groups of tandem duplicated genes (10 WRKYs) along with expressions from three groups (Figure 4B). The clustering heatmap of expression clearly exhibited the expression variations between each two tandem duplicated genes. As a contrast with the tandem 1 group, Solyc05g050050.1.1 were completely not expressed among all timepoints and conditions while its tandem duplicated paralog Solyc05g050040.3 highly expressed in response to Lso-positive psyllids treatment at the week 2. We also noticed the variations from tandem group 3 where Solyc12g056750.3 down-regulated at week 2 under the Lso-positive psyllids compared to week 4 under both the Lso-negative/positive psyllids. However, its paralogs Solyc12g056745.1 exhibited an adverse regulation pattern. These expression profile variations implied the functional variations during the expansion of WRKY TFs derived from TD event.
Similarly, we compared the transcription profiles of 17 WRKYs derived from the WGT event (Figure 4C). Among three paralogous WRKYs identified in S. lycopersicum cv. Heinz1706 relative to their V. vinifera ortholog Vitvi02g00114, two WRKYs (Solyc08g008280.3 and Solyc10g009550.3) presented the high-consensus level expression profile while the uniquely elevated expression at the week 4 positive treatment was identified from the rest paralog Solyc08g082110.4. For the rest seven orthologous groups relative to V. vinifera WRKYs, expression variations at the diverse levels in between respective paralogous genes were identified, indicating that both functional redundancy and sub-functionalization occurred during the WRKYs evolution, and both WGT and TD events might be the forces leading to functional divergence among WRKYs in S. lycopersicum cv. Heinz1706.
Discussion
Classification of WRKYs in Solanum lineage
The primary characteristics of WRKY proteins are the DNA-binding domain, which contains the WRKYGQK sequence and a CX4–5CX22–23HXH zinc binding (Bakshi and Oelmüller, 2014). Based on the numbers of the WRKY domain, WRKY proteins could be divided into different groups. WRKY proteins from group I are encoded by two WRKY domains and only one domain was found from the remaining two WRKY groups. These two groups were further classified based on the presence of C2-H2 (C-X4-5-CX22-23-H-X1-H) motif from group II and the C2-HC (C-X7-C-X23-H-X-C) zinc finger motif from group III (Li et al., 2011). The WRKY gene family was illustrated comprehensively in A. thaliana (Rushton et al., 2010). In this project, we combined the phylogeny analysis with full-length protein sequences and the classification of WRKY groups in A. thaliana to classify the WRKY groups in Solanum lineage. Generally, highly conserved WRKYs will group together, and perform similar or identical molecular functions, belonging to the same WRKY group. So, the Solanum WRKYs were clustered together with A. thaliana WRKY groups I, II, and III, named Solanum WRKYs groups I, II, and III. In Cluster1, there is a group without A. thaliana WRKYs, and we named it a Solanum specific group. In Cluster2, the Solanum WRKYs were clustered together with the members of A. thaliana WRKY groups I, II, and III, and this group was named as a mixed group.
Expansion or loss of WRKY gene family in tomato and its wild relatives
From a comparison between V. vinifera and six Solanum genomes, we got 50, 46, 39, 45, 48, and 45 WRKYs representing 56.82%, 59.74%, 48.75%, 52.94%, 56.47%, and 54.88% of corresponding total WRKYs in S. lycopersicoides, S. pennellii, S. pimpinellifolium, S. lycopersicum var. cerasiforme, S. lycopersicum cv. Heinz1706, and S. lycopersicum cv. M82 genomes, respectively. From the analysis of TD events for WRKYs in three tomatoes and their three wild relatives, we found that 10.2%, 7.8%, 10%, 7%, 11.8%, and 9.8% of total WRKYs in S. lycopersicoides, S. pennellii, S. pimpinellifolium, S. lycopersicum var. cerasiforme, S. lycopersicum cv. Heinz1706, and S. lycopersicum cv. M82 genomes respectively were generated by the TD event. These results demonstrated that the WGT event played an important role in the expansion of WRKY gene family in Solanum lineage. Phylogeny analysis of nine species revealed that Cluster1 contained Solanum-specific WRKY group, which meant that these WRKYs were generated after the WGT event of the Eudicot ancestor, leading to the expansion of WRKY gene family in six Solanum genomes. In the syntenic analysis between V. vinifera and six Solanum genomes, 44 WRKYs of 64 V. vinifera WRKYs were detected as orthologous WRKYs in Solanum lineage, which meant that the orthologous WRKYs of 20 V. vinifera WRKYs totally lost in six Solanum genomes after the WGT event of Eudicot ancestor. So, the WRKY gene family in Solanum genomes experienced complex retention or loss and formed the current WRKY gene family in Solanum lineage.
