- School of Stomatology, Weifang Medical University, Weifang, China
Mesenchymal stem cells (MSCs) are characterized by self-renewal, rapid proliferation, multipotent differentiation, and low immunogenicity. In addition, the tropism of MSCs towards injured tissues and tumor lesions makes them attractive candidates as cell carriers for therapeutic agent delivery and genetic material transfer. The interaction between tumor cells and MSCs in the tumor microenvironment plays an important role in tumor progression. Oral cancer is one of the most common malignant diseases in the head and neck. Although considerable improvements in the treatment of oral cancer were achieved, more effective and safer novel agents and treatments are still needed, and deeper studies on the etiology, pathology, and treatment of the oral cancer are desirable. In the past decades, many studies have reported the beneficial effects of MSCs-based therapies in the treatment of various diseases, including oral cancers. Meanwhile, other studies demonstrated that MSCs may enhance the growth and metastasis of oral cancer. In this paper, we reviewed the research progress of the effects of MSCs on oral cancers, the underlying mechanisms, and their potential applications in the treatment of oral cancers.
1 Introduction
MSCs are a class of non-hematopoietic stem cells belonging to the mesoderm, with the characteristics of self-renewal, high proliferation, multi-directional differentiation potential. It has been demonstrated that one of the possible sources of MSCs is the blood vessel wall, which is a type of perivascular cells. This provides a great potential for its involvement in tissue regeneration (da Silva Meirelles et al., 2008). Many studies have been conducted to validate the use of MSCs in bone regeneration and nerve regeneration (Liau et al., 2020; Fu et al., 2021). MSCs exhibit chemotactic properties similar to immune cells in response to tissue injury and inflammation. MSCs can release various bioactive factors, such as immunosuppressive molecules, growth factors, chemokines, and complement components to regulate the inflammatory process and create a balanced inflammatory and regenerative microenvironment in damaged tissues, thereby treating various degenerative and inflammatory diseases (Shi et al., 2018). Because of their inherent ability to migrate and colonize into tumor tissues, MSCs were reported to closely interacted with tumor and tumor cells. It was shown that interleukin (IL)-12-expressing MSCs could inhibit the growth of tumor in a model of mouse renal cell carcinoma (Gao et al., 2010), and in a mouse melanoma brain metastasis model, intracarotid administration of oncolytic herpes simplex virus-armed MSCs significantly prolonged the survival of mice (Du et al., 2017). Thus, MSCs are attracting increasing interest in the field of oncology.
Oral cancer is one of the most common malignant tumors of the head and neck, often occurring in the middle-aged and elderly population and more than 90% of oral cancer are oral squamous cell carcinoma (OSCC) (Choi and Myers, 2008). Over the past decades, the incidence of oral cancer has been on a gradual increase. Smoking, excessive alcohol consumption as well as betel nut chewing, are the main causative factors of oral cancer. In addition, it cannot be ignored that the number of young patients suffering oral cancer also has been increasing. According to public reports, the number of new oral cancer cases and patients of new deaths reached 377,000 and 177,000, respectively, worldwide in 2020 (Chai et al., 2020; Grigolato et al., 2021; Sung et al., 2021).
A growing number of studies have demonstrated the beneficial effects of MSCs-based therapies in the treatment of various diseases, including oral cancers. However, some other studies showed that MSCs may enhance the growth and metastasis of oral cancer. The exact role of MSCs’ effects on tumor, i.e., whether MSCs could exert tumor-suppressive effects or, conversely, whether they favor tumor growth, has not been fully elucidated, and some concerns, including the mechanisms responsible for these phenomenons remain to be not yet clear. To better serve the discovery of potential strategies for the treatment of oral cancer, we reviewed the effects of MSCs on oral cancer, the underlying mechanisms, and MSCs’ potential applications in the treatment of oral cancer.
2 Discovery and Characterization of Mesenchymal Stem Cells
Originated from the mesoderm and firstly found in bone marrow, MSCs can be isolated from multifarious post-natal tissues, including adipose, umbilical cord, umbilical cord blood, amniotic fluid, and other tissues (Whiteside, 2018; Almeida-Porada et al., 2020; Tavakoli et al., 2020). Apart from these tissues, MSCs have also been isolated from dental tissues, including dental pulp, dental follicle, apical papillae, periodontal ligament, and gingiva (Shi and Gronthos, 2003; Pierdomenico et al., 2005; Janjanin et al., 2008; Lindroos et al., 2008; Sonoyama et al., 2008). MSCs are positive for CD105, CD90, CD73, CD146, CD29, STRO-1, but are negative for CD14, CD34, CD45 (Lei et al., 2014; Hung et al., 2015; Ji et al., 2016). Under suitable inductive medium, MSCs are capable of differentiating into osteoblasts, adipocyte, chondrocytes, and many other cells (Whiteside, 2018).
MSCs are localized throughout the adult body as a small population in the stroma of the tissue concerned, and the micro-environment protect their self-renewal potential and undifferentiated state (Urbanek et al., 2006; Wang et al., 2011). Upon tissue injury or inflammation insult, MSCs are activated and leave their ecological niche and migrate to the site of injury, inflammation and tumors, where they are able to secrete various cytokines, chemokines, and growth factors that closely interact with the inflammatory environment and the tumor environment (TME), respectively (Sun et al., 2014).
Compared to embryonic stem cells, MSCs are related with fewer ethical issues, and have emerged as one of the most promising cell therapy tools due to their excellent biological properties, such as relatively simple cell isolation procedure, high potential for expansion, low immunogenicity, pluripotency, and the ability to secrete mediators that support tissue transformation or replacement (McLeod and Baylis, 2006; Caulfield and Ogbogu, 2011; Widdows et al., 2014; Kariminekoo et al., 2016; Musiał-Wysocka et al., 2019). With the accumulation of data about the interaction between MSCs and tumor cells, MSCs have been demonstrated the natural anti-tumor functions, which is the basis for intensive research for new methods using MSCs as a tool to inhibit tumor growth and invasion, although the dualistic role of MSCs on tumors still exists.
3 Interaction Between Mesenchymal Stem Cells and Tumors
The interaction of MSCs and malignant tumors provides new clinical ideas for the treatment of malignant tumors. However, the promotive or inhibitory effects of MSCs on tumors are still inconclusive. It is shown that the normal tissue microenvironment, whose stability is usually maintained by MSCs, is subsequently changed to TME once a tumor develops and the in vivo homeostasis is disrupted, and TME also could be remodeled by MSCs of normal tissue origin (Whiteside, 2018). Interactions between MSCs and tumors in TME are described in Figure 1.
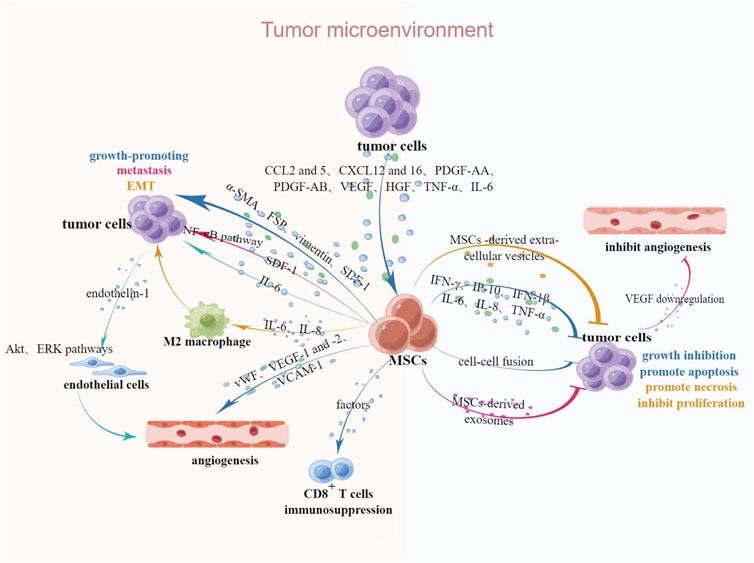
FIGURE 1. Interaction between MSCs and tumors in TME. Upon tumorigenesis, tumors secrete relevant chemokines, cytokines and growth factors to attract MSCs, after which MSCs undergo a series of phenotypic and functional changes and secrete relevant bioactive factors to act on neighboring cells, thus exerting tumor-promoting or suppressive effects. Abbreviations: CCL2 and 5, CC-chemokine ligands 2 and 5; CXCL12 and 16, chemokine ligands 12 and 16; HGF, hepatocyte growth factor; α-SMA, α-smooth muscle actin; FSP, fibroblast surface protein; SDF-1, stromal-derived factor 1; IP-10, IFN-γ-inducible protein 10. This figure was created by using Figdraw.
