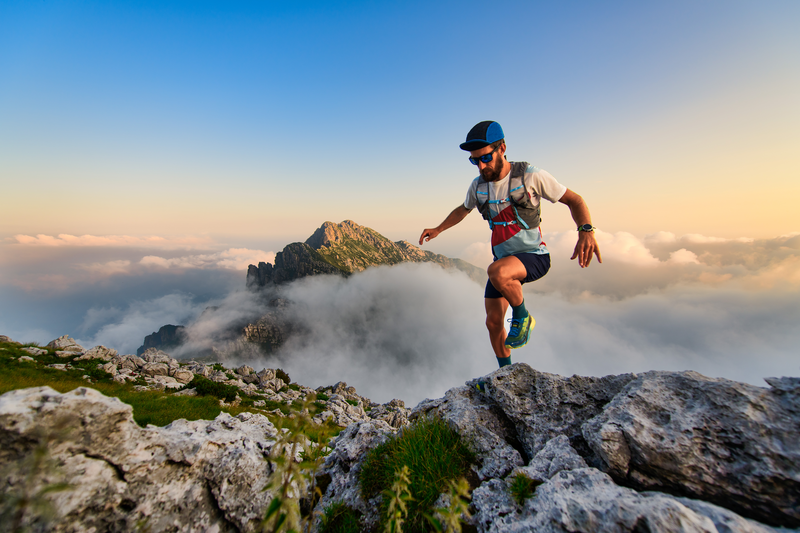
95% of researchers rate our articles as excellent or good
Learn more about the work of our research integrity team to safeguard the quality of each article we publish.
Find out more
ORIGINAL RESEARCH article
Front. Genet. , 30 September 2022
Sec. Genomics of Plants and the Phytoecosystem
Volume 13 - 2022 | https://doi.org/10.3389/fgene.2022.941954
This article is part of the Research Topic Accelerating Genetic Gain for Key Traits Using Genome-Wide Association Studies and Genomic Selection: Promising Breeding Tools for Sustainable Agriculture View all 11 articles
Ovate family proteins (OFPs) are transcriptional inhibitors that regulate plant growth and development and play important roles in the synthesis of secondary cell walls during pollen development. This study identified the pepper OFP gene family based on the genome-wide analysis and used bioinformatics methods to provide a fundamental profile of the gene family. 74 OFP genes with typical Ovate domain were identified in cultivated pepper Zunla-1, wild pepper Chiltepin and CM334. Chromosome mapping revealed that CazOFP genes were unevenly distributed on 11 chromosomes and Chr00 in Zunla-1, CacOFP genes on 12 chromosomes in Chiltepin, and CamOFP genes on 12 chromosomes and two Scaffflods in CM334. Gene structure analysis revealed that CaOFP genes possessed 1-3 exons, and the analysis of physicochemical properties suggested that CaOFPs were hydrophilic. Many cis-acting elements were identified in the promoter region of CaOFP genes, including ABRE, ARE, Box 4, G-box, TC-rich, and TCT-motif. The expression patterns of pepper at different growth stages showed that CaOFP genes were actively involved in the growth and fruit development of pepper, and CazOFP16 and CazOFP17 were actively involved in response to multiple hormones and stress events. qRT-PCR was also used to verify the expression of CazOFP gene in two developmental stages of seven pepper varieties with different fruit shapes, and it was found that CaOFP genes may be involved in the formation of fruit type in pepper. This study provides theoretical and practical evidence for future research on the OFP gene family.
Transcription factors (TFs) play fundamental roles in the growth and development of higher plants, as well as in their responses to the external environment. Typical TFs in higher plants possess DNA-binding domains, transcription regulation domains, oligomerization sites, and nuclear localization signals; the interaction between these domains and cis-acting elements enables TFs to regulate gene expression (Jiang et al., 2011). Ovate family proteins (OFPs) are plant-specific, multigene family members usually with a conserved Ovate domain. Homologs of OFPs have been reported in higher plants, mosses, and lycophytes (Wang et al., 2016).
Pepper is an important vegetable with high standards for its quality, spiciness, and shape, but less research has been done to study its fruit shape. Fruit shape is one of the most important quality traits of pepper, and is also one of the main attributes for the classification of pepper fruit types (Paran and Van Der Knaap, 2007). In crops, yield is regulated by environmental factors and fruit morphology. Genes regulating fruit morphology were first identified in tomatoes, and OFP genes are highly involved in this process (Liu et al., 2002; Snouffer et al., 2020). According to previous studies, OFP genes were found to be involved in the regulation of shape in different varieties of crops, such as rice, tomato, and melon. Moreover, overexpression of OFP destroyed plant organ shape in the process of phytohormone regulation, which suggested that OFP genes are involved in regulating hormones and signaling pathways within developing organs (Snouffer et al., 2020). OFP genes related to pepper fruit shape were cloned from different pepper varieties, and there were significant differences among the different fruit shapes (Tsaballa et al., 2011). A recent study revealed that virus-induced gene silencing of the pepper ortholog CaOFP20 resulted in increased fruit elongation on two independent backgrounds (Borovsky et al., 2021).
