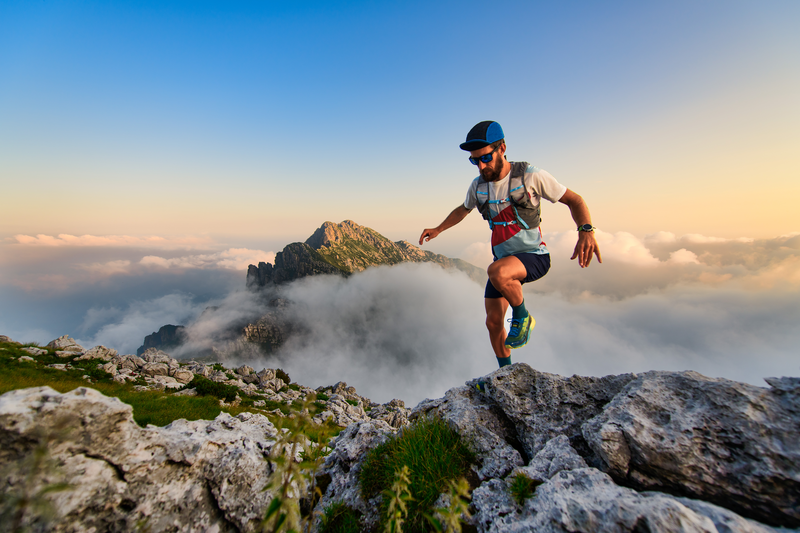
95% of researchers rate our articles as excellent or good
Learn more about the work of our research integrity team to safeguard the quality of each article we publish.
Find out more
ORIGINAL RESEARCH article
Front. Genet. , 08 June 2022
Sec. Genomics of Plants and the Phytoecosystem
Volume 13 - 2022 | https://doi.org/10.3389/fgene.2022.921096
This article is part of the Research Topic Breaking the Myth: Breeding for Stress Tolerance, Grain Yield, and Quality Traits Simultaneously by Diversifying the Narrow Genetic Base View all 42 articles
Trichomes protect plants against insects, microbes, herbivores, and abiotic damages and assist seed dispersal. The function of CPR5 genes have been found to be involved in the trichome development but the research on the underlying genetic and molecular mechanisms are extremely limited. Herein, genome wide identification and characterization of CPR5 genes was performed. In total, 26 CPR5 family members were identified in Gossypium species. Phylogenetic analysis, structural characteristics, and synteny analysis of CPR5s showed the conserved evolution relationships of CPR5. The promoter analysis of CPR5 genes revealed hormone, stress, and development-related cis-elements. Gene ontology (GO) enrichment analysis showed that the CPR5 genes were largely related to biological regulation, developmental process, multicellular organismal process. Protein-protein interaction analysis predicted several trichome development related proteins (SIM, LGO, and GRL) directly interacting with CPR5 genes. Further, nine putative Gossypium-miRNAs were also identified, targeting Gossypium CPR5 genes. RNA-Seq data of G. arboreum (with trichomes) and G. herbaceum (with no trichomes) was used to perform the co-expression network analysis. GheCPR5.1 was identified as a hub gene in a co-expression network analysis. RT-qPCR of GheCPR5.1 gene in different tissues suggests that this gene has higher expressions in the petiole and might be a key candidate involved in the trichome development. Virus induced gene silencing of GheCPR5.1 (Ghe02G17590) confirms its role in trichome development and elongation. Current results provide proofs of the possible role of CPR5 genes and provide preliminary information for further studies of GheCPR5.1 functions in trichome development.
Trichomes have been found in most terrestrial plants and been proved to be the first barrier to protect from insects while being harmless to the environment. (Konarska and Lotocka, 2020; Kitagawa and Jackson, 2022). The special structure of trichomes, which are generally unicellular and multicellular, make trichomes a unique model to study the cell development process on plants (Kusstatscher et al., 2020; Ghazalli et al., 2021). During the past decades, the research on the formation mechanism of plant trichomes showed that multiple genes participate in the regulation of trichome initiation and elongation, such as genes involved with classical bHLH-MYB-WD40 complex (Liu et al., 2021; Li et al., 2022). However, various genes which also play important roles in trichome formation had been not studied deeply, such as CPR5 (constitutive expressor of pathogenesis-related genes 5) (Bowling et al., 1997).
