- Department of Gastroenterology, the First Affiliated Hospital of Nanchang University, Nanchang, China
DNA in cells is frequently damaged by endogenous and exogenous agents. However, comprehensive mechanisms to combat and repair DNA damage have evolved to ensure genomic stability and integrity. Improper DNA damage repair may result in various diseases, including some types of tumors and autoimmune diseases. Therefore, DNA damage repair mechanisms have been proposed as novel antitumor drug targets. To date, numerous drugs targeting DNA damage mechanisms have been developed. For example, PARP inhibitors that elicit synthetic lethality are widely used in individualized cancer therapies. In this review, we describe the latent DNA damage repair mechanisms in gastric cancer, the types of DNA damage that can contribute to the development of gastric cancer, and new therapeutic approaches for gastric cancer that target DNA damage repair pathways.
Introduction
According to the 2020 Global Cancer Statistics, gastric cancer was responsible for 1,089,103 new cases in 2020 and approximately 768,793 deaths (corresponding to 1 in every 13 deaths universally) worldwide, making it the fifth most frequently diagnosed cancer and the fourth leading cause of cancer-related death (Hyuna et al., 2021). Gastric cancer is the fourth and seventh leading cause of new cancer cases in males and females, respectively, and the fourth and fifth leading cause of cancer-related death in males and females, respectively (Hyuna et al., 2021). Most new cases occur in developing countries, especially in China (Zhou et al., 2020). The global incidence of gastric cancer is 42.6% and the mortality rate is 45.0% (Ferlay et al., 2014). Data from cancer registries have revealed that gastric cancer is more likely to occur in locations where individuals have unhealthy diets, such as remote rural areas (Hongli et al., 2017).
Hence, novel strategies are urgently being researched and developed to improve the prognosis and survival of patients with gastric cancer. Twenty years ago, some studies demonstrated that DNA damage repair (DDR) mechanisms play a significant role in the tumorigenesis, progression, and treatment validity of gastric cancer (Hoeijmakers, 2001). In recent years, some clinical and preclinical studies have concluded that DDR pathway inhibitors may prolong the survival time of patients (Young et al., 2016).
Latent DNA Damage Repair Mechanisms
Genome instability leads to mutations in DNA repair genes and is a symbol of cancer evolution and one of the universal mechanisms of tumorigenesis (Tian et al., 2015). DNA damage refers to the physical or chemical changes in the DNA found in cells, affecting the interpretation and transmission of genetic information. Extensive DNA damage may subsequently activate oncogenes or inactivate tumor suppressor genes, such as p53 (Kim et al., 2019a). To repair this DNA damage, cells have developed a remarkable mechanism—DDR pathways. DDR pathways play a crucial role in the development of human cells and can repair different types of DNA damage (single-strand breaks (SSBs), pyrimidine dimers, A–C or A–G or T–C or T–G mismatches, DNA interstrand crosslinks, and double-strand breaks (DSBs)) to maintain genomic stability (Sokolova and Naumann, 2019a; Rahman et al., 2020). This damage can be caused by both exogenous and endogenous factors, such as replication fork stalling, reactive oxygen species (ROS) generation, genetically toxic substances, and ultraviolet rays (Sokolova and Naumann, 2019a).
Many signaling pathways and over 450 related proteins are involved in DDR processes (Jackson and Bartek, 2009; Pearl et al., 2015; Mauri et al., 2020). In addition, different DNA repair mechanisms are used to repair different types of damage, such as homologous recombination repair, nonhomologous end joining (NHEJ), base excision repair (BER), nucleotide excision repair, and mismatch repair (Huang and Zhou, 2020). These repair methods and the outcomes of DNA damage are shown in Figure 1. DDR processes are important for protecting against tumorigenesis. Defects in gene expression and genomic instability are associated with a high risk of gastric cancer and significantly contribute to tumorigenesis and gastric cancer development. The survival of DDR patients with a defective DDR can be improved by the development of therapeutics targeting the DDR.
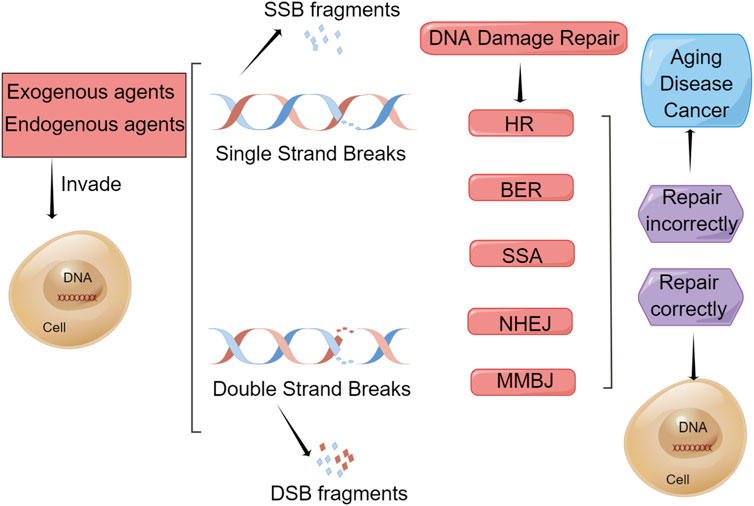
FIGURE 1. Repair methods and outcomes of DNA damage. Endogenous and exogenous factors can damage DNA in a cell, such as reactive oxygen species (ROS) generation, replication fork stalling, chemical agents, ultraviolet radiation, and ionizing radiation, which cause single-strand breaks (SSBs) and double-strand breaks (DSBs) to produce SSB fragments and DSB fragments, respectively. To mitigate this damage, cells have evolved certain types of DNA damage repair (DDR) mechanism. BER is used to repair SSBs and HR, NHEJ, MMEJ, and SSA are used to repair DSBs. If repaired correctly, the cell will remain a healthy cell. However, if repaired incorrectly, these cells will undergo senescence and apoptosis, leading to aging, disease, and cancer.
Many genes also play an important role in the process of DDR, such as 53BP1, BRCA1, RAD51, ATM, and ATR. 53BP1 is a key mediator involved in DSB repair, maintaining the balance between repair pathway selection and genome stability. Recent evidence suggests a molecular mechanism that encodes 53BP1 and DNA break response effectors to DSB sites and promotes NHEJ-mediated DSB repair through 53BP1 (Lei et al., 2022). The roles of the BRCA1 and RAD51 genes are mainly to maintain genome integrity through different mechanisms in response to DNA damage, and its maladjustment is related to the development of a tumor and the change of sensitivity to chemotherapy drugs. Studies have shown that high cytoplasmic expression of BRCA1 has a higher overall survival (OS) rate in gastric cancer, whereas nuclear expression of BRCA1 usually indicates adverse outcomes (Wang et al., 2018). Studies also have shown that gastric cancer tissues have higher levels of RAD51 expression compared with normal tissues (Xu et al., 2021). Therefore, BRCA1 and RAD51 can be used as biomarkers for the clinical diagnosis of gastric cancer to evaluate the prognostic effect of the disease. ATM is involved in DDR through downstream interactions with BRCA1 and other proteins involved in DSB repair. DNA damage-induced ATM activation promotes β-TRCP-mediated ubiquitination and destruction of ARID1A in gastric cancer cells, thereby exacerbating the development of gastric cancer (Jiang et al., 2019). Moreover, novel studies revealed that germline pathogenic variants in ATM genes are associated with a high and moderate risk of many cancers (Hall et al., 2021). ATR is considered an important direction in cancer therapy because of its deleterious effects on cancer cells that contain defects in homologous recombination. Recent studies have shown that AZD6738, as a novel oral ATR inhibitor, can induce synthetic death of gastric cancer cells through ATM defect (Min et al., 2017; Sheridan, 2018). Understanding the role and association of these genes in DDR will facilitate further clinical research to develop new treatment strategies for gastric cancer. Some genes/proteins that function in DDR in gastric cancer are shown in Table 1.