Some chemokines, cytokines and growth factors in TME have strong chemotactic effects on MSCs, such as CC-chemokine ligands 2 and 5, chemokine ligands 12 and 16, platelet-derived growth factor (PDGF)-AB, vascular endothelial growth factor (VEGF), hepatocyte growth factor and tumor necrosis factor (TNF)-α (Ponte et al., 2007; Shi et al., 2017). It is reported that after exposure to tumor cell-conditioned medium (CM), bone marrow derived MSCs (BMSCs) acquired a myofibroblast phenotype, which characterized by increased expression of α-smooth muscle actin, fibroblast surface protein, vimentin and stromal-derived factor 1, thereby promoting the growth of tumor cells (Mishra et al., 2008). After co-culture with VEGF, MSCs acquired a lymphatic phenotype and an endothelial cells phenotype characterized by the expression of von Willebrand factor, VEGF receptors 1 and 2, vascular endothelial-keratin, and vascular cell adhesion molecule-1, which promote vascular and lymphatic angiogenesis, ultimately lead to regional lymph node metastasis (Oswald et al., 2004; Conrad et al., 2009). These effects are also verified by the study that the BMSCs-mediated tumor promotion is associated with enhanced angiogenesis induced by the secretion of pro-angiogenic factors. In a study of colorectal cancer, Huang et al. (2013) found that MSCs-derived IL-6 could activate Akt and ERK pathways in endothelial cells through upregulation of endothelin-1 in cancer cells, which subsequently enhanced tropism and angiogenesis to tumors. Stromal-derived factor-1α secreted by BMSCs promotes the invasion of squamous cell carcinoma by activating nuclear factor-κB (Chung et al., 2006; Rehman and Wang, 2008; Xu et al., 2019). The epithelial-mesenchymal transformation (EMT) is a key factor in the migration and invasion of malignant tumors. The morphology and phenotype of cancer cells post-EMT are altered and acquire the ability to metastasize to distant sites. Tumor-derived MSCs secrete large amounts of IL-6 and IL-8 to stimulate M2 macrophage polarization, which enhances the EMT of gastric cancer, thus promoting migration and invasion of tumor (Dominici et al., 2006; Li et al., 2019b). MSCs have been reported to favor tumor growth due to immunosuppression, and Djouad et al. (2003) demonstrated that soluble factors produced by MSCs exert immunosuppressive effects by mediating the inhibition of lymphocyte proliferation by CD8+ regulatory cells, and further identified the tumor growth-promoting effect of MSCs in a study of B16 melanoma.
In contrast with the above results, a growing number of studies have identified a dual role of MSCs for tumor progression. Pakravan et al. (2017) demonstrated that exosomes derived from BMSCs were able to downregulate the expression of VEGF in breast cancer cells, thereby inhibiting vascular growth. It is reported that interferon (IFN)-γ, IFN-γ-inducible protein 10, IFN-1β, IL-6, IL-8, and TNF-α appear to play an important role in the inhibitory effect of MSCs on tumor cells (Tomchuck et al., 2008). MSCs may inhibit tumor progression by means of cell fusion. Wang et al. (2012b) successfully inhibited the progression of esophageal cancer by inhibiting tumor cells growth and increasing apoptosis by fusing human umbilical cord MSCs with esophageal cancer cells. Human BMSCs derived extracellular vesicles can promote apoptosis or necrosis and inhibit the proliferation of tumor cells in hepatocellular carcinoma, ovarian cancer and Kaposi’s sarcoma by activating cell cycle negative regulators (Bruno et al., 2013).
Given the complex interactions between MSCs, tumor cells, and TME, no simple factor by itself can determine the fate of tumor, and further studies are needed to better understand the special functions of MSCs in the TME before these cells become valuable tools in cancer therapy.
4 Effects of Mesenchymal Stem Cells on Oral Cancer
In recent years, there have been gradually increasing studies on the relationship between the MSCs and oral cancer, and in these studies, it was found that, on the one hand, MSCs have an inhibitory effect on oral cancer, while on the other hand, MSCs can promote the progression of oral cancer. In addition, the effects of MSCs derived from different sources on oral cancer were not consistent. The effects of different MSCs on oral cancer are shown in Table 1.
4.1 Dental Tissues Derived Mesenchymal Stem Cells (DMSCs)
Ji et al. (2016) showed that gingival tissue derived MSCs (GMSCs) inhibited the growth of tongue squamous cell carcinoma (TSCC) cell lines CAL27 and WSU-HN6 in vitro, and the soluble factors in CM of GMSCs played a key role in this process by inducing apoptosis of tumor cells. GMSCs could upregulate the expression of the apoptotic associated genes Bax, cleaved poly ADP-ribose polymerase, cleaved caspase-3, and downregulate the proliferation related genes, including Bcl-2, phosphorylation extracellular signal-regulated kinase 1/2, cyclin dependent kinase 4, cyclin D1, proliferating cell nuclear antigen and survivin, which indicating that GMSCs could inhibit the growth of tongue TSCC by activating the JNK signaling pathway. In addition, subcutaneous injection of GMSCs in nude mice model significantly inhibited the growth of tumors.
As mentioned earlier, MSCs can influence tumor progression by regulating intra-tumor angiogenesis. Anti-angiogenic therapy may be a possible treatment strategy of tumors in the future. Liu et al. (2022) treated TSCC CAL27 cells with exosomes derived from human deciduous exfoliated teeth by direct multi-point intratumor injection and found that the tumor volume was significantly smaller than that of the control group. Further experiments showed that exosomes from human deciduous exfoliated teeth downregulated VEGF-A expression through miR-100-5p and miR-1246, which significantly reduced the generation of microvasculature around TSCC.
Dental pulp stem cells (DPSCs)-derived CM induced apoptosis in tumor cells, inhibited the proliferation of TSCC cells AW13516 by enhancing the expression of p16, enhanced the invasion, adhesion, and multidrug resistance of AW13516 by upregulating angiopoietin-2, epidermal growth factor, macrophage-stimulating factor, PDGF-AA, PDGF-BB, TNF-α, and IL-2, downregulating the anti-inflammatory cytokines TNF-β1, and pro-inflammatory cytokine IL-4 (Raj et al., 2021a).
Based on Ki-67 assays, Raj et al. (2021b) indicated the dual effect of MSCs on tumors. DPSCs-CM inhibited the proliferation of TSCC cells AW13516 at 50% and 100% concentrations and promoted the proliferation at a 20% concentration. Several growth factors, including VEGF, hepatocyte growth factor, angiopoietin-2, transforming growth factor (TGF)-α, stem cell factor, erythropoietin, colony-stimulating factor, fibroblast growth factor, and PDGF-BB, and pro-inflammatory cytokines, TNF-α and IL-8, may play a dominant role in promoting the proliferation of tumor cells.
4.2 BMSCs
4.2.1 Promotional Effects of BMSCs on Oral Cancer
Human BMSCs inhibited the proliferation of TSCC cells HSC-3, but promoted the invasion of tumor cells by upregulating the expression of the C-C Motif Chemokine 5. In addition, BMSCs-induced production of type I collagen after interaction with HSC-3 is associated with poor prognosis in TSCC cells patients (Salo et al., 2013).
Human BMSCs-CM was demonstrated to promote the expression of periostin, and further contribute to CAL27 progression through the activation of the phosphoinositide 3-kinase /Akt/mammalian target of rapamycin signaling pathway. In a murine model of TSCC, the authors found that BMSCs promoted tumor growth, invasion, metastasis and enhanced the expression of periostin in tumor tissues. After co-cultured with BMSCs-CM, CAL27 cells’ expressions of Snail, Twist, N-cadherin and vimentin were significantly increased, and the expression of E-cadherin was significantly decreased, which suggests that BMSCs could promote EMT of TSCC cells (Liu et al., 2018).
