- International Center for Biosaline Agriculture (ICBA), Dubai, United Arab Emirates
As one of the oldest fruit trees of the Arabian peninsula, other Middle-Eastern countries, and also North Africa, the date palm (Phoenix dactylifera L.), is highly significant for the economy of the region. Listed as part of UNESCO’s Intangible Cultural Heritage of Humanity, the date palm is believed to be the first tree cultivated by human beings, and was probably first harvested for its fruit nearly 7,000 years ago. Initial research efforts in date palm genetics focused on understanding the genetic diversity of date palm germplasm collections and its phylogenetic history, both important prerequisites for plant improvement. Despite various efforts, the center of origin of the date palm is still unclear, although genomic studies suggest two probable domestication events: one in the Middle East and the other in North Africa, with two separate gene pools. The current review covers studies related to omics analyses that have sought to decipher the present genetic diversity of the date palm. With advances and cost reductions in sequencing technologies, rapid progress has been made in the past few years in date palm genomics research. Along with organellar genomes, several reference genomes of the date palm are now available. In addition, several genotypes have been re-sequenced, either to detect single nucleotide polymorphisms (SNPs), or to study domestication and identification of key genes/loci associated with important agronomic traits, such as sex, fruit color, and sugar composition. These genomics research progress has paved the way to perform fast-track and precise germplasm improvement processes in date palm. In this study, we review the advances made in the genetics and genomics of the date palm so as to strategize targeted crop improvement plans for marginal areas of the Middle Eastern peninsula, North Africa, and other parts of the world.
Introduction
Date palm (Phoenix dactylifera L.) is one of the oldest fruit trees in the Arabian Peninsula (AP), other countries of the Middle East, and the arid regions of North Africa. Its origin is not yet known; however, evidence indicates this was possibly near Iraq. In the AP, the date palm holds cultural importance for the people, besides being a critically important staple food and a major source of income. It is known for multiple products and purposes, including fruit, fiber, fuel, and sheltering material. In addition to providing calories, dates serve as a source of vitamins and minerals (El Hadrami and Al-Khayri, 2012), rendering them a healthy and nutritious calorie option. The global trade in dates was valued at about USD $1.2 billion in 2016, thereby contributing to the livelihood and income of millions of rural smallholders in the AP and surrounding areas [FAO: Microsoft Word - Conference-side-event-Dates-Saudi-Arabia.docx (fao.org)]. Globally, date palms are cultivated on 1.1 million hectares of land with a production of about 8.7 million tons (FAOSTAT, 2018). Iran, Algeria, Iraq, Saudi Arabia, and Egypt share 59% of the total harvested area and 66.5% of the total production, with maximum yield coming from Egypt (Table 1) (FAOSTAT, 2020). The area under date palm cultivation has also increased continuously during recent decades because of the crop’s adaptability to the harsh climate.
Despite being one of the most successful fruit crops in arid, semiarid, tropical, and subtropical regions, there has been relatively little research into the genetics and molecular genetics of the date palm compared to other commercial fruit trees. Genomics approaches are an exception here, and rapid advances have been made in the past decade. So far, the date palm genome, along with its organellar genomes, has been sequenced (Al-Dous et al., 2011; Fang et al., 2012; Khan et al., 2012; Al-Mssallem et al., 2013; Asaf et al., 2018). Several genotypes have been re-sequenced, either to detect single nucleotide polymorphisms (SNPs) (Thareja et al., 2018), or to study domestication and marker-trait association (Hazzouri et al., 2015, 2019; Gros-Balthazard et al., 2017). In addition to SNPs, other marker resources have been used in the past for diversity studies and the identification of cultivars, including random amplified polymorphic DNA (RAPD), inter simple sequence repeats (ISSR), simple sequence repeats (SSR), and amplified fragment length polymorphisms (AFLP), etc. Early sex deteremnation is an important trait in the date palm. Because the date palm is dioecious in nature, efforts have been made to develop specific markers for early detection of the female plant. Molecular markers have also been developed for brittle leaf disease (BLD) resistance in the tree. In the recent past, red palm weevil (Rhynchophorus ferrugineus Olivier) has had a devastating effect on date palm yields in the Arabian Peninsula (Kassem et al., 2020). At the International Center for Biosaline Agriculture (ICBA), UAE, efforts are underway to address this biotic stress with the help of advanced genomics tools (ICBA, unpublished).
Although there has been little progress in genomics applications in date palm improvement, this study represents an effort to review what progress has been made, as well as identify the future prospects for date palm genomics, given the importance of this crop for the livelihood of vast numbers of farmers in the AP.
Botanical description of date palm
The date palm (Phoenix dactylifera L.) is a perennial monocotyledonous plant belonging to the family Arecaceae (Palmae). Mature date palm plants are the tallest among Phoenix spp. and can attain heights as tall as 25–30 m, with a single main terminal shoot apex for linear growth. The date palm has a well-developed fibrous root system in which primary roots develop directly from the seeds/tree trunk with an average length of 4–6 m. The lateral roots originate from primary roots, which further produce lateral roots throughout their length. All date palm roots contain pneumatics, which act as respiratory organs. The plant contains pinnate leaves arranged alternately along the trunk. An adult date palm plant contains 100–125 leaves, with 40% juvenile, 10% fast-growing, and 50% photosynthetically active (Zaid and De Wet, 1999). The date palm is dioecious in nature, with separate trees producing male and female flowers in clusters called spadixes or spikes, within axils of leaves of the growth of previous years. Rarely, both pistillate and staminate flowers are produced on the same spike, and hermaphrodite flowers have also been reported in the inflorescence (Mason, 1915; Milne, 1918), although in very few cases. The staminate flowers are sweet-scented and generally have six stamens, each composed of two little pollen sacs. The stamens are surrounded by three waxy sepals and petals. The female flowers contain rudimentary stamens and are tricarpellate, consisting of three carpels that are closely pressed together and surrounded by a short perianth with a superior ovary (Figure 1). Pollination occurs by wind, or artificially, by dusting pollen grains collected from male spikelets onto female inflorescences. The fruit normally develops after fertilization from one carpel, which develops faster, while the other two carpels degenerate and drop later. The development of seeded fruit follows a sigmoidal curve with four distinct ripening stages: kimri, khalal (also know as bisir/bisr), rutab, and tamer stages (the names being of Arabic origin), which represent immature green, mature full-colored, soft brown, and hard raisin-like stages, respectively, containing average moisture content of 80%, 60%, 40%, and 20%, respectively (Fayadh and Al-Showiman, 1990; Al-Shahib and Marshall, 2003). The date fruit varies in size and shape depending on the cultivar and environment. With advancement in the developmental stages of the fruit, antioxidant activity increases until bisir and then decreases (Awad et al., 2011; Mohamed Lemine et al., 2014), whereas sugar content increases with ripening in the date palm fruit (Al-Mssallem et al., 2013) (Figure 1).
Distribution and biodiversity
The date palm is one of the earliest cultivated fruit trees, with records of its cultivation in the areas of the Euphrates and Nile rivers going back to 3700 BC; while in Iran, Egypt, and Pakistan, the earliest records go back 7,000 years (Munier, 1973). The exact center of origin of the date palm is not certain; however, it is believed to have originated from the modern Iraqi region of Mesopotamia (Wrigley, 1995). Interestingly, the oldest seeds of palm, dating back to 5110 BC and 4670 BC, were reported from an island of Abu Dhabi known as Dalma (Rhouma et al., 2010). One of two wild species, Phoenix reclinata Jacq. from tropical Africa, or P. sylvestris (L.) Roxb. from India, or a hybrid of these two, is believed to be the progenitor of the date palm. However, many researchers believe that the tree originates from the Mesopotamian-Arabian Gulf area (Zohary and Hopf, 2000; Tengberg, 2012) and was later introduced into North Africa. However, genomic studies suggest that the genotypes from North Africa and the Middle East are genetically distinct, with higher genetic diversity in the North African date palm population (Hazzouri et al., 2015). Along with archaeological records, the population structure suggests two probable domestication sources in the date palm, one from the Middle East and the other from North Africa, forming two separate gene pools that diverged before domestication (Hazzouri et al., 2015; Zehdi-Azouzi et al., 2015). Also, the presence of admixed genotypes suggests that gene flow occurred between populations of eastern and western origins, primarily from east to west, as a result of human-mediated dispersal of the species after domestication (Zehdi-Azouzi et al., 2015). Whole-genome sequencing of wild and cultivated date palms reveals a complex domestication history with the contribution of at least two wild sources to the African cultivated palms (Gros-Balthazard et al., 2017). The date palm is thought to have spread globally in two directions: one from Mesopotamia to Iran, India, and Pakistan, and the other from Egypt toward Libya and the countries of the Maghreb and Sahel (Racchi and Camussi, 2018). The date palm is now more abundant in the arid regions of the Old World than in the temperate regions of the New World, with the most date palm trees found in Middle Eastern countries (Iraq, Iran, Saudi Arabia, United Arab Emirates, Oman, Yemen, etc.), followed by Africa (Algeria, Egypt, Libya, Mali, Morocco, Mauritania, Niger, Somalia, Sudan, Chad, Tunisia, etc.) (FAOSTAT, 2019). Apart from the Middle East and Africa, with the expansion of Islam the date palm has also been introduced into the United States and Europe (Chao and Krueger, 2007; Rivera et al., 2013).
The genus Phoenix consists of 12 closely-related species, making them cross-compatible for natural hybridization (Moore, 1963; Munier, 1973). Several natural hybrids were obtained from different countries: P. dactylifera × P. sylvestris (India), P. dactylifera × P. canariensis (Morocco, Algeria, and Israel), and P. dactylifera × P. reclinata (Senegal).
The date palm is generally diploid in nature, with 2n = 36 chromosomes (Darlington and Wylie, 1956), although polyploidy has been reported in Iraqi varieties (n = 64) (Panga, 2014). Also, differences in chromosome numbers have been observed between varieties Sayer (2n = 32) and Khasab (2n = 36). Al Salih et al. (1987) reported 2n = 32, 34, 36, and 64 date palm chromosomes. It would be interesting to investigate the crossability among accessions with different ploidy levels for creating newer genetic variations.
Molecular marker-assisted genetic diversity in the date palm
Genetic diversity refers to the genetic variability present within species, subspecies, cultivars, or populations, and can be measured at the morphological, physiological, biochemical, or molecular levels. A total of 3,000–5,000 date palm cultivars exist globally. The cultivar diversity and production indices across major date palm growing countries are presented in Table 1 (Abul-Soad et al., 2015; Al-Yahyai and Al-Khanjari, 2008; Al-Yahyai and Khan, 2015; Ba-Angood, 2015; Bashah, 1996; Battaglia et al., 2015; Bouguedoura et al., 2015; Elshibli, 2009; Hajian and Hamidi-Esfahani, 2015; Mahar, 2007; Osman, 1984; Rabei et al., 2012; Sedra, 2015; Zabar and Borowy, 2012; Zaid and De Wet, 1999; FAOSTAT, 2020). Despite collections of traditionally cultivated genotypes, duplications have also been reported among them. Therefore, approximately only 10% of the total cultivars existing globally are believed to be unique and commercially important (Johnson, 2011). The commercialization of preferred date palm cultivars prompted an increase in area of cultivation, thereby enhancing the practice of monoculture, which ultimately led to a significant decline in genetic (or species) diversity in the date palm. Characterizing, conserving, and using date palm collections globally is one of the felt needs that can be effectively met using high-density genomics approaches. In recent years, initiatives aimed at characterizing large collections of gene banks have succeeded. As an example, 100,000 wheat and 30,000 maize accessions of the gene bank of the International Maize and Wheat Improvement Center (CIMMYT) were characterized (Seeds of Discovery: Unlocking the genetic potential of maize and wheat). This sort of approach could provide a suitable option for characterizing date palm genetic resources globally.