In addition, the cell cycle checkpoint genes Wee1, CHK1, and CDK also play irreplaceable roles. The CDK family plays a key role in regulating multiple signaling pathways concerning transcription and cell cycle processes. CDK affects DNA repair and contributes to the fidelity of cell division and the maintenance of genomic integrity after DNA damage (Kciuk et al., 2022). It has been shown that reduced Wee1 activity leads to ectopic activation of CDK1 activity, which drives unrepaired DNA into mitosis prematurely, leading to mutations (Bukhari et al., 2022). Therefore, Wee1 inhibitors can also be used to treat different types of cancer and regulate therapeutic immune responses. CHK1 is a key regulator of the cell cycle in DDR. CHK1 plays an important role in promoting the survival and growth of gastric cancer cells, which is an effective therapeutic target for gastric cancer. Recent clinical trials have shown that CHK1 inhibitor LY2606368 can induce DNA damage and inhibit cancer proliferation (Koustas et al., 2020). However, other researchers have speculated that CHK1 inhibitors may also lead to CHK1 inhibitor toxicity by increasing DNA damage in nontumor cells (Brooks et al., 2021). If some inhibitor compounds acting on a DDR can be found to achieve the targeting therapy, it will pave the way for the treatment of gastric cancer and improve the survival time and the prognosis of patients.
DNA Damage Repair in Gastric Cancer Because of Helicobacter pylori Infection and Other Risk Factors
Risk factors for gastric cancer include many nonmodifiable variables, such as age, sex, and race. Other risk factors are controllable, such as infection with Helicobacter pylori, smoking, and a diet high in nitrites and nitrates (Lawrence et al., 2012). There are also several relatively rare risk factors, such as a history of previous stomach surgery and hereditary diffuse gastric cancer (CDH1) (Samantha et al., 2015). Next, we will focus on the mechanism by which Helicobacter pylori and other risk factors cause gastric cancer.
Nitrite is a common potential risk factor for gastric cancer. Because the stomach is acidic, nitrite is protonated in the stomach to form nitrous acid. Nitrous acid reacts directly with DNA to form a deaminated base. All of these changes lead to mutations when DNA polymerase is at work replicating DNA (Zhang et al., 2019). Nitrite can also react with food to form N-nitrosamines, some of which are carcinogens to humans because they can react with DNA as alkylation reagents to produce admixtures that can cause harm. At the same time, irregular diet, environmental factors, drinking, and smoking can also lead to tumorigenesis and progression of gastric cancer (Praud et al., 2018).
Helicobacter pylori is a strongly virulent Gram-negative bacterium that colonizes the stomach of almost half of all people and is classified as a class Ⅰ carcinogen of gastric cancer (Helicobacter pylori Infection, 2022). Helicobacter pylori is a dominant hazard factor for the occurrence mechanism of gastric cancer and is responsible for approximately 90% of new cases. Helicobacter pylori can induce DNA damage that can lead to genomic instability and ultimately result in the formation of gastric cancer (Sokolova and Naumann, 2019b). People infected with the bacterium frequently suffer chronic nonatrophic gastritis, eventually resulting in gastric cancer through a complex series of changes. The way in which Helicobacter pylori causes DNA damage and then results in gastric cancer has always been a hot topic of research. Next, we summarized the interaction between Helicobacter pylori and DNA damage and the effects of this damage on the development of gastric cancer.
Certain cancers in the body develop as a result of the direct carcinogenic effects of certain tumorigenic substances or as a result of genomic instability caused by accompanying inflammation and DNA damage (Kim et al., 2019b). Helicobacter pylori infection can induce both innate and adaptive immune responses, including oxidative stress, which leads to DNA damage (Reyes and Peniche, 2019). The build-up of DNA damage can eventually lead to mutations that activate or inactivate tumor suppressor genes. Oxidative stress leads to the production of ROS/RON, and their concentration determines their effect on the body (Han et al., 2022). ROS/RON-mediated DNA damage leads to the breakage of chemical bonds, which is the most common mechanism of carcinogenesis after Helicobacter pylori infection. Once the body is infected by Helicobacter pylori, innate and acquired immune responses occur, and then an interrelated cellular response produces ROS/RON, leading to oxidative stress. According to previous studies, APE-1 is the main regulator of the cellular response to oxidative stress. APE-1 can repair the sites of oxidative damage in DNA, reduce the activity reduction of many transcription factors, decrease the damage because of ROS and RON in cells and tissues, and maintain the mitochondrial function (Futagami et al., 2008). APE-1 is involved in the transcriptional regulation of genes involved in the adaptive response to oxidative stress and the BER pathway.
ROS and RON are also involved in many related pathways in the body. The PLK1/P13K/Akt pathway plays an important role in the development of gastric cancer and is inseparably related to ROS and RON. Next, we will introduce the mechanisms of the PLK1/P13K/Akt pathway in detail. Helicobacter pylori can express CagA, a virulence factor that is recognized by cells and phosphorylated by Src family kinases (Zhu et al., 2016). CagA possesses functions that are distinct from conventional toxins and can counterbalance the activity of the established Helicobacter pylori toxin VacA. Meanwhile, phosphorylated CagA can regulate the expression of PLK1 and then phosphorylate PTEN and AKT, while nonphosphorylated CagA can regulate PDK1 to some extent (Su et al., 2022). The activation of AKT kinase leads to the activation of the mTOR complex, producing ROS/RON, and the accumulation of RON/RON in cells leads to DNA damage and genomic instability, leading to gastric cancer. The mechanism by which Helicobacter pylori induces DNA damage through the PLK1/P13K/Akt signaling pathway, leading to gene stability and ultimately gastric cancer, is shown in Figure 2.
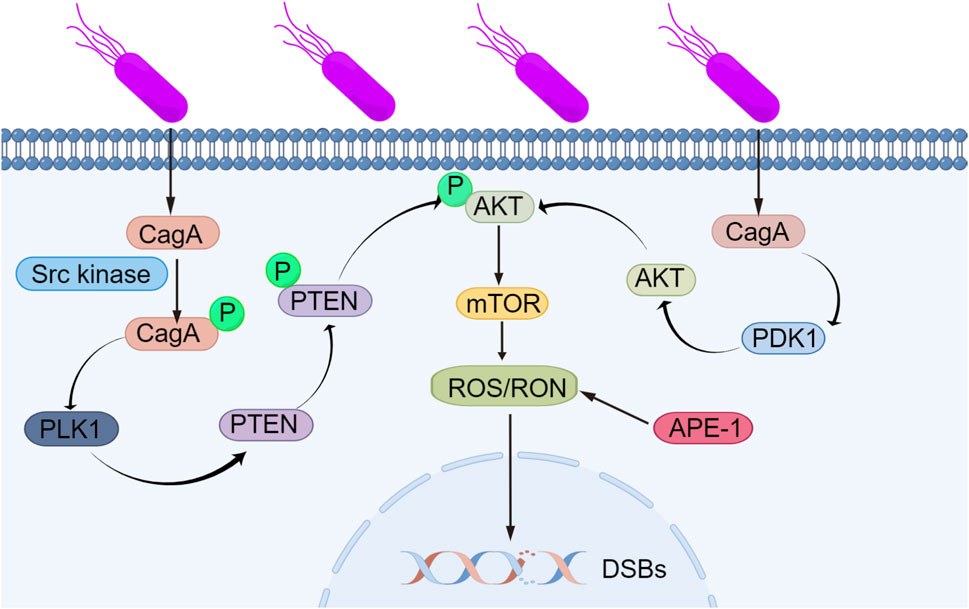
FIGURE 2. Mechanism by which Helicobacter pylori induces DNA damage, leading to genomic instability. Helicobacter pylori can express the virulence factor CagA, which is recognized by cells and phosphorylated via Src family kinases. Phosphorylated CagA affects the expression of PLK1, leading to the phosphorylation of PTEN and AKT, while unphosphorylated CagA interacts with PDK1. The activation of AKT kinase leads to subsequent activation of the mTOR complex, producing ROS/RON, and the accumulation of RON/RON in cells causes DNA damage and genomic instability, leading to gastric cancer.
Research results have shown that long-term Helicobacter pylori infection interferes with the activity of the electron transport chain and damages oxidative phosphorylation, resulting in changes in APE-1 gene expression and decreasing APE-1 expression. Furthermore, there is a certain relationship between APE-1 gene polymorphisms and the tumorigenesis and development of gastric cancer. Chronic Helicobacter pylori infection may inhibit APE-1 expression and ultimately lead to genomic instability (Chattopadhyay et al., 2010). Genomic instability is an evolving feature that is caused by mutations in DNA repair genes to drive the development of cancer (Asatryan and Komarova, 2016). Genomic instability includes microsatellite instability (MSI), chromosomal instability (CIN), and the improper activation of telomerase. Microsatellites are repeats of DNA sequences that are almost randomly distributed in all genomes (Polom et al., 2018a). During the process of replication and recombination, errors such as the insertion or deletion of bases can lead to gene mutations that cause MSI. MSI results in the abnormal expression of target genes and ultimately leads to the tumorigenesis of gastric cancer (Silva-Fernandes et al., 2017). As important evidence, the expression rate of MSI is more obvious in elderly women with gastric cancer (Polom et al., 2018b).