A series of in vitro and in vivo experiments showed that C-X-C chemokine receptor 2 expressed by BMSCs combined with IL-8 expressed by CAL27 promoted the migration of BMSCs to the tumor stroma. The TGF-β/rat sarcoma virus/rapidly accelerated fibrosarcoma/extracellular signal-regulated kinase pathway activated by BMSCs promoted EMT of TSCC, thereby promoting its proliferation, migration and infiltration (Meng et al., 2020).
4.2.2 Inhibitory Effects of BMSCs on Oral Cancer
By using hamsters OSCC model, Bruna et al. (2018) demonstrated that systematic administration of allogeneic BMSCs did not aggravate the progression of precancerous lesions. On the contrary, administration of BMSCs at the hypoplasia stage of precancerous lesions inhibited tumor growth, whereas only a small proportion of lesions transformed to OSCC by applying BMSCs at the papilloma stage.
Xie et al. (2019) demonstrated that human BMSCs could transfer microRNA-101-3p to human TSCC cells TCA8113 via exosomes, and collagen type X alpha 1 chain is negatively regulated by microRNA-101-3p as its target gene. By down-regulating collagen type X alpha 1 chain, microRNA-101-3p could inhibit the proliferation, invasion and migration of TCA8113 cells. Subcutaneous injection of human BMSCs-microRNA-101-3p in nude mice revealed a significant reduction in tumor volume and weight, confirming the inhibitory effect of BMSCs derived microRNA-101-3p on tumor growth in vivo.
Bagheri et al. (2021) demonstrated that human BMSCs-CM exhibited a time-dependent inhibitory effect on TSCC cells CAL27, which showed the lowest cells viability after 72 h of co-culture with BMSCs-CM. The decrease of proliferation marker proliferating cell nuclear antigen, anti-apoptotic marker BCL-2 and Ki67 positive cells at 24 and 72 h of co-culture indicated that BMSCs-CM decreased the proliferation of CAL27 cells and increased apoptosis.
Another study demonstrated that 3 weeks after oral tissue injection of human OSCC (moderately differentiated tumor of buccal mucosa) in nude mice, direct intra-tumor injection of mice-derived BMSCs in combination with cisplatin revealed an inhibition of tumor growth and an increase in the lifespan of the mice, these effects may be due to BMSCs’ promotion of cisplatin distribution for better anti-cancer action and increased apoptosis of tumor cells (Zurmukhtashvili et al., 2020).
4.3 Other Mesenchymal Stem Cells
Among the applications of MSCs for cancer treatment, adipose tissue derived MSCs (AMSCs) have received increasing attention due to the advantages of relatively easy collection and production. Sinha et al. (2020) demonstrated that after co-culture with human TSCC cell HSC-3, AMSCs did not induce proliferation, migration, and invasion of tumor cells, which providing preliminary evidence that AMSCs may have more suitable properties for tumor therapy relative to other types of MSCs. Because there are relatively few studies on interaction of AMSCs and tumors, more studies are needed before further applications.
In a study of human amniotic membrane MSCs (HAMCSs)-derived CM, its promoting effect on TSCC cells CAL27 was demonstrated after a 24 h of co-culture with human HAMSCs-CM, manifested by an increase in CAL27 cell viability. After 72 h of co-culture, the increased expression of proliferating cell nuclear antigen indicated that HAMSCs-CM promoted the proliferation of CAL27, and the results of flow cytometry showed a decrease in the number of apoptotic CAL27 cells (Bagheri et al., 2021).
Chen et al. (2019) demonstrated that rat oral mucosa malignancy-derived MSCs can inhibit the proliferation of T cells and promote the apoptosis of T cell through soluble factors and intercellular contacts, whereas T cell migration was not affected. The immunosuppressive effect of MSCs on T cells is enhanced with increasing tumor malignancy. The higher the number of MSCs at the tumor sites, the higher the proliferative status of tumor cells, showing that tumor-derived MSCs play an important role in the malignant progression of oral mucosa.
Increased expression of microRNA-8485 in exosomes derived from human oral leukoplakia with dysplasia and oral cancer (species not specified) derived-MSCs promotes the proliferation, migration and invasion of SCC15 cells in vitro and reduces the expression of the oncogene p53 (Li et al., 2019a). Ji et al. (2021) showed that OSCC-derived MSCs promoted the migration and invasion of OSCC cell lines CAL27 and WSU-HN6. With a significant decrease in E-cadherin, alpha E catenin and a significant increase in N-cadherin, OSCC-MSCs may promote OSCC metastasis through EMT. Further experiments showed that upregulation of CPNE7, a calcium-dependent phospholipid -binding protein in tumor-derived MSCs, promotes phosphorylation of p65 and IκBα as well as nuclear translocation of p65, which activates the NF-κB pathway, promotes the expression of IL-8, and thus promotes tumor metastasis.
5 Treatment of Oral Cancer
5.1 Current Status of Oral Cancer Treatment
The treatment of oral cancer depends mainly on the severity of the disease. OSCC is the most common type of oral cancers with a poor prognosis and a high recurrence rate (Satija et al., 2009) and a huge potential for regional metastasis even in the early stages (Vargas-Ferreira et al., 2012). A proportion of OSCC can be detected at an early stage, but current treatment modalities adversely affect patients physically and psychologically, severely affecting their quality of life. Most OSCC is not detected until advanced stage, by which time the survival rate of patients has been markedly reduced. Among the many treatment modalities, surgical treatment is the main modality for oral cancer. When the primary tumor is large and incomplete resection or signs of infiltration are suspected, radiation adjuvant therapy is administered after surgery. Molecularly targeted therapy with cetuximab added to postoperative radiotherapy, targeting the epidermal growth factor receptor, has been approved for the treatment of OSCC. Docetaxel, cisplatin and 5-fluorouracil and other anticancer drug-induced chemotherapy are usually used as induction therapy before surgery or alone or in combination with radiotherapy after surgery. Combined surgery-radiotherapy has become the standard procedure for the treatment of advanced oral cancer (Colevas et al., 2018). In recent years, antitumor immunotherapies such as the programmed death-1 inhibitor, which block tumor immunosuppressive signals and enhance antitumor immune responses by targeting the programmed death-1/ programmed death-ligand 1 pathway, have played an important role in recurrence and metastasis oral cancer (Cramer et al., 2019).
Although currently available treatment strategies include excision of malignant tissue in combination with radiotherapy and chemotherapy, the 5-years survival rate is still about 50% (Sasahira et al., 2014). The ultimate goal of surgery is to remove the tumor tissue, but inadequate removal has a high chance of causing recurrence (Brennan et al., 1995). The oral cavity as a functional organ of mastication, speech, and articulation, surgery may lead to serious aesthetic and functional problems, as well as psychological trauma for the patient. Radiotherapy can cause altered taste, dysphagia, dry mouth, and hypothyroidism, causing temporary or permanent damage to healthy tissues. Chemotherapy can also lead to severe systemic reactions such as nausea, vomiting, hair loss, infection and diarrhea, which can seriously affect patients’ health and quality of life. Therefore, it is particularly important to find new methods for the treatment of oral cancer.
As previously mentioned, MSCs can inhibit tumor progression in multiple ways, such as inhibition of angiogenesis, suppression of cell proliferation and metastasis, induction of apoptosis, cell cycle arrest, inflammatory infiltration, and regulation of oncogenes. In light of these studies on MSCs, there has been increasing interest in MSCs-based cancer therapy in recent years, and advanced approaches to modify MSCs to become powerful and precise targeting tools for killing cancer cells rather than normal healthy cells have been continuously explored.