Research into developing biochemical and molecular markers for date palms began in the late 1970s, and was later used for genetic diversity analysis. Various isozyme markers were used to study the inheritance of multiple traits in date palm seedlings (Torres and Tisserat, 1980) and genetic diversity (Bennaceur et al., 1991), or to develop a cultivar identification system (Bendiab et al., 1998) for date palms. Advances in molecular biology, and the development of a PCR-based marker system during the 1990s led to studies using various molecular marker systems, either individually or in combination, to unravel the genetic diversity and phylogenetics of the date palm. Initially, DNA-based marker systems, such as RFLP and RAPD, were used to identify polymorphic markers associated with date palm cultivars (Corniquel and Mercier, 1994). Even though RAPD markers have low reproducibility, they have been used to study the genetic diversity in various date palm accessions in different countries (Table 2). Other types of dominant multi-locus markers, such as ISSR markers on their own or in combination with RAPD markers, have also been used in genetic diversity analysis of the date palm (Table 2). Genetic diversity analyses using RAPD or ISSR markers, or a combination of both, have shown that huge genetic similarity (more than 90%) exists among various date palm genotypes. A comparison between four female date palm trees and four unknown male trees of the Egyptian date palm, using RAPD markers, shows that genetic similarity existed not only between female cultivars (87.5%–98.9%), but also between unknown male trees (88.9%–95.3%) (Soliman et al., 2003). A more reproducible multi-locus marker, AFLP, has been used either alone, or in combination with other marker systems, to assess genetic variations present in date palm cultivars across the globe. Initially, an attempt was made to develop a genetic map using AFLP markers with a population derived from Um-Assla and KL-96 (El-Kharbotly et al., 1998). Later, either AFLP or fluorescently-labeled AFLP primers were used to study genetic diversity among various accessions of the date palm, with genetic similarity ranging from approximately 10%–75% (Cao and Chao, 2002; Devanand and Chao, 2003a, 2003b; El-Khishin et al., 2003; El-Assar et al., 2005; Jubrael et al., 2005; Elhoumaizi et al., 2006; Rhouma et al., 2007; Khierallah H. et al., 2011, Khierallah et al., 2011 H. S.). The AFLP markers were also used to assess intra-varietal differences (El-Assar et al., 2005; Elhoumaizi et al., 2006), and to study the genetic fidelity of plants raised in vitro (Diaz et al., 2003; Al Kaabi et al., 2007). Apart from being used to study diversity, these multi-locus markers have also been used for cultivar identification (Table 2) (Corniquel and Mercier 1994; Al-Moshileh et al., 2004; Sabir J. S. et al., 2014).
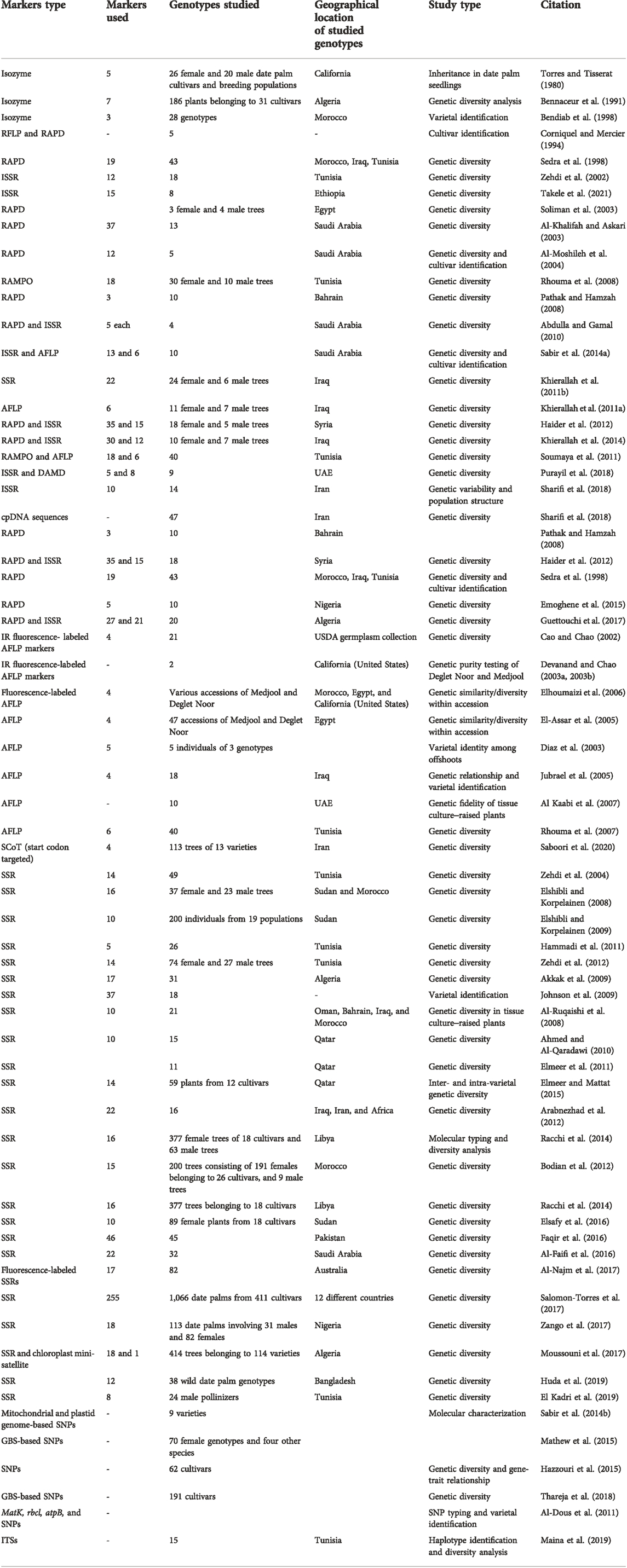
TABLE 2. Application of biochemical and molecular markers in genetic diversity studies of the date palm.
Because of their abundance and dispersion throughout the genome, their co-dominance nature, ease of usage, and ability to automate, microsatellites or simple sequence repeats (SSR) have proven an ideal choice for cultivar identification and genetic diversity analysis, as well as for linkage and QTL mapping, and marker-assisted breeding. Billotte et al. (2004) made the first attempt to develop SSR markers for date palms using a (GA)n-enriched library. They further designed 16 SSR primers, and tested their amplification on 40 DNA samples of P. dactylifera from various origins, as well as on 11 other species of Phoenix. Later, several attempts were made to identify and develop SSR markers for date palms using a genomic DNA library enriched for microsatellite sequences (Akkak et al., 2009; Arabnezhad et al., 2012; Al-Faifi et al., 2016). Apart from using microsatellite-enriched libraries, available sequence information, such as ESTs, have been used to develop EST-SSRs as well as gene-based SSRs, and they have been characterized with their functional annotations (Zhao et al., 2012, 2017). With the availability of various draft assemblies of date palm genomes, "genome sequence information has been used to develop 1090 SSRmarkers (Hamwieh et al., 2010). Using the draft genome sequence of the date palm, Mokhtar et al. (2016) identified 172,075 SSR motifs, with a frequency of 450.97 SSRs per Mb. A total of 130,014 SSRs (75.6%) were located within the intergenic regions, while 42,061 SSRs (24.4%) were located in the genic regions. Furthermore, 111,403 SSR primer pairs were designed, with a density of 291.9 SSR primers per Mb.
Numerous genetic diversity analyses have been conducted with the help of microsatellite markers in different countries: Tunisia, Qatar, Libya, Morocco, Sudan, Pakistan, Saudi Arabia, Niger, Algeria, Sudan, the United States (California), Australia, etc. (Zehdi et al., 2004, 2012; Al-Ruqaishi et al., 2008; Elshibli and Korpelainen, 2008, 2009; Akkak et al., 2009; Ahmed and Al-Qaradawi, 2010; Hamwieh et al., 2010; Hammadi et al., 2011; Bodian et al., 2014; Racchi et al., 2014; Elmeer and Mattat, 2015; Al-Faifi et al., 2016; Elsafy et al., 2016; Faqir et al., 2016; Al-Najm et al., 2017; Moussouni et al., 2017; Zango et al., 2017; El Kadri et al., 2019) (Table 2).
Salomon-Torres et al. (2017) reviewed the performance of 255 SSR markers for studying diversity among 1,066 date palm plants from 411 cultivars in 12 countries, and recommended a set of 19 SSR markers as useful for further genetic diversity analysis. Recently, studies have looked into the genetic diversity of worldwide date palm germplasm accessions, using SSRs (Chaluvadi et al., 2014; Zehdi-Azouzi et al., 2015; Salomon-Torres et al., 2017), SNPs (Hazzouri et al., 2015; Mathew et al., 2015), or comparisons of whole genomes (Hazzouri et al., 2015). Through sequencing of 62 varieties of date palms from 12 countries, Hazzouri et al. (2015) show that Middle Eastern genotypes form a separate group from North African genotypes, with North African genotypes having higher nucleotide diversity than Middle Eastern/South Asian genotypes. Similar results were obtained by Mathew et al. (2015), where the authors used the sequence data from 70 date palm accessions. Such accessions are mostly propagated through tissue culture; however, variations among accessions with the same name suggest that somaclonal mutation is ongoing during the process of subculturing during tissue culture. Under some circumstances, huge genetic variations within the same accession suggest that, since the date palm is dioecious in nature, there is a probability of random crossing events in addition to the controlled outcrossing, and the plants might have been raised from the seeds, resulting in an increase in the genetic distance between the genotypes.
Apart from their use in genetic diversity analysis, SSR markers have been used in developing a molecular identification key, as well as in molecular-typing for identification of the characterized cultivars (Zehdi et al., 2012; Racchi et al., 2014). These SSR markers, developed and characterized across various date palm accessions, can further be used for identifying candidate genes and understanding the genetic basis of traits of interest, which may further help in molecular breeding for the genetic improvement of the date palm tree.
Single nucleotide polymorphisms
As the third generation of molecular markers, single nucleotide polymorphisms (SNPs) are more stable, and have higher conformity of inheritance than other marker systems (Gupta et al., 2001). With the whole-genome sequencing of the date palm, the first attempt to identify SNPs was carried out by Al-Dous et al. (2011), where researchers called 1,748,109 SNPs in 381 Mb of sequence, yielding a heterozygosity rate of 0.46%, or 1 SNP/217 bp, but the distribution of the SNPs was skewed, with 49% of the SNPs within every 50 bp. Sabir J. S. M. et al. (2014) used the mitochondrial and plastid genome sequences of nine date palm varieties to examine SNPs, but found a low level of variation, suggesting the preferred use of nuclear SNPs for molecular characterization of date palm cultivars. A genotyping-by-sequencing (GBS) approach was used to identify 13,000–65000 SNPs comparing the genomes of 70 female cultivars from different date palm growing regions and four other Phoenix species (Mathew et al., 2015). Based on the whole-genome re-sequencing of 62 cultivars, a catalog of approximately 7 million SNPs in date palms was developed (Hazzouri et al., 2015). Recently, the GBS approach was followed by using re-sequenced data of 191 date palm cultivars to identify SNPs and assess the genetic diversity among the date palm trees grown in Qatar. This study revealed that these trees in Qatar are of eastern origin and their genetic diversity does not associate with different regions (Thareja et al., 2018). Faqir et al. (2019) sequenced maturase K (matK), ribulose biphosphate carboxylase larger subunit (rbcL), the ATP synthase subunit b (atpB) gene of the chloroplast genome, and 12 DNA fragments from the nuclear genome of seven cultivars. Based on the sequenced data, the researchers identified unique SNP signatures and developed an SNP-typing system for varietal identification of date palm cultivars from Pakistan.
The internal transcribed spacer (ITS) sequences of 15 Tunisian date palm accessions were compared to identify four haplotypes, and the haplotypic and nucleotide diversities were found to be low among the studied genotypes (Maina et al., 2019). Further phylogenetic analysis revealed that the Tunisian populations of date palm evolved under a neutral model, and a demographic equilibrium seems to be maintained within the studied genotypes.
Trait-specific markers in the date palm
Most of the molecular studies of the date palm have been carried out for genetic diversity and phylogenetic analysis, as well as for cultivar identification, with limited progress made in developing trait-specific molecular markers. Most efforts have been aimed at identifying markers associated with Bayoud disease resistance, or for sex determination. Fusarium oxysporum f. sp. albedensis causes Bayoud disease, which is one of the most devastating of all diseases in date palm trees (Michielse and Rep, 2009; El Modafar, 2010).
Bendiab et al. (1992) carried out isozyme polymorphism analysis using esterase (EST), glutamate oxaloacetate transaminase (GOT), endopeptidase (ENP), and alcohol dehydrogenase (ADH) polymorphisms in different F1 populations derived from seven female cultivars crossed with two males (Table 3). They found out three loci viz., Got2, Est 1, and Enp that could be used for hybrid screening. Benslimane et al. (1994, 1996) isolated two mitochondrial-like plasmid DNA (S and R plasmids) sharing 99% sequence similarity, except for 109 bp of sequence that was present in only the S plasmid. The S plasmid was found in Bayoud-susceptible genotypes, whereas the R plasmid was found in Bayoud-resistant Moroccan genotypes. Later, employing a PCR-based approach on 36 date palm varieties, Quenzar et al. (2001) confirmed the study of Benslimane et al. (1994, 1996), and reported that the simultaneous presence of the R plasmid and absence of the S plasmid can be considered a reliable marker for Bayoud resistance (Table 3). Salem et al. (2007) used this plasmid-based analysis system to check the susceptibility of Mauritanian date palm cultivars to Bayoud disease. Furthermore, using progenies of two controlled crosses, the authors showed that Bayoud strictly follows maternal transmission as controlled by the mitochondrial genome. The R and S mitochondrial plasmids have been used for molecular characterization of date palm cultivars from Algeria (Guettouchi et al., 2017), Syria (Haider and Nabulsi, 2012), and Saudi Arabia (Saleh et al., 2015).