High-frequency mutations in microsatellite regions are an indicator of MSI in the DNA sequence, but if such genomic changes occur at the chromosomal level, it is regarded as CIN (Maleki and Röcken, 2017), and its definition continues to change as different types of cancer are continually studied. Some groups have referred to CIN as aneuploidy or polyploidy, while others have defined CIN as multiple structural rearrangements or frequent changes in the chromosome number (Tsai et al., 2018). The formation of CIN is caused by the breakdown of the DNA replication fork induced by an oncogene, which leads to DSB and genomic instability (Kohlruss et al., 2021). Although CIN in cancer has been studied extensively, its exact cause remains unclear. To reveal the exact etiology of CIN in tumors, further studies are needed to investigate its mechanism and the affected pathways.
Telomerase is a reverse transcriptase that uses its own DNA as a template to synthesize telomeres to supplement the telomeres lost during the process of cell division and proliferation so that cells can continue to divide and proliferate (Yucheng and Amir, 2016). Telomerase is an indispensable factor in tumor immortalization and tumorigenesis (Hiyama et al., 1995). According to clinical research data, the telomerase activity in chronic atrophic gastritis cells caused by Helicobacter pylori infection is much higher than that in normal cells. The decreased length of telomerase is also a contributing factor to the poor prognosis of gastric cancer (Hiyama et al., 1995). A shortened telomere length will be recognized by the body as a DSB, thereby activating the DNA damage reaction pathway to protect the body, but unfortunately, this aberrant activation can cause great harm. The telomere length has important research value in studies on neurobiology and gastrointestinal microbes. Genomic instability leads to the loss of tumor suppressor genes and the improper activation of oncogenes, triggering uncontrolled cell proliferation and continued malignant cell development (Haojian et al., 2021). This mechanism of gene instability is shown in Figure 3.
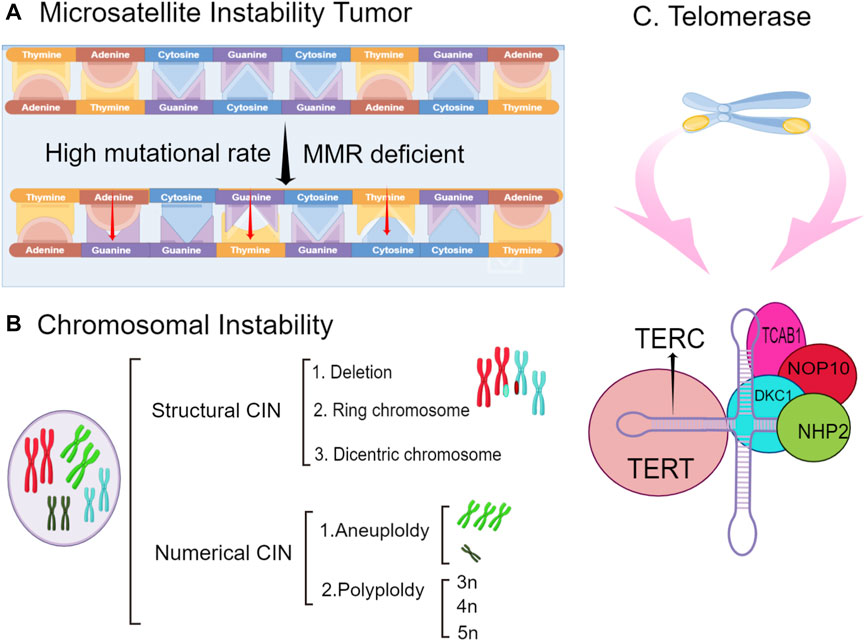
FIGURE 3. The types of genomic instability include microsatellite instability (MSI), chromosomal instability, and telomerase inactivation. (A). tumorigenesis because of MSI is caused by the abnormal regulation of the expression of target genes (B). chromosomes undergo structural or numerical changes, such as deletions and exchanges and aneuploidy and polyploidy, respectively. (C). telomerase inactivation on chromosomes can lead to genomic instability and gastric cancer. The structure of telomerase is shown.
In conclusion, we also review our previous work on the relationship between Helicobacter pylori and DNA damage. We have confirmed that transforming growth factor-β is an important mediator in the pathogenesis of Helicobacter pylori (Nianshuang et al., 2016). We have identified the molecular mechanisms underlying the interaction between the Hippo and Wnt signaling pathways and elucidated their roles in tumorigenesis of the gastrointestinal tract, especially the intestine, stomach, and liver (Li et al., 2019). We further verified that the inhibition of autophagy activates the DNA damage response and initiates gastric tumorigenesis via Rad51 ubiquitination in response to Helicobacter pylori infection (Chuan et al., 2020). In the future, we will continue to study the strong link between Helicobacter pylori and gastric cancer.
Therapeutic Approaches for Treating DNA Damage in Gastric Cancer
Considering that the individual DDR capacity of gastric cancer patients varies greatly, the diversity of DDR genes not only is a crucial genetic element but also provides a novel approach to treat gastric cancer and will become a focus and hotspot in the cancer research field. Regarding the treatment of gastric cancer, some approaches have been proposed and validated. For example, the US Food and Drug Administration (FDA) has approved some special DNA-damaging agents for the clinical treatment of gastric cancer, such as PARP inhibitors. Furthermore, certain chemotherapy and radiotherapy regimens are also effective. Below is a summary of the DNA-damaging agents and chemoradiotherapy used in the current treatment of gastric cancer.
DNA-Damaging Agents in Gastric Cancer
DNA-damaging agents induce diverse types of DNA damage, mainly DSBs and SSBs. These types of DNA damage are sensed and repaired by proteins involved in the DNA damage response. Therefore, the abnormal expression of a particular DNA damage response protein could be a biomarker of resistance or of a favorable response to therapies that induce the corresponding types of DNA damage. For instance, SLFN11 has been shown to suppress gastric cancer growth both in vitro and in vivo and to enhance the capacity of cisplatin to induce S-phrase arrest and apoptosis in gastric cancer. Therefore, the use of SLFN11 has contributed to improvement in the prognosis and survival of cancer patients (Yaojun et al., 2019).
Aurora kinase A (AURKA) is highly overexpressed in gastric cancer and inversely correlated with prognosis. AURKA restricts survivin ubiquitylation and degradation in gastric cancer to promote drug resistance, and hence, the AURKA–survivin axis can be targeted to enhance the efficacy of DNA-damaging agents in treating gastric cancer (Kamran et al., 2017). In recent years, a retrospective multicenter data analysis of gastric cancer with BRCA1 or BRCA2 germline mutations (gBRCAm) was conducted to identify 10 gastric cancer patients with gBRACm, 6 of whom had metastatic disease. The results of the analysis demonstrated that the median OS was 4.5 months for all 10 gastric cancer patients, 55.5 months for patients with operable disease, and 32 months for patients with metastatic disease. These preliminary data suggested that gBRCAm is associated with favorable outcomes in gastric cancer patients (Naama et al., 2020). However, the data samples of these cases are only 10, and further experimental research studies are needed to verify them. In contrast, a large number of DNA-damaging agents have been developed, and among them, some have partly been tested for their ability to enhance DNA damage-induced tumor cell killing in preclinical studies and clinical trials. As this technology improves, more uncertainties will be resolved in future experiments.
Different types of DNA damage trigger phosphorylation-mediated signaling cascades that lead to stimulating specific cellular responses. In the NF-KB signaling pathway, the transcription factor RelA is critical to these DNA damage response pathways. Different DNA damage agents can induce different cell outcomes through transcription factor RelA, but its specific coordinated signal transduction mechanism remains unclear (Campbell et al., 2021). The P13k/AKT signaling pathway also plays an important role in DDR. The dual inhibition of P13k/AKT signaling and DNA damage checkpoints in p53-deficient cells accelerates rapid apoptotic cell death during the G (2) period (Skladanowski et al., 2007). Maintenance of genomic integrity after DNA damage depends on the activation of the tumor suppressor P53, which then coordinates the DNA repair system/cell cycle checkpoint. The Wnt/β-catenin signaling pathway is one of the main targets of p53. Therefore, when related DNA damage agents act on the Wnt/β-catenin signaling pathway, DNA damage will occur, leading to genomic instability and thus aggravating the tumorigenesis and progression of gastric cancer (Karimaian et al., 2017). It is worth adding that DNA damage agents can also activate p53 through different upper signaling pathways, such as SAPK signaling (Shi et al., 2021). When the mechanism of the signal pathway involved in the occurrence and development of gastric cancer is clearly studied, its treatment will also become feasible.