5.2 Mesenchymal Stem Cells in the Treatment of Oral Cancer
As important participants in TME remodeling, the interaction between MSCs and tumors begins at the early stage of tumor growth, MSCs are attracted to the tumor sites and interact with surrounding cells, undergoing phenotypic and functional changes and secreting a variety of bioactive factors that can significantly alter the growth, proliferation, and apoptosis of neighboring cells, thereby affecting tumor progression. As mentioned earlier, MSCs show both promoting and inhibiting effects on oral cancer, therefore, their mechanisms should be further explored before applying MSCs to the treatment of oral cancer. In addition, circumventing the promotive effects of MSCs on oral cancer and making full use of the effects of MSCs on oral cancer, such as homing and inhibition, and using them as therapeutic drug carriers or MSCs to directly inhibit tumors would be beneficial to discover the potential applications of MSCs for oral cancer treatment.
Both wild-type MSCs as well as modified MSCs have been used for the treatment of oral cancer, as shown in Table 2. MSCs can be used as carriers for delivery of therapeutic proteins or anticancer drugs, and are genetically modified to over-express several anti-tumor factors, such as IL, IFN, pro-drugs, oncolytic viruses, pro-apoptotic proteins, anti-angiogenic agents and growth factor antagonists (Shah, 2012). MSCs can selectively migrate and aggregate at the tumor sites, thus exerting a therapeutic effect, improving therapeutic efficacy and reducing systemic toxicity.
Unlike cell therapies, MSCs derived secretomes can be better evaluated for their safety, dosage and potency. Secretomes, aside from avoiding the inconveniences of administering living proliferating cells, show other additional advantages, including cheaper, safer, and more practical for clinical use. For instance, exosomes derived from MSCs, one of the secretomes, have been evaluated for their potential to be used as drug delivery vehicles (Eiro et al., 2021). The treatments of different MSCs on oral cancer are shown in Table 2.
5.2.1 Direct Application of Mesenchymal Stem Cells
Isolated from gingival tissues, GMSCs possessed the properties of easy isolation, rapid expansion, profound immunomodulatory and anti-inflammatory functions, making them potential source for stem cell-based therapy (Ji et al., 2016). GMSCs can inhibit the growth of oral cancer cells in vitro and in vivo by altering the microenvironment of surrounding oral cancer cells, suggesting that GMSCs have potential applications in the treatment of oral dysplasia and oral cancer (Ji et al., 2016).
MSCs can influence tumor progression by regulating intra-tumor angiogenesis. Anti-angiogenic therapy may be a possible treatment strategy of tumors in the future. Liu et al. (2022) treated TSCC CAL27 cells with exosomes derived from human deciduous exfoliated teeth by direct multi-point intratumor injection and found that the tumor volume was significantly smaller than that of the control group. Further experiments showed that exosomes from human deciduous exfoliated teeth downregulated VEGF-A expression through miR-100-5p and miR-1246, which significantly reduced the generation of microvasculature around TSCC.
Up to date, the influence of BMSCs on cancer remains uncertain. Some studies have shown that BMSCs promote cancer progression, whereas others show that BMSCs suppress cancer progression, and also some studies found BMSCs have no significant impact on cancer progression (Barcellos-de-Souza et al., 2013; Gao et al., 2016; Mi and Gong, 2017; Wu et al., 2019; Zhang et al., 2019). By establishing an animal model, Bruna et al. (2017) showed that the administration of BMSCs at the precancerous stage (papilloma stage) reduced the proliferation rate of OSCC tumors and increased the apoptosis rate, thereby stopping tumor growth and preventing epithelial dedifferentiation of OSCC. Zurmukhtashvili et al. (2020) established an OSCC model in mice and demonstrated that intratumorally injected BMSCs reduce inflammation, increase micro-vascularization, and minimize hypoxia of OSCC tissues. Moreover, combined treatment with cisplatin leads to higher apoptotic activity and reduced tumor tissue growth.
5.2.2 As Drug Delivery Vehicles
GMSCs exhibit the capability to encapsulate and release anticancer drugs without any genetic changes. Coccè et al. (2017) demonstrated by in vitro experiments that GMSCs can efficiently bind three important antitumor drugs and then release them in active form: paclitaxel, doxorubicin, and gemcitabine, thereby significantly inhibiting the growth of TSCC cell SCC154, indicating that MSCs-mediated drug delivery systems have potential applications in the field of oral oncology.
Zhou et al. (2021) showed that OSCC recruits DPSCs through the CXCL8-CXCR2 axis. They used DPSCs membranes (DPSCM) modified with metal-organic framework nanoparticles (MOFs) to create a novel nanoparticle, MOF@DPSCM, which can effectively deliver antitumor drugs to target OSCC. MOF@DPSCM carries doxorubicin (DOX) can induce the death of CAL27 cells and block the growth of CAL27 tumor. The data suggests that this novel MOF-DOX@DPSCM nanoparticle is a potential targeted drug delivery system that can be used for OSCC.
Sun et al. (2020) used MSCs membrane-encapsulated oxygen-carrying perfluorocarbon and sonosensitizer verteporfin to develop a novel biomimetic sonosensitizer, named M/LPV/O2, which could induce cancer cell death by increasing uptake cancer cells and stimulating intracellular reactive oxygen species production under hypoxic conditions. M/LPV/O2 can accumulate in oral tumor, relieved hypoxia, and effectively inhibited tumor. In addition, exhibiting minimal systemic side effects, M/LPV/O2 can successfully maintain oral function without causing aesthetic problems.
5.2.3 Expression of Anti-Tumor Factors by Gene Modification
Tumor necrosis factor-related apoptosis-inducing ligand (TRAIL) is a promising target that can selectively induce apoptosis in cancer cells. Demonstrating in vitro that human GMSCs can migrate to TSCC cell lines (TCA8113 and CAL27), Xia et al. (2015) used GMSCs as a cellular vector and transduced with full-length TRAIL (GMSCs with full-length TRAIL; GMSCFLT), and found that GMSCFLT induced massive necrosis and apoptosis of tumor cells by co-culture with TCA8113 and CAL27, respectively. In addition, further antitumor assays using GMSCFLT tail vein injection into nude mice significantly inhibited the growth of TSCC. These data confirmed the tumor suppressive effect of gene therapy vectors TRAIL-expressing GMSCs.
Previous studies demonstrated the inhibitory effect of IFN-β gene-modified MSCs on breast, pancreatic and prostate cancers (Kidd et al., 2010; Ling et al., 2010; Wang et al., 2012a). Du et al. (2019) constructed IFN-β gene-modified GMSCs (GMSCs/IFN-β), and found that GMSCs/IFN-β inhibited the proliferation of CAL27 cells in vitro. Through in vivo experiments, they showed that GMSCs/IFN-β expressed high levels of IFN-β, which significantly inhibited tumor growth. Further experiments demonstrated that GMSCs/IFN-β inhibited the growth of TSCC xenografts by suppressing cell proliferation and inducing apoptosis. In addition, GMSCs are genetically modified to release other cytokines, such as IFN-γ, IL-2, IL-12, and IL-24 (Matsuzuka et al., 2010; Zhang et al., 2013; Yang et al., 2014; You et al., 2015), to achieve antitumor effects.
As previously mentioned, exosomes from human BMSCs with upregulated miR-101-3p may serve as a promising new direction for the development of oral cancer therapeutics. Human BMSCs can transfer microRNA-101-3p to human TSCC cells TCA8113 via exosomes to negatively regulate the collagen X-type α1 chain of the target gene, thereby inhibiting the proliferation, invasion and migration of TCA8113 cells (Xie et al., 2019).
Oral potentially malignant disorders are usually asymptomatic clinical lesions that appear prior to OSCC (Wang et al., 2019). Put mice genetically modified BMSCs-derived extracellular vesicles (EVs) highly expressing microRNA-185 (BMSCs-EVs-miR-185) pasted on oral potentially malignant disorders induced with dimethylbenzanthracene, and found that BMSCs-EVs-miR-185 could attenuate the inflammatory condition and reduce the dysplasia in the lesion tissue. In addition, BMSCs-EVs-miR-185 may inhibit disease progression by suppressing proliferation, angiogenesis and promoting the activation of the Akt pathway to increase apoptosis of tumor cells.
6 Concluding Remarks
Currently, MSCs are used in clinics for anti-inflammatory therapy, tissue regeneration, graft-versus-host response, autoimmune diseases and gene transfection. In addition, MSCs-based tumor therapy is promising, as MSCs can migrate directionally to target tissues to exert their own effects, and can also exert the effects of therapeutic factors by means of gene transfection and secretion of several kinds of factors, presenting the anti-tumor effects.