Cotton trichomes are mainly distributed on the surface of the seed, leaf, and stem. The trichomes located on the seed coat of cotton, also known as cotton fiber, are the main economic harvest product. Cotton leaf and stem surface trichomes are similar to cotton fiber in structure, and the development process is regulated by genes related to fiber development (Bao and Hua, 2014; Wan et al., 2014). GhTCP14 was mainly expressed in cotton fiber, especially during the initiation and elongation of cotton fiber cells. When GhTCP14 was overexpressed in Arabidopsis thaliana, the number and length of trichomes were significantly increased. Overexpressed Arabidopsis thaliana plants having GhPIN1a, GhPIN6, and GhPIN8 had more and longer epidermal trichomes on the leaves (Zhang et al., 2017). GbML1 and GhMYB25, related to fiber development, shared a similar expression pattern and function on trichomes density and length in Arabidopsis thaliana (Zhang et al., 2010). GbML1 and GhMYB25 form a physical interaction through the START-domain (GbML1) and SAD-domain (GhMYB25) (Walford et al., 2012). Therefore, it was speculated that GbML1 may act as a molecular chaperone to regulate the development of fibroblasts by enhancing the expression of GhMYB25. GaHOX1 of Gossypium hirsutum had high homology with Gl2, which was mainly expressed in fiber at the early stage of development (Wang et al., 2013). GaHOX1 could complement the phenotypic defects of Arabidopsis mutant Gl2 and make it grow again. Overexpression of GhHOX3 in Gossypium hirsutum could make the cotton fiber longer and silencing the gene could lead to a reduction of more than 80% of the cotton fiber length (Hu et al., 2018). There is a similarity between the mechanism of hairiness control and cotton fiber development. The research on the mechanism of trichome elongation and development can provide a reference for revealing the mechanism of cotton fiber development to a certain extent.
According to the gene function annotation, there were five genes involved with constitutive expression of pathogenesis-related (PR) genes, CPR1 with F-box domain, CPR5, CPR6, CPR20, and CPR21 (Wei et al., 2019; Anisimova et al., 2021). All of them had been detected as participating in signal transduction pathways involved in plant defense, but not sharing the same domain. Besides, only CPR5 was found to relate with the trichome development process (Kirik et al., 2001; Peng et al., 2020), which made it more important to determine the resistance to biotic and abiotic stress in plant species.
CPR5 was identified as a defense response gene with a striking feature 5’ C-terminal transmembrane segments (TMSs) in a 1 + 4 TMS arrangement (Van Dingenen, 2022). Generally, the TMSs structure of CPR5 protein C-terminal showed their function on being a nuclear membrane protein as a component of nuclear pore complex (NPC) and regulating the effector-triggered immunity (ETI) and programmed cell death (PCD) on plants (Yoshida et al., 2002; Peng et al., 2020). For the C-terminal parts of the CPR5 protein, a previous study indicated that three parts existed: nuclear localization signals clusters, casein kinase phosphorylation sites clusters, and alternative mutation sites (Gu et al., 2016). CPR5 participated in various pathways, including plant growth, bacterial and fungal resistance, and trichome development (Jing et al., 2007; Jing and Dijkwel, 2008).
According to past research, the CPR5 was nearly found in plans, such as Vitis vinifera, Theobroma cacao, Qryza sativa, Gossypium hirsutum, and Triticumm aestivum (Orjuela et al., 2013; Pinel-Galzi et al., 2016). Various CPR5 homologous genes were present in different plant species. The numbers of most CPR5 genes were consistent with the genome numbers of the host plants. The CPR5 gene was first cloned in an Arabidopsis mutant line which showed resistance to Pseudomonas syringae and abnormal trichomes (Kirik et al., 2001). Based on the research of CPR5 sequence and structure, CPR5 was found to be a transmembrane protein and sustain the balance between homeostasis, cell division, and cell death by gating multiple transcription factors entering the nucleus (Deuschle et al., 2001; Gao et al., 2011; Gu et al., 2016). The involvement of CPR5 with plant disease defense had been deeply studied in Arabidopsis. The study showed that CPR5 played important roles in signaling pathways, such as CKI-RB-E2F cell cycle signaling pathway, activated immune responses, and ROS signal transduction (Borghi et al., 2011; Chen et al., 2022). CPR5 was also found to be involved with multiple plant hormones signaling pathways, such as salicylic acid and ethylene (Clarke et al., 2004). Besides the CPR5 function to disease resistance, the mechanism of trichomes development also been detected in CPR5 mutant plants, such as Arabidopsis, Soybean, and Mucuna (Okuma et al., 2014; Meng et al., 2017). The study showed that CPR5 was a downstream gene controlled by two CKI signaling pathway genes, SIM and SMR1. SIM SMR1 double loss mutant produced a cell death and branchless trichomes in Arabidopsis (Yi et al., 2014; Kumar et al., 2015). Another study showed that UVI4 and OSD1 interacted with CPR5 to regulate the trichomes development, indicating that CPR5 was essential in cell cycle progression (Heyman et al., 2011). Those studies had showed that CPR5 was involved in trichomes development, but the mechanism was still unclear.