Some related inhibitors are also being developed, such as Wee1 inhibitors, CHK1 inhibitors, and PARP inhibitors. Wee1 inhibitors are undergoing clinical trials. The Wee1 inhibitor AZD1775 has an effective checkpoint inhibitory activation effect, which can significantly inhibit the proliferation of gastric cancer cells and induce apoptosis and cell cycle arrest, especially in gastric cancer cells with high Wee1 expression (Chen et al., 2018). When combined with CHK1 inhibitors, Wee1 inhibitors can overcome the resistance of tumor cells to CHK1 and thus enhance the anticancer activity (Li et al., 2020). In the meantime, the combination of CHK1 inhibitor LY2606368 and PARP inhibitor BMN673 showed a better synergistic anticancer effect (Parmar et al., 2019). It can be seen from these studies that Wee1, CHK1, PARP, and others are all valuable targets in the treatment of gastric cancer, and the combination of different target-related inhibitors may be a more effective strategy for the treatment of gastric cancer in the future, which improves the overall treatment outcome in patients with advanced gastric cancer.
For now, PARP inhibitors have made great strides in the treatment of cancer. We also systematically analyzed the application of PARP inhibitors in gastric cancer. ADP-ribosylation is the modification of target proteins by ADP-ribosyltransferase using NAD+ and ADP-ribosyltransferase (Hopp et al., 2019), which can alter the physical and chemical properties of target proteins in many important processes (Lin and Caroll, 2018), such as DNA repair, transcription, telomere length and senescence, protein degradation, apoptosis, and necrosis (Veneris et al., 2020). The only known ADP-ribosylated proteins are the members of the PARP family. This family of proteins consists of 17 members with different domains, activities, subcellular localizations, and functions. PARP is a DNA sensor; its polymeric ADP–ribose strands act as a platform for protein signaling to coordinate the DNA repair process (D'Andrea, 2018). However, PARP also affects the degree of DNA damage. For lower degrees of DNA damage, PARP activity can be beneficial for DNA repair and cell survival, but under ischemic conditions or in the presence of severe inflammation, widespread DNA damage can occur, activating at least two different mechanisms to cause cell death, namely, cell necrosis induced by energy depletion and apoptosis-inducing factor–dependent apoptosis (Luo and Kraus, 2012). It is also worth noting that some studies had found that PARP can also affect many important biological activities in cells by changing the AMP/ATP ratio (Min and Im, 2020).
Next, we will focus on the main mechanisms by which PARP inhibitors combat tumors in cancer cells. First, we can think of PARP inhibitors as “poisons” that cause the PARP enzyme to become trapped on the DNA strand, facilitating the formation of poly(ADP-ribose) chains from NAD+ (Kim et al., 2021). Second, PARP inhibitors inhibit the repair of NHEJ, leading to cell apoptosis or necrosis after DNA damage occurs (Gupta et al., 2018). Third, some new studies have shown that PARP inhibitors reduce the repair efficiency of MMEJ, an auxiliary DNA repair mechanism (Tomasini et al., 2021). This speeds up the process of apoptosis, or death, in cancer cells that do not initiate the required DNA repair. Several PARP inhibitors have been developed. For example, olaparib is an oral PARP inhibitor used for the treatment of gastric cancer. It can both activate DDR pathways and reactivate DNA checkpoints. In addition, olaparib can be combined with many other substances to enhance the capacity of these repair pathways. A long-standing study showed that when olaparib was combined with paclitaxel, olaparib plus paclitaxel was well tolerated and led to a statistically significant improvement in OS and ATM-pts with a larger benefit in ATM-pts (Yung-Jue et al., 2013). In recent years, some scholars have pointed out that the combination of the P13K inhibitor BKM120 with olaparib can inhibit the proliferation of gastric cancer cells with ARID1A deficiency (Lin et al., 2018). After this, olaparib plus AZD1775 was found to augment the antitumor activity by disrupting DDR pathways and DNA damage checkpoints (Xiaoting et al., 2018). Another study demonstrated that olaparib combined with talaporfin photodynamic therapy can improve the efficacy by inducing the formation of PARP-DNA complexes in gastric cancer (Mamoru et al., 2021). This strategy may be potentially useful for the treatment of gastric cancer. Although olaparib and other substances have been used clinically, the pathogenesis of gastric cancer is different among different patients. Therefore, olaparib still needs further development (National Library of Medicine, 2006). In Table 2, we describe the clinical and nonclinical uses of five typical PARP inhibitor drugs. PARP inhibitors are particularly sensitive and fit with the new concept of “synthetic lethality,” in which the function of two repair pathways is synergistically lost, leading to cell death (Ashworth and Lord, 2018). Therefore, the clinical success of PARP inhibitors has brought new hope for synthetic lethal anticancer therapy, representing one of the next generations of anticancer drugs targeting DDR. In essence, PARP inhibitors prevent the repair of single-stranded DNA breaks, leading to cell apoptosis.
In the case of circumscribed gastric cancer, surgical resection is the most suitable treatment strategy (Riquelme et al., 2015). However, most gastric cancers are diagnosed as advanced and incurable when they are discovered, which limits traditional treatments and makes the development of effective anti-DDR therapeutic strategies so promising. Anti-DDR therapeutic strategies have obvious advantages over other therapeutic strategies. A recent comparative clinical study showed that the experimental group receiving anti-DDR therapeutic strategies had longer OS, reduced tumor response rate and serious adverse reactions, and improved prognosis and quality of life (Reddavid et al., 2021). Anti-DDR therapeutic strategies are the most advanced and can achieve precise effects similar to the “guiding rocket,” which directly acts on the cancerous parts and tissues, causing less damage to normal cells and resulting in better therapeutic effects (Patel and Cecchini, 2020). The development of anti-DDR therapeutic strategy-related pathways and protein inhibitors will help humans to achieve personalized treatment for patients with advanced gastric cancer.
Efficient Management of Gastric Cancer via Chemoradiotherapy
As the standard treatment for cancer, chemoradiotherapy has been extensively applied to clinical practice worldwide; however, its efficacy in the eradication of cancer cells, suppression of metastasis, and improvement of the OS of patients is poor. The mechanism of chemoradiotherapy primarily induces DNA damage to kill cancer cells; thereby, the efficacy of chemoradiotherapy depends on the generation of DNA damage. After long-term exposure to endogenous and exogenous DNA damage, the body may evolve a DNA damage response mechanism. It is normally used to sense and repair DNA damage. If the damaged lesion is completely repaired, the cell will survive; otherwise, the cells will die.
Compared with open gastrectomy, laparoscopic surgery in patients with advanced gastric cancer following neoadjuvant chemotherapy causes fewer complications and leads to a better prognosis, faster recovery of intestinal function, and prolonged survival (Liao et al., 2021). Selected gastric cancer patients with limited regional lymph node recurrence may benefit from radiotherapy combined with chemotherapy and high-dose radiotherapy (≥54 Gy) leads to a better progression-free survival and tends to extend the OS (Liang et al., 2020). A recent analysis of related research data demonstrated that tumor bleeding could be adequately controlled by palliative radiotherapy in patients with unresectable advanced gastric cancer (Jesang et al., 2021). Receptor tyrosine kinase MET overexpression is frequently observed in a range of different cancers, is associated with poor prognosis, and has been investigated in several clinical trials (Georgina et al., 2021). Olaparib can be used as an adjunct to chemotherapy to more effectively treat gastric cancer. It activates DDR and DNA checkpoint pathways to achieve a better treatment effect in gastric cancer. Some adjuvants in combination with radiotherapy may have adequate benefits in other areas as well (Marie et al., 2017). Pembrolizumab synergizes with radiotherapy, resulting in significantly improved outcomes in patients with nonsmall-cell lung cancer (NSCLC). Data from the KEYNOTE-189 phase III trial confirm that adding pembrolizumab to first-line chemotherapy improves the outcomes of patients with metastatic NSCLC, improving survival by several months (Sidaway, 2018).
Although chemotherapy and radiotherapy have not worked as well as we would like, more trials are needed to yield further innovations in cancer treatment.