Under normal conditions, MSCs maintain a stable internal environment in tissues, and once a tumor develops, the normal tissue microenvironment is subsequently changed to a TME. Tumor-secreted cytokines and growth factors, such as PDGF-AB, VEGF, hepatocyte growth factor, IL-8, etc., can recruit local and distant MSCs, and MSCs can also influence tumor progression by affecting angiogenesis, regulating immunity, inducing apoptosis, inhibiting or promoting proliferation, invasion, migration, etc. As mentioned above, MSCs from different tissues have different effects on oral tumors, including those from normal, precancerous and cancerous tissues, which provide a new perspective for further exploration of oral cancer progression and treatment. At the same time, administration of different doses of MSCs may also produce different results. In addition, it seems that different results can be obtained by using different cancer models and by different routes of administration of MSCs at different times of cancer progression. Notably, anticancer treatment can also affect the recruitment of MSCs.
However, since most of the currently available evidence is obtained through non-human xenografts, the literature supporting the direct use of MSCs for the treatment of cancer patients is still insufficient and their safety remains a major consideration, and more research must be conducted to provide evidence and improve the therapeutic efficacy of MSCs in cancer treatment. These cells have great potential to revolutionize existing cancer therapies.
Author Contributions
TY designed the study, searched literature, analyzed data and wrote the article. ST and SP searched literature and analyzed data. GD designed the study, wrote the article and supervised the study.
Funding
This work was supported by grants from the Natural Science Foundation of Shandong Province (No.ZR2021MH051 to GD), the National Natural Science Foundation of China (No.81570945 to GD), and the Postgraduate Education Quality Improvement Plan of Shandong Province (No. SDYAL21150 to GD).
Conflict of Interest
The authors declare that the research was conducted in the absence of any commercial or financial relationships that could be construed as a potential conflict of interest.
Publisher’s Note
All claims expressed in this article are solely those of the authors and do not necessarily represent those of their affiliated organizations, or those of the publisher, the editors and the reviewers. Any product that may be evaluated in this article, or claim that may be made by its manufacturer, is not guaranteed or endorsed by the publisher.
References
Almeida-Porada, G., Atala, A. J., and Porada, C. D. (2020). Therapeutic Mesenchymal Stromal Cells for Immunotherapy and for Gene and Drug Delivery. Mol. Ther. - Methods & Clin. Dev. 16, 204–224. doi:10.1016/j.omtm.2020.01.005
Bagheri, R., Bitazar, R., Talebi, S., Alaeddini, M., Etemad-Moghadam, S., and Eini, L. (2021). Conditioned Media Derived from Mesenchymal Stem Cells Induces Apoptosis and Decreases Cell Viability and Proliferation in Squamous Carcinoma Cell Lines. Gene 782, 145542. doi:10.1016/j.gene.2021.145542
Barcellos-de-Souza, P., Gori, V., Bambi, F., and Chiarugi, P. (2013). Tumor Microenvironment: Bone Marrow-Mesenchymal Stem Cells as Key Players. Biochimica Biophysica Acta (BBA) - Rev. Cancer 1836 (2), 321–335. doi:10.1016/j.bbcan.2013.10.004
Brennan, J. A., Mao, L., Hruban, R. H., Boyle, J. O., Eby, Y. J., Koch, W. M., et al. (1995). Molecular Assessment of Histopathological Staging in Squamous-Cell Carcinoma of the Head and Neck. N. Engl. J. Med. 332 (7), 429–435. doi:10.1056/nejm199502163320704
Bruna, F., Arango-Rodríguez, M., Plaza, A., Espinoza, I., and Conget, P. (2017). The Administration of Multipotent Stromal Cells at Precancerous Stage Precludes Tumor Growth and Epithelial Dedifferentiation of Oral Squamous Cell Carcinoma. Stem Cell. Res. 18, 5–13. doi:10.1016/j.scr.2016.11.016
Bruna, F., Plaza, A., Arango, M., Espinoza, I., and Conget, P. (2018). Systemically Administered Allogeneic Mesenchymal Stem Cells Do Not Aggravate the Progression of Precancerous Lesions: a New Biosafety Insight. Stem Cell. Res. Ther. 9 (1), 137. doi:10.1186/s13287-018-0878-1
Bruno, S., Collino, F., Deregibus, M. C., Grange, C., Tetta, C., and Camussi, G. (2013). Microvesicles Derived from Human Bone Marrow Mesenchymal Stem Cells Inhibit Tumor Growth. Stem Cells Dev. 22 (5), 758–771. doi:10.1089/scd.2012.0304
Caulfield, T., and Ogbogu, U. (2011). Stem Cell Research, Scientific Freedom and the Commodification Concern. EMBO Rep. 13 (1), 12–16. doi:10.1038/embor.2011.232
Chai, A. W. Y., Lim, K. P., and Cheong, S. C. (2020). Translational Genomics and Recent Advances in Oral Squamous Cell Carcinoma. Seminars Cancer Biol. 61, 71–83. doi:10.1016/j.semcancer.2019.09.011
Chen, Y., Wang, X., Fang, J., Song, J., Ma, D., Luo, L., et al. (2019). Mesenchymal Stem Cells Participate in Oral Mucosa Carcinogenesis by Regulating T Cell Proliferation. Clin. Immunol. 198, 46–53. doi:10.1016/j.clim.2018.12.001
Choi, S., and Myers, J. N. (2008). Molecular Pathogenesis of Oral Squamous Cell Carcinoma: Implications for Therapy. J. Dent. Res. 87 (1), 14–32. doi:10.1177/154405910808700104
Chung, C. H., Parker, J. S., Ely, K., Carter, J., Yi, Y., Murphy, B. A., et al. (2006). Gene Expression Profiles Identify Epithelial-To-Mesenchymal Transition and Activation of Nuclear Factor-Κb Signaling as Characteristics of a High-Risk Head and Neck Squamous Cell Carcinoma. Cancer Res. 66 (16), 8210–8218. doi:10.1158/0008-5472.Can-06-1213
Coccè, V., Farronato, D., Brini, A. T., Masia, C., Giannì, A. B., Piovani, G., et al. (2017). Drug Loaded Gingival Mesenchymal Stromal Cells (GinPa-MSCs) Inhibit In Vitro Proliferation of Oral Squamous Cell Carcinoma. Sci. Rep. 7 (1), 9376. doi:10.1038/s41598-017-09175-4
Colevas, A. D., Yom, S. S., Pfister, D. G., Spencer, S., Adelstein, D., Adkins, D., et al. (2018). NCCN Guidelines Insights: Head and Neck Cancers, Version 1.2018. J. Natl. Compr. Canc Netw. 16 (5), 479–490. doi:10.6004/jnccn.2018.0026
Conrad, C., Niess, H., Huss, R., Huber, S., von Luettichau, I., Nelson, P. J., et al. (2009). Multipotent Mesenchymal Stem Cells Acquire a Lymphendothelial Phenotype and Enhance Lymphatic Regeneration In Vivo. Circulation 119 (2), 281–289. doi:10.1161/circulationaha.108.793208