Currently, we performed the genome wide analysis of CPR5 genes in cotton and explored their role in trichome development. Expression levels of the CPR5 gene in different tissues suggests that Ghe02G17590 might be the true candidate gene that plays a very important role in trichome development.
In order to identify CPR5 genes, the sequences of one At5g64930 protein was retrieved from the A. thaliana genome and was subsequently used for determining the CPR5 genes in the genomes of Gossypium arboreum (A1), Gossypium herbaceum (A2), Gossypium anomalum (B1), Gossypium sturtianum (C1), Gossypium thurberi (D1), Gossypium raimondii (D5), Gossypium stocksii (E1), Gossypium longicalyx (F1), Gossypium australe (G2), Gossypium rotundifolium (K2), Gossypium hirsutum (AD1), Gossypium barbadense (AD2), Gossypium tomentosum (AD3), Gossypium mustelinum (AD4), Gossypium darwinii (AD5). Besides, four other plant species (Arabidopsis thaliana, Oryza sativa, Theobroma cacao, Vitis vinifera) via reciprocal blast with BLASTP program (Altschulet al., 1997). The default parameters with E-values of less than 1 E−10 were set in the BLASTP searches (Supplementary Table S1).
The genome, protein, and structure information of Gossypium herbaceum (A1, version WHU_V1), Gossypium arboretum (A2, version WHU_updated_V1), Gossypium raimondii (D5, version JGI_V2_a2.1), Gossypium hirsutum (AD1, version HAU_V1.1), Gossypium barbadense (D5, version HAU_V2_a1), were downloaded form Cottongen (https://www.cottongen.org/). The data for the other ten cotton species were obtained from NCBI (https://www.ncbi.nlm.nih.gov/). Data for rabidopsis thaliana, Oryza sativa, Theobroma cacao, and Vitis vinifera were obtained from phytozome database 12 (https://phytozome-next.jgi.doe.gov/).
The protein sequences of all the discovered CPR5 genes from the cotton species as well as Arabidopsis thaliana, Oryza sativa, Theobroma cacao, and Vitis vinifera were aligned via Clustal X using default parameters (Larkin et al., 2007). The phylogenetic tree of CPR5 from each species was constructed using the numerous sequence alignments imported and displayed in MEGA seven using the neighbor joining approach. For statistical reliability, tree nodes were calculated using the Bootstrap method with 1,000 repeats (Tamura et al., 2007).
The gene structure of CPR5 genes was examined by GSDS 2.0 (https://gsds.cbi.pku.edu.cn/) using the Genomic DNA and CDS sequence of each species (Hu et al., 2015). CottonFGD genome annotation files were used to identify the chromosome locations of CPR5 genes in these species. This information was also used to construct chromosomal mapping which were then displayed by TBtools (Chen et al., 2020). All of the proteins sequences were submitted to the online motif and domain identification tool MEME (http://meme-suite.org/) in order to discover the conserved domains present in the CPR5 proteins (Bailey et al., 2009). The motif search was carried out using a total count of 15 motifs. The MAST tool was used to show the protein database for the identified motifs. Multicollinearity ScanToolkit was used for analyzing the synteny relationship between and within four cotton species, G. hirsutum and G. barbadense, G. herbaceum, and G. raimondii. The collinearity among orthologs and paralogs genes were displayed by TBtools (Chen et al., 2020).
The protein sequences of CPR5 genes were uploaded to WoLF PSORT online website (https://wolfpsort.hgc.jp) for subcellular localization prediction.
The promoter regions up to 2000 bp upstream in the CPR5 genes were downloaded from the cotton database (www.cottonfgd.org) and used to analyze potential cis-regulatory elements by the online tool PlantCARE (Lescot et al., 2002) (https://bioinformatics.psb.ugent.be/webtools/plantcare/html/).
G. arboreum and G. herbaceum were used for the transcriptome sequencing. The RNA-Seq libraries were prepared by a previously described method (Wang et al., 2018), and a 1% agarose gel was used to check for contamination and degradation of RNA. First, the purity of RNA was analyzed using a Nano Photometer® spectrophotometer (IMPLEN, CA, United States). Next, estimating RNA concentration was performed using a Qubit® RNA Assay Kit and a Qubit® 2.0 Fluorometer (Life Technologies, CA, United States). Finally, RNA integrity was checked using an Agilent Nano 6000 assay kit (Santa Clara, California, United States). Reads counting features (genes, in this case) were performed using HTSeq v0.6.125. Gene lengths and read counts mapped to genes were used to calculate FPKM values (Mortazavi et al., 2008). The original data was uploaded to NCBI (PRJNA833579).