Brittle leaf disease, known as maladie des feuilles cassantes in French, was first observed in southern Tunisia (Djerbi, 1983). It later spread to reach epidemic levels by 1986. The exact causal pathogen is not yet determined; however, the symptom of the disease is associated with manganese deficiency and the presence of a small double-stranded chloroplast RNA (Triki et al., 2003; Namsi et al., 2006, 2007; Marqués et al., 2008). Namsi et al. (2006) used chloroplast RNA, and developed a digoxigenin (DIG)-labeled probe for early diagnosis of BLD, which consistently gave positive hybridization signals, irrespective of cultivars, the severity of symptoms, or the geographic location (Table 3).
The date palm is a dioecious plant, and the sex of the plants can be determined only at the time of flowering, which takes 5–7 years (Shaheen, 1990). If the sex of the plants could be determined at the early seedling stage, this could save resources and time, as farmers need many female plants and only a few superior male plants for pollination. Hence, maintaining a proper male:female ratio is of the utmost importance for better production in the field. Sex determination at the early seedling stage is thus one of the major requisites for establishing commercial date palm orchards. Therefore, the identification of markers linked to the sex of plants is of key importance for date palm cultivation. For the first time, Siljak-Yakovlev et al. (1996) developed a cytological method in which staining with chromomycin shows the presence of an extra heterochromatin region on both the arms of the male chromosome, which was considered sex determinant. Atia et al. (2017) describe cytological-based markers to distinguish date palm sex through localization of 45S and 5S rDNA markers on date palm chromosomes using the fluorescence in situ hybridization (FISH) technique.
A few biochemical markers, such as peroxidase and glutamate oxaloacetate, reportedly differentiate between male and female date palms, with a differential response of peroxidase and glutamate oxaloacetate activity observed in female plants versus male plants (Qacif et al., 2007; Bekheet et al., 2008). Over the past 2 decades, several attempts have been made to understand the genetic basis of sex determination in date palms using various types of DNA markers, such as RFLP, RAPD, ISSR, and SSRs. Using RAPD primers, several polymorphic markers have been identified with the potential to distinguish male from female plants among different cultivars (Ben et al., 2000; Soliman et al., 2003; Bekheet et al., 2008).
Younis et al. (2008) used a combination of RAPD and ISSR techniques to identify three fragments derived from RAPD markers specific to females (OPA10-490, OPA12-750, OPD10-800), and two for males in RAPD analysis (OPA12-370, OPD10-675), as well as five specific markers for males through ISSR analysis (HB10-1010, HB9-340, HB12-375, 814-590, 844A-920) (Table 3). However, in the past decade, attempts have been made to develop SCAR (sequence-characterized amplified region) markers for sex determination in date palms. The genomic DNA of 10 male genotypes of unknown origin and 10 female genotypes were pooled in equal quantities separately, and 100 RAPD primers and 104 ISSR primers were used to identify sex-specific markers. One of the RAPD primers, OPA-02, amplified an ≈1.0-kb fragment specifically in pooled as well as individual samples of male genotypes, and was later converted into a SCAR marker, which amplified a fragment of 406 bp in both female and male genotypes, and a unique fragment of 354 bp only in male genotypes (Dhawan et al., 2013). The developed SCAR marker was further validated in 25 female and 10 male date palms belonging to different varieties collected from different locations. Later, using an ISSR marker, Al-Ameri et al. (2016b) identified a 390-bp fragment from the amplicons of primer IS_A02, specifically in a female plant, and a 380-bp fragment from the amplicons of primer IS_A71, specifically in male plants only. These fragments were sequenced further to develop sequence-specific markers. Al-Ameri et al. (2016a) developed a SCAR marker of size 253 bp, specific to male trees based on cDNA fingerprinting of start codon targeted (SCoT) marker, and validated it independently on male and female trees. Al-Qurainy et al. (2018) developed a SCAR marker linked to sex-specific regions in the genome of the date palm using RAPD marker OPC-06, which was producing a band of 186 bp in male plants only. Recently, a gene, SRY1, involved in initiating sex determination, was identified on the Y chromosome of the date palm, and was tested with 100% efficiency for identifying male plants at the seedling stage (Mohei et al., 2019). Apart from RAPD and ISSR markers, a few microsatellite markers (e.g., mPdIRDP80, mPdIRDP50, mPdIRDP52, mpdCIR48, and DP-168) possessing the capacity for sex differentiation in the date palm have also been identified (Elmeer and Mattat, 2012; Cherif et al., 2013; Maryam Jaskani et al., 2016). Al-Dous et al. (2011) identified a region harboring 1,605 SNPs linked to sex through de novo genome sequencing, and proposed that the date palm follows an XY system of gender inheritance (Table 3). A 6-Mb region has been further mapped onto the distal end of chromosome 12, which has been found to be associated with sex determination (Hazzouri et al., 2019). Recently, Torres et al. (2021) identified 16-bp male-specific sequences in the date palm Y chromosome.
Date palms are facing a severe threat around the globe from red palm weevil (Rhynchophorus ferrugineus Olivier). So far, no molecular marker has been reported that deciphers resistance to this dreaded date palm pest. Using a historic long-term ongoing field trial with 18 date palm varieties, researchers at the International Center for Biosaline Agriculture have identified the pattern of preference/sensitivity and non-preference/tolerance (anti-xenosis behavior) for red palm weevil of specific date palm varieties. They are further trying to understand if there could be a robust molecular/genetic basis of RPW resistance in the date palm, and further to identify the molecular markers linked to this RPW resistance. The developed markers will not only help in selecting resistant genotypes, but will also help in developing genotypes with RPW resistance through accelerated molecular breeding.
Date palm genomics
Genomics deals with the sequencing and analysis of the structure of the genome of an organism, predicting the genes, and their locations and functions in the genome. Initially, the date palm genome was considered to be relatively smaller than 250 Mb, with 41% of the region consisting of genes, and the remaining genome considered a non-coding region (Barakat et al., 1999). However, it was later found that the size of the date palm genome ranged from 550 to 650 Mbp (Malek, 2010). Initially, a random genomic library of Tunisian date palm varieties was constructed from total cellular DNA, and amplified using RAPD markers. The library consisted of inserts from 200 to 1,600 bp and was supposed to have potential application for generating probes for molecular characterization of date palm varieties through southern hybridization. Al-Faifi et al. (2017) generated 6,943 high-quality ESTs from a normalized cDNA library of the date palm cultivar, Sukkari. The generated ESTs were assembled into 6,362 unigenes and were further functionally annotated. The first genetic map of the date palm cv. Khalas was developed by Mathew et al. (2014), using ∼4,000 SNPs spanning a total of 1,293 cM. Furthermore, the analysis suggested that the telomeric region on linkage group 12 may be the sex-determination region of the date palm. A total of 19% of the draft genome sequence scaffolds were placed onto the linkage groups, and the analysis results showed that approximately 1.9 cM represents 1 Mb on the map (Mathew et al., 2014). The chronological developments in genome sequencing of date palm is given in Figure 2.
Organellar genomes
With the advances in next-generation sequencing (NGS) technologies during the past decade, progress in the genomics of the date palm has been made at an unprecedented pace. The complete chloroplast genome of the date palm cultivar Khalas was sequenced using pyrosequencing and was found to be of 158,462 bp in size, consisting of 112 unique genes and 19 duplicated fragments in the inverted repeat (IR) regions, and arranged in a typical quadripartite structure (Yang et al., 2010). Furthermore, 78 SNPs located in genes with vital functions were identified with potential for detecting intra-varietal polymorphisms within a date palm population. Using a combination of Sanger-based and next-generation sequencing strategies, Khan et al. (2012) sequenced the complete date palm chloroplast genome from the Pakistani cultivar Aseel. The size of the genome was found to be 158,458 bp, consisting of a large single-copy (LSC) region of 86,195 bp, and a small single-copy (SSC) region of 17,711 bp, separated by an IR region of 27,276 bp. The chloroplast genome consisted of 138 genes, of which 89 were protein-coding, 39 were tRNA, and 8 were rRNA genes. Furthermore, a comparison of the Khalas and Aseel chloroplast genome led to the identification of SNPs and mono-nucleotide SSRs. Recently, Khan et al. (2018) sequenced the chloroplast genome of two economically important date palm cultivars, Khanezi and Naghal, using the Illumina HiSeq4000 sequencing platform. The chloroplast genome sizes of Naghal and Khanezi were 158,210 bp and 158,211 bp, respectively, consisting of 138 genes. The phylogenetic analysis based on the whole chloroplast genome and 68 shared genes of four cultivars (Khanezi, Naghal, Khalas, and Aseel) yielded identical phylogenetic trees, with Khanezi and Naghal forming single clades with cultivars Khalas and Aseel, respectively.
Fang et al. (2012) published the first mitochondrial genome of the date palm cv. Khalas. The genome assembly consisted of 715,001 bp encoding 38 proteins, 30 tRNAs, and 3 ribosomal RNAs. The protein-coding sequence consists of only 6.5% (46,770 bp) of the mitochondrial genome, whereas the rest of the genome sequence (93.5%) was found to comprise chloroplast-derived (10.3%) and non-coding sequences. Recently, the mitochondrial genome of P. dactylifera var. Khanezi, consisting of 715,120 bp, was published (Asaf et al., 2018). The mitochondrial genome consisted of 67 genes encoding 24 transfer RNAs, 3 ribosomal RNAs, and 40 protein-coding genes. Apart from these two mitochondrial genomes, another unpublished assembly is available in GenBank from an unknown cultivar (MG257490.1), consisting of 585,493 bp (Figure 2).
Nuclear reference genomes
Whole-genome sequencing is fundamental for understanding the molecular basis of complex traits for crop improvement. With the rapid progress in NGS technology and the simultaneous availability of bioinformatics tools, the past decade has seen unprecedented developments in date palm genomics, leading to the development of two draft genome sequences and genetic maps. The first attempt to develop the draft genome sequence of the date palm cv. Khalas was made by Al-Dous et al. (2011). The genome was sequenced from tissue culture‒raised plants using the Illumina platform. Unfortunately, it covered only ∼60% of the genome and consisted of 380 Mb of sequence, spanning mainly gene-rich regions, including 25,059 gene models. However, this reference genome was found to be highly fragmented, with about 60,000 scaffolds showing a median length of ∼30 kb. These authors further identified a genomic region linked to the sex of the plant, and provided evidence that the date palm follows an XY system of gender inheritance. Subsequently, using pyrosequencing, Al-Mssallem et al. (2013) reported another genome assembly of higher quality from the same date palm cultivar Khalas. This genome assembly has a total length of 605.4 Mb, covering more than 90% of the genome and 96% of the genes. They further built a larger pool of gene models, consisting of 41,660 models with a total of 42,957 isoforms in 10,363 scaffolds. The sequenced genome analysis demonstrated genome-wide duplication after either ancient whole-genome duplications or massive segmental duplications. Genetic diversity analysis showed that the stress resistance and sugar metabolism-related genes are enriched in the chromosomal regions where the density of SNPs is relatively low. Scrutiny of the late embryogenesis abundant (LEA) gene family revealed that group 2 LEA genes are specifically abundant in date palms, with 62 group 2 LEA members showing generally ubiquitous expression, whereas LEA1, LEA3, LEA4, LEA5, LEA6, seed maturation protein, and dehydrin were found to be either seed or male flower associated. This date palm draft genome assembly has also been included in the reference sequence (RefSeq) collection in the National Center for Biotechnology Information (NCBI), and gene models have been included in UniProtKB and the Kyoto Encyclopedia of Genes and Genomes (KEGG) databases for further exploration. In 2019, Hazzouri et al. (2019) released a new date palm draft genome (“BC4 male”). This draft genome spanned 772 Mb and was assembled into 2,390 scaffolds (Figure 2).