OFP genes play important roles in the growth and development of plants, including flowering (Yuan et al., 2016), growth of roots and stems (Xu et al., 2018), and formation of secondary cell walls (Li et al., 2011). Yuan et al. (2016) detected 12 VvOFP genes in different tissues, and the expression levels of VvOFP1, VvOFP2, VvOFP4, VvOFP5, VvOFP6, VvOFP7, VvOFP12, and VvOFP17 were relatively high in the week before flowering, suggesting that OFP TFs regulate fruit development during anthesis and the week before anthesis, whereas VvOFP5 and VvOFP2 are involved in the regulation of flowering and reproductive growth, respectively (Yuan et al., 2016). Li et al. (2011) also found that AtOFP4 regulates the formation of secondary cell walls via its interaction with the KNAX7 protein.
In this study, we systematically characterized the CaOFP transcription factors at the genome-wide level, analyzed its phylogenetic tree, physicochemical properties, conserved structure, chromosomal localization, response to biotic and abiotic stresses, and provided a theoretical basis for studying the function of OFP genes in pepper.
Seven pepper varieties with different fruit shapes were used in this study. Plants were grown in the greenhouse of the department of modern agriculture, Zunyi vocational and technical college in the spring of 2022 (Zunyi, Guizhou, 107°045 ′E, 27°710′ N). The pepper fruits were harvested at two different developmental stages including fruit with mature green and fruit at breaker plus 5 days. All experiments contained three biological repeats, and each replicate was a pooled sample of 10 fruits of uniform size from five individual plants. In total, 42 samples were immediately frozen in liquid nitrogen. RNA was extracted from the collected samples using the TianGene RNA Extraction Kit (DP432, Beijing, China). We then added fruit material with a weight of 50–100 ng for aseptic freezing grinding; 450 μL for oscillation mixing. This was transferred to the CS filter column and centrifuged for 3 min (12,000 rpm). The supernatant was transferred from the collection tube with a pipette gun to the Rnase-free centrifuge tube. Then, the supernatant was added and 0.5 times of anhydrous ethanol was mixed into the centrifuge tube and then transferred to the adsorption column CR3 for centrifugation for 30 s (12,000 rpm). A drop of 80 μL DNase I was added to the center of the collecting tube and left at room temperature for 15 min 250 μL of protein-removing solution RW1 was added to the adsorption column CR3, and left to stand at room temperature for 2 min, before being centrifuged for 30 s (12,000 rpm) (this procedure was repeated once). We then took an enzyme-free centrifuge tube and placed the adsorption column in a new centrifuge tube for several minutes (until the rinsing solution RW was dried). 50 μL Rnase-free ddH2O was then vertically added to the adsorption column, and the obtained RNA was stored at −80°C for further Quantitative Real-Time Polymerase Chain Reaction (qRT-PCR).
The whole-genome data (v2.0) of Capsicum annuum L. (Zunla-1 and Chiltepin) were downloaded from the database (http://peppersequence.genomics.cn) (Qin et al., 2014). CM334 genome sequence was downloaded from National Center for Biotechnology Information (https://www.ncbi.nlm.nih.gov/, GCA_000512255.2) (S Kim et al., 2014). To identify the OFP TFs, the Pfam model (PF04844) was downloaded from the Pfam database (Mistry et al., 2021), and the HMMER package was used for whole genomic and proteomic alignments. The verification was performed using an online tool, the HMMER web server (https://www.ebi.ac.uk/Tools/hmmer/) (Potter et al., 2018). AtOFPS were obtained from the Arabidopsis reference genome (https://www.arabidopsis.org/).
The phylogenetic tree was constructed based on the CaOFPs protein sequences in pepper and AtOFPs protein sequences in Arabidopsis thaliana. MEGA X was used to construct a phylogenetic tree of CaOFP genes according to the neighbor-joining method with 1000 bootstrap reiterations (Kumar et al., 2016). The structures of CaOFP genes were analyzed using the online tool GSDS 2.0 (http://gsds.gao-lab.org/) (Hu et al., 2015), and the analysis of conserved motifs was conducted using Meme (https://meme-suite.org/meme/) (Bailey et al., 2015) and visualized using TBtools (Chen et al., 2020). Physicochemical properties of the OFP gene family were analyzed using the online tool Expasy (Artimo et al., 2012).
The structure file of OFP genes in pepper was extracted by TBtools to determine their starting and ending position in the chromosomes (Chen et al., 2020). The protein database was constructed using the Zunla-1 genomic protein file, Chiltepin genomic protein file and CM334 genomic protein file for comparison. The collinearity analysis was performed using MCScanX software (Wang et al., 2013). The chromosome mapping and collinearity results of OFP genes were visualized using TBtools (Chen et al., 2020).
The online tool Pal2nal (http://www.bork.embl.de/pal2nal/) (Suyama et al., 2006) was used for the analysis of selection pressure of nine pairs of paralogous genes. The 2000-bp upstream sequences of the OFP genes were extracted using Bedtools (Quinlan, 2014), analyzed using the online tool Plantcare (Lescot et al., 2002), and visualized using TBtools.
Taking zunla-1 as the reference genome, the gene expression data of pepper were obtained from the NCBI (Accession No. GSE45037) and Pepper Information Data Center (Qin et al., 2014; Liu et al., 2017) (http://pepperhub.hzau.edu.cn). Experimental treatment and data analysis were conducted as described by (Liu et al., 2017), and the gene expression data were visualized using TBtools (Chen et al., 2020).