The differentially expressed genes (DEGs) between diploid and tetraploid were identified with the DESeq R package (Andino et al., 2016), and Benjamini–Hochberg-adjusted p-values < 0.05 were considered statistically significant (Benjamini and Hochberg, 1995; Anders and Huber, 2010).
The gene ontology information of all CPR5s was obtained by using online genome-wide functional annotation tool EGGNOG-MAPPER (http://eggnog-mapper.embl.de/) and displayed by TBtools. The amino acid sequences of Arabidopsis CPR5 genes were used as query sequences to obtain the protein-protein network by using STRING website (https://cn.string-db.org/).
The gene sequences of the Gossypium CPR5 genes were used as candidate genes to identify possible miRNAs via observing against the existing Gossypium reference of miRNA sequences via the psRNATarget database (https://www.zhaolab.org/psRNATarget/analysis?function=2, accessed on 5 May 2022) with default parameters (Raza et al., 2021). Cytoscape (V3.8.2, https://cytoscape.org/download.html, accessed on 5 May 2022) software was used to build an interaction network between the identified miRNAs and the equivalent target Gossypium CPR5 genes.
We used RNA-Seq data of G. arboreum with trichomes and G. herbaceum with no trichomes to study the expression patterns of the CPR5 gene family. The links between genes involved in trichome development were examined by coexpression network analysis. A coexpression regulation network was created using the Cytoscape software (version 3.7.2) (Shannon et al., 2003). The threshold for the coexpression network map was set as p > 0.99. The topological coefficient of each node with a degree >20 was used to identify the network as hub genes.
A 285 bp fragment from the CDS sequence of GheCPR5.1 was selected to detect the gene function in regulation of trichome development. The TRV: 00 plasmid was digested with the restriction enzymes EcoRI and BamHI and combined with the target fragment to generate TRV: GheCPR5.1. TRV: GhCHL1 was used as the positive control. TRV: 00, TRV: GheCPR5.1, TRV: GhCHL1 were transformed in Agrobacterium tunefaciens LBA4404 and infiltrated into the cotyledons of 10-day-old Dianya-10. Three biological replicates (each with 20 plants) were performed. Cotton seedlings were grown in the plastic pot filled with solid culture medium (vol/vol, sterile sand: vermiculite: nutritious soil = 1:1:2) in incubators at 25°C during the day and 20°C at night, with 60% relative humidity and a 16/8 h light/dark photoperiod. The leaf blenching phenotype appeared in the TRV: GhCHL1 plants at 15–25 days, the trichome phenotype appeared in wild type plants at 20 days, we selected 25 days as the time to investigate the phenotype and VIGS silencing efficiency. The primers used for vector construction and RT-qPCR are listed in Supplementary Table S2. The number of trichomes present of the petiole of wild type, TRV: 00 and TRV: GheCPR5.1 was counted under a Stereo microscope and classified as either villi (trichomes are extremely short and prostrate) or hairs (trichomes are long and upright). For each individual, the numbers of hair type trichomes were counted. The number of trichomes will be only counted on one side of petiole. The data was analyzed and displayed by Graphpad prism seven software.
The gene expression profiles of GheCPR5.1 were analyzed from RNA-Seq data. The FPKM values were used to present the GheCPR5.1expression levels. RNA was extracted by TIANGEN kit following the protocol guidelines and was reverse transcribed into cDNA-by-cDNA synthesis SuperMix for qPCR (one step for gDNA removal). Finally, quantitative RT-qPCR was analyzed using the SYBR Green SuperMix kit according to the instruction manual. The experiment was performed using three technical and biological replications. The relative expression data were calculated using the 2−ΔΔCT method (Schmittgen and Livak, 2008). The primers used in the experiment are designed via NCBI (https://www.ncbi.nlm.nih.gov/) and Ghactin7 (LOC107959437) gene was used as an internal control (Supplementary Table S2).