Functional genomics of the date palm
The first attempt to gain insight into carbon partitioning, comparative transcriptome, and metabolome analysis in oil palm and date palm mesocarp led to the identification of several sugars and fatty acid metabolism genes/transporters involved in fatty acid and sugar accumulation in date and oil palm, respectively (Bourgis et al., 2011). Yin et al. (2012) carried out cDNA sequencing of the date palm fruits of Khalas at seven different developmental stages and identified 10 core cell division genes, 18 ripening-related genes, and 7 starch metabolic enzymes, which are involved in nutrition storage and sugar/starch metabolism. To generate and annotate the gene model of the date palm, Zhang et al. (2012) carried out in-depth transcriptomic sequencing from different tissues and at several developmental stages, and generated 30,854 annotated gene models from the cultivar Khalas. These were further assigned to Gene Ontology and KEGG pathways for future research aimed to unravel the genetic regulatory networks governing organ development and differentiation in the date palm (P. dactylifera). Whole-genome transcriptome analysis of eight tissues (root, seed, bud, fruit, green leaf, yellow leaf, female flower, and male flower), using the Roche/454 GS FLX platform, showed higher gene expression levels in developing tissues, such as male and female flower, root, and bud, than in the four other tissues, due to the need for more energy than in the relatively mature tissues (Fang et al., 2012). To identify the differentially expressed genes (DEGs) involved in fruit development and ripening, Al-Mssallem et al. (2013) carried out transcriptome analysis at seven distinct fruit developmental stages (0, 15, 30, 60, 90, 120, and 135 days post-pollination), and identified 4,134 DEGs whose expression varies significantly among the seven fruit developmental stages. The enrichment analysis of DEGs revealed that most of the molecular events involved in biological regulation, transcription, and regulation of RNA metabolic processes are down-regulated in the late stage of fruit development, whereas events involved in sugar accumulation, such as gluconeogenesis, cellular carbohydrate metabolism, and small molecule biosynthesis were up-regulated, resulting in unusually high sugar content in the dates. Hazzouri et al. (2019) carried out RNA-Seq analysis in date palm fruit at different developmental stages. The results indicated that the expression of alkaline/neutral invertase (A/N-INV1) was maximum at ≈105 days after pollination, whereas the expression of cell wall invertase (CWINV1 and CWINV3) genes peaked at 120 days after pollination, showing their positive role in sugar accumulation during fruit development. Recently, Naganeeswaran et al. (2020) performed transcriptome assembly from the embryogenic calli of the date palm cultivar Khalas, and reported 63,888 Gene Ontology (GO) terms and 122 small RNAs that were annotated from the assembly (Table 4).
Date palms generally grow under adverse climatic conditions and have therefore developed stress tolerance during their evolution. The date palm can survive under extreme drought, heat, and relatively high soil salinity (Yaish and Kumar, 2015), thereby providing a valuable genome source for mining abiotic stress tolerance genes. However, limited research work has been carried out to identify and exploit the abiotic stress-responsive genes from the date palm. To understand the molecular mechanisms underlying salinity tolerance in the date palm, Radwan et al. (2015) undertook salinity-responsive transcriptome analysis in young roots of the date palm cv. Deglet Beida, which led to the identification of 1939 differentially expressed genes involved in tolerance of salt stress. RNA-Seq analysis further revealed that salinity stress activates abscisic acid signaling pathways through SNF1-related protein kinase 2, and several key genes involved in sodium uptake and transport were found to be down-regulated, thereby slowing down uptake and transportation in plant tissues under stress conditions. Yaish et al. (2015) generated salinity-responsive small RNA libraries from leaves and roots of date palm seedlings. Deep sequencing using Illumina Hiseq2000 led to the identification of 153 homologs of conserved miRNAs, 89 miRNA variants, and 180 putative novel miRNAs from the date palm plant. Differential expression analysis revealed that 57 miRNAs in leaves and 27 miRNAs in roots were significantly regulated in response to salinity, whereas 12 miRNAs were commonly regulated in both leaves and roots. The targets of the identified miRNAs were the genes with known functions in plant salt tolerance, such as potassium channel AKT2-like proteins, vacuolar protein sorting-associated protein, calcium-dependent protein, and mitogen-activated proteins. Later, expression profiling in the leaves and roots of date palm seedlings revealed 194 differentially expressed transcripts in both leaf and root tissue in response to salinity stress (Yaish et al., 2017). Gene ontology analysis revealed that metabolic pathways, such as photosynthesis, sucrose and starch metabolism, and oxidative phosphorylation were enriched in leaves, whereas genes involved in membrane transport; phenylpropanoid biosynthesis; purine, thiamine, and tryptophan metabolism; and Casparian strip development, were enriched in roots in response to salinity stress. Salinity-responsive genes, such as putative potassium transporter 8, abscisic acid receptor PYR1 and 4, indole-3-acetic acid-amido synthetase GH3, along with a pyrophosphate-energized vacuolar membrane proton pump, were commonly induced in both roots and leaves. Using transcriptomic and metabolomic profiling, Safronov et al. (2017) studied the adaptation mechanism in the date palm toward mild heat, drought, and the combination of both. The results showed an increase in soluble carbohydrates, such as fructose and glucose derivatives, suggesting a switch to carbohydrate metabolism and cell wall biogenesis in response to these stresses. Increased transcriptional activation of genes involved in reactive oxygen species production occurred in response to all three treatments (drought, heat, and combined heat and drought). By contrast, under heat and combined heat and drought stress, genes enriched for circadian and diurnal rhythm motifs were differentially expressed, suggesting a stress avoidance mechanism in response to these stresses (Safronov et al., 2017). Another group of researchers employed salinity-responsive whole-genome bisulfite sequencing and mRNA sequencing in the roots of date palms (Al-Harrasi et al., 2018). The bisulfite sequencing revealed that the methylated regions increased in response to salinity, specifically at mCHG and mCHH sequences. However, when researchers correlated gene expression with DNA methylation, they observed that DNA methylation was not the primary agent that controls gene expression under salinity conditions (Al-Harrasi et al., 2018). Overexpressing the cDNA library of the date palm in Saccharomyces cerevisiae, and screening on a synthetic minimal medium containing 1.0 M of NaCl, resulted in the identification of genes such as aquaporins (PIP), serine/threonine protein kinases (STKs), ethylene-responsive transcription factor 1 (ERF1), and peroxidases (PRX) with potential salt-tolerance functions (Patankar et al., 2018). Rekik et al. (2019), through transcriptome analysis in leaves of Phoenix dactylifera cv. Deglet Nour, proposed a glutathione pathway involved in detoxifying cadmium under Cd stress conditions, and further identified genes encoding heavy metal transporters and chelators in response to heavy metal stress. Patankar et al. (2019b) isolated aquaporin genes (PdPIP1;2) and characterized their role in response to drought and salinity tolerance by overexpressing them in yeast and Arabidopsis. The overexpression of an aquaporin gene in yeast resulted in improved oxidative stress tolerance, whereas overexpression in Arabidopsis resulted in increased salinity and drought tolerance with increased biomass, chlorophyll content, and root length in transgenic plants (Patankar et al., 2019a). Further, Patankar et al. (2019a) isolated metallothionein 2A (PdMT2A) and characterized its role in abiotic stress tolerance in yeast and Arabidopsis. The transformed yeast cells have shown tolerance against drought, salinity, and oxidative stresses. The Arabidopsis plants overexpressing the metallothionein 2A (PdMT2A) gene have shown tolerance against salinity by maintaining a high K+/Na+ ratio, and against drought and oxidative stress (Patankar et al., 2019b). Al-Harrasi et al. (2020) isolated a salt-inducible vascular highway 1-interacting kinase (PdVIK) and characterized its role in response to various abiotic stresses through heterologous overexpression in yeast and Arabidopsis. Jana and Yaish (2020, 2021) isolated and characterized the glyoxalase-I gene (PdGLX1) and glyoxalase III genes (PdDJ-1) for their roles in mitigation of abiotic stress tolerance through overexpression in bacterial and yeast systems. This study further suggested that PdGLX1 and PdDJ-1 genes play an important role in methylglyoxal detoxification and in maintaining reactive oxygen species balance under stress conditions in date palms.
Apart from understanding the transcriptional response of abiotic stress tolerance and fruit development, a couple of studies have been carried out to identify the genes involved in biotic stress tolerance. To understand the molecular mechanisms involved in the BLD of the date palm, Saidi et al. (2010) constructed suppression-subtractive cDNA libraries from BLD-affected and non-affected trees and identified the genes that were up-regulated in response to BLD. The genes associated with stress response, metabolism, protein synthesis, and signal transduction were found to be specifically up-regulated in BLD-affected trees. Later, through RT-PCR analysis, Saidi et al. (2012) showed that the transcripts of MnSOD decreased in affected leaves and roots, unlike the transcripts of FeSOD and Cu/Zn-SOD, whose expression increased in these tissues, revealing that BLD decreases the expression of manganese-related genes in date palm trees. To understand the molecular basis of red palm weevil (Rhynchophorus ferrugineus Olivier) resistance in Phoenix canariensis, Giovino et al. (2015) carried out deep transcriptome analysis in leaves of healthy and infested trees at two stages (middle and late infestation) and identified 54 genes that were differentially regulated during the middle stage in response to RPW infestation (Table 3). Further enrichment analysis showed that phenylpropanoid-related pathways were induced during the middle infestation period.
Resequencing of the date palm
With the availability of genetic maps, organellar and nuclear reference genomes of the date palm, several research groups carried out whole-genome resequencing of date palms to identify QTLs and SNP markers as well as to study date palm diversity and phylogenetic history. Hazzouri et al. (2015) resequenced 61 female date palm accessions and 1 male (cv. Fard4), and detected 7,176,238 SNPs at a rate of ∼12 SNPs per kb. Genome-wide scans for selection suggested that there were ∼36 genomic regions in the genotypes of the Middle East, and 20 genomic regions in North African genotypes associated with positive selection which may underlie the geographic adaptation of these genotypes in these areas. They further characterized candidate mutations in the genes of the pathways associated with key agronomic traits, such as disease resistance, fruit ripening, fruit color, flowering time, and sugar metabolism. Hazzouri et al. (2015) further suggested that the R2R3 myb-like virescens (VIR) gene controls fruit color in the date palm. The varieties with red fruit color were found to have an intact VIR gene in the homozygous state, whereas the varieties with yellow fruit color had a copia-like retrotransposon insertion in the VIR gene in either the homozygous or heterozygous state. Using the GBS approach on 70 female cultivars from different date palm growing regions and four other Phoenix species, Mathew et al. (2015) showed that there are two centers of earliest cultivation and that the date palm is indigenous to North Africa. Whole-genome sequencing of several wild and cultivated date palms revealed a complex domestication history of date palm trees involving the contribution of a wild relative during the spread of cultivation from their original domestication center in the Arabian Gulf to North Africa (Gros-Balthazard et al., 2017). Sequence analysis of more than 200 mitochondrial and chloroplast genomes from a geographically diverse set of date palms showed that the most common cultivated date palms contain four haplotypes associated with the geographic region of cultivar origin (Mohamoud et al., 2019). Recently, Hazzouri et al. (2019) carried out genome-wide association studies of the sex-determining region, and of 21 fruit traits. GWAS analysis resulted in the identification of the R2R3-MYB transcription factor (VIR gene) associated with fruit color. The authors further identified an ≈1.1-Mb region consisting of invertase genes that were found to be associated with sugar composition in date palm fruit (Table 5).
Genomic databases for date palm
During the past decade, several attempts have been made to sequence and re-sequence the several date palm genotypes, leading to the accumulation of a huge amount of genomic data. Further, several SSR and SNP markers have been developed. However, this information is scattered across research publications. This necessitates the development of genomic databases for the date palm so that the developed genomic information can be used more efficiently. The first attempt at this was by Mokhtar et al. (2016), who established a Date Palm Molecular Markers Database (DPMMD) providing useful genomic information (http://dpmmd.easyomics.org/). This database contains information on more than 3,611,400 DNA markers involving SSRs and SNPs, genetic linkage maps, KEGG maps, DNA-barcode, as well as all previously published date palm articles in PubMed-indexed journals from 1976 to 2017. Apart from this, the DRDB (Date Palm Resequence Database) was developed by CAS Key Laboratory of Genome Sciences and Information and Joint Center of Excellence in Genomics, King Abdulaziz City for Science and Technology (He et al., 2017). This database consists of information about 6.3 million SNPs and 246,000 SSRs from 62 date palm cultivars. Apart from these two, there is no concise database for date palm genomics.