Expression pattern analysis was investigated using qRT-PCR. Its amplification was carried out using SYBR Green Pro Taq HS (Takara, Dalian, China) according to the manufacturer’s instructions. qRT-PCR was performed on an Applied Biosystems 7500 Real-Time PCR System (Applied Biosystems, Foster City, CA, United States) using the following program: 95°C for 30 s, followed by 40 cycles of 95°C for 5 s and 60°C for 30 s. The CaActin (GenBank No. DQ832719) and CaUbiquitin (GenBank No. AY496112) pepper genes was amplified as two control genes. Three biological replicates and three measurements for each replicate were performed under identical conditions. Analysis of the relative mRNA expression data was performed using 2–ΔΔCt (Livak and Schmittgen, 2001). All primers, designed by Primer3plus (http://www.primer3plus.com/cgi-bin/dev/primer3plus.cgi) and used in this study, were listed in Supplementary Table S1.
After aligning to the pepper proteomic database, 74 CaOFP genes were identified in Zunla-1, Chiltepin and CM334 (Table 1; Supplementary Table S2), and the conserved structures were found in all CaOFP genes. Phylogenetic data showed that the pepper OFP genes were divided into two subfamilies, including Class I and II. The number of CaOFP genes of Zunla-1, Chiltepin and CM334 in two subfamilies was 12, 12, and 13, respectively (Figure 1). All OFP genes were named according to their positions on the chromosomes. CaOFPs consisted of 103–436 amino acids; their molecular mass ranged from 11.82 to 49.45 (kDa), and the isoelectric point (pI) ranged from 4.33 to 9.94. The results indicated that CaOFPs are amphoteric proteins, with the instability index ranging from 31.34 to 76.51. The total average hydrophilicity was between -1.030–0.072, and almost all CaOFPs were hydrophilic proteins except for CamOFP25, which was 0.072 hydrophobic protein.
FIGURE 1. Phylogenetic analysis of OFP genes in pepper. Caz: Zunla-1; Cac: Chiltepin; Cam: CM334. The solid diamond with red color represented CazOFP gene of Zunla-1; the solid star with green color represented CacOFP gene of Chiltepin; and the solid circle with blue color represents CamOFP gene of CM334.
The motif analysis of OFP genes based on 10 motifs showed that the highest number of motifs was recorded for CazOFP17, CazOFP21, CacOFP17, CacOFP18, CacOFP20, CacOFP21, CamOFP10 and CamOFP20, containing 9 motifs (Figure 2C). According to their motifs, Class I can be divided into motif 1, motif 2, motif 4, motif 6 and motif 9 as Class I-A subclade and motif 1, motif 2, motif 4, motif 6, motif 7, motif 9 as Class I-B subclade, and OFP genes in Group I only contained 4–5 motifs. The 16 OFP genes in Class I-A subclade did not contain introns, while the Class I-B subclade had 1-2 exons. The largest number of exons was presented by CacOFP18, which contained five exons, and the number of exons in Class II was higher than that in Class I. Sequence comparison of 74 pepper OFP genes showed that all OFP genes contained motif1 and 69 OFP genes contained motif2 with the typical conserved structure of OVATE domain except for CazOFP24, CacOFP2, CamOFP4, CamOFP8, and CamOFP19 (Figure 3). Gene structure analysis showed that the OFP genes possessed 1-5 exons (Figure 2D).
FIGURE 2. Characteristics analysis of all OFP gene family in pepper. [(A,B) Phylogenetic tree analysis, (C) Gene motifs, (D) Gene structure].
Chromosome mapping analysis showed that 24 CazOFP genes were unevenly distributed on 11 chromosomes and Chr00 in Zunla-1, and 24 CacOFP genes unevenly on 12 chromosomes in Chiltepin (Figure 4A). Among them, ZChr10 and CChr10 had the highest number of OFP genes (8 and 7 genes, respectively), while Chr04, Chr05, Chr07, and Chr012 in Zunla-1 and Chiltepin had only one OFP gene each. Interestingly, only ZChr00 contained one OFP gene Six genes (CaOFP15–CaOFP21) on ZChr10 and CChr10 were tandem repeats with colinear regions as well as other chromosomes in Zunla-1 and Chiltepin, but ZChr08 vs. CChr08 and CChr00 vs. ZChr00 did not have colinear regions (Figure 4A). In another comparison (Figure 4B), most chromosomes had colinear regions except for ZChr00, ZChr08, MChr08, and scaffold2890 in Zunla-1 or CM334.
FIGURE 4. Chromosomal localization and collinearity analysis of OFP genes in pepper. [(A) ZChr: Zunla-1; CChr: Chiltepin. (B) ZChr: Zunla-1; MChr: CM334].
Gene synonymous substitution rate (Ks), non-synonymous substitution rate (Ka), and their ratio (Ka/Ks) can reflect the selection pressure in the evolutionary process. The evolutionary selection pressure (Ka/Ks) of the seven pairs of paralogous genes identified among 74 CaOFP genes were greater than one, suggesting that OFP genes were subjected to positive selection. Out of these, nine pairs were under evolutionary selection pressure. The Ka/Ks of two pairs (CacOFP6-CacOFP4 and CamOFP14-CamOFP18) were less than one, indicating that they were under purification selection, while their Ka/Ks of the other seven pairs were more than one, while the other seven pairs were all above 1, indicating that these genes were under positive selection during the evolution of the CaOFP genes in pepper (Table 2; Supplementary Table S3).