In total, 33 CPR5 family members were detected in the plant species mentioned before four CPR5 family members were found in Oryza sativa and Gossypium barbadense, three CPR5 family members were found in Gossypium hirsutum, Gossypium mustelium and Gossypium darwinii, two were found in Gossypium tomentosum, 1 was found in Arabidopsis, Vitis vinifera, Theobroma cacao, and all ten diploid cotton species, respectively. After filtering the splicing transcripts of CPR5s, the result showed that all tetraploid cotton species included only two CPR5s, including O. sativa. The tetraploid cotton species contain twice the number of CPR5 genes as compared to diploid cotton. The result showed the conserved process of CPR5 gene family duplication during cotton whole genome duplicated events. The CPR5 gene family were renamed according to their positions on chromosomes and the number of splicing viraties. Ghe, Gar, Ghir, Gbar, Gtom, Gmus, Gdar, Gano, Gstu, Gthu. Grai, Gsto, Glon, Gaus, and Grot, were used as prefixes before the names of CPR5 genes, respectively.
Among all 26 CPR5 genes in Gossypium, the amino acid length ranged from 401 amino acid (G. hirsutum and G. barbadanse) to 610 amino acid (G. thurberii). PI (isoelectric point) ranged from 8.56 (GlonCPR5.1) to 9.26 (G.thurberi), MW (molecular weight) ranged from 30379.1 (GlonCPR5.1) to 68467.08 (GthuCPR5.1), Instability index ranged from 37.26 (GlonCPR5.2) to 52.08 (GtomCPR5.2). Most of the CPR5 genes were predicted to be located in the plasma membrane. Physical and chemical properties of CPR5 genes in Gossypium were given in (Supplementary Table S1).
To better understand the evolutionary relationship among CPR5 genes in Gossypium species mentioned in materials part and O. sativa, T. cacao, A. thaliana, the amino acid sequences were aligned with Clustal X software, and an unrooted phylogenetic tree was constructed using MEGA seven software (Figure 1). For the phylogenetic tree among eleven plant species, the 33 CPR5s were divided into five clades (clade A to E) depending on the common conserved CPR5 features. Clade E and D were the largest clades containing 13 and 10 CPR5s genes respectively and interestingly all of them belong to Gossypium species. Clade C with five CPR5 genes showed more associations with Vitis vinifera and Theobroma cacao. Clade A contains only one CPR5 gene belonging to Arabidopsis. Meanwhile clade B had four CPR5 genes belonging to O. sativa. The results suggested that Gossypium CPR5s show a closer relationship with CPR5s from Vitis vinifera and Theobroma cacao. The phylogenetic analysis suggests that the cotton CPR5 genes are more closely linked to each other. For example, pairs of homologous genes, GheCPR5.1/GarCPR5.1, GhirCPR5.1/GbarCPR5.3 were clustered in one group.
FIGURE 1. Phylogenetic tree of CPR5 proteins from A. thaliana, O. sativa, Vitis vinifera, and Theobroma cacao. The CPR5 were divided into five groups (A–E) on the clustering of the protein sequences. Cluster A contains one protein from A. thaliana, cluster B contains four proteins from O. sativa. Cluster C contains the proteins from G. herbaceum, Gossypium mustelium, Gossypium darwinii, Gossypium tomentosum, Gossypium hirsutum, Gossypium sturtianum, Gossypium austral, Gossypium rotundifolium. T. cacao, and Vitis vinifera, whereas, clades (D,E) contains 10 and 13 genes each belonging to Gossypium species.
We investigated the genetic architecture of CPR5 proteins by studying the exon-intron structural distribution (Figure 2). The number and size of introns and exons of genes were usually conserved in plant species. The conserved features could be used to detect the evolutionary relationship among the gene family. The results showed that CPR5s of all Gossypium species which we used in this study harbored four introns and five exons except the variety splicing CPR5 genes, such as GbarCPR5.2 and GhirCPR5.1.
FIGURE 2. Motif and gene structure analysis of CPR5 genes. On the left side is the distribution of the CPR5 conserved motifs in cotton and rice. Five motifs, i.e., motifs 1, 2, 6, 7, and 13 were conserved motifs, shown by green, yellow, purple, light green, and magenta. Right side: intron and exon structure of cotton and rice CPR5 genes. Exons and the UTR are shown as green and yellow boxes, respectively whereas introns are represented as grey lines.
By analyzing the protein sequences of CPR5 genes in Gossypium species using the MEME online tool, 15 motifs with significant E-values were found. The most five conserved motifs, motif 1, 2, 6, 7, and 13 were observed in all CPR5s. According to the CPR5 motif function annotation in Arabidopsis, these five motifs were identified as five transmembrane features, which played an essential role in regulating multiple pathways of CPR5. Motifs 4, 10, 11, 12, and 14 were only found in Gossypium species. Those five motifs sites in Arabidopsis were predicted as Mobidblt-Consensus Disorder region, which was identified as containing putative nuclear localization signal sites. Most CPR5 genes clustered in the same group shared the same motif features. For example, the CPR5s lacking motifs 2, 6, 7, and 13 were only observed in group 2. The result of motif analysis was consistent with the phylogenetic relationship. The clades with special motifs likely shared different functions.