Summary and way forward
The date palm has immense regional relevance but requires global attention, as not many advanced research laboratories outside the Middle East and North Africa are giving due attention to date palm genomics. Although limited genomic studies of the date palm over the last decade have led to the identification of a couple of key genes associated with fruit color and sugar accumulation, this is still a long way from what is needed to unravel the hidden mysteries of this tree. Despite the huge existing diversity within the date palm genus, there is little understanding of the genetic factors underlying various biotic and abiotic stresses. The robustness and reliability of a marker are central to its usefulness in a genetic improvement program. Several breeder-friendly molecular markers, such as SSRs and SNPs, have been identified, but the extent to which these markers explain variation still needs to be validated on a large scale. Several abiotic stress responsive genes, and genes associated with fruit traits, have been identified. However, the identified genes/QTLs need to be introgressed in date palm improvement programs, either through breeding or genetic engineering. The use of genetic engineering tools for genome editing is the need of the moment, at least for game-changing traits such as the genetic mechanism of red palm weevil resistance, but this is still lagging because of limited concerted efforts with this crop. Further, studies on the role of small RNAs (siRNA and miRNA) are lacking. It is time to obtain feedback from stakeholders on desired traits in the different genetic backgrounds, and to generate foundational knowledge from diverse research disciplines, including genomics. An extensive germplasm exploration is required for the desired trait combinations ranging from plant architecture and stress tolerance to fruit yield and quality. A concerted effort is therefore needed, employing genomics, transcriptomics, proteomics and metabolomics for identification of candidate genes/genomic regions associated with complex agronomic traits, which can then be further introgressed in popular date palm cultivars/accessions, either through genetic engineering/editing or conventional breeding. An efficient ideotype breeding strategy for the desired date palm variety will be helpful for its improvement (Figure 3). In sum, there should be consortium- or mission-mode-based collaborative efforts to generate and use genomic information in breeding, genetic engineering, or genome editing research for developing new farmer-friendly date palm varieties.
Author contributions
RS conceived the idea for the manuscript. HR drafted the manuscript, and PV, ZH, and RS revised it. All authors agreed to the final submitted version of the manuscript.
Funding
This work enjoyed funding support from the International Center for Biosaline Agriculture, Dubai.
Conflict of interest
The authors declare that the research was conducted in the absence of any commercial or financial relationships that could be construed as a potential conflict of interest.
Publisher’s note
All claims expressed in this article are solely those of the authors and do not necessarily represent those of their affiliated organizations, or those of the publisher, the editors, and the reviewers. Any product that may be evaluated in this article, or claim that may be made by its manufacturer, is not guaranteed or endorsed by the publisher.
References
Abdulla, M., and Gamal, O. (2010). Investigation on molecular phylogeny of some date palm (Phoenix dactylifra L.) cultivars by protein, RAPD and ISSR markers in Saudi Arabia. Aust. J. Crop Sci. 4, 23.
Abul-Soad, A. A., Mahdi, S. M., and Markhand, G. S. (2015). “Date palm status and perspective in Pakistan,” in Date palm genetic resources and utilization (Springer), 153–205.
Ahmed, T. A., and Al-Qaradawi, A. Y. (2010). Genetic diversity of date palm genotypes in Qatar as determined by SSR and ISSR markers. Acta Hortic. 882, 279–286. doi:10.17660/actahortic.2010.882.30
Akkak, A., Scariot, V., Marinoni, D. T., Boccacci, P., Beltramo, C., and Botta, R. (2009). Development and evaluation of microsatellite markers in Phoenix dactylifera L. and their transferability to other Phoenix species. Biol. plant. 53, 164–166. doi:10.1007/s10535-009-0026-y
Al Kaabi, H. H., Zaid, A., Shephard, H., and Ainsworth, C. (2007). AFLP variation in tissue culture-derived date palm (Phoenix dactylifera L.) plants. Acta Hortic. 736, 135–160. doi:10.17660/actahortic.2007.736.12
Al Salih, A. A., Hussain, N., and Al Jarrah, A. (1987). Chromosomes number of a date palm male: Cultivar ghannami akhdar. Date Palm J.
Al-Ameri, A. A., Al-Qurainy, F., Gaafar, A.-R. Z., Khan, S., and Nadeem, M. (2016b). Molecular identification of sex in Phoenix dactylifera using inter simple sequence repeat markers. Biomed. Res. Int. 2016, 4530846. doi:10.1155/2016/4530846
Al-Ameri, A. A., Al-Qurainy, F., Gaafar, A. Z., Khan, S., and Nadeem, M. (2016a). Male specific gene expression in dioecious Phoenix dactylifera (Date palm) tree at flowering stage. Pak. J. Bot. 48, 131–135.
Al-Dous, E. K., George, B., Al-Mahmoud, M. E., Al-Jaber, M. Y., Wang, H., Salameh, Y. M., et al. (2011). De novo genome sequencing and comparative genomics of date palm (Phoenix dactylifera). Nat. Biotechnol. 29, 521–527. doi:10.1038/nbt.1860
Al-Faifi, S. A., Migdadi, H. M., Algamdi, S. S., Khan, M. A., Al-Obeed, R. S., Ammar, M. H., et al. (2017). “Analysis of expressed sequence tags (EST) in date palm,” in Date palm biotechnology protocols (Springer), 283–313.
Al-Faifi, S. A., Migdadi, H. M., Algamdi, S. S., Khan, M. A., Ammar, M. H., Al-Obeed, R. S., et al. (2016). Development, characterization and use of genomic SSR markers for assessment of genetic diversity in some Saudi date palm (Phoenix dactylifera L.) cultivars. Electron. J. Biotechnol. 21, 18–25. doi:10.1016/j.ejbt.2016.01.006
Al-Harrasi, I., Al-Yahyai, R., and Yaish, M. W. (2018). Differential DNA methylation and transcription profiles in date palm roots exposed to salinity. PloS one 13, e0191492. doi:10.1371/journal.pone.0191492
Al-Harrasi, I., Patankar, H. V., Al-Yahyai, R., Sunkar, R., Krishnamurthy, P., Kumar, P. P., et al. (2020). Molecular characterization of a date palm vascular highway 1-interacting kinase (PdVIK) under abiotic stresses. Genes 11, 568. doi:10.3390/genes11050568
Al-Khalifah, N. S., and Askari, E. (2003). Molecular phylogeny of date palm (Phoenix dactylifera L.) cultivars from Saudi Arabia by DNA fingerprinting. Theor. Appl. Genet. 107, 1266–1270. doi:10.1007/s00122-003-1369-y
Al-Moshileh, A. M., Motawei, M. I., Ai-Wasel, A., and Abdel-Latif, T. (2004). Identification of some date palm (Phoenix dactylifera L.) cultivars in Saudi Arabia using RAPD fingerprints. Jour. Agri. Mar. Scie. 9, 1–3. doi:10.24200/jams.vol9iss1pp1-3
Al-Mssallem, I. S., Hu, S., Zhang, X., Lin, Q., Liu, W., Tan, J., et al. (2013). Genome sequence of the date palm Phoenix dactylifera L. Nat. Commun. 4, 2274. doi:10.1038/ncomms3274
Al-Najm, A., Luo, S., Ahmad, N. M., Pourkheirandish, M., and Trethowan, R. (2017). Molecular variability and population structure of a core collection of date palm (Phoenix dactylifera L.) cultivars from Australia and the Middle East. Aust. J. Crop Sci. 11, 1106–1115. doi:10.21475/ajcs.17.11.09.pne517
Al-Qurainy, F., Al-Ameri, A. A., Khan, S., Nadeem, M., Gaafar, A.-R. Z., and Tarroum, M. (2018). SCAR marker for gender identification in date palm (Phoenix dactylifera L.) at the seedling stage. Int. J. Genomics 2018, 3035406. doi:10.1155/2018/3035406
Al-Ruqaishi, I. A., Davey, M., Alderson, P., and Mayes, S. (2008). Genetic relationships and genotype tracing in date palms (Phoenix dactylifera L.) in Oman, based on microsatellite markers. Plant Genet. Resour. 6, 70–72. doi:10.1017/s1479262108923820
Al-Shahib, W., and Marshall, R. J. (2003). The fruit of the date palm: Its possible use as the best food for the future? Int. J. Food Sci. Nutr. 54, 247–259. doi:10.1080/09637480120091982
Al-Yahyai, R., and Al-Khanjari, S. (2008). Biodiversity of date palm in the Sultanate of Oman. Afr. J. Agric. Res. 3, 389–395.
Al-Yahyai, R., and Khan, M. M. (2015). “Date palm status and perspective in Oman,” in Date palm genetic resources and utilization (Springer), 207–240.
Arabnezhad, H., Bahar, M., Mohammadi, H. R., and Latifian, M. (2012). Development, characterization and use of microsatellite markers for germplasm analysis in date palm (Phoenix dactylifera L.). Sci. Hortic. 134, 150–156. doi:10.1016/j.scienta.2011.11.032
Asaf, S., Khan, A. L., Al-Harrasi, A., and Al-Rawahi, A. (2018). First complete mitochondrial genome of Phoenix dactylifera var. Khanezi. Mitochondrial DNA. B Resour. 3, 778–779. doi:10.1080/23802359.2018.1491339
Atia, M. A., Adawy, S. S., and El-Itriby, H. A. (2017). “Date palm sex differentiation based on fluorescence in situ hybridization (FISH),” in Date palm biotechnology protocols (Springer), 245–256.
Awad, M. A., Al-Qurashi, A. D., and Mohamed, S. A. (2011). Antioxidant capacity, antioxidant compounds and antioxidant enzyme activities in five date cultivars during development and ripening. Sci. Hortic. 129, 688–693. doi:10.1016/j.scienta.2011.05.019
Ba-Angood, S. (2015). “Date palm status and perspective in Yemen,” in Date palm genetic resources and utilization (Springer), 241–263.
Barakat, A., Han, D. T., Benslimane, A.-A., Rode, A., and Bernardi, G. (1999). The gene distribution in the genomes of pea, tomato and date palm. FEBS Lett. 463, 139–142. doi:10.1016/s0014-5793(99)01587-2
Bashah, M. A. (1996). Date variety in the Kingdom of Saudi Arabia. Guidance booklet, palms and dates. Riyadh: King Abdulaziz University Press.
Battaglia, M., Ghsera, B., Mancini, M., Bergesio, C., Camussi, A., and Racchi, M. L. (2015). “Date palm status and perspective in Libya,” in Date palm genetic resources and utilization (Springer), 223–255.
Bekheet, S. A., Taha, H. S., Hanafy, M. S., and Solliman, M. E. (2008). Morphogenesis of sexual embryos of date palm cultured in vitro and early identification of sex type. J. Appl. Sci. Res. 4, 345–352.
Ben, A., Stiti, K., Lepoivre, P., and Jardin, P. D. (2000). Date palm (Phoenix dactylifera L.) cultivar identification using random amplified polymorphic DNA (RAPD). Cah. Agric. 9, 103–107.
Bendiab, K., Baaziz, M., Brakez, Z., and Sedra, My. H. (1992). Correlation of isoenzyme polymorphism and Bayoud-disease resistance in date palm cultivars and progeny. Euphytica 65, 23–32. doi:10.1007/BF00022196
Bendiab, K., Baaziz, M., and Majourhat, K. (1998). Preliminary date palm cultivar composition of Moroccan palm groves as revealed by leaf isoenzyme phenotypes. Biochem. Syst. Ecol. 26, 71–82. doi:10.1016/s0305-1978(97)00078-1
Bennaceur, M., Lanaud, C., Chevallier, M. H., and Bounaga, N. (1991). Genetic diversity of the date palm (Phoenix dactylifera L.) from Algeria revealed by enzyme markers. Plant Breed. 107, 56–69. doi:10.1111/j.1439-0523.1991.tb00528.x
Benslimane, A. A., Hartmann, C., Ouenzar, B., and Rode, A. (1996). Intramolecular recombination of a mitochondrial minicircular plasmid-like DNA of date-palm mediated by a set of short direct-repeat sequences. Curr. Genet. 29, 591–593. doi:10.1007/BF02426965
Benslimane, A. A., Rode, A., Quétier, F., and Hartmann, C. (1994). Characterization of two minicircular plasmid-like DNAs isolated from date-palm mitochondria. Curr. Genet. 26, 535–541. doi:10.1007/BF00309946
Billotte, N., Marseillac, N., Brottier, P., Noyer, J.-L., Jacquemoud‐Collet, J.-P., Moreau, C., et al. (2004). Nuclear microsatellite markers for the date palm (Phoenix dactylifera L.): Characterization and utility across the genus Phoenix and in other palm genera. Mol. Ecol. Notes 4, 256–258. doi:10.1111/j.1471-8286.2004.00634.x
Bodian, A., El Houmaizi, M. A., Ndoye Ndir, K., Hasnaoui, A., Nachtigall, M., and Wehling, P. (2012). Genetic diversity analysis of date palm (Phoenix dactylifera L.) cultivars from Figuig oasis (Morocco) using SSR markers. IJSAT 2, 96–104.