Conclusion and Perspectives
Abundant data have proven that DDR pathways play a crucial role in the tumorigenesis, progression, treatment, prognosis, and other aspects of breast cancer. Hence, for the treatment of gastric cancer, precise DDR mechanisms in cells should be clarified with further experimentation. In this article, we reviewed latent DDR mechanisms and the DDR in gastric cancer associated with Helicobacter pylori infection, which represent novel therapeutic targets. In general, the road ahead is long, and further research will be indispensable. Further studies on DDR mechanisms will aid in providing a more comprehensive understanding of the etiology of gastric cancer (and other tumors) and will provide evidence regarding the best therapies for individual patients. Moreover, it is expected that the incidence and mortality of gastric cancer will sharply decrease with the efforts of scientific researchers.
Author Contributions
MW contributed to the draft of this paper. CX contributed to the revision of this paper.
Funding
This work was supported by the National Natural Science Foundation of China (82100599 and 81960112), the Jiangxi Provincial Department of Science and Technology (20212ACB216003), and The Young Talents Project of Jiangxi Provincial Academic and Technical Leaders Training Program for Major Disciplines (20204BCJ23022).
Conflict of Interest
The authors declare that the research was conducted in the absence of any commercial or financial relationships that could be construed as a potential conflict of interest.
Publisher’s Note
All claims expressed in this article are solely those of the authors and do not necessarily represent those of their affiliated organizations, or those of the publisher, the editors, and the reviewers. Any product that may be evaluated in this article, or claim that may be made by its manufacturer, is not guaranteed or endorsed by the publisher.
References
Asatryan, A. D., and Komarova, N. L. (2016). Evolution of Genetic Instability in Heterogeneous Tumors. J. Theor. Biol. 396, 1–12. doi:10.1016/j.jtbi.2015.11.028
Ashworth, A., and Lord, C. J. (2018). Synthetic Lethal Therapies for Cancer: What's Next after PARP Inhibitors? Nat. Rev. Clin. Oncol. 15 (9), 564–576. doi:10.1038/s41571-018-0055-6
Brooks, T., Wayne, J., and Massey, A. J. (2021). Chk1 Inhibition Induces a DNA Damage Bystander Effect in Cocultured Tumour Cells. DNA Repair 101, 103099. doi:10.1016/j.dnarep.2021.103099
Bukhari, A. B., Chan, G. K., and Gamper, A. M. (2022). Targeting the DNA Damage Response for Cancer Therapy by Inhibiting the Kinase Wee1. Front. Oncol. 12, 828684. doi:10.3389/fonc.2022.828684
Bushra, N., Showkat, A. K., Nuzhat Shaheen, K., Idrees, A. S., Misbah, M., Falaque Ul, A., et al. (2018). DNA Repair Gene XRCC1 and XPD Polymorphisms and Gastric Cancer Risk: A Case-Control Study Outcome from Kashmir, India. Anal. Cell. Pathol. 2018, 3806514. doi:10.1155/2018/3806514
Campbell, A. E., Ferraz Franco, C., Su, L-I., Corbin, E. K., Perkins, S., Kalyuzhnyy, A., et al. (2021). Temporal Modulation of the NF-Κb RelA Network in Response to Different Types of DNA Damage. Biochem. J. 478 (3), 533–551. doi:10.1042/bcj20200627
Chattopadhyay, R., Bhattacharyya, A., and Crowe, S. E. (2010). Dual Regulation by Apurinic/apyrimidinic Endonuclease-1 Inhibits Gastric Epithelial Cell Apoptosis during Helicobacter pylori Infection. Cancer Res. 70 (7), 2799–2808. doi:10.1158/0008-5472.CAN-09-4136
Chen, D., Lin, X., Gao, J., Shen, L., Li, Z., Dong, B., et al. (2018). Wee1 Inhibitor AZD1775 Combined With Cisplatin Potentiates Anticancer Activity against Gastric Cancer by Increasing DNA Damage and Cell Apoptosis. Biomed Res. Int., 5813292. doi:10.1080/19490976.2020.1774311
Chuan, X., Nianshuang, L., Huan, W., Cong, H., Yi, H., Chao, P., et al. (2020). Inhibition of Autophagy Aggravates DNA Damage Response and Gastric Tumorigenesis via Rad51 Ubiquitination in Response to H. pylori Infection. Gut Microbes 11 (6), 1567–1589. doi:10.1080/19490976.2020.1774311
D'Andrea, A. D. (2018). Mechanisms of PARP Inhibitor Sensitivity and Resistance. DNA Repair (Amst) 71, 172–176. doi:10.1016/j.dnarep.2018.08.021
Dong, Z., Huaping, C., Cedric, M., Sadia, A., Buck, E. R., Joel, R. G., et al. (2021). Radiosynthesis and Evaluation of Talazoparib and its Derivatives as PARP-1-Targeting Agents. Biomedicines 9 (5), 565. doi:10.3390/biomedicines9050565
Efi, F., Nick, G., Nikolaos, A., John, G., Nektarios, A., Nikolaos, K., et al. (2020). Replication Protein A (RPA1, RPA2 and RPA3) Expression in Gastric Cancer: Correlation with Clinicopathologic Parameters and Patients' Survival. J. BUON official J. Balkan Union Oncol. 25 (3), 1482–1489.
Ferlay, J., Soerjomataram, I., Dikshit, R., Eser, S., Mathers, C., Rebelo, M., et al. (2014). Cancer Incidence and Mortality Worldwide: Sources, Methods and Major Patterns in GLOBOCAN 2012. Int. J. Cancer 136 (5), E359–E386. doi:10.1002/ijc.29210
Fu-Rong, W., Yu-Cai, W., Zhi-Jian, H., Wen-Ting, H., Xiao-Ying, G., Hao, C., et al. (2017). Aberrant DNA-PKcs and ERGIC1 Expression May Be Involved in Initiation of Gastric Cancer. World J. Gastroenterology 23 (33), 6119–6127. doi:10.3748/wjg.v23.i33.6119
Futagami, S., Hiratsuka, T., Shindo, T., Horie, A., Hamamoto, T., Suzuki, K., et al. (2008). Expression of Apurinic/apyrimidinic Endonuclease-1 (APE-1) in H. Pylori-Associated Gastritis, Gastric Adenoma, and Gastric Cancer. Helicobacter 13 (3), 209–218. doi:10.1111/j.1523-5378.2008.00605.x
Georgina, E. W., Helen, H., Danielle, M. H., and Stéphanie, K. (2021). The Role of MET in Chemotherapy Resistance. Oncogene 40 (11), 1927–1941. doi:10.1038/s41388-020-01577-5
Gupta, R., Somyajit, K., Narita, T., Maskey, E., Stanlie, A., Kremer, M., et al. (2018). DNA Repair Network Analysis Reveals Shieldin as a Key Regulator of NHEJ and PARP Inhibitor Sensitivity. Cell 173 (4), 972–988. doi:10.1016/j.cell.2018.03.050
Hall, M. J., Bernhisel, R., Hughes, E., Larson, K., Rosenthal, E. T., Singh, N. A., et al. (2021). Germline Pathogenic Variants in the Ataxia Telangiectasia Mutated (ATM) Gene Are Associated with High and Moderate Risks for Multiple Cancers. Cancer Prev. Res. 14 (4), 433–440. doi:10.1158/1940-6207.CAPR-20-0448
Han, L., Shu, X., and Wang, J. (2022). Helicobacter Pylori-Mediated Oxidative Stress and Gastric Diseases: A Review. Front. Microbiol. 13, 811258. doi:10.3389/fmicb.2022.811258
Hannes, H., Thorunn, R., Halla, S. O., Jon, G. J., Asgeir, S., Simon, N. S., et al. (2015). Loss-of-function Variants in ATM Confer Risk of Gastric Cancer. Nat. Genet. 47 (8), 906–910. doi:10.1038/ng.3342
Haojian, L., Susan, E. Z., and Urbain, W. (2021). Genomic Instability and Metabolism in Cancer. Int. Rev. Cell Mol. Biol. 364, 241–265. doi:10.1016/bs.ircmb.2021.05.004
Hee Sung, K., Gyu, H., Hye Young, M., Yung-Jue, B., and Woo Ho, K. (2019). Clinical Significance of BRCA1 and BRCA2 mRNA and Protein Expression in Patients with Sporadic Gastric Cancer. Oncol. Lett. 17 (5), 4383–4392. doi:10.3892/ol.2019.10132
Helicobacter pylori Infection (2022). Viral Evolution and the Future of Monoclonal Antibodies. N. Engl. J. Med. 386 (24), e75. doi:10.1056/NEJMe2207933
Hiyama, E., Yokoyama, T., Tatsumoto, N., Hiyama, K., Imamura, Y., Murakami, Y., et al. (1995). Telomerase Activity in Gastric Cancer. Cancer Res. 55 (15), 3258–3262.