The gene distribution of CPR5 genes on the chromosomes varies between the tetraploid and diploid cotton species (Figure 3). For diploid Gossypium species, G. herbaceum, G. arboretum, and G. raimondii, the CPR5 genes were only found on chromosomes 2. For tetraploid Gossypium species, the CPR5 genes were only found on chromosomes 2 and chromosomes 3. The gene distribution of G. herbaceum, G. arboreum, and G. raimondii was shown to be highly conserved and consistent with the A and D subgenome of G. hirsutum and G. barbadense, indicating the CPR5s conserved the evolution pattern between diploid cotton and tetraploid cotton. All CPR5 genes were found on the two end sides of chromosomes in cotton.
FIGURE 3. Distribution of the CPR5 genes on chromosomes of different cotton species i.e., A1, A2, AD1, AD2, D5. (A) A1 (Gossypium herbaceum). (B) A2 (Gossypium arboreum). (C) AD1 (Gossypium hirsutum). (D) AD2 (Gossypium barbadense). (E) D5 (Gossypium raimondii). The scale bar represents the chromosome length. The yellow bar indicates the chromosomes. The gene on each chromosome is highlighted in red.
The gene duplication was the main reason to produce and expand the gene family. Three events were observed in plant species gene duplication, including tandem (a chromosomal region within 200 kb containing two or more genes is defined as a tandem duplication event), segmental (multiple genes through polyploidy followed by chromosome rearrangements), and whole genome duplication (the process by which a region of DNA coding for a gene creates additional copies of the gene). The gene duplication events may influence the gene function diversity among plant species. In this study, we used two tetraploid cotton species, G. hirsutum (AD1) and G. barbadense (AD2), and two diploid cotton species. G. herbaceum (A2) and G. raimondii (D5) to demonstrate the evolutionary relationship and syntenic association of CPR5s in cotton. Synteny analysis between G. hirsutum (AD1), G. herbaceum (A2) and G. raimondii (D5) revealed that the CPR5s were reserved among three species (Figure 4). For within genome synteny analysis, two duplication gene pairs, GhirCPR5.1/GhirCPR5.3 and GbarCPR5.4/GbarCPR5.1, were found in G. hirsutum and G. barbadense, respectively (Figures 4C,D).
FIGURE 4. Syntenic relationships among CPR5 genes in A2, D5 and AD2 and AD1 and AD2. (A, B) CPR5 genes are plotted against their projected complements in the five species. The chromosomes of AD1 are shown as number A1‐A13, and the chromosomes of D5 are shown as D2-01 to D2-13 whereas in AD2 the chromosomes were shown as Gbar A1-Gbar-A13. The grey lines indicate the collinear blocks between different species. The blue lines represent the collinear CPR5 gene pairs between A2, D5 and AD2. The orange line represents the collinear CPR5 gene pairs between AD1 and AD2. (C, D) The CPR5 collinear gene pairs on A2, D5 and AD2 chromosomes. Red line represents a pair of collinear genes.
The cis-elements analysis results showed that the TCA-element and TATA-box motifs were distributed in all CPR5 genes of Gossypium species (Figure 5). Compared with other diploid cotton species, the number of TCA-elements and TATA-box motifs were higher in tetraploid cotton species. The results revealed that these two cis-elements have essential roles in CPR5 function in disease resistance and stress response. All cis-elements predicted from cotton species are mostly involved in stress environment response, such as light response, low temperature, wound, and multiple hormone pathways, such as salicylic acid, abscisic acid, auxin, and gibberellin. Generally, the light response elements and salicylic acid response elements are distributed in most CPR5s.
FIGURE 5. Predicted cis-elements in CPR5 promoters. Promoter sequences (2,000 bp upstream regions) of 18 CPR5 genes were examined online at PlantCARE web server. Diverse colors were used for representing different cis-elements, as given in on the right side.