Conclusion
Based on the highly conserved domain of WRKY gene family, we identified 33, 64, 48, 88, 77, 80, 85, 85, and 82 WRKY transcription factors in A. trichopoda, V. vinifera, A. thaliana, S. lycopersicoides, S. pennellii, S. pimpinellifolium, S. lycopersicum var. cerasiforme, S. lycopersicum cv. Heinz1706, and S. lycopersicum cv. M82, respectively. Through the analysis of WRKY distribution on Solanum genomes, there is no WRKY located on Chr11 pseudo-molecular chromosome in six Solanum genomes. Phylogenetic analysis indicated that all the WRKYs among nine genomes were divided into two different clusters, Cluster1 and Cluster2. These two clusters contained 194 and 448 WRKYs representing 30.22% and 69.78% of total WRKYs in nine genomes. From the analysis of WGT and TD events, we found that the WGT event brought a stronger influence on the expansion of the WRKY gene family in Solanum lineage. Expression analyses revealed that the expressed WRKYs in S. lycopersicum cv. Heinz1706 grouped into two clusters, and the kinds of paralogous WRKYs generated by WGT and TD events showed different expression patterns. This project is the first to illustrate the evolutionary history and expression characteristics of WRKYs in Solanum lineage, which will provide a novel view to study the expansion or loss of the WRKY gene family, and expression divergence of duplicated WRKYs in community.
Data availability statement
The datasets presented in this study can be found in online repositories. The names of the repository/repositories and accession number(s) can be found in the article/Supplementary Material.
Author contributions
XX and TZ conceived the project. GL analyzed the data and prepared the manuscript. GL, DZ, HY, JL, and JJ performed phylogenetic analysis and revised the draft manuscript. HZ revised the manuscript. All authors read and approved the final manuscript.
Funding
This work was supported by the Natural Science Outstanding Youth Foundation of Heilongjiang Province (YQ 2020C038) and the Basic Scientific Research Foundation of Heilongjiang Provincial universities (2020-KYYWF-1048).
Conflict of interest
The authors declare that the research was conducted in the absence of any commercial or financial relationships that could be construed as a potential conflict of interest.
Publisher’s note
All claims expressed in this article are solely those of the authors and do not necessarily represent those of their affiliated organizations, or those of the publisher, the editors and the reviewers. Any product that may be evaluated in this article, or claim that may be made by its manufacturer, is not guaranteed or endorsed by the publisher.
Supplementary material
The Supplementary Material for this article can be found online at: https://www.frontiersin.org/articles/10.3389/fgene.2022.962975/full#supplementary-material
Abbreviations
HMM, Hidden Markov model; ML, Maximum likelihood; Myr, Million years; TD, tandem duplication; WGT, whole-genome triplication; WRKYs, WRKY transcription factors.
References
Ayadi, M., Hanana, M., Kharrat, N., Merchaoui, H., Marzoug, R. B., Lauvergeat, V., et al. (2016). The WRKY transcription factor family in citrus: Valuable and useful candidate genes for citrus breeding. Appl. Biochem. Biotechnol. 180, 516–543. doi:10.1007/s12010-016-2114-8
Bakshi, M., and Oelmüller, R. (2014). Wrky transcription factors jack of many trades in plants. Plant Signal. Behav. 9, e27700. doi:10.4161/psb.27700
Bolger, A., Scossa, F., Bolger, M. E., Lanz, C., Maumus, F., Tohge, T., et al. (2014). The genome of the stress-tolerant wild tomato species Solanum pennellii. Nat. Genet. 46, 1034–1038. doi:10.1038/ng.3046
Buchfink, B., Reuter, K., and Drost, H. G. (2021). Sensitive protein alignments at tree-of-life scale using DIAMOND. Nat. Methods 18, 366–368. doi:10.1038/s41592-021-01101-x