Hoeijmakers, J. H. J. (2001). Genome Maintenance Mechanisms for Preventing Cancer. Nature 411 (6835), 366–374. doi:10.1038/35077232
Hongli, L., Shaohua, G., and Yi, B. (2017). Nutritional Status and Quality of Life in Patients with Gastric Cancer in China. J. Clin. Oncol. 35, e15508. doi:10.1200/jco.2017.35.15_suppl.e15508
Hopp, A. K., Grüter, P., and Hottiger, M. O. (2019). Erratum: Hopp, A.K., et al. Regulation of Glucose Metabolism by NAD+ and ADP-Ribosylation. Cells 2019, 8, 890. Cells 8 (8), 1371. doi:10.3390/cells8111371
Huang, R.-X., and Zhou, P.-K. (2020). DNA Damage Response Signaling Pathways and Targets for Radiotherapy Sensitization in Cancer. Sig Transduct. Target Ther. 5 (1), 60. doi:10.1038/s41392-020-0150-x
Huiying, S., Rui, Z., Yannan, Z., Zhaowei, W., Dingling, Z., Dongqiang, Z., et al. (2021). CRIP1 Cooperates with BRCA2 to Drive the Nuclear Enrichment of RAD51 and to Facilitate Homologous Repair upon DNA Damage Induced by Chemotherapy. Oncogene 40 (34), 5342–5355. doi:10.1038/s41388-021-01932-0
Hyuna, S., Jacques, F., Rebecca, L. S., Mathieu, L., Isabelle, S., Ahmedin, J., et al. (2021). Global Cancer Statistics 2020: GLOBOCAN Estimates of Incidence and Mortality Worldwide for 36 Cancers in 185 Countries. CA: A Cancer Journal for Clinicians.
Jackson, S. P., and Bartek, J. (2009). The DNA-Damage Response in Human Biology and Disease. Nature 461 (7267), 1071–1078. doi:10.1038/nature08467
Jesang, Y., Jinhong, J., Sook Ryun, P., Min-Hee, R., Jin-hong, P., Jong Hoon, K., et al. (2021). Role of Palliative Radiotherapy in Bleeding Control in Patients with Unresectable Advanced Gastric Cancer. BMC Cancer 21 (1), 413. doi:10.1186/s12885-021-08145-4
Jiang, Z-H., Peng, T., Qian, H-L., Lu, C-d., Qiu, F., and Zhang, S-Z. (2019). DNA Damage-Induced Activation of ATM Promotes β-TRCP-mediated ARID1A Ubiquitination and Destruction in Gastric Cancer Cells. Cancer Cell Int. 19, 162. doi:10.1186/s12935-019-0878-y
Jin Won, K., Seock-Ah, I., Min, A. K., Hyun Jin, C., Dae Won, L., Kyung-Hun, L., et al. (2013). Ataxia-telangiectasia-mutated Protein Expression with Microsatellite Instability in Gastric Cancer as Prognostic Marker. Int. J. Cancer 134 (1), 72–80. doi:10.1002/ijc.28245
Joel Del Bel, P., Carolline Fontes Alves, M., Alexandre Todorovic, F., Daniela Pretti da Cunha, T., Ajith Kumar, S., José Sebastião dos, S., et al. (2022). Prognostic Value of the Immunohistochemical Expression of RAD51 and BRCA2 in Gastric Adenocarcinoma. J. Histochem. Cytochem. 70 (3), 199–210. doi:10.1369/00221554211065834
Kamran, M., Long, Z. J., Xu, D., Lv, S. S., Liu, B., Wang, C. L., et al. (2017). Aurora Kinase A Regulates Survivin Stability through Targeting FBXL7 in Gastric Cancer Drug Resistance and Prognosis. Oncogenesis 6 (2), e298. doi:10.1038/oncsis.2016.80
Karimaian, A., Majidinia, M., Bannazadeh Baghi, H., and Yousefi, B. (2017). The Crosstalk between Wnt/β-Catenin Signaling Pathway with DNA Damage Response and Oxidative Stress: Implications in Cancer Therapy. DNA Repair 51, 14–19. doi:10.1016/j.dnarep.2017.01.003
Kathleen, N. M., Angeles Alvarez, S., Melissa, A. G., David Scott, M., Noelle, C., Gini, F. F., et al. (2019). Niraparib Monotherapy for Late-Line Treatment of Ovarian Cancer (QUADRA): a Multicentre, Open-Label, Single-Arm, Phase 2 Trial. Lancet Oncol. 20 (5), 636–648. doi:10.1016/S1470-2045(19)30029-4
Kciuk, M., Gielecińska, A., Mujwar, S., Mojzych, M., and Kontek, R. (2022). Cyclin-dependent Kinases in DNA Damage Response. Biochimica Biophysica Acta (BBA) - Rev. Cancer 1877 (3), 188716. doi:10.1016/j.bbcan.2022.188716
Kim, D.-S., Camacho, C. V., and Kraus, W. L. (2021). Alternate Therapeutic Pathways for PARP Inhibitors and Potential Mechanisms of Resistance. Exp. Mol. Med. 53 (1), 42–51. doi:10.1038/s12276-021-00557-3
Kim, H. S., Kim, J. W., Hwang, I. G., Lee, H. S., and Kim, W. H. (2019). Expression of DNA Damage Response Markers in Early-Onset or Familial Gastric Cancers. Asian Pac J. Cancer Prev. 20 (5), 1369–1376. doi:10.31557/apjcp.2019.20.5.1369
Kim, H. S., Kim, J. W., Hwang, I. G., Lee, H. S., and Kim, W. H. (2019). Expression of DNA Damage Response Markers in Early-Onset or Familial Gastric Cancers. Asian Pac J. Cancer Prev. 20 (5), 1369–1376. doi:10.31557/APJCP.2019.20.5.1369
Kohlruss, M., Krenauer, M., Grosser, B., Pfarr, N., Jesinghaus, M., Slotta-Huspenina, J., et al. (2021). Diverse 'just-Right' Levels of Chromosomal Instability and Their Clinical Implications in Neoadjuvant Treated Gastric Cancer. Br. J. Cancer 125 (12), 1621–1631. doi:10.1038/s41416-021-01587-4
Koustas, E., Karamouzis, M. V., Sarantis, P., Schizas, D., and Papavassiliou, A. G. (2020). Inhibition of c-MET Increases the Antitumour Activity of PARP Inhibitors in Gastric Cancer Models. J. Cell Mol. Med. 24 (18), 10420–10431. doi:10.1111/jcmm.15655
Lawrence, H. K., Colleen, D., Marji, M., Cheryl, L. R., Wendy, D-W., Elisa, V. B., et al. (2012). American Cancer Society Guidelines on Nutrition and Physical Activity for Cancer Prevention. CA: A Cancer Journal for Clinicians.
Lei, T., Du, S., Peng, Z., and Chen, L. (2022). Multifaceted Regulation and Functions of 53BP1 in NHEJ-Mediated DSB Repair (Review). Int. J. Mol. Med.