Cramer, J. D., Burtness, B., and Ferris, R. L. (2019). Immunotherapy for Head and Neck Cancer: Recent Advances and Future Directions. Oral Oncol. 99, 104460. doi:10.1016/j.oraloncology.2019.104460
da Silva Meirelles, L., Caplan, A. I., and Nardi, N. B. (2008). In Search of the In Vivo Identity of Mesenchymal Stem Cells. Stem Cells 26 (9), 2287–2299. doi:10.1634/stemcells.2007-1122
Djouad, F., Plence, P., Bony, C., Tropel, P., Apparailly, F., Sany, J., et al. (2003). Immunosuppressive Effect of Mesenchymal Stem Cells Favors Tumor Growth in Allogeneic Animals. Blood 102 (10), 3837–3844. doi:10.1182/blood-2003-04-1193
Dominici, M., Le Blanc, K., Mueller, I., Slaper-Cortenbach, I., Marini, F. C., Krause, D. S., et al. (2006). Minimal Criteria for Defining Multipotent Mesenchymal Stromal Cells. The International Society for Cellular Therapy Position Statement. Cytotherapy 8 (4), 315–317. doi:10.1080/14653240600855905
Du, L., Liang, Q., Ge, S., Yang, C., and Yang, P. (2019). The Growth Inhibitory Effect of Human Gingiva-Derived Mesenchymal Stromal Cells Expressing Interferon-β on Tongue Squamous Cell Carcinoma Cells and Xenograft Model. Stem Cell. Res. Ther. 10 (1), 224. doi:10.1186/s13287-019-1320-z
Du, W., Seah, I., Bougazzoul, O., Choi, G., Meeth, K., Bosenberg, M. W., et al. (2017). Stem Cell-Released Oncolytic Herpes Simplex Virus Has Therapeutic Efficacy in Brain Metastatic Melanomas. Proc. Natl. Acad. Sci. U.S.A. 114 (30), E6157–e6165. doi:10.1073/pnas.1700363114
Eiro, N., Fraile, M., Fernández-Francos, S., Sánchez, R., Costa, L. A., and Vizoso, F. J. (2021). Importance of the Origin of Mesenchymal (Stem) Stromal Cells in Cancer Biology: "alliance" or "war" in Intercellular Signals. Cell. Biosci. 11 (1), 109. doi:10.1186/s13578-021-00620-6
Fu, J., Wang, Y., Jiang, Y., Du, J., Xu, J., and Liu, Y. (2021). Systemic Therapy of MSCs in Bone Regeneration: a Systematic Review and Meta-Analysis. Stem Cell. Res. Ther. 12 (1), 377. doi:10.1186/s13287-021-02456-w
Gao, P., Ding, Q., Wu, Z., Jiang, H., and Fang, Z. (2010). Therapeutic Potential of Human Mesenchymal Stem Cells Producing IL-12 in a Mouse Xenograft Model of Renal Cell Carcinoma. Cancer Lett. 290 (2), 157–166. doi:10.1016/j.canlet.2009.08.031
Gao, Y., Zhou, Z., Lu, S., Huang, X., Zhang, C., Jiang, R., et al. (2016). Chemokine CCL15 Mediates Migration of Human Bone Marrow-Derived Mesenchymal Stem Cells toward Hepatocellular Carcinoma. Stem Cells 34 (4), 1112–1122. doi:10.1002/stem.2275
Grigolato, R., Accorona, R., Lombardo, G., Corrocher, G., Garagiola, U., Massari, F., et al. (2021). Oral Cancer in Non-smoker Non-drinker Patients. Could Comparative Pet Oncology Help to Understand Risk Factors and Pathogenesis? Crit. Rev. Oncology/Hematology 166, 103458. doi:10.1016/j.critrevonc.2021.103458
Huang, W.-H., Chang, M.-C., Tsai, K.-S., Hung, M.-C., Chen, H.-L., and Hung, S.-C. (2013). Mesenchymal Stem Cells Promote Growth and Angiogenesis of Tumors in Mice. Oncogene 32 (37), 4343–4354. doi:10.1038/onc.2012.458
Hung, B. P., Hutton, D. L., Kozielski, K. L., Bishop, C. J., Naved, B., Green, J. J., et al. (2015). Platelet-Derived Growth Factor BB Enhances Osteogenesis of Adipose-Derived but Not Bone Marrow-Derived Mesenchymal Stromal/Stem Cells. Stem Cells 33 (9), 2773–2784. doi:10.1002/stem.2060
Janjanin, S., Djouad, F., Shanti, R. M., Baksh, D., Gollapudi, K., Prgomet, D., et al. (2008). Human Palatine Tonsil: a New Potential Tissue Source of Multipotent Mesenchymal Progenitor Cells. Arthritis Res. Ther. 10 (4), R83. doi:10.1186/ar2459
Ji, X., Sun, T., Xie, S., Qian, H., Song, L., Wang, L., et al. (2021). Upregulation of CPNE7 in Mesenchymal Stromal Cells Promotes Oral Squamous Cell Carcinoma Metastasis through the NF-Κb Pathway. Cell. Death Discov. 7 (1), 294. doi:10.1038/s41420-021-00684-w
Ji, X., Zhang, Z., Han, Y., Song, J., Xu, X., Jin, J., et al. (2016). Mesenchymal Stem Cells Derived from Normal Gingival Tissue Inhibit the Proliferation of Oral Cancer Cells In Vitro and In Vivo. Int. J. Oncol. 49 (5), 2011–2022. doi:10.3892/ijo.2016.3715
Kariminekoo, S., Movassaghpour, A., Rahimzadeh, A., Talebi, M., Shamsasenjan, K., and Akbarzadeh, A. (2016). Implications of Mesenchymal Stem Cells in Regenerative Medicine. Artif. Cells, Nanomedicine, Biotechnol. 44 (3), 749–757. doi:10.3109/21691401.2015.1129620
Kidd, S., Caldwell, L., Dietrich, M., Samudio, I., Spaeth, E. L., Watson, K., et al. (2010). Mesenchymal Stromal Cells Alone or Expressing Interferon-β Suppress Pancreatic Tumors In Vivo, an Effect Countered by Anti-inflammatory Treatment. Cytotherapy 12 (5), 615–625. doi:10.3109/14653241003631815
Lei, M., Li, K., Li, B., Gao, L.-N., Chen, F.-M., and Jin, Y. (2014). Mesenchymal Stem Cell Characteristics of Dental Pulp and Periodontal Ligament Stem Cells after In Vivo Transplantation. Biomaterials 35 (24), 6332–6343. doi:10.1016/j.biomaterials.2014.04.071
Li, W., Han, Y., Zhao, Z., Ji, X., Wang, X., Jin, J., et al. (2019a). Oral Mucosal Mesenchymal Stem Cell-derived E-xosomes: A P-otential T-herapeutic T-arget in O-ral P-remalignant L-esions. Int. J. Oncol. 54 (5), 1567–1578. doi:10.3892/ijo.2019.4756
Li, W., Zhang, X., Wu, F., Zhou, Y., Bao, Z., Li, H., et al. (2019b). Gastric Cancer-Derived Mesenchymal Stromal Cells Trigger M2 Macrophage Polarization that Promotes Metastasis and EMT in Gastric Cancer. Cell. Death Dis. 10 (12), 918. doi:10.1038/s41419-019-2131-y
Liau, L. L., Looi, Q. H., Chia, W. C., Subramaniam, T., Ng, M. H., and Law, J. X. (2020). Treatment of Spinal Cord Injury with Mesenchymal Stem Cells. Cell. Biosci. 10, 112. doi:10.1186/s13578-020-00475-3
Lindroos, B., Mäenpää, K., Ylikomi, T., Oja, H., Suuronen, R., and Miettinen, S. (2008). Characterisation of Human Dental Stem Cells and Buccal Mucosa Fibroblasts. Biochem. Biophysical Res. Commun. 368 (2), 329–335. doi:10.1016/j.bbrc.2008.01.081
Ling, X., Marini, F., Konopleva, M., Schober, W., Shi, Y., Burks, J., et al. (2010). Mesenchymal Stem Cells Overexpressing IFN-β Inhibit Breast Cancer Growth and Metastases through Stat3 Signaling in a Syngeneic Tumor Model. Cancer Microenviron. 3 (1), 83–95. doi:10.1007/s12307-010-0041-8