The analysis of the 2000-bp upstream sequence of CaOFP genes showed that the OFP gene family contained numerous basic elements, such as the CAAT box and TATA box (Figure 5), as well as the ABA-related cis-acting element ABRE, anaerobic induction-required cis-acting element ARE, photoresponse-related conserved element Box 4, photoresponse-related cis-acting element G-box, defense and stress response-related cis-acting element TC-rich repeats, photoresponse element TCT-motif, cis-acting element involved in salicylic acid responsiveness TCA-element, MYB binding site involved in drought-inducibility MBS and cis-acting element involved in low-temperature responsiveness LTR (Supplementary Table S4). It was speculated that these genes may respond to biological stress and be involve in plant growth.
The expression levels of 24 CazOFP genes in five tissues including developing roots, developing stems, mature leaves, closed flower buds and open flowers from Zunla-1 pepper data were analyzed. It showed that CazOFP17∼CazOFP21 were not expressed, and other 19 CazOFP were expressed in five different tissues on different levels (Figure 6A). During the nine stages of fruit development in Zunla-1, 22 CazOFP genes except for CazOFP18 and CazOFP20 were expressed in the first eight stages, showing that these CazOFP genes were mainly involved in early fruit development. One gene (CazOFP10), by contrast, was mainly involved in late fruit development (Figure 6B).
FIGURE 6. Expression pattern of OFP genes in pepper.[(A) Roots: developing roots; Stems: developing stems; Leaves: mature leaves; Bud: closed flower buds; Flowers: open flowers. (B) Dev1: Fruits with length between 0–1 cm; Dev2: Fruits with length between 1–3 cm; Dev3: Fruits with length between 3–4 cm; Dev4: Fruits with length between 4–5 cm; Dev5: mature green fruit; Dev6: Fruit at breaker (fruit turning red); Dev7: Fruit at breaker plus 3 days; Dev8: Fruit at breaker plus 5 days; Dev9: Fruit at Breaker plus 7 days].
As showed in Figure 7, the expression of CazOFP16, CazOFP17, and CazOFP18 in pepper leaves was up-regulated upon salicylic acid (SA, 2 mM) treatment. Jasmonic acid (JA, 10 μM) treatment similarly up-regulated the expression of CazOFP16 and CazOFP17. Pepper OFP genes expression was mainly up-regulated in roots after indole acetic acid (IAA, 2 μM) treatment; the expression of CazOFP24 in roots was higher than in leaves at a late expression stage. When treated with gibberellic acid (GA3, 2 μM), the expression of CazOFP16, CazOFP17, and CazOFP21 was higher than that of other OFP genes, and the level of up-regulation was greater in roots than in leaves. Abscisic acid (ABA, 30 μM) treatment up-regulated the expression of CazOFP11 and CazOFP12 in roots at stage AR4, while CazOFP17 had the highest expression level in leaves at stage AL5. The expression of CazOFP17 was significantly up-regulated upon salicylic acid (SA, 2 mM), JA, IAA, GA3, and ABA treatments, which suggested that CazOFP17 participates in plant response to the hormone, whereas CazOFP18, CazOFP19, and CazOFP20 were not expressed.
FIGURE 7. Analysis of CazOFP gene expression profiles in pepper. Red: up-regulated expression; Gray: not expressive; Blue: down-regulated expression. CL: Control Leaf; SL: SA Leaf; JL:JA Leaf; IL: IAA Leaf; GL: GA3 Leaf; CR: Control Root; SR:SA Root; JR: JA Root; IR: IAA Root; GR: GA3 Root; AL: ABA Leaf; FL: Cold Leaf; RL: H2O2 Leaf; HL: Heat Leaf; ML: Mannitol Leaf; NL: NaCl Leaf; AR: ABA Root; FR: Cold Root; RR: H2O2 Root; HR: Heat Root; MR: Mannitol Root; NR: NaCl Root.
The expression of CazOFP17 was up-regulated in roots under cold stress at stage FR1 (Root/Freezing 1), and the expression of CazOFP16 was up-regulated in leaves at stage RL3 when treated with H2O2 (Figure 7). Heat stress up-regulated the expression of CazOFP16 in roots at stage HR3 and that of CazOFP17 in leaves at stage HL5 (Figure 7). When treated with D-mannitol, the expression of CazOFP15 was up-regulated in leaves; the expression of CazOFPs was higher in roots than in leaves. Salt stress up-regulated the expression of CazOFP16 in leaves at stage NL4 (Figure 7). In summary, CazOFP18-21 were not expressed, and the number of genes expressed in roots was higher than that in leaves.
In order to verify wheather CaOFP genes involve in pepper development and fruit shape formation (Figure 8A), eight CazOFP genes were randomly selected and qRT-PCR performed. These genes except for CazOFP12 were mainly expressed in developmental stage 1 (fruit with mature green) of seven pepper varieties with different fruit shapes (Figure 8B), indicating that these genes were mainly involved in early fruit development, which were consistent with transcriptome results mentioned above. In seven pepper varieties with different fruit shapes, the expression of CazOFP3 and CazOFP12 at developmental stage 2 (fruit at breaker plus 5 days) in T803 was higher than that at developmental stage 1 (fruit with mature green), as well as CazOFP14 in Zunla-1. Moreover, CazOFP3, CazOFP9 and CazOFP14 were expressed in two developmental stages of seven pepper varieties, showing that the three genes may be involved in the formation of pepper fruit shape.