To identify the function of 26 CPR5 family genes, we performed the GO annotation and enrichment analysis based on their biological process, molecular function, and cellular component classes. The GO annotation results showed that several GO terms were enriched in biological processes and cellular components (Figure 6A). The GO-biological process enrichment results suggested that 10 terms were highly enriched, such as biological process involved in interspecies interaction between organisms (GO: 0044419), biological regulation (GO: 0065007), developmental process (GO: 0032502), immune system process (GO: 0002376), multicellular organismal process (GO: 0032501), response to stimulus (GO: 0050896), etc., These terms confirmed the function of CPR5 genes in the disease defense and immune system regulation of plants. The GO-cellular component enrichment results suggested that one term was highly enriched, the cellular anatomical entity (GO: 0110165). This result also was consistent with the subcellular localization prediction of CPR5 proteins. To further identify the potential biological functions of CPR5 in Arabidopsis, the protein-protein interaction analysis was performed and 10 potential interactors were detected (Figure 6B). Notably, several trichomes development related proteins (SIM, LGO, and GRL) directly interacted with CPR5, suggesting its regulatory role in trichomes formation. Moreover, CPR5 showed a highly closed relationship with several hormone response proteins, such as Salicylic acid signaling pathway regulators (SSI2, ACD6, EDS16, PAD4, and EDS1), Auxin signaling pathway regulator (AXR4).
FIGURE 6. Gene ontology and protein-protein network analysis of CPR5. (A)- Gene ontology analysis of CPR5 genes. (B)- Protein-protein analysis of CPR5 in Arabidopsis. Colored nodes: query proteins and first shell of interactors. Red lines: gene fusions. Green lines: gene neighborhood. Black lines: co-expression. Blue lines: gene co-occurrence.
It has been reported previously that miRNAs dependent regulations have significant impacts on plant growth and regulation. Thus, to strengthen our understanding of the miRNAs associated with the regulation of Gossypium CPR5 genes that are involved in the development of trichomes, we identified nine putative miRNAs targeting 25 Gossypium CPR5 genes (Figure 7). The detailed information of the miRNA targeted sites is presented in Supplementary Table S3. We found that ghr-miR7490 interacts with one gene GlonCPR5.2, ghr-miR7496a, b interacts with GanoCPR5.1, ghr-miR7504a interacts with GraiCPR5.1, ghr-miR7484a,b interacts with GarCPR5.1 and GrotCPR5.1, ghr-miR7499 interacts with GtomCPR5.1, GausCPR5.1, GhirCPR5.1, GthuCPR5.1, GstuCPR5.1, and GheCPR5.1. Ghr-miR7488 interacts with GrotCPR5.1, GausCPR5.1, GarCPR5.1, GthuCPR5.1, GstuCPR5.1, and GheCPR5.1. Whereas ghir-miR7493 interacts with all the CPR5 genes except GlonCPR5.2 (Figure 7; Supplementary Table S3).
FIGURE 7. A network representation of the regulatory connections among the predicted miRNAs and Gossypium CPR5 genes. Different colors highlight the interacting miRNAs. Similarly, different node colors and shapes clearly represent the interaction among miRNAs and Gossypium CPR5 genes.
In total, 9,673 differentially expressed genes were identified between G. arboreum with a high density of trichomes and G. herbaceum with barely any trichomes. A co-expression network analysis was performed by using the differentially expressed genes, to identify the hub genes linked to trichomes development and elongation (Figure 8). Correlation based relationships were plotted using a Pearson correlation coefficient greater than 0.99. A threshold level of >20 edges was considered as hub genes from the analysis. Owing to this, we found Ghe02G17590 (GheCPR5.1) as a hub gene that might be involved in the trichome development.
FIGURE 8. The Pearson correlation network analysis. Co-expression network of DEGs. The gene in the center of the network with red color and octagonal shape is represented as a hub gene.
Expression of all identified CPR5 genes was recorded in roots, stems, leaves and petioles of different cotton species (Figure 9A). Results suggested that higher expressions were recorded in the petioles as compared to the other tissues. Moreover, we noticed that the expression of GheCPR5.1 was higher in the cotton species with trichomes.
FIGURE 9. Expression of CPR5 genes in different cotton species. (A)- Differential expression of all the CPR5 genes in roots, stem, leaves and petioles. (B)- Expression analysis of GheCPR5.1 in different tissues of G. arboretum and G. herbaceum. (C)- Density of trichomes in G. arboretum and G. herbaceum. (D)- Trichome density in all the Gossypium species used in this research work.
RT-qPCR was performed to check the expression patterns of GheCPR5.1 which was identified as a hub gene in the co-expression network analysis (Figure 9B). Expression was recorded in different tissues, i.e., of root, stem, leaves, and petiole of G. arboreum and G. herbaceum. We also performed the RT-qPCR to validate the expression of our candidate gene and the authenticity of RNA-seq data. G. arboreum with trichomes have higher expressions of GheCPR5.1in all the tissues, specifically petiole, as compared to G. herbaceum which has no trichomes. The RT-qPCR data was consistent with the RNA-seq data.