Liu, C., Feng, X., Wang, B., Wang, X., Wang, C., Yu, M., et al. (2018). Bone Marrow Mesenchymal Stem Cells Promote Head and Neck Cancer Progression through Periostin-Mediated Phosphoinositide 3-kinase/Akt/mammalian Target of Rapamycin. Cancer Sci. 109 (3), 688–698. doi:10.1111/cas.13479
Liu, P., Zhang, Q., Mi, J., Wang, S., Xu, Q., Zhuang, D., et al. (2022). Exosomes Derived from Stem Cells of Human Deciduous Exfoliated Teeth Inhibit Angiogenesis In Vivo and In Vitro via the Transfer of miR-100-5p and miR-1246. Stem Cell. Res. Ther. 13 (1), 89. doi:10.1186/s13287-022-02764-9
Lu, S., Fan, H., Zhou, B., Ju, Z., Yu, L., Guo, L., et al. (2012b). Fusion of Human Umbilical Cord Mesenchymal Stem Cells with Esophageal Carcinoma Cells Inhibits the Tumorigenicity of Esophageal Carcinoma Cells. Int. J. Oncol. 40 (2), 370–377. doi:10.3892/ijo.2011.1232
Matsuzuka, T., Rachakatla, R. S., Doi, C., Maurya, D. K., Ohta, N., Kawabata, A., et al. (2010). Human Umbilical Cord Matrix-Derived Stem Cells Expressing Interferon-β Gene Significantly Attenuate Bronchioloalveolar Carcinoma Xenografts in SCID Mice. Lung Cancer 70 (1), 28–36. doi:10.1016/j.lungcan.2010.01.003
McLeod, C., and Baylis, F. (2006). Feminists on the Inalienability of Human Embryos. Hypatia 21 (1), 1–14. doi:10.1111/j.1527-2001.2006.tb00961.x
Meng, L., Zhao, Y., Bu, W., Li, X., Liu, X., Zhou, D., et al. (2020). Bone Mesenchymal Stem Cells Are Recruited via CXCL8‐CXCR2 and Promote EMT through TGF‐β Signal Pathways in Oral Squamous Carcinoma. Cell. Prolif. 53 (8), e12859. doi:10.1111/cpr.12859
Mi, F., and Gong, L. (2017). Secretion of Interleukin-6 by Bone Marrow Mesenchymal Stem Cells Promotes Metastasis in Hepatocellular Carcinoma. Biosci. Rep. 37 (4). doi:10.1042/bsr20170181
Mishra, P. J., Mishra, P. J., Humeniuk, R., Medina, D. J., Alexe, G., Mesirov, J. P., et al. (2008). Carcinoma-associated Fibroblast-like Differentiation of Human Mesenchymal Stem Cells. Cancer Res. 68 (11), 4331–4339. doi:10.1158/0008-5472.Can-08-0943
Musiał-Wysocka, A., Kot, M., and Majka, M. (2019). The Pros and Cons of Mesenchymal Stem Cell-Based Therapies. Cell. Transpl. 28 (7), 801–812. doi:10.1177/0963689719837897
Oswald, J., Boxberger, S., Jørgensen, B., Feldmann, S., Ehninger, G., Bornhäuser, M., et al. (2004). Mesenchymal Stem Cells Can Be Differentiated into Endothelial Cells In Vitro. Stem Cells 22 (3), 377–384. doi:10.1634/stemcells.22-3-377
Pakravan, K., Babashah, S., Sadeghizadeh, M., Mowla, S. J., Mossahebi-Mohammadi, M., Ataei, F., et al. (2017). MicroRNA-100 Shuttled by Mesenchymal Stem Cell-Derived Exosomes Suppresses In Vitro Angiogenesis through Modulating the mTOR/HIF-1α/VEGF Signaling axis in Breast Cancer Cells. Cell. Oncol. 40 (5), 457–470. doi:10.1007/s13402-017-0335-7
Pierdomenico, L., Bonsi, L., Calvitti, M., Rondelli, D., Arpinati, M., Chirumbolo, G., et al. (2005). Multipotent Mesenchymal Stem Cells with Immunosuppressive Activity Can Be Easily Isolated from Dental Pulp. Transplantation 80 (6), 836–842. doi:10.1097/01.tp.0000173794.72151.88
Ponte, A. L., Marais, E., Gallay, N., Langonné, A., Delorme, B., Hérault, O., et al. (2007). The In Vitro Migration Capacity of Human Bone Marrow Mesenchymal Stem Cells: Comparison of Chemokine and Growth Factor Chemotactic Activities. Stem Cells 25 (7), 1737–1745. doi:10.1634/stemcells.2007-0054
Raj, A. T., Kheur, S., Bhonde, R., Mani, V. R., Baeshen, H. A., and Patil, S. (2021a). Assessing the Effect of Human Dental Pulp Mesenchymal Stem Cell Secretome on Human Oral, Breast, and Melanoma Cancer Cell Lines. Saudi J. Biol. Sci. 28 (11), 6556–6567. doi:10.1016/j.sjbs.2021.07.029
Raj, A. T., Kheur, S., Khurshid, Z., Sayed, M. E., Mugri, M. H., Almasri, M. A., et al. (2021b). The Growth Factors and Cytokines of Dental Pulp Mesenchymal Stem Cell Secretome May Potentially Aid in Oral Cancer Proliferation. Molecules 26 (18), 5683. doi:10.3390/molecules26185683
Rehman, A. O., and Wang, C.-Y. (2008). SDF-1α Promotes Invasion of Head and Neck Squamous Cell Carcinoma by Activating NF-Κb. J. Biol. Chem. 283 (29), 19888–19894. doi:10.1074/jbc.M710432200
Salo, S., Bitu, C., Merkku, K., Nyberg, P., Bello, I. O., Vuoristo, J., et al. (2013). Human Bone Marrow Mesenchymal Stem Cells Induce Collagen Production and Tongue Cancer Invasion. PLoS One 8 (10), e77692. doi:10.1371/journal.pone.0077692
Sasahira, T., Kurihara, M., Yamamoto, K., Ueda, N., Nakashima, C., Matsushima, S., et al. (2014). HuD Promotes Progression of Oral Squamous Cell Carcinoma. Pathobiology 81 (4), 206–214. doi:10.1159/000366022
Satija, N. K., Singh, V. K., Verma, Y. K., Gupta, P., Sharma, S., Afrin, F., et al. (2009). Mesenchymal Stem Cell-Based Therapy: a New Paradigm in Regenerative Medicine. J. Cell. Mol. Med. 13 (11-12), 4385–4402. doi:10.1111/j.1582-4934.2009.00857.x
Shah, K. (2012). Mesenchymal Stem Cells Engineered for Cancer Therapy. Adv. Drug Deliv. Rev. 64 (8), 739–748. doi:10.1016/j.addr.2011.06.010
Shi, S., and Gronthos, S. (2003). Perivascular Niche of Postnatal Mesenchymal Stem Cells in Human Bone Marrow and Dental Pulp. J. Bone Min. Res. 18 (4), 696–704. doi:10.1359/jbmr.2003.18.4.696
Shi, Y., Du, L., Lin, L., and Wang, Y. (2017). Tumour-associated Mesenchymal Stem/stromal Cells: Emerging Therapeutic Targets. Nat. Rev. Drug Discov. 16 (1), 35–52. doi:10.1038/nrd.2016.193
Shi, Y., Wang, Y., Li, Q., Liu, K., Hou, J., Shao, C., et al. (2018). Immunoregulatory Mechanisms of Mesenchymal Stem and Stromal Cells in Inflammatory Diseases. Nat. Rev. Nephrol. 14 (8), 493–507. doi:10.1038/s41581-018-0023-5
Sinha, S., Narjus-Sterba, M., Tuomainen, K., Kaur, S., Seppänen-Kaijansinkko, R., Salo, T., et al. (2020). Adipose-Derived Mesenchymal Stem Cells Do Not Affect the Invasion and Migration Potential of Oral Squamous Carcinoma Cells. Ijms 21 (18), 6455. doi:10.3390/ijms21186455
Sonoyama, W., Liu, Y., Yamaza, T., Tuan, R. S., Wang, S., Shi, S., et al. (2008). Characterization of the Apical Papilla and its Residing Stem Cells from Human Immature Permanent Teeth: a Pilot Study. J. Endod. 34 (2), 166–171. doi:10.1016/j.joen.2007.11.021
Sun, L., Xu, Y., Zhang, X., Gao, Y., Chen, J., Zhou, A., et al. (2020). Mesenchymal Stem Cells Functionalized Sonodynamic Treatment for Improving Therapeutic Efficacy and Compliance of Orthotopic Oral Cancer. Adv. Mat. 32 (48), 2005295. doi:10.1002/adma.202005295