Li, F., Kozono, D., Deraska, P., Branigan, T., Dunn, C., Zheng, X.-F., et al. (2020). CHK1 Inhibitor Blocks Phosphorylation of FAM122A and Promotes Replication Stress. Mol. Cell 80 (3), 410–422. doi:10.1016/j.molcel.2020.10.008
Li, N., Lu, N., and Xie, C. (2019). The Hippo and Wnt Signalling Pathways: Crosstalk during Neoplastic Progression in Gastrointestinal Tissue. Febs J. 286 (19), 3745–3756. doi:10.1111/febs.15017
Liang, C., Ganlu, O., Xin, W., Zhiping, L., and Yali, S. (2020). Radiotherapy Combined with Chemotherapy for Regional Lymph Node Recurrence in Gastric Cancer. Cancer Manag. Res. 12, 13339–13346. doi:10.2147/CMAR.S280225
Liao, X.-L., Liang, X.-W., Pang, H.-Y., Yang, K., Chen, X.-Z., Chen, X.-L., et al. (2021). Safety and Efficacy of Laparoscopic versus Open Gastrectomy in Patients with Advanced Gastric Cancer Following Neoadjuvant Chemotherapy: A Meta-Analysis. Front. Oncol. 11, 704244. doi:10.3389/fonc.2021.704244
Lifeng, T., Lucie, P., Linghua, W., Zhaohui, Y., Linzhao, C., David, A. W., et al. (2012). Whole Genome Sequencing of Four CD34+-Derived iPSC Polycythemia Vera Clones from a Single Female. Blood 120 (21), 1755. doi:10.1182/blood.V120.21.1755.1755
Lin, H., and Caroll, K. S. (2018). Introduction: Posttranslational Protein Modification. Chem. Rev. 118 (3), 887–888. doi:10.1021/acs.chemrev.7b00756
Lin, Y., Guanghai, Y., Yingjun, D., Yu, H., Shunfang, L., Lei, Z., et al. (2018). Combined Treatment with PI3K Inhibitor BKM120 and PARP Inhibitor Olaparib Is Effective in Inhibiting the Gastric Cancer Cells with ARID1A Deficiency. Oncol. Rep. 40 (1), 479–487. doi:10.3892/or.2018.6445
Luo, X., and Kraus, W. L. (2012). On PAR with PARP: Cellular Stress Signaling through poly(ADP-Ribose) and PARP-1. Genes Dev. 26 (5), 417–432. doi:10.1101/gad.183509.111
Maleki, S. S., and Röcken, C. (2017). Chromosomal Instability in Gastric Cancer Biology. Neoplasia 19 (5), 412–420. doi:10.1016/j.neo.2017.02.012
Mamoru, T., Makiko, S., Taketo, S., Hirotada, N., and Hiromi, K. (2021). Combination of Talaporfin Photodynamic Therapy and Poly (ADP-Ribose) Polymerase (PARP) Inhibitor in Gastric Cancer. Biochem. Biophysical Res. Commun. 539, 1–7. doi:10.1016/j.bbrc.2020.12.073
Marie, R., Jean-Sébastien, F., Carole, G., Anne, P., Paule, A., and Mario, C. (2017). Olaparib for the Treatment of Breast Cancer. Expert Opin. Investigational Drugs 18 (6), 519–530. doi:10.1080/14737140.2018.1458613
Mauri, G., Arena, S., Siena, S., Bardelli, A., and Sartore-Bianchi, A. (2020). The DNA Damage Response Pathway as a Land of Therapeutic Opportunities for Colorectal Cancer. Ann. Oncol. 31 (9), 1135–1147. doi:10.1016/j.annonc.2020.05.027
Min, A., Im, S-A., Jang, H., Kim, S., Lee, M., Kim, D. K., et al. (2017). AZD6738, A Novel Oral Inhibitor of ATR, Induces Synthetic Lethality with ATM Deficiency in Gastric Cancer Cells. Mol. Cancer Ther. 16 (4), 566–577. doi:10.1158/1535-7163.MCT-16-0378
Min, A., and Im, S. A. (2020). PARP Inhibitors as Therapeutics: Beyond Modulation of PARylation. Cancers (Basel) 12 (2), 394. doi:10.3390/cancers12020394
Naama, H., Albert, G., Ben, B., Talia, G., Ofer, M., Dan, A., et al. (2020). Clinical Characteristics and Prognosis of Gastric Cancer Patients with BRCA 1/2 Germline Mutations: Report of Ten Cases and a Literature Review. OncoTargets Ther. 13, 11637–11644. doi:10.2147/OTT.S276814
National Library of Medicine (2006). Olaparib. Drugs and Lactation Database (LactMed). Bethesda (MD): National Library of Medicine.
Nianshuang, L., Chuan, X., and Nong-Hua, L. (2016). p53, a Potential Predictor of Helicobacter pylori Infection-Associated Gastric Carcinogenesis? Oncotarget 7 (40), 66276–66286. doi:10.18632/oncotarget.11414
Parmar, K., Kochupurakkal, B. S., Lazaro, J-B., Wang, Z. C., Palakurthi, S., Kirschmeier, P. T., et al. (2019). The CHK1 Inhibitor Prexasertib Exhibits Monotherapy Activity in High-Grade Serous Ovarian Cancer Models and Sensitizes to PARP Inhibition. Clin. Cancer Res. 25 (20), 6127–6140. doi:10.1158/1078-0432.ccr-19-0448
Patel, T. H., and Cecchini, M. (2020). Targeted Therapies in Advanced Gastric Cancer. Curr. Treat. Options Oncol. 21 (9), 70. doi:10.1007/s11864-020-00774-4
Pearl, L. H., Schierz, A. C., Ward, S. E., Al-Lazikani, B., and Pearl, F. M. G. (2015). Therapeutic Opportunities within the DNA Damage Response. Nat. Rev. Cancer 15 (3), 166–180. doi:10.1038/nrc3891
Polom, K., Marrelli, D., Smyth, E. C., Voglino, C., Roviello, G., Pascale, V., et al. (2018). The Role of Microsatellite Instability in Positive Margin Gastric Cancer Patients. Surg. Innov. 25 (2), 99–104. doi:10.1177/1553350617751461
Polom, K., Marrelli, D., Voglino, C., Roviello, G., De Franco, L., Vindigni, C., et al. (2018). Familial Aggregation of Gastric Cancer with Microsatellite Instability*. Acta Chir. Belg. 118 (5), 287–293. doi:10.1080/00015458.2017.1379789
Praud, D., Rota, M., Pelucchi, C., Bertuccio, P., Rosso, T., Galeone, C., et al. (2018). Cigarette Smoking and Gastric Cancer in the Stomach Cancer Pooling (StoP) Project. Eur. J. Cancer Prev. 27 (2), 124–133. doi:10.1097/CEJ.0000000000000290
Rahman, S., Canny, M. D., Buschmann, T. A., and Latham, M. P. (2020). A Survey of Reported Disease-Related Mutations in the MRE11-RAD50-NBS1 Complex. Cells 9 (7), 1678. doi:10.3390/cells9071678
Reddavid, R., Dagatti, S., Franco, C., Puca, L., Tomatis, M., Corso, S., et al. (2021). Molecularly Targeted Therapies for Gastric Cancer. State of the Art. Cancers (Basel) 13 (16), 4094. doi:10.3390/cancers13164094
Reyes, V. E., and Peniche, A. G. (2019). Helicobacter pylori Deregulates T and B Cell Signaling to Trigger Immune Evasion. Curr. Top. Microbiol. Immunol. 421, 229–265. doi:10.1007/978-3-030-15138-6_10
Riquelme, I., Saavedra, K., Espinoza, J. A., Weber, H., García, P., Nervi, B., et al. (2015). Molecular Classification of Gastric Cancer: Towards a Pathway-Driven Targeted Therapy. Oncotarget 6 (28), 24750–24779. doi:10.18632/oncotarget.4990
Samantha, H., Pardeep, K., Hector, L-C., Michelle, W., Janine, S., Hugo, P., et al. (2015). Hereditary Diffuse Gastric Cancer Syndrome: CDH1 Mutations and beyond. JAMA Oncol. 1 (1), 23–32. doi:10.1001/jamaoncol.2014.168
Sheridan, MH. Talazoparib: First Global Approval. Drugs. (2018).78(18):1939-1946.doi:10.1007/s40265-018-1026-z
Shi, T., van Soest, D. M. K., Polderman, P. E., Burgering, B. M. T., and Dansen, T. B. (2021). DNA Damage and Oxidant Stress Activate P53 through Differential Upstream Signaling Pathways. Free Radic. Biol. Med. 172, 298–311. doi:10.1016/j.freeradbiomed.2021.06.013
Sidaway, P. (2018). Pembrolizumab Synergizes with Chemotherapy. Nat. Rev. Clin. Oncol. 15 (7), 402–403. doi:10.1038/s41571-018-0031-1
Silva-Fernandes, I. J. d. L., Oliveira, E. S. d., Santos, J. C., Ribeiro, M. L., Ferrasi, A. C., Pardini, M. I. d. M. C., et al. (2017). The Intricate Interplay between MSI and Polymorphisms of DNA Repair Enzymes in Gastric Cancer H.Pylori Associated. Mutagenesis 32 (4), 471–478. doi:10.1093/mutage/gex013