Cheng, C. Y., Krishnakumar, V., Chan, A. P., Thibaud-Nissen, F., Schobel, S., and Town, C. D. (2017). Araport11: a complete reannotation of the Arabidopsis thaliana reference genome. Plant J. 89, 789–804. doi:10.1111/tpj.13415
DePamphilis, C. W., Palmer, J. D., Rounsley, S., Sankoff, D., Schuster, S. C., Ammiraju, J. S. S., et al. (2013). The Amborella genome and the evolution of flowering plants. Science 342, 1241089. doi:10.1126/science.1241089
Fernandez-Pozo, N., Menda, N., Edwards, J. D., Saha, S., Tecle, I. Y., Strickler, S. R., et al. (2015). The Sol Genomics Network (SGN)-from genotype to phenotype to breeding. Nucleic Acids Res. 43, D1036–D1041. doi:10.1093/nar/gku1195
He, G. H., Xu, J. Y., Wang, Y. X., Liu, J. M., Li, P. S., Chen, M., et al. (2016). Drought-responsive WRKY transcription factor genes TaWRKY1 and TaWRKY33 from wheat confer drought and/or heat resistance in Arabidopsis. BMC Plant Biol. 16, 116–16. doi:10.1186/s12870-016-0806-4
Howe, K. L., Contreras-Moreira, B., De Silva, N., Maslen, G., Akanni, W., Allen, J., et al. (2020). Ensembl Genomes 2020-enabling non-vertebrate genomic research. Nucleic Acids Res. 48, D689–D695. doi:10.1093/nar/gkz890
Huot, O. B., Levy, J. G., and Tamborindeguy, C. (2018). Global gene regulation in tomato plant (Solanum lycopersicum) responding to vector (Bactericera cockerelli) feeding and pathogen (‘Candidatus Liberibacter solanacearum’) infection. Plant Mol. Biol. 97, 57–72. doi:10.1007/s11103-018-0724-y
Hussain, A., Noman, A., Khan, M. I., Zaynab, M., Aqeel, M., Anwar, M., et al. (2019). Molecular regulation of pepper innate immunity and stress tolerance: An overview of WRKY TFs. Microb. Pathog. 135, 103610. doi:10.1016/j.micpath.2019.103610
Jaillon, O., Aury, J. M., Noel, B., Policriti, A., Clepet, C., Casagrande, A., et al. (2007). The grapevine genome sequence suggests ancestral hexaploidization in major angiosperm phyla. Nature 449, 463–467. doi:10.1038/nature06148
Jones, P., Binns, D., Chang, H. Y., Fraser, M., Li, W., McAnulla, C., et al. (2014). InterProScan 5: Genome-scale protein function classification. Bioinformatics 30, 1236–1240. doi:10.1093/bioinformatics/btu031
Joshi, R., Wani, S. H., Singh, B., Bohra, A., Dar, Z. A., Lone, A. A., et al. (2016). Transcription factors and plants response to drought stress: Current understanding and future directions. Front. Plant Sci. 7, 1029–1115. doi:10.3389/fpls.2016.01029
Kodama, Y., Shumway, M., and Leinonen, R. (2012). The sequence read archive: Explosive growth of sequencing data. Nucleic Acids Res. 40, D54–D56. doi:10.1093/nar/gkr854
Kumar, S., Stecher, G., Li, M., Knyaz, C., and Tamura, K. (2018). MEGA X: Molecular evolutionary genetics analysis across computing platforms. Mol. Biol. Evol. 35, 1547–1549. doi:10.1093/molbev/msy096
Li, S., Fu, Q., Chen, L., Huang, W., and Yu, D. (2011). Arabidopsis thaliana WRKY25, WRKY26, and WRKY33 coordinate induction of plant thermotolerance. Planta 233, 1237–1252. doi:10.1007/s00425-011-1375-2
Love, M. I., Huber, W., and Anders, S. (2014). Moderated estimation of fold change and dispersion for RNA-seq data with DESeq2. Genome Biol. 15, 550–621. doi:10.1186/s13059-014-0550-8
Meng, D., Li, Y., Bai, Y., Li, M., and Cheng, L. (2016). Genome-wide identification and characterization of WRKY transcriptional factor family in apple and analysis of their responses to waterlogging and drought stress. Plant Physiol. Biochem. 103, 71–83. doi:10.1016/j.plaphy.2016.02.006
Mistry, J., Chuguransky, S., Williams, L., Qureshi, M., Salazar, G. A., Sonnhammer, E. L. L., et al. (2021). Pfam: The protein families database in 2021. Nucleic Acids Res. 49, D412–D419. doi:10.1093/nar/gkaa913
Patro, R., Duggal, G., Love, M. I., Irizarry, R. A., and Kingsford, C. (2017). Salmon provides fast and bias-aware quantification of transcript expression. Nat. Methods 14, 417–419. doi:10.1038/nmeth.4197
Powell, A. F., Feder, A., Li, J., Schmidt, M. H. ‐W., Courtney, L., Alseekh, S., et al. (2022). A Solanum lycopersicoides reference genome facilitates insights into tomato specialized metabolism and immunity. Plant J. 110, 1791–1810. doi:10.1111/tpj.15770