Bodian, A., Nachtigall, M., Frese, L., Aziz Elhoumaizi, M., and Hasnaoui, A. (2014). Genetic diversity analysis of date palm (Phoenix dactylifera L.) cultivars from Morocco using SSR markers. J. Biodivers. Biopros. Dev. 1, 126. doi:10.4172/2376-0214.1000126
Bouguedoura, N., Bennaceur, M., Babahani, S., and Benziouche, S. E. (2015). “Date palm status and perspective in Algeria,” in Date palm genetic resources and utilization (Springer), 125–168.
Bourgis, F., Kilaru, A., Cao, X., Ngando-Ebongue, G.-F., Drira, N., Ohlrogge, J. B., et al. (2011). Comparative transcriptome and metabolite analysis of oil palm and date palm mesocarp that differ dramatically in carbon partitioning. Proc. Natl. Acad. Sci. U. S. A. 108, 12527–12532. doi:10.1073/pnas.1106502108
Cao, B. R., and Chao, C.-C. T. (2002). Identification of date cultivars in California using AFLP markers. HortScience 37, 966–968. doi:10.21273/hortsci.37.6.966
Chaluvadi, S. R., Khanam, S., Aly, M. A., and Bennetzen, J. L. (2014). Genetic diversity and population structure of native and introduced date palm (Phoenix dactylifera) germplasm in the United Arab Emirates. Trop. Plant Biol. 7, 30–41. doi:10.1007/s12042-014-9135-7
Chao, C. T., and Krueger, R. R. (2007). The date palm (Phoenix dactylifera L.): Overview of biology, uses, and cultivation. HortScience 42, 1077–1082. doi:10.21273/hortsci.42.5.1077
Cherif, E., Zehdi, S., Castillo, K., Chabrillange, N., Abdoulkader, S., Pintaud, J.-C., et al. (2013). Male‐specific DNA markers provide genetic evidence of an XY chromosome system, a recombination arrest and allow the tracing of paternal lineages in date palm. New Phytol. 197, 409–415. doi:10.1111/nph.12069
Corniquel, B., and Mercier, L. (1994). Date palm (Phoenix dactylifera L.) cultivar identification by RFLP and RAPD. Plant Sci. 101, 163–172. doi:10.1016/0168-9452(94)90252-6
Darlington, C. D., and Wylie, A. P. (1956). Chromosome atlas of flowering plants. New York: The Macmillan Company, 519.
Devanand, P. S., and Chao, C. T. (2003a). Genetic variation within ‘Medjool’and ‘Deglet Noor’ date (Phoenix dactylifera L.) cultivars in California detected by fluorescent-AFLP markers. J. Hortic. Sci. Biotechnol. 78, 405–409. doi:10.1080/14620316.2003.11511639
Devanand, P. S., and Chao, C. T. (2003b). Identification of genetic strains of ‘Medjool’ and ‘Deglet Noor’ date palm cultivars in California using amplified fragment length polymorpohism (AFLP) markers. Acta Hortic.
Dhawan, C., Kharb, P., Sharma, R., Uppal, S., and Aggarwal, R. K. (2013). Development of male-specific SCAR marker in date palm (Phoenix dactylifera L.). Tree Genet. Genomes 9, 1143–1150. doi:10.1007/s11295-013-0617-9
Diaz, S., Pire, C., Ferrer, J., and Bonete, M. J. (2003). Identification of Phoenix dactylifera L. varieties based on amplified fragment length polymorphism (AFLP) markers. Cell. Mol. Biol. Lett. 8, 891–899.
Djerbi, M. (1983). Regional project for palm and dates research centre in near east and North Africa.Diseases of the date palm (Phoenix dactylifera)
El Hadrami, A., and Al-Khayri, J. M. (2012). Socioeconomic and traditional importance of date palm. Emir. J. Food Agric. 24, 371.
El Kadri, N., Mimoun, M. B., and Hormaza, J. I. (2019). Genetic diversity of Tunisian male date palm (Phoenix dactylifera L.) genotypes using morphological descriptors and molecular markers. Sci. Hortic. 253, 24–34. doi:10.1016/j.scienta.2019.04.026
El Modafar, C. (2010). Mechanisms of date palm resistance to Bayoud disease: Current state of knowledge and research prospects. Physiological Mol. Plant Pathology 74, 287–294. doi:10.1016/j.pmpp.2010.06.008
El-Assar, A. M., Krueger, R. R., Devanand, P. S., and Chao, C.-C. T. (2005). Genetic analysis of Egyptian date (Phoenix dactylifera L.) accessions using AFLP markers. Genet. Resour. Crop Evol. 52, 601–607. doi:10.1007/s10722-004-0583-z
El-Kharbotly, A., El-Mardi, O., Al-Saidi, N., and Al-Mahruki, Y. (1998). “Towards the construction of genetic map of date palms using AFLP technique,” in Proceedings of the first international conference on date palm (Al-Ain: United Arab Emirates University), 8–10.
El-Khishin, D. A., Adawy, S. S., Hussein, E. H., and El-Itriby, H. A. (2003). AFLP fingerprinting of some Egyptian date palm (Phoenix dactylifera L.) cultivars. Arab J. Biotechnol. 6, 223–234.
Elhoumaizi, M. A., Devanand, P. S., Fang, J., and Chao, C.-C. T. (2006). Confirmation of Medj∞l′dateasalandracevarietythrough≥≠ticanalysisofMedjool' accessions in Morocco. J. Am. Soc. Hortic. Sci. 131, 403–407. doi:10.21273/jashs.131.3.403
Elmeer, K., and Mattat, I. (2015). Genetic diversity of Qatari date palm using SSR markers. Genet. Mol. Res. 14, 1624–1635. doi:10.4238/2015.March.6.9
Elmeer, K., and Mattat, I. (2012). Marker-assisted sex differentiation in date palm using simple sequence repeats. 3 Biotech. 2, 241–247. doi:10.1007/s13205-012-0052-x
Elmeer, K., Sarwath, H., Malek, J., Baum, M., and Hamwieh, A. (2011). New microsatellite markers for assessment of genetic diversity in date palm (Phoenix dactylifera L.). 3 Biotech. 1, 91–97. doi:10.1007/s13205-011-0010-z
Elsafy, M., Zborowska, A., Bryngelsson, T., Fatih, M., Mujaju, C., and Garkava-Gustavsson, L. (2016). Elucidating the genetic diversity of farmer cultivars of female date palms (Phoenix dactylifera L.) from Sudan by microsatellite markers. Genet. Resour. Crop Evol. 63, 975–986. doi:10.1007/s10722-015-0294-7
Elshibli, S. (2009). Genetic diversity and adaptation of date palm (Phoenix dactylifera L.). Dissertation. Finland: University of Helsinki.
Elshibli, S., and Korpelainen, H. (2009). Excess heterozygosity and scarce genetic differentiation in the populations of Phoenix dactylifera L.: Human impact or ecological determinants. Plant Genet. Resour. 7, 95–104. doi:10.1017/s1479262108060905
Elshibli, S., and Korpelainen, H. (2008). Microsatellite markers reveal high genetic diversity in date palm (Phoenix dactylifera L.) germplasm from Sudan. Genetica 134, 251–260. doi:10.1007/s10709-007-9232-8
Emoghene, B. O., Asemota, O., Idu, M., and Eke, C. R. (2015). Molecular characterization of some date palms in Nigeria using RAPD markers. J. Appl. Biol. Biotechnol. 3, 026–030.
Fang, Y., Wu, H., Zhang, T., Yang, M., Yin, Y., Pan, L., et al. (2012). A complete sequence and transcriptomic analyses of date palm (Phoenix dactylifera L.) mitochondrial genome. PloS one 7, e37164. doi:10.1371/journal.pone.0037164
Faqir, N., Muhammad, A., Ali, G. M., Shehzad, A., Ali, K., Rahman, H. U., et al. (2019). Development of a single nucleotide polymorphism-based genetic identification key for date palm cultivars. Crop Sci. 59, 2509–2520.
Faqir, N., Muhammad, A., Shehzad, A., Hyder, M. Z., and Ali, G. M. (2016). Simple sequence repeat (SSR) markers show greater similarity among morphologically diverse date palm (Phoenix dactylifera L.) cultivars grown in Pakistan. Pure Appl. Biol. 5, 483. doi:10.19045/bspab.2016.50063
Fayadh, J. M., and Al-Showiman, S. S. (1990). Chemical composition of date palm (Phoenix dactylifera L.). J. Chem. Soc. Pak. 12, 84–103.
Giovino, A., Bertolini, E., Fileccia, V., Al Hassan, M., Labra, M., and Martinelli, F. (2015). Transcriptome analysis of Phoenix canariensis Chabaud in response to Rhynchophorus ferrugineus Olivier attacks. Front. Plant Sci. 6, 817. doi:10.3389/fpls.2015.00817
Gros-Balthazard, M., Galimberti, M., Kousathanas, A., Newton, C., Ivorra, S., Paradis, L., et al. (2017). The discovery of wild date palms in Oman reveals a complex domestication history involving centers in the Middle East and Africa. Curr. Biol. 27, 2211–2218. doi:10.1016/j.cub.2017.06.045
Guettouchi, A., Elshibli, S., Haider, N., Nabulsi, I., and Ykhlef, N. (2017). “Molecular diversity in date palm (Phoenix dactylifera L.) cultivars from Algeria indicated by RAPD and ISSR polymorphisms,” in Plant cell biotechnology and molecular biology, 76–89.
Gupta, P. K., Roy, J. K., and Prasad, M. (2001). Single nucleotide polymorphisms: A new paradigm for molecular marker technology and DNA polymorphism detection with emphasis on their use in plants. Curr. Sci., 524–535.
Haider, N., Nabulsi, I., and MirAli, N. (2012). Phylogenetic relationships among date palm (Phoenix dactylifera L.) cultivars in Syria using RAPD and ISSR markers. J. Plant Biol. Crop Res. 1, 12–24.
Haider, N., and Nabulsi, I. (2012). Molecular characterization of Syrian date palm cultivars using plasmid-like DNA markers. Russ. J. Genet. 48, 240–244. doi:10.1134/s1022795412010085
Hajian, S., and Hamidi-Esfahani, Z. (2015). “Date palm status and perspective in Iran,” in Date palm genetic resources and utilization (Springer), 19–47.
Hammadi, H., Vendramin, G. G., and Ali, A. F. (2011). Microsatellite diversity among Tunisian date palm (Phoenix dactylifera L.) subpopulations. Pak. J. Bot. 43, 1257–1264.
Hamwieh, A., Farah, J., Moussally, S, Khaled El-Shamaa, Almer, K., Khierallah, H., et al. (2010). Development of 1000 microsatellite markers across the date palm (Phoenix dactylifera L.) genome. Acta Hortic. 882, 269–277. doi:10.17660/actahortic.2010.882.29
Hazzouri, K. M., Flowers, J. M., Visser, H. J., Khierallah, H. S., Rosas, U., Pham, G. M., et al. (2015). Whole genome re-sequencing of date palms yields insights into diversification of a fruit tree crop. Nat. Commun. 6. doi:10.1038/ncomms9824
Hazzouri, K. M., Gros-Balthazard, M., Flowers, J. M., Copetti, D., Lemansour, A., Lebrun, M., et al. (2019). Genome-wide association mapping of date palm fruit traits. Nat. Commun. 10. doi:10.1038/s41467-019-12604-9
He, Z., Zhang, C., Liu, W., Lin, Q., Wei, T., Aljohi, H. A., et al. (2017). Drdb: An online date palm genomic resource database. Front. Plant Sci. 8, 1889. doi:10.3389/fpls.2017.01889
Huda, M. N., Hasan, M., Abdullah, H. M., and Sarker, U. (2019). Spatial distribution and genetic diversity of wild date palm (Phoenix sylvestris) growing in coastal Bangladesh. Tree Genet. Genomes 15, 3. doi:10.1007/s11295-018-1310-9
Jana, G. A., Krishnamurthy, P., Kumar, P. P., and Yaish, M. W. (2021). Functional characterization and expression profiling of glyoxalase III genes in date palm grown under abiotic stresses. Physiol. Plant. 172, 780–794. doi:10.1111/ppl.13239
Jana, G. A., and Yaish, M. W. (2020). Functional characterization of the Glyoxalase-I (PdGLX1) gene family in date palm under abiotic stresses. Plant Signal. Behav. 15, 1811527. doi:10.1080/15592324.2020.1811527
Johnson, C., Cullis, T. A., Cullis, M. A., and Cullis, C. A. (2009). DNA markers for variety identification in date palm (Phoenix dactylifera L.). J. Hortic. Sci. Biotechnol. 84, 591–594. doi:10.1080/14620316.2009.11512570
Johnson, D. V. (2011). “Introduction: Date palm biotechnology from theory to practice,” in Date palm biotechnology (Springer), 1–11.