FIGURE 8. Expression of eight CazOFP genes in two developmental stages of seven pepper varieties with different fruit shapes. (A) Fruit diagrams of seven varieties with different fruit shapes. These varieties (M1-M7) are zunla-1, ZJ-5, 11C255-1, ZJ11, HYL, ZJ-1 and T803. Above is the fruit development stage 1 (fruit with mature green), Below is the fruit development stage 2 (fruit at breaker plus 5 days). (B) The levels of these eight CazOFP genes detected by qRT-PCR.
We identified 24 CaOFP genes in cultivated pepper Zunla-1, 24 in wild pepper Chiltepin, and 26 in another cultivated pepper CM334. So far, OFP genes have been identified in many species with the development of genome sequencing, such as 18 AtOFP genes in A. thaliana (Wang et al., 2011), 35 RsOFP genes in Raphanus sativus, 31 SlOFP genes in Solanum lycopersicum (Huang et al., 2013), 29 BrOFP genes in Brassica rapa (Wang et al., 2021), and 8 VvOFP genes in Vitis vinifera (Wang et al., 2018).
In reported studies, the tomato SlOFP genes can be divided into three subfamilies (Huang et al., 2013), and the apple OFP genes can be divided into 15 subfamilies by carrying out evolutionary analysis of 727 protein sequences from 32 species (Li et al., 2019), while Liu et al. found that OFP genes in angiosperms were divided into 11 subfamilies by phylogenetic tree analysis (Liu et al., 2014). In the study, the CaOFP genes were divided into two subfamilies, namely Class I and Class II by identifying and classifying three varieties of pepper (Zunla-1, Chiltepin and CM334). However, these CaOFP genes can be subdivided according to their conserved domains and motifs. For example, Class I contains only six motifs, while Class II contains motif1, motif5, motif8, and motif10. These results indicated that the subfamily classification of OFP genes was quite different according to their plant properties, conserved domains and exon size. In a previous study, OFP genes of Selaginella moellendorffii, an early terrestrial plant, were found to have more than dozens of exons (Dangwal and Das, 2018), while there were 1-5 exons in the CaOFP gene family of pepper, and Class I-A subclade did not contain introns according to its subgroup classification.
Some studies have found that OFP was not only involved in the regulation of plant growth and development, but also in plant stress response and fruit morphology formation. Liu et al. cloned OVATE in tomato for the first time and found that it could control the shape of tomato fruit. A point mutation of OVATE gene led to premature termination of translation, which increased the longitudinal diameter of wild tomato fruit and limited the growth of its neck, thus developing from round fruit to pear fruit (Liu et al., 2002). In the yeast two-hybrid assay, nine OVATE proteins were found to interact with TALE homeobox proteins, and AtOFP1 and AtOFP5 co-regulated the subcellular localization of a TALE homeobox protein BLH1. When these two genes were co-expressed in tobacco leaves, The BLH1 protein was transported from the nucleus to the cytoplasm (Hackbusch et al., 2005). Numerous studies have shown that OFP played a role by interacting with KNOX and BELL transcription factors. For example, Schmitz et al. found that OsOFP2 could regulate KNOX-BELL function to participate in the development process of rice (Schmitz et al., 2015). Another study found that the CmOFP13 gene fsqs8.1 could alter the recessive structure of the gene in melon, which could lead to structural variation and affect the melon fruit morphology (Martínez et al., 2022). Current biochemical evidence suggests that OFPs may function in this feedback through interactions with three amino acid loop extension (TALE) proteins, as well as through interactions with additional proteins within signaling pathways (Snouffer et al., 2020). Expression patterns of OFP genes in different tissues and development stages of pepper and seven pepper varieties with different fruit shapes indicated that some CaOFP genes were actively involved in the growth and development of pepper, and participated in plant stress response process and fruit morphological development through mediating mediates.
Recent studies have found that OFP gene was responsive to salt stress in plants. Tang et al. found that PpOFP1 had salt tolerance through yeast experiments in vitro (Tan et al., 2021). Ma et al. found that the OsOFP6 overexpression lines had slower water loss and less H2O2 accumulation under drought condition, while the RNAi lines had faster water loss and higher H2O2 content, which indicated that OsOFP6 may have drought resistance in rice (Ma et al., 2017). Characterization of CaOFP genes expression patterns under different stresses in pepper showed that CazOFP15, CazOFP16 and CazOFP17 can respond to regulation and participate in the salt stress process. Aabscisic acid responsiveness, salicylic acid responsiveness, defense and stress responsiveness, drought-inducibility, low-temperature responsiveness were also found in the upstream 2000 bp of pepper CaOFP genes. Defense and stress responsiveness, drought-inducibility, low-temperature responsiveness, etc. Now studies on OFP mainly focus on growth and development, including defense and stress response, low temperature response and drought induction, while no other studies under SA, JA, IAA, GA3, ABA, H2O2, Mannitol stress have been reported. According to the analysis of cis-acting elements in pepper, It is speculated that CazOFP plays a functional role in responding to relevant elements, which provides new insights for subsequent studies on pepper’s response to biotic and abiotic stresses. By studying the expression profile of CazOFP genes under different tissues and stress conditions, this study provides theoretical support for studying the stress response process of OFP and its participation in the regulation of growth and development in pepper.