Skladanowski, A., Bozko, P., Sabisz, M., and Larsen, A. K. (2007). Dual Inhibition of PI3K/Akt Signaling and the DNA Damage Checkpoint in P53-Deficient Cells with Strong Survival Signaling: Implications for Cancer Therapy. Cell Cycle 6 (18), 2268–2275. doi:10.4161/cc.6.18.4705
Sokolova, O., and Naumann, M. (2019). Crosstalk between DNA Damage and Inflammation in the Multiple Steps of Gastric Carcinogenesis. Curr. Top. Microbiol. Immunol. 421, 107–137. doi:10.1007/978-3-030-15138-6_5
Sokolova, O., and Naumann, M. (2019). Crosstalk between DNA Damage and Inflammation in the Multiple Steps of Gastric Carcinogenesis. Curr. Top. Microbiol. Immunol. 421, 107–137. doi:10.1007/978-3-030-15138-6_5
Su, S., Chhabra, G., Singh, C. K., Ndiaye, M. A., and Ahmad, N. (2022). PLK1 Inhibition-Based Combination Therapies for Cancer Management. Transl. Oncol. 16, 101332. doi:10.1016/j.tranon.2021.101332
Sylvia, B., Stephanie, B., and Uwe, M. M. (2018). Olaparib. Recent results cancer Res. Fortschritte der Krebsforschung Progres dans les recherches sur le cancer 211, 217–233. doi:10.1007/978-3-319-91442-8_15
Tian, H., Gao, Z., Li, H., Zhang, B., Wang, G., Zhang, Q., et al. (2015). DNA Damage Response - A Double-Edged Sword in Cancer Prevention and Cancer Therapy. Cancer Lett. 358 (1), 8–16. doi:10.1016/j.canlet.2014.12.038
Tomasini, P. P., Guecheva, T. N., Leguisamo, N. M., Péricart, S., Brunac, A. C., Hoffmann, J. S., et al. (2021). Analyzing the Opportunities to Target DNA Double-Strand Breaks Repair and Replicative Stress Responses to Improve Therapeutic Index of Colorectal Cancer. Cancers (Basel) 13 (13), 3130. doi:10.3390/cancers13133130
Tsai, C.-K., Yeh, T.-S., Wu, R.-C., Lai, Y.-C., Chiang, M.-H., Lu, K.-Y., et al. (2018). Metabolomic Alterations and Chromosomal Instability Status in Gastric Cancer. Wjg 24 (33), 3760–3769. doi:10.3748/wjg.v24.i33.3760
Veneris, J. T., Matulonis, U. A., Liu, J. F., and Konstantinopoulos, P. A. (2020). Choosing Wisely: Selecting PARP Inhibitor Combinations to Promote Anti-tumor Immune Responses beyond BRCA Mutations. Gynecol. Oncol. 156 (2), 488–497. doi:10.1016/j.ygyno.2019.09.021
Vinod Vijay, S., Shi Hui, T., Mei Shi, Y., Fui Leng, Y., Praveen, C. P., Natalia, L., et al. (2016). ATM Expression Predicts Veliparib and Irinotecan Sensitivity in Gastric Cancer by Mediating P53-independent Regulation of Cell Cycle and Apoptosis. Mol. Cancer Ther. 15 (12), 3087–3096. doi:10.1158/1535-7163.MCT-15-1002
Wang, G.-H., Zhao, C.-M., Huang, Y., Wang, W., Zhang, S., and Wang, X. (2018). BRCA1 and BRCA2 Expression Patterns and Prognostic Significance in Digestive System Cancers. Hum. Pathol. 71, 135–144. doi:10.1016/j.humpath.2017.10.032
Wang, S., Wu, X., Chen, Y., Zhang, J., Ding, J., Zhou, Y., et al. (2012). Prognostic and Predictive Role of JWA and XRCC1 Expressions in Gastric Cancer. Clin. Cancer Res. 18 (10), 2987–2996. doi:10.1158/1078-0432.ccr-11-2863
Wei, L., Chuan, X., Zhen, Y., Jiang, C., and Nong-Hua, L. (2013). Abnormal DNA-PKcs and Ku 70/80 Expression May Promote Malignant Pathological Processes in Gastric Carcinoma. World J. Gastroenterology 19 (40), 6894–6901. doi:10.3748/wjg.v19.i40.6894
Xiaoting, L., Dongshao, C., Cheng, Z., Xiaotian, Z., Zhongwu, L., Bin, D., et al. (2018). Augmented Antitumor Activity by Olaparib Plus AZD1775 in Gastric Cancer through Disrupting DNA Damage Repair Pathways and DNA Damage Checkpoint. J. Exp. Clin. Cancer Res. 37 (1), 129. doi:10.1186/s13046-018-0790-7
Xu, X-B., Li, N-S., Wang, H., Hu, Y., Wu, X-D., Hong, J-B., et al. (2021). Expression and Prognostic Significance of the DNA Damage Response Pathway and Autophagy Markers in Gastric Cancer. Neoplasma 68 (6), 1310–1319. doi:10.4149/neo_2021_210515N66710.4149/neo_2021_210515n667
Yahiya, Y. S. (2017). Rucaparib: First Global Approval. Drugs 77 (5), 585–592. doi:10.1007/s40265-017-0716-2
Yali, T., Yuxian, M., Rongbiao, Y., Shasha, C., and Zhiping, W. (2020). The ATM Rs189037 G>A Polymorphism Is Associated with the Risk and Prognosis of Gastric Cancer in Chinese Individuals: A Case-Control Study. Gene 741, 144578. doi:10.1016/j.gene.2020.144578
Yaojun, P., Li, W., Liangliang, W., Ling, Z., Guangjun, N., and Mingzhou, G. (2019). Methylation of SLFN11 Promotes Gastric Cancer Growth and Increases Gastric Cancer Cell Resistance to Cisplatin. J. Cancer 10 (24), 6124–6134. doi:10.7150/jca.32511
Young, K., Starling, N., and Cunningham, D. (2016). Targeting Deficient DNA Damage Repair in Gastric Cancer. Expert Opin. Pharmacother. 17 (13), 1757–1766. doi:10.1080/14656566.2016.1217992
Yucheng, X., and Amir, G. (2016). Telomere and Telomerase Therapeutics in Cancer. Genes 7 (6), 22. doi:10.3390/genes7060022
Yujie, Z., and Chaoran, Y. (2020). Distinct Expression and Prognostic Values of the Replication Protein A Family in Gastric Cancer. Oncol. Lett. 19 (3), 1831–1841. doi:10.3892/ol.2020.11253
Yung-Jue, B., Seock-Ah, I., Keun-Wook, L., Jae Yong, C., Eun-Kee, S., Kyung Hee, L., et al. (2013). Olaparib Plus Paclitaxel in Patients with Recurrent or Metastatic Gastric Cancer: A Randomized, Double-Blind Phase II Study. J. Clin. Oncol. 31, 4013. doi:10.1200/jco.2013.31.15_suppl.4013
Zehang, J., Zhixian, L., Mengyuan, L., Cai, C., and Xiaosheng, W. (2018). Immunogenomics Analysis Reveals that TP53 Mutations Inhibit Tumor Immunity in Gastric Cancer. Transl. Oncol. 11 (5), 1171–1187. doi:10.1016/j.tranon.2018.07.012
Zhang, F-X., Miao, Y., Ruan, J-G., Meng, S-P., Dong, J-D., Yin, H., et al. (2019). Association between Nitrite and Nitrate Intake and Risk of Gastric Cancer: A Systematic Review and Meta-Analysis. Med. Sci. Monit. Int. Med. J. Exp. Clin. Res. 25, 1788–1799. doi:10.12659/msm.914621
Zhou, J., Zhao, Z., Zhang, Y., Bao, C., Cui, L., Cai, S., et al. (2020). Pathogenic Germline Mutations in Chinese Patients with Gastric Cancer Identified by Next-Generation Sequencing. Oncology 98 (8), 583–588. doi:10.1159/000505664
Keywords: DNA damage repair, Helicobacter pylori, therapeutic approaches, gastric cancer, PARP inhibitors
Citation: Wang M and Xie C (2022) DNA Damage Repair and Current Therapeutic Approaches in Gastric Cancer: A Comprehensive Review. Front. Genet. 13:931866. doi: 10.3389/fgene.2022.931866
Received: 29 April 2022; Accepted: 15 June 2022;
Published: 12 August 2022.
Edited by:
Domenico Maiorano, Centre National de la Recherche Scientifique (CNRS), FranceReviewed by:
Mayurika Lahiri, Indian Institute of Science Education and Research, IndiaFeng Li, Dana–Farber Cancer Institute, United States
Copyright © 2022 Wang and Xie. This is an open-access article distributed under the terms of the Creative Commons Attribution License (CC BY). The use, distribution or reproduction in other forums is permitted, provided the original author(s) and the copyright owner(s) are credited and that the original publication in this journal is cited, in accordance with accepted academic practice. No use, distribution or reproduction is permitted which does not comply with these terms.
*Correspondence: Chuan Xie, xcsghhz@ncu.edu.cn