Jubrael, J. M., Udupa, S. M., and Baum, M. (2005). Assessment of AFLP-based genetic relationships among date palm (Phoenix dactylifera L.) varieties of Iraq. J. Am. Soc. Hortic. Sci. 130, 442–447. doi:10.21273/jashs.130.3.442
Kassem, H. S., Alotaibi, B. A., Ahmed, A., and Aldosri, F. O. (2020). Sustainable management of the red palm weevil: The nexus between farmers’ adoption of integrated pest management and their knowledge of symptoms. Sustainability 12, 9647. doi:10.3390/su12229647
Khan, A., Khan, I. A., Heinze, B., and Azim, M. K. (2012). The chloroplast genome sequence of date palm (Phoenix dactylifera L. cv. ‘Aseel’). Plant Mol. Biol. Rep. 30, 666–678. doi:10.1007/s11105-011-0373-7
Khan, A. L., Asaf, S., Lee, I.-J., Al-Harrasi, A., and Al-Rawahi, A. (2018). First chloroplast genomics study of Phoenix dactylifera (var. Naghal and Khanezi): A comparative analysis. PloS one 13, e0200104. doi:10.1371/journal.pone.0200104
Khierallah, H., Bader, S., Baum, M., and Hamwieh, A. (2011a). Assessment of genetic diversity for some Iraqi date palms (Phoenix dactylifera L.) using amplified fragment length polymorphism (AFLP) markers. Afr. J. Biotechnol. 10, 9570–9576. doi:10.5897/ajb11.055
Khierallah, H. S., Al-Sammarraie, S. K., and Mohammed, H. I. (2014). Molecular characterization of some Iraqi date palm cultivars using RAPD and ISSR markers. J. Asian Sci. Res. 4, 490.
Khierallah, H. S., Bader, S. M., Baum, M., and Hamwieh, A. (2011b). Genetic diversity of Iraqi date palms revealed by microsatellite polymorphism. J. Am. Soc. Hortic. Sci. 136, 282–287. doi:10.21273/jashs.136.4.282
Mahar, A. Q. (2007). Post-harvest studies of different cultivars of date palm (Phoenix dactylifera L.) fruits, their protection, identification, processing and preservation at district Khairpur, Sindh, Pakistan. Khairpur, Sindh, Pakistan: PhD thesis, Date Palm Research Institute, Shah Abdul Latif University.
Maina, N., Baraket, G., Salhi-Hannachi, A., and Sakka, H. (2019). Sequence analysis and molecular evolution of Tunisian date palm cultivars (Phoenix dactylifera L.) based on the internal transcribed spacers (ITSs) region of the nuclear ribosomal DNA. Sci. Hortic. 247, 373–379. doi:10.1016/j.scienta.2018.12.045
Malek, J. A. (2010). Next generation DNA sequencing applied to the Date palm tree (Phoenix dactylifera). Acta Hortic. 882, 249–252. doi:10.17660/actahortic.2010.882.27
Marqués, J., Fadda, Z. G. N., Duran-Vila, N., Flores, R., Bové, J. M., and Daròs, J. A. (2008). A set of novel RNAs transcribed from the chloroplast genome accumulates in date palm leaflets affected by brittle leaf disease. Phytopathology 98, 337–344. doi:10.1094/PHYTO-98-3-0337
Maryam Jaskani, M. J., Awan, F. S., Ahmad, S., and Khan, I. A. (2016). Development of molecular method for sex identification in date palm (Phoenix dactylifera L.) plantlets using novel sex-linked microsatellite markers. 3 Biotech. 6, 22. doi:10.1007/s13205-015-0321-6
Mason, S. C. (1915). Botanical characters of the leaves of the date palm used in distinguishing cultivated varieties. Washington, D.C: US Department of Agriculture.
Mathew, L. S., Seidel, M. A., George, B., Mathew, S., Spannagl, M., Haberer, G., et al. (2015). A genome-wide survey of date palm cultivars supports two major subpopulations in Phoenix dactylifera. G3 5, 1429–1438. doi:10.1534/g3.115.018341
Mathew, L. S., Spannagl, M., Al-Malki, A., George, B., Torres, M. F., Al-Dous, E. K., et al. (2014). A first genetic map of date palm (Phoenix dactylifera) reveals long-range genome structure conservation in the palms. BMC Genomics 15, 285. doi:10.1186/1471-2164-15-285
Michielse, C. B., and Rep, M. (2009). Pathogen profile update: Fusarium oxysporum. Mol. Plant Pathol. 10, 311–324. doi:10.1111/j.1364-3703.2009.00538.x
Mohamed Lemine, F. M., Mohamed Ahmed, M. V. O., Ben Mohamed Maoulainine, L., Bouna, Z. el A. O., Samb, A., and Boukhary, O. (2014). Antioxidant activity of various Mauritanian date palm (Phoenix dactylifera) fruits at two edible ripening stages. Food Sci. Nutr. 2 (6), 700–705. doi:10.1002/fsn3.167
Mohamoud, Y. A., Mathew, L. S., Torres, M. F., Younuskunju, S., Krueger, R., Suhre, K., et al. (2019). Novel subpopulations in date palm (Phoenix dactylifera) identified by population-wide organellar genome sequencing. BMC Genomics 20, 498. doi:10.1186/s12864-019-5834-7
Mohei, E. L., Mohasseb, H. A. A., Al-Khateeb, A. A., Al-Khateeb, S. A., Chowdhury, K., El-Shemy, H. A., et al. (2019). Identification and sequencing of date-SRY gene: A novel tool for sex determination of date palm (Phoenix dactylifera L.). Saudi J. Biol. Sci. 26, 514–523. doi:10.1016/j.sjbs.2017.08.002
Mokhtar, M. M., Adawy, S. S., El-Assal, S. E.-D. S., and Hussein, E. H. (2016). Genic and intergenic SSR database generation, SNPs determination and pathway annotations in date palm (Phoenix dactylifera L.). PLOS one 11, e0159268. doi:10.1371/journal.pone.0159268
Moussouni, S., Pintaud, J.-C., Vigouroux, Y., and Bouguedoura, N. (2017). Diversity of Algerian oases date palm (Phoenix dactylifera L., Arecaceae): Heterozygote excess and cryptic structure suggest farmer management had a major impact on diversity. PloS one 12, e0175232. doi:10.1371/journal.pone.0175232
Munier, P. (1973). Le palmier dattier-techniques agricoles et productions tropicales. France: Maisonneuve et Larousse.
Naganeeswaran, S., Fayas, T. P., and Rajesh, M. K. (2020). Dataset of transcriptome assembly of date palm embryogenic calli and functional annotation. Data in Brief 20.105760. doi:10.1016/j.dib.2020.105760
Namsi, A., Marqués, J., Fadda, Z., Takrouni, M. L., Mahamoud, O. B., Zouba, A., et al. (2006). Diagnosis of “maladie des feuilles cassantes” or brittle leaf disease of date palms by detection of associated chloroplast encoded double stranded RNAs. Mol. Cell. Probes 20, 366–370. doi:10.1016/j.mcp.2006.05.001
Namsi, A., Montarone, M., Serra, P., Mahamoud, O. B., Takrouni, M. L., Zouba, A., et al. (2007). Manganese and brittle leaf disease of date palm trees. J. Plant Pathology, 125–136.
Osman, A. M. A. (1984). “The performance of date palms in the Sudan,” in Acta horticulturae (Leuven, Belgium): International Society for Horticultural Science ISHS), 231–238. doi:10.17660/ActaHortic.1984.143.25
Patankar, H. V., Al-Harrasi, I., Al Kharusi, L., Jana, G. A., Al-Yahyai, R., Sunkar, R., et al. (2019a). Overexpression of a metallothionein 2A gene from date palm confers abiotic stress tolerance to yeast and Arabidopsis thaliana. Int. J. Mol. Sci. 20, 2871. doi:10.3390/ijms20122871
Patankar, H. V., Al-Harrasi, I., Al-Yahyai, R., and Yaish, M. W. (2019b). Functional characterization of date palm aquaporin gene PdPIP1;2 confers drought and salinity tolerance to yeast and Arabidopsis. Genes 10, 390. doi:10.3390/genes10050390
Patankar, H. V., Al-Harrasi, I., Al-Yahyai, R., and Yaish, M. W. (2018). Identification of candidate genes involved in the salt tolerance of Date Palm (Phoenix dactylifera L.) based on a Yeast functional bioassay. DNA Cell Biol. 37, 524–534. doi:10.1089/dna.2018.4159
Pathak, M. R., and Hamzah, R. Y. (2008). RAPD analysis of date palm cultivars of Bahrain. Group 1, 00.
Purayil, F. T., Robert, G. A., Gothandam, K. M., Kurup, S. S., Subramaniam, S., and Cheruth, A. J. (2018). Genetic variability in selected date palm (Phoenix dactylifera L.) cultivars of United Arab Emirates using ISSR and DAMD markers. 3 Biotech. 8, 109. doi:10.1007/s13205-018-1108-3
Qacif, N., Baaziz, M., and Bendiab, K. (2007). Biochemical investigations on peroxidase contents of male and female inflorescences of date palm (Phoenix dactylifera L.). Sci. Hortic. 114, 298–301. doi:10.1016/j.scienta.2007.07.015
Quenzar, B., Trifi, M., Bouachrine, B., Hartmann, C., Marrakchi, M., Benslimane, A. A., et al. (2001). A mitochondrial molecular marker of resistance to Bayoud disease in date palm. Theor. Appl. Genet. 103, 366–370. doi:10.1007/s001220100572
Rabei, S., Said, W. M., Rizk, R. M., and El, S. F. (2012). Morphometric taxonomy of date palm diversity growing in Egypt. J. Bot. 189, 175–189.
Racchi, M. L., Bove, A., Turchi, A., Bashir, G., Battaglia, M., and Camussi, A. (2014). Genetic characterization of Libyan date palm resources by microsatellite markers. 3 Biotech. 4, 21–32. doi:10.1007/s13205-013-0116-6
Racchi, M. L., and Camussi, A. (2018). The date palms of Al jufrah-Libya: A survey on genetic diversity of local varieties. J. Agric. Environ. Int. Dev. (JAEID) 112, 161–184.