OFPgenes have been found to reduce the length of hypocotyls, rosette leaves, stem leaves, inflorescence stems and floral organs in Arabidopsis mutants, while AtOFP1 regulates the target gene AtGA20ox1, resulting in insufficient gibberellin synthesis. These results indicated that OFP transcription factors negatively regulated plant growth and development (Wang et al., 2007). OFP can also promote chlorophyll accumulation and delay leaf senescence. Zhou et al. overexpressed SlOFP20 in tomato and found that the expressions of genes encoding transcription factors SlGLK1, SlGLK2 and HY5 related to chloroplast development and chlorophyll level were significantly up-regulated, which indicated that OFP had a positive regulatory effect on chlorophyll accumulation and retarding leaf senescence (Zhou et al., 2019). At present, it had been reported that OFP was involved in the fruit shape development process of pepper, and CaOVATE was found to be involved in the fruit shape process of tomato through the study of pepper varieties with different fruit shapes (Tsaballa et al., 2011). Expression pattern analysis of Citrus OFPs (CitOFPs) showed that CitOFP19 had significantly higher expression level in the ovaries of round pummelo than in those of pear-shaped pummelo (Wu et al., 2022). We also found that the CazOFP genes were up-regulated in the early stages of pepper fruit development (Dev1∼Dev5) in the study. However, CazOFPs expression tended to be down-regulated in the late fruit stages. The expression levels of CazOFP9 and CazOFP13 in thin pepper varieties were higher than those in large pepper varieties, suggesting that CazOFP9 and CazOFP13 were involved in the fruit shape development of thin pepper varieties. In brief, CazOFP genes with different expression levels in pepper may be involved in plant growth and development, thus playing both positive and negative regulatory roles.
Fruit shape is an important quality and yield trait of pepper. High quality pepper and excellent fruit shape help to improve its market competitiveness and increase economic output value. The mechanism and functional verification of OFP transcription factors regulating the growth and development of pepper will become the focus of future research. With the development of plant genome field, it is helpful to explore more OFP gene functions and provide theoretical and practical support for the study of plant shape and morphological development.
Publicly available datasets were analyzed in this study. The names of the repository/repositories and accession number(s) can be found in the article/Supplementary Material.
YL, SY, XL, XZ, and CQ conceived and designed the experiments and drafted the manuscript. JL, TL, XT, and FL performed the experiments. YL, SY, and CQ analyzed the data. All authors read and approved the manuscript.
This work was supported by the Guizhou Province Science and technology plan program of China (Qian Kehe support Nos. [2021]1Y088, [2021] yiban267, [2019]2413 and [2022] yiban117), the Zunyi Excellent Young Scientific and Technological Innovative Talents Training Project (Zunyouqingke No. 201806), and the Zunyi Innovative Talent Team Training Project of China (Zunshike rencai No. 201904).
The authors declare that the research was conducted in the absence of any commercial or financial relationships that could be construed as a potential conflict of interest.
All claims expressed in this article are solely those of the authors and do not necessarily represent those of their affiliated organizations, or those of the publisher, the editors and the reviewers. Any product that may be evaluated in this article, or claim that may be made by its manufacturer, is not guaranteed or endorsed by the publisher.
The Supplementary Material for this article can be found online at: https://www.frontiersin.org/articles/10.3389/fgene.2022.941954/full#supplementary-material
Artimo, P., Jonnalagedda, M., Arnold, K., Baratin, D., Csardi, G., De Castro, E., et al. (2012). Expasy: Sib bioinformatics resource portal. Nucleic Acids Res. 40 (W1), W597–W603. doi:10.1093/nar/gks400
Bailey, T. L., Johnson, J., Grant, C. E., and Noble, W. S. (2015). The meme suite. Nucleic Acids Res. 43 (W1), W39–W49. doi:10.1093/nar/gkv416
Borovsky, Y., Raz, A., Doron-Faigenboim, A., Zemach, H., Karavani, E., and Paran, I. (2021). Pepper fruit elongation is controlled by Capsicum annuum ovate family protein 20. Front. Plant Sci. 12, 815589. doi:10.3389/fpls.2021.815589
Chen, C., Chen, H., Zhang, Y., Thomas, H. R., Frank, M. H., He, Y., et al. (2020). TBtools: An integrative toolkit developed for interactive analyses of big biological data. Mol. Plant 13 (8), 1194–1202. doi:10.1016/j.molp.2020.06.009