Rushton, P. J., Somssich, I. E., Ringler, P., and Shen, Q. J. (2010). WRKY transcription factors. Trends Plant Sci. 15, 247–258. doi:10.1016/j.tplants.2010.02.006
Sato, S., Tabata, S., Hirakawa, H., Asamizu, E., Shirasawa, K., Isobe, S., et al. (2012). The tomato genome sequence provides insights into fleshy fruit evolution. Nature 485, 635–641. doi:10.1038/nature11119
Shi, W., Liu, D., Hao, L., Wu, C. A., Guo, X., and Li, H. (2014). GhWRKY39, a member of the WRKY transcription factor family in cotton, has a positive role in disease resistance and salt stress tolerance. Plant Cell Tissue Organ Cult. 118, 17–32. doi:10.1007/s11240-014-0458-8
Takei, H., Shirasawa, K., Kuwabara, K., Toyoda, A., Matsuzawa, Y., Iioka, S., et al. (2021). De novo genome assembly of two tomato ancestors, Solanum pimpinellifolium and Solanum lycopersicum var. cerasiforme, by long-read sequencing. DNA Res. 28, dsaa029–9. doi:10.1093/dnares/dsaa029
The Arabidopsis Genome Initiative (2000). Analysis of the genome sequence of the flowering plant Arabidopsis thaliana. Nature 408, 796–815. doi:10.1038/35048692
Wang, D., Zhang, Y., Zhang, Z., Zhu, J., and Yu, J. (2010). KaKs_Calculator 2.0: a toolkit incorporating gamma-series methods and sliding window strategies. Genomics Proteomics Bioinforma. 8, 77–80. doi:10.1016/S1672-0229(10)60008-3
Wang, Y., Tang, H., Debarry, J. D., Tan, X., Li, J., Wang, X., et al. (2012). MCScanX: A toolkit for detection and evolutionary analysis of gene synteny and collinearity. Nucleic Acids Res. 40, e49–14. doi:10.1093/nar/gkr1293
Wang, X., Yan, Y., Li, Y., Chu, X., Wu, C., and Guo, X. (2014). GhWRKY40, a multiple stress-responsive cotton WRKY gene, plays an important role in the wounding response and enhances susceptibility to Ralstonia solanacearum infection in transgenic Nicotiana benthamiana. PLoS One 9, e93577. doi:10.1371/journal.pone.0093577
Wang, X., Gao, L., Jiao, C., Stravoravdis, S., Hosmani, P. S., Saha, S., et al. (2020). Genome of Solanum pimpinellifolium provides insights into structural variants during tomato breeding. Nat. Commun. 11, 5817. doi:10.1038/s41467-020-19682-0
Wei, K. F., Chen, J., Chen, Y. F., Wu, L. J., and Xie, D. X. (2012). Molecular phylogenetic and expression analysis of the complete WRKY transcription factor family in maize. DNA Res. 19, 153–164. doi:10.1093/dnares/dsr048
Wei, W., Hu, Y., Han, Y. T., Zhang, K., Zhao, F. L., and Feng, J. Y. (2016). The WRKY transcription factors in the diploid woodland strawberry Fragaria vesca: Identification and expression analysis under biotic and abiotic stresses. Plant Physiol. Biochem. 105, 129–144. doi:10.1016/j.plaphy.2016.04.014
Keywords: genome-wide, WRKY transcription factor, tomato, whole genome duplication, tandem duplication, expression divergence
Citation: Liu G, Zhang D, Zhao T, Yang H, Jiang J, Li J, Zhang H and Xu X (2022) Genome-wide analysis of the WRKY gene family unveil evolutionary history and expression characteristics in tomato and its wild relatives. Front. Genet. 13:962975. doi: 10.3389/fgene.2022.962975
Received: 07 June 2022; Accepted: 18 July 2022;
Published: 15 September 2022.
Edited by:
Zefeng Yang, Yangzhou University, ChinaReviewed by:
A. Anicet Batcho, Academy of Sciences of the Czech Republic, CzechiaHui Liu, Chinese Academy of Tropical Agricultural Sciences, China
Copyright © 2022 Liu, Zhang, Zhao, Yang, Jiang, Li, Zhang and Xu. This is an open-access article distributed under the terms of the Creative Commons Attribution License (CC BY). The use, distribution or reproduction in other forums is permitted, provided the original author(s) and the copyright owner(s) are credited and that the original publication in this journal is cited, in accordance with accepted academic practice. No use, distribution or reproduction is permitted which does not comply with these terms.
*Correspondence: Xiangyang Xu, xxy709@126.com