Sun, Z., Wang, S., and Zhao, R. C. (2014). The Roles of Mesenchymal Stem Cells in Tumor Inflammatory Microenvironment. J. Hematol. Oncol. 7, 14. doi:10.1186/1756-8722-7-14
Sung, H., Ferlay, J., Siegel, R. L., Laversanne, M., Soerjomataram, I., Jemal, A., et al. (2021). Global Cancer Statistics 2020: GLOBOCAN Estimates of Incidence and Mortality Worldwide for 36 Cancers in 185 Countries. CA A Cancer J. Clin. 71 (3), 209–249. doi:10.3322/caac.21660
Tavakoli, S., Ghaderi Jafarbeigloo, H. R., Shariati, A., Jahangiryan, A., Jadidi, F., Jadidi Kouhbanani, M. A., et al. (2020). Mesenchymal Stromal Cells; a New Horizon in Regenerative Medicine. J. Cell. Physiology 235 (12), 9185–9210. doi:10.1002/jcp.29803
Tomchuck, S. L., Zwezdaryk, K. J., Coffelt, S. B., Waterman, R. S., Danka, E. S., and Scandurro, A. B. (2008). Toll-like Receptors on Human Mesenchymal Stem Cells Drive Their Migration and Immunomodulating Responses. Stem Cells 26 (1), 99–107. doi:10.1634/stemcells.2007-0563
Urbanek, K., Cesselli, D., Rota, M., Nascimbene, A., De Angelis, A., Hosoda, T., et al. (2006). Stem Cell Niches in the Adult Mouse Heart. Proc. Natl. Acad. Sci. U.S.A. 103 (24), 9226–9231. doi:10.1073/pnas.0600635103
Vargas-Ferreira, F., Nedel, F., Etges, A., Gomes, A. P. N., Furuse, C., and Tarquinio, S. B. C. (2012). Etiologic Factors Associated with Oral Squamous Cell Carcinoma in Non-smokers and Non-alcoholic Drinkers: a Brief Approach. Braz. Dent. J. 23 (5), 586–590. doi:10.1590/s0103-64402012000500020
Wang, F., Yu, M., Yan, X., Wen, Y., Zeng, Q., Yue, W., et al. (2011). Gingiva-derived Mesenchymal Stem Cell-Mediated Therapeutic Approach for Bone Tissue Regeneration. Stem Cells Dev. 20 (12), 2093–2102. doi:10.1089/scd.2010.0523
Wang, G.-X., Zhan, Y.-A., Hu, H.-L., Wang, Y., and Fu, B. (2012a). Mesenchymal Stem Cells Modified to Express Interferon-β Inhibit the Growth of Prostate Cancer in a Mouse Model. J. Int. Med. Res. 40 (1), 317–327. doi:10.1177/147323001204000132
Wang, L., Yin, P., Wang, J., Wang, Y., Sun, Z., Zhou, Y., et al. (2019). Delivery of Mesenchymal Stem Cells-Derived Extracellular Vesicles with Enriched miR-185 Inhibits Progression of OPMD. Artif. Cells, Nanomedicine, Biotechnol. 47 (1), 2481–2491. doi:10.1080/21691401.2019.1623232
Whiteside, T. L. (2018). Exosome and Mesenchymal Stem Cell Cross-Talk in the Tumor Microenvironment. Seminars Immunol. 35, 69–79. doi:10.1016/j.smim.2017.12.003
Widdows, H., Marway, H., and Johnson, S.-L. (2014). “Commodification of Human Tissue,” in Handbook of Global Bioethics. Editor H. ten Have (Springer), 581–598.
Wu, D.-M., Wen, X., Han, X.-R., Wang, S., Wang, Y.-J., Shen, M., et al. (2019). Bone Marrow Mesenchymal Stem Cell-Derived Exosomal MicroRNA-126-3p Inhibits Pancreatic Cancer Development by Targeting ADAM9. Mol. Ther. - Nucleic Acids 16, 229–245. doi:10.1016/j.omtn.2019.02.022
Xia, L., Peng, R., Leng, W., Jia, R., Zeng, X., Yang, X., et al. (2015). TRAIL-expressing Gingival-Derived Mesenchymal Stem Cells Inhibit Tumorigenesis of Tongue Squamous Cell Carcinoma. J. Dent. Res. 94 (1), 219–228. doi:10.1177/0022034514557815
Xie, C., Du, L.-Y., Guo, F., Li, X., and Cheng, B. (2019). Exosomes Derived from microRNA-101-3p-Overexpressing Human Bone Marrow Mesenchymal Stem Cells Suppress Oral Cancer Cell Proliferation, Invasion, and Migration. Mol. Cell. Biochem. 458 (1-2), 11–26. doi:10.1007/s11010-019-03526-7
Xu, M., Wei, X., Fang, J., and Xiao, L. (2019). Combination of SDF-1 and bFGF Promotes Bone Marrow Stem Cell-Mediated Periodontal Ligament Regeneration. Biosci. Rep. 39 (12), BSR20190785. doi:10.1042/bsr20190785
Yang, X., Du, J., Xu, X., Xu, C., and Song, W. (20142014). IFN-γ-Secreting-Mesenchymal Stem Cells Exert an Antitumor EffectIn Vivovia the TRAIL Pathway. J. Immunol. Res. 2014, 1–9. doi:10.1155/2014/318098
You, Q., Yao, Y., Zhang, Y., Fu, S., Du, M., and Zhang, G. (2015). Effect of Targeted Ovarian Cancer Therapy Using Amniotic Fluid Mesenchymal Stem Cells Transfected with Enhanced Green Fluorescent Protein-Human Interleukin-2 In Vivo. Mol. Med. Rep. 12 (4), 4859–4866. doi:10.3892/mmr.2015.4076
Zhang, X., Sai, B., Wang, F., Wang, L., Wang, Y., Zheng, L., et al. (2019). Hypoxic BMSC-Derived Exosomal miRNAs Promote Metastasis of Lung Cancer Cells via STAT3-Induced EMT. Mol. Cancer 18 (1), 40. doi:10.1186/s12943-019-0959-5
Zhang, X., Zhang, L., Xu, W., Qian, H., Ye, S., Zhu, W., et al. (2013). Experimental Therapy for Lung Cancer: Umbilical Cord-Derived Mesenchymal Stem Cell-Mediated Interleukin-24 Delivery. Curr. Cancer Drug Targets 13 (1), 92–102. doi:10.2174/156800913804486665
Zhou, D., Chen, Y., Bu, W., Meng, L., Wang, C., Jin, N., et al. (2021). Modification of Metal-Organic Framework Nanoparticles Using Dental Pulp Mesenchymal Stem Cell Membranes to Target Oral Squamous Cell Carcinoma. J. Colloid Interface Sci. 601, 650–660. doi:10.1016/j.jcis.2021.05.126
Zurmukhtashvili, M., Machavariani, A., Dugashvili, G., Grdzelidze, T., Gogilashvili, K., Menabde, G., et al. (2020). Mesenchymal Stem Cell Transplantation Attenuates Growth of Chemotherapy Treated Oral Squamous Cell Carcinoma in an Animal Model. J. Oral Pathol. Med. 49 (7), 655–664. doi:10.1111/jop.13006
Keywords: mesenchymal stem cells, oral cancer, tumor microenvironment, therapy, delivery vehicles
Citation: Yang T, Tang S, Peng S and Ding G (2022) The Effects of Mesenchymal Stem Cells on Oral Cancer and Possible Therapy Regime. Front. Genet. 13:949770. doi: 10.3389/fgene.2022.949770
Received: 21 May 2022; Accepted: 10 June 2022;
Published: 30 June 2022.
Edited by:
Ousheng Liu, Central South University, ChinaReviewed by:
Shaohua Ge, Shandong University, ChinaHongzhi Quan, Central South University, China
Haixia Zhang, Central South University, China
Copyright © 2022 Yang, Tang, Peng and Ding. This is an open-access article distributed under the terms of the Creative Commons Attribution License (CC BY). The use, distribution or reproduction in other forums is permitted, provided the original author(s) and the copyright owner(s) are credited and that the original publication in this journal is cited, in accordance with accepted academic practice. No use, distribution or reproduction is permitted which does not comply with these terms.
*Correspondence: Gang Ding, ZGluZ2dhbmdAd2ZtYy5lZHUuY24=