Dangwal, M., and Das, S. (2018). Identification and analysis of OVATE family members from genome of the early land plants provide insights into evolutionary history of OFP family and function. J. Mol. Evol. 86, 511–530. doi:10.1007/s00239-018-9863-7
Hackbusch, J., Richter, K., Müller, J., Salamini, F., and Uhrig, J. F. (2005). A central role of Arabidopsis thaliana ovate family proteins in networking and subcellular localization of 3-aa loop extension homeodomain proteins. Proc. Natl. Acad. Sci. U. S. A. 102 (13), 4908–4912. doi:10.1073/pnas.0501181102
Hu, B., Jin, J., Guo, A., Zhang, H., Luo, J., and Gao, G. (2015). GSDS 2.0: An upgraded gene feature visualization server. Bioinformatics 31 (8), 1296–1297. doi:10.1093/bioinformatics/btu817
Huang, Z., Houten, J. V., Gonzalez, G., Xiao, H., and Knaap, E. (2013). Genome-wide identification, phylogeny and expression analysis of SUN, OFP and YABBY gene family in tomato. Mol. Genet. Genomics 288 (3), 111–129. doi:10.1007/s00438-013-0733-0
Jiang, T., Lin, Y. X., Liu, X., Jiang, H. Y., and Zhu, S. W. (2011). Genome-wide analysis of the WRKY transcription factor family in Medicago truncatula. Acta Prat. Sin. 20 (3), 211–218. doi:10.11686/cyxb20110326
Kim, S., Park, M., Yeom, S. I., Kim, Y. M., Lee, J. M., Lee, H-A., et al. (2014). Genome sequence of the hot pepper provides insights into the evolution of pungency in Capsicum species. Nat. Genet. 46, 270–278. doi:10.1038/ng.2877
Kumar, S., Stecher, G., and Tamura, K. (2016). MEGA7: Molecular evolutionary genetics analysis version 7.0 for bigger datasets. Mol. Biol. Evol. 33 (7), 1870–1874. doi:10.1093/molbev/msw054
Lescot, M., Déhais, P., Thijs, G., Marchal, K., Moreau, Y., Van de Peer, Y., et al. (2002). PlantCare, a database of plant cis-acting regulatory elements and a portal to tools for in silico analysis of promoter sequences. Nucleic Acids Res. 30 (1), 325–327. doi:10.1093/nar/30.1.325
Li, E., Wang, S., Liu, Y., Chen, J. G., and Douglas, C. J. (2011). Ovate family protein4 (OFP4) interaction with KNAT7 regulates secondary cell wall formation in Arabidopsis thaliana. Plant J. 67 (2), 328–341. doi:10.1111/j.1365-313X.2011.04595.x
Li, H., Dong, Q., Zhao, Q., and Ran, K. (2019). Genome-wide identification, expression profiling, and protein-protein interaction properties of ovate family proteins in apple. Tree Genet. Genomes 15, 45. doi:10.1007/s11295-019-1354-5
Liu, D., Sun, W., Yuan, Y., Zhang, N., Hayward, A., Liu, Y., et al. (2014). Phylogenetic analyses provide the first insights into the evolution of OVATE family proteins in land plants. Ann. Bot. 113 (7), 1219–1233. doi:10.1093/aob/mcu061
Liu, F., Yu, H., Deng, Y., Zheng, J., Liu, M., Ou, L., et al. (2017). Pepperhub, an informatics hub for the chili pepper research community. Mol. Plant 10 (8), 1129–1132. doi:10.1016/j.molp.2017.03.005
Liu, J., Van Eck, J., Cong, B., and Tanksley, S. D. (2002). A new class of regulatory genes underlying the cause of pear-shaped tomato fruit. Proc. Natl. Acad. Sci. U. S. A. 99 (20), 13302–13306. doi:10.1073/pnas.162485999
Livak, K. J., and Schmittgen, T. D. (2001). Analysis of relative gene expression data using real-time quantitative PCR and the 2(-Delta Delta C(T)) Method. Methods 25 (4), 402–408. doi:10.1006/meth.2001.1262
Ma, Y., Yang, C., He, Y., Tian, Z., and Li, J. (2017). Rice OVATE family protein 6 regulates plant development and confers resistance to drought and cold stresses. J. Exp. Bot. 68, 4885–4898. doi:10.1093/jxb/erx309
Martínez, C., Gonzalo, M., Sipowicz, P., Campos, M., Martínez, F. I., Leida, C., et al. (2022). A cryptic variation in a member of the Ovate Family Proteins is underlying the melon fruit shape QTL fsqs8.1. Theor. Appl. Genet. 135, 785–801. doi:10.1007/s00122-021-03998-6
Mistry, J., Chuguransky, S., Williams, L., Qureshi, M., Salazar, G. A., Sonnhammer, E. L., et al. (2021). Pfam: The protein families database in 2021. Nucleic Acids Res. 49 (D1), D412–D419. doi:10.1093/nar/gkaa913
Paran, I., and Van Der Knaap, E. (2007). Genetic and molecular regulation of fruit and plant domestication traits in tomato and pepper. J. Exp. Bot. 58 (14), 3841–3852. doi:10.1093/jxb/erm257
Potter, S. C., Luciani, A., Eddy, S. R., Park, Y., Lopez, R., and Finn, R. D. (2018). Hmmer web server: 2018 update. Nucleic Acids Res. 46 (W1), W200-W204–W204. doi:10.1093/nar/gky448
Qin, C., Yu, C., Shen, Y., Fang, X., Chen, L., Min, J., et al. (2014). Whole-genome sequencing of cultivated and wild peppers provides insights into capsicum domestication and specialization. Proc. Natl. Acad. Sci. U. S. A. 111 (14), 5135–5140. doi:10.1073/pnas.1400975111
Quinlan, A. R. (2014). BEDTools: The swiss‐army tool for genome feature analysis. Curr. Protoc. Bioinforma. 47 (1), 11–34. doi:10.1002/0471250953.bi1112s47