Radwan, O., Arro, J., Keller, C., and Korban, S. S. (2015). RNA-Seq transcriptome analysis in date palm suggests multi-dimensional responses to salinity stress. Trop. Plant Biol. 8, 74–86. doi:10.1007/s12042-015-9155-y
Rekik, I., Chaâbene, Z., Kriaa, W., Rorat, A., Franck, V., Hafedh, M., et al. (2019). Transcriptome assembly and abiotic related gene expression analysis of date palm reveal candidate genes involved in response to cadmium stress. Comp. Biochem. Physiol. C. Toxicol. Pharmacol. 225, 108569. doi:10.1016/j.cbpc.2019.108569
Rhouma, S., Dakhlaoui-Dkhil, S., Salem, A. O. M., Zehdi-Azouzi, S., Rhouma, A., Marrakchi, M., et al. (2008). Genetic diversity and phylogenic relationships in date-palms (Phoenix dactylifera L.) as assessed by random amplified microsatellite polymorphism markers (RAMPOs). Sci. Hortic. 117, 53–57. doi:10.1016/j.scienta.2008.03.013
Rhouma, S., Zehdi-Azouzi, S., Dakhlaoui-Dkhil, S., Salem, A. O. M., Othmani, A., Cherif, E., et al. (2010). Genetic variation in the Tunisian date palm (Phoenix dactylifera L.). Desert Plants, 355–370. doi:10.1007/978-3-642-02550-1_17
Rhouma, S., Zehdi-Azouzi, S., Ould Mohamed Salem, A., Rhouma, A., Marrakchi, M., and Trifi, M. (2007). Genetic diversity in ecotypes of Tunisian date-palm (Phoenix dactylifera L.) assessed by AFLP markers. J. Hortic. Sci. Biotechnol. 82, 929–933. doi:10.1080/14620316.2007.11512328
Rivera, D., Johnson, D., Delgadillo, J., Carrillo, M. H., Obón, C., Krueger, R., et al. (2013). Historical evidence of the Spanish introduction of date palm (Phoenix dactylifera L., Arecaceae) into the Americas. Genet. Resour. Crop Evol. 60, 1433–1452. doi:10.1007/s10722-012-9932-5
Sabir, J. S., Abo-Aba, S., Bafeel, S., Zari, T. A., Edris, S., Shokry, A. M., et al. (2014a). Characterization of ten date palm (Phoenix dactylifera L.) cultivars from Saudi Arabia using AFLP and ISSR markers. C. R. Biol. 337, 6–18. doi:10.1016/j.crvi.2013.11.003
Sabir, J. S. M., Arasappan, D., Bahieldin, A., Abo-Aba, S., Bafeel, S., Zari, T. A., et al. (2014b). Whole mitochondrial and plastid genome SNP analysis of nine date palm cultivars reveals plastid heteroplasmy and close phylogenetic relationships among cultivars. PloS one 9, e94158. doi:10.1371/journal.pone.0094158
Saboori, S., Noormohammadi, Z., Sheidai, M., and Marashi, S. (2020). SCoT molecular markers and genetic fingerprinting of date palm (Phoenix dactylifera L.) cultivars. Genet. Resour. Crop Evol. 67, 73–82. doi:10.1007/s10722-019-00854-x
Safronov, O., Kreuzwieser, J., Haberer, G., Alyousif, M. S., Schulze, W., Al-Harbi, N., et al. (2017). Detecting early signs of heat and drought stress in Phoenix dactylifera (date palm). PloS one 12, e0177883. doi:10.1371/journal.pone.0177883
Saidi, M. N., Jbir, R., Ghorbel, I., Namsi, A., Drira, N., and Gargouri-Bouzid, R. (2012). Brittle leaf disease induces an oxidative stress and decreases the expression of manganese-related genes in date palm (Phoenix dactylifera L.). Plant Physiol. biochem. 50, 1–7. doi:10.1016/j.plaphy.2011.09.016
Saidi, M. N., Ladouce, N., Hadhri, R., Grima-Pettenati, J., Drira, N., and Gargouri-Bouzid, R. (2010). Identification and characterization of differentially expressed ESTs in date palm leaves affected by brittle leaf disease. Plant Sci. 179, 325–332. doi:10.1016/j.plantsci.2010.06.002
Saleh, A. A., El_Komy, M. H., Eranthodi, A., Hamoud, A. S., and Molan, Y. Y. (2015). Variation in a molecular marker for resistance of Saudi date palm germplasm to Fusarium oxysporum f. sp. albedinis, the causal agent of Bayoud disease. Eur. J. Plant Pathol. 143, 507–514. doi:10.1007/s10658-015-0703-y
Salem, A. O. M., Rhouma, S., Zehdi, S., Marrakchi, M., and Trifi, M. (2007). Molecular characterization of Mauritanian date palm cultivars using plasmid-like DNA markers. Biol. Plant. 51, 169–172.
Salomon-Torres, R., Ortiz-Uribe, N., Villa-Angulo, C., Villa-Angulo, R., and Yaurima-Basaldúa, V. H. (2017). Assessment of SSR markers used in analysis of genetic diversity of date palm (Phoenix dactylifera L.). Plant Cell Biotechnol. Mol. Biol. 18, 269–280.
Sedra, M. H. (2015). “Date palm status and perspective in Morocco,” in Date palm genetic resources and utilization (Springer), 257–323.
Sedra, M. H., Lashermes, P., Trouslot, P., Combes, M.-C., and Hamon, S. (1998). Identification and genetic diversity analysis of date palm (Phoenix dactylifera L.) varieties from Morocco using RAPD markers. Euphytica 103, 75–82. doi:10.1023/a:1018377827903
Shaheen, M. A. (1990). Propagation of date palm through tissue culture: A review and an interpretation. Ann. Agric. Sci. 35, 895–909.
Sharifi, M., Sheidai, M., and Koohdar, F. (2018). Genetic fingerprinting of date palm (Phoenix dactylifera L.) by using ISSR and cpDNA sequences. Indian J. Genet. 78, 507–514.
Siljak-Yakovlev, S., Cerbah, M., Sarr, A., Benmalek, S., Bounaga, N., de la Pena, T. C., et al. (1996). Chromosomal sex determination and heterochromatin structure in date palm. Sex. Plant Reprod. 9, 127–132. doi:10.1007/bf02221391
Soliman, S. S., Ali, B. A., and Ahmed, M. M. M. (2003). Genetic comparisons of Egyptian date palm cultivars (Phoenix dactylifera L.) by RAPD-PCR. Afr. J. Biotechnol. 2, 86–87. doi:10.5897/ajb2003.000-1017
Soumaya, R. C., Ghada, B., Sonia, D. D., Salwa, Z.-A., and Mokhtar, T. (2011). Molecular research on the genetic diversity of Tunisian date palm (Phoenix dactylifera L.) using the random amplified microsatellite polymorphism (RAMPO) and amplified fragment length polymorphism (AFLP) methods. Afr. J. Biotechnol. 10, 10352–10365. doi:10.5897/ajb10.2242
Takele, D., Tsegaw, M., and Indracanti, M. (2021). Genetic diversity assessment in some landraces and varieties of date palm (Phoenix dactylifera L.) from Afar Region, Ethiopia, using ISSR markers. Ecol. Genet. Genomics 19, 100085. doi:10.1016/j.egg.2021.100085
Tengberg, M. (2012). Beginnings and early history of date palm garden cultivation in the Middle East. J. Arid Environ. 86, 139–147. doi:10.1016/j.jaridenv.2011.11.022
Thareja, G., Mathew, S., Mathew, L. S., Mohamoud, Y. A., Suhre, K., and Malek, J. A. (2018). Genotyping-by-sequencing identifies date palm clone preference in agronomics of the State of Qatar. PLoS one 13, e0207299. doi:10.1371/journal.pone.0207299
Torres, A. M., and Tisserat, B. (1980). Leaf isozymes as genetic markers in date palms. Am. J. Bot. 67, 162–167. doi:10.1002/j.1537-2197.1980.tb07637.x
Torres, M. F., Mathew, L. S., Ahmed, I., Al-Azwani, I. K., Krueger, R., Rivera-Nuñez, D., et al. (2018). Genus-wide sequencing supports a two-locus model for sex-determination in Phoenix. Nat. Commun. 9, 3969–9. doi:10.1038/s41467-018-06375-y
Torres, M. F., Mohamoud, Y. A., Younuskunju, S., Suhre, K., and Malek, J. A. (2021). Evidence of recombination suppression blocks on the Y chromosome of date palm (Phoenix dactylifera). Front. Plant Sci. 12, 697. doi:10.3389/fpls.2021.634901
Triki, M. A., Zouba, A., Khoualdia, O., Mahamoud, O. B., Takrouni, M. L., Garnier, M., et al. (2003). Maladie des feuilles cassantes” or brittle leaf disease of date palms in Tunisia: Biotic or abiotic disease? J. Plant Pathology, 71–79.
Yaish, M. W., and Kumar, P. P. (2015). Salt tolerance research in date palm tree (Phoenix dactylifera L.), past, present, and future perspectives. Front. Plant Sci. 6, 348. doi:10.3389/fpls.2015.00348
Yaish, M. W., Patankar, H. V., Assaha, D. V., Zheng, Y., Al-Yahyai, R., and Sunkar, R. (2017). Genome-wide expression profiling in leaves and roots of date palm (Phoenix dactylifera L.) exposed to salinity. BMC Genomics 18, 246. doi:10.1186/s12864-017-3633-6
Yaish, M. W., Sunkar, R., Zheng, Y., Ji, B., Al-Yahyai, R., and Farooq, S. A. (2015). A genome-wide identification of the miRNAome in response to salinity stress in date palm (Phoenix dactylifera L.). Front. Plant Sci. 6, 946. doi:10.3389/fpls.2015.00946
Yang, M., Zhang, X., Liu, G., Yin, Y., Chen, K., Yun, Q., et al. (2010). The complete chloroplast genome sequence of date palm (Phoenix dactylifera L.). PloS one 5, e12762. doi:10.1371/journal.pone.0012762
Yin, Y., Zhang, X., Fang, Y., Pan, L., Sun, G., Xin, C., et al. (2012). High-throughput sequencing-based gene profiling on multi-staged fruit development of date palm (Phoenix dactylifera, L.). Plant Mol. Biol. 78, 617–626. doi:10.1007/s11103-012-9890-5
Younis, R. A., Ismail, O. M., and Soliman, S. S. (2008). Identification of sex-specific DNA markers for date palm (Phoenix dactylifera L.) using RAPD and ISSR techniques. Res. J. Agric. Biol. Sci. 4, 278–284.
Zabar, A. F., and Borowy, A. (2012). in Cultivation of date palm in Iraq, 22, 39–54.Annales Universitatis Mariae Curie-SkłodowskaSect. EEE, Hortic.
Zaid, A., and De Wet, P. F. (1999). “Chapter I botanical and systematic description of date palm,” in FAO plant production and protection papers, 1–28.
Zango, O., Cherif, E., Chabrillange, N., Zehdi-Azouzi, S., Gros-Balthazard, M., Naqvi, S. A., et al. (2017). Genetic diversity of Southeastern Nigerien date palms reveals a secondary structure within Western populations. Tree Genet. Genomes 13, 75. doi:10.1007/s11295-017-1150-z
Zehdi, S., Cherif, E., Rhouma, S., Santoni, S., Hannachi, A. S., and Pintaud, J. C. (2012). Molecular polymorphism and genetic relationships in date palm (Phoenix dactylifera L.): The utility of nuclear microsatellite markers. Sci. Hortic. 148, 255–263. doi:10.1016/j.scienta.2012.10.011
Zehdi, S., Trifi, M., Billotte, N., Marrakchi, M., and Christophe Pintaud, J. (2004). Genetic diversity of Tunisian date palms (Phoenix dactylifera L.) revealed by nuclear microsatellite polymorphism. Hereditas 141, 278–287. doi:10.1111/j.1601-5223.2004.01855.x
Zehdi, S., Trifi, M., Ould Mohamed Salem, A., Marrakchi, M., and Rhouma, A. (2002). Survey of inter simple sequence repeat polymorphisms in Tunisian date palms (Phoenix dactylifera L.). J. Genet. Breed. (Italy).
Zehdi-Azouzi, S., Cherif, E., Moussouni, S., Gros-Balthazard, M., Abbas Naqvi, S., Ludena, B., et al. (2015). Genetic structure of the date palm (Phoenix dactylifera) in the Old World reveals a strong differentiation between eastern and western populations. Ann. Bot. 116, 847–112. doi:10.1093/aob/mcv132
Zhang, G., Pan, L., Yin, Y., Liu, W., Huang, D., Zhang, T., et al. (2012). Large-scale collection and annotation of gene models for date palm (Phoenix dactylifera, L.). Plant Mol. Biol. 79, 521–536. doi:10.1007/s11103-012-9924-z
Zhao, Y., Keremane, M., Prakash, C. S., and He, G. (2017). Characterization and amplification of gene-based simple sequence repeat (SSR) markers in date palm. Methods Mol. Biol. 1638, 259–271. doi:10.1007/978-1-4939-7159-6_21
Keywords: date palm, genomics, diversity, molecular markers, transcriptomics
Citation: Rahman H, Vikram P, Hammami Z and Singh RK (2022) Recent advances in date palm genomics: A comprehensive review. Front. Genet. 13:959266. doi: 10.3389/fgene.2022.959266
Received: 01 June 2022; Accepted: 11 August 2022;
Published: 13 September 2022.
Edited by:
Deepmala Sehgal, International Maize and Wheat Improvement Center, MexicoReviewed by:
Saurabh Badoni, International Rice Research Institute (IRRI), PhilippinesMahmoud Yaish, Sultan Qaboos University, Oman
Ulises Rosas, Institute of Biology, National Autonomous University of Mexico, Mexico
Copyright © 2022 Rahman, Vikram, Hammami and Singh. This is an open-access article distributed under the terms of the Creative Commons Attribution License (CC BY). The use, distribution or reproduction in other forums is permitted, provided the original author(s) and the copyright owner(s) are credited and that the original publication in this journal is cited, in accordance with accepted academic practice. No use, distribution or reproduction is permitted which does not comply with these terms.
*Correspondence: Hifzur Rahman, r.hifzurrahman@biosaline.org.ae, rahman.biotech@gmail.com