The VIGS assay was performed to investigate the GheCPR5.1 function related with the trichome development and elongation. The VIGS vector TRV: GheCPR5.1 was injected into G. arboreum (Dianya-10, the long trichome line), to generate the GheCPR5.1-silenced plants. Twenty days after inoculation, the blenching phenotypes were observed on TRV: GheCPR5.1. The expression level of GheCPR5.1 in wild type, negative control plant TRV: 00, and receptor plants TRV: GheCPR5.1 were detected by RT-qPCR. No significant variations were observed in the expression of the GheCPR5.1 gene in WT and TRV: 00. The expression of GheCPR5.1 in TRV: GheCPR5.1 lines was significantly lower than that of wild type and TRV: 00, indicating the success of the VIGS experiment Figure 10. The GheCPR5.1-silenced Dianya-10 plants exhibited a significant low density of long trichomes, compared with wild type and TRV: 00. These data suggest that GheCPR5.1 plays a key role in trichome elongation in cotton.
FIGURE 10. Virus induced gene silencing to elucidate the role of GheCPR5.1 in trichome development and elongation. (A)- Visual images of trichomes after gene silencing. (B)- The blenching phenotypes were observed on TRV: GheCPR5.1 plants. (C)- Relative expression level of GheCPR5.1 in root stem and leaves of silences plants. (D)- Trichome density in the leaves and petioles after GheCPR5.1 silencing in cotton.
In recent years, trichomes have been proven to be important for their involvement in multiple development pathways in various plant species, such as Arabidopsis, soybean, tomato, and tobacco (Lam and Pedigo, 2001; Choi et al., 2012; Cox and Smith, 2019; Fumin Wang et al., 2021). The trichomes functions of disease response, biotic stress defense, and maintaining the normal plant growth process have been deeply studied (Zhou et al., 2011; Yang and Ye, 2013; Zhou et al., 2013; Papierowska et al., 2020). Based on those results, lots of functional genes and signaling response pathways involved in trichomes initiation and elongation had been reported (Zhou et al., 2013; Yuan et al., 2019; Yu et al., 2021; Yuan et al., 2021). CPR5 was first isolated from an Arabidopsis disease response mutant line, and its function to non-normal trichomes development had been identified in 1997 (Bowling et al., 1997). Compared with a classical WD40-MYB-bHLH complex (Zhao et al., 2013; Zhao et al., 2019; Yang et al., 2021) and other genes involved in trichomes development (Zhang et al., 2005; Zhang et al., 2019; Yu et al., 2021), the mechanism of CPR5 regulating trichomes was still not clear. Furthermore, the cell structure of trichomes and fiber had been proved to share most similarity in cotton (Wang et al., 2004; Yang and Ye, 2013). Few research efforts focused on the genes regulating both trichomes and fiber and their function to cotton development (Shangguan et al., 2008; Shan et al., 2014).
In past few years, more research had been done on CPR5 gene function analysis, especially in Arabidopsis. Research demonstrated multiple CPR5 roles in plant growth (Kirik et al., 2001; Gao et al., 2011), plant immunity (Faisal et al., 2020; Van Dingenen, 2022), and trichome development by using mutant lines constructing, gene overexpression, and yeast split ubiquitin assay (Aki et al., 2007; Campbell et al., 2019). Previous results provided basic information of CPR5 gene location, function, and its regulatory factors, but did not suggest their roles in different plant species evolutionary processes. In this study, we first identified all 26 CPR5s in Gossypium species, including four cultivars and seven wild species. Previous studies showed that CPR5 genes were identified in both monocotyledons and dicotyledons. There were few CPR5s genes and their number varies in various plant species, such as six in Triticum aestivum, three in Brassica rapa, two in Populus trichocarpa and one in Vitis vinifera. Our results suggested that the CPR5 genes number is consistent with the genomes number of plant species. Generally, only one copy of the CPR5 gene is distributed in a diploid plant species, which indicated that CPR5 is a classical single copy gene.
Unlike the traditional method of only reserving the longest transcript sequence for each gene identified through blastP, in this study, we chose the differential splicing CPR5 sequences for the following analysis. The differential splicing alterations had been proved to produce numerous protein structures and function variances from one gene (Reddy, 2007; Park et al., 2013; Rehman et al., 2021). In the current study, we had identified multiple protein isoforms from one or two CPR5 genes in some Gossypium species, GhirCPR5.2/GhirCPR5.3,GbarCPR5.1/GbarCPR5