Schmitz, A. J., Begcy, K., Sarath, G., and Walia, H. (2015). Rice Ovate Family Protein 2 (OFP2) alters hormonal homeostasis and vasculature development. Plant Sci. 241, 177–188. doi:10.1016/j.plantsci.2015.10.011
Snouffer, A., Kraus, C., and Knaap, E. (2020). The shape of things to come: Ovate family proteins regulate plant organ shape. Curr. Opin. Plant Biol. 53, 98–105. doi:10.1016/j.pbi.2019.10.005
Suyama, M., Torrents, D., and Bork, P. (2006). PAL2NAL: Robust conversion of protein sequence alignments into the corresponding codon alignments. Nucleic Acids Res. 34 (2), W609–W612. doi:10.1093/nar/gkl315
Tan, Q., Jiang, S., Wang, N., Liu, X., Zhang, X., Wen, B., et al. (2021). OVATE family protein PpOFP1 physically interacts with PpZFHD1 and confers salt tolerance to tomato and yeast. Front. Plant Sci. 12, 759955. doi:10.3389/fpls.2021.759955
Tsaballa, A., Pasentsis, K., Darzentas, N., and Tsaftaris, A. S. (2011). Multiple evidence for the role of an ovate-like gene in determining fruit shape in pepper. BMC Plant Biol. 11 (1), 46–16. doi:10.1186/1471-2229-11-46
Wang, L., Zhang, S., Zhang, X., Hu, X., Guo, C., Wang, X., et al. (2018). Evolutionary and expression analysis of Vitis vinifera OFP gene family. Plant Syst. Evol. 304, 995–1008. doi:10.1007/s00606-018-1528-x
Wang, R., Han, T., Sun, J., Xu, L., Liu, C., Cao, H., et al. (2021). Genome-wide identification and characterization of the OFP gene family in Chinese cabbage ( Brassica rapa L. ssp. pekinensis ). PeerJ 9, e10934. doi:10.7717/peerj.10934
Wang, S., Chang, Y., and Ellis, B. (2016). Overview of ovate family proteins, a novel class of plant-specific growth regulators. Front. Plant Sci. 7, 417. doi:10.3389/fpls.2016.00417
Wang, S., Chang, Y., Guo, J., and Chen, J. G. (2007). Arabidopsis Ovate Family Protein 1 is a transcriptional repressor that suppresses cell elongation. Plant J. 50, 858–872. doi:10.1111/j.1365-313X.2007.03096.x
Wang, S., Chang, Y., Guo, J., Zeng, Q., Ellis, B. E., and Chen, J. G. (2011). Arabidopsis ovate family proteins, a novel transcriptional repressor family, control multiple aspects of plant growth and development. Plos One 6, e23896. doi:10.1371/journal.pone.0023896
Wang, Y., Li, J., and Paterson, A. H. (2013). MCScanX-transposed: Detecting transposed gene duplications based on multiple colinearity scans. Bioinformatics 29 (11), 1458–1460. doi:10.1093/bioinformatics/btt150
Wu, Q., Sun, u., Fu, J., Yu, H., Wang, X., Wang, S., et al. (2022). Genome-wide identification of ovate family in Citrus and functional characterization of CitOFP19. Plant Sci. 321, 111328. doi:10.1016/j.plantsci.2022.111328
Xu, R., Li, R., Wang, X., and Hao, Y. (2018). Identification and expression analysis under abiotic stresses of ofp gene family in apple. Sci. Agr. Sin. 51 (10), 1948–1959. doi:10.3864/j.issn.0578-1752.2018.10.014
Yuan, Y., Zhang, Y. G., Gao, S. M., and Tao, J. M. (2016). Bioinformatics and expression of the ovate gene family in grape. Sci. Agr. Sin. 49 (19), 3786–3797. doi:10.3864/j.issn.0578-1752.2016.19.010
Keywords: pepper, OFP genes, phylogenetic tree, expression characteristics analysis, qRT-PCR
Citation: Luo Y, Yang S, Luo X, Li J, Li T, Tang X, Liu F, Zou X and Qin C (2022) Genome-wide analysis of OFP gene family in pepper (Capsicum annuum L.). Front. Genet. 13:941954. doi: 10.3389/fgene.2022.941954
Received: 12 May 2022; Accepted: 13 September 2022;
Published: 30 September 2022.
Edited by:
Dwijesh Chandra Mishra, Indian Council of Agricultural Research, IndiaReviewed by:
Jun You, Oil Crops Research Institute (CAAS), ChinaCopyright © 2022 Luo, Yang, Luo, Li, Li, Tang, Liu, Zou and Qin. This is an open-access article distributed under the terms of the Creative Commons Attribution License (CC BY). The use, distribution or reproduction in other forums is permitted, provided the original author(s) and the copyright owner(s) are credited and that the original publication in this journal is cited, in accordance with accepted academic practice. No use, distribution or reproduction is permitted which does not comply with these terms.
*Correspondence: Xuexiao Zou, em91eHVleGlhbzQyOEAxNjMuY29t; Cheng Qin, cWluY2hlbmcxMDAxQDE2My5jb20=
†These authors have contributed equally to this work
Disclaimer: All claims expressed in this article are solely those of the authors and do not necessarily represent those of their affiliated organizations, or those of the publisher, the editors and the reviewers. Any product that may be evaluated in this article or claim that may be made by its manufacturer is not guaranteed or endorsed by the publisher.
Research integrity at Frontiers
Learn more about the work of our research integrity team to safeguard the quality of each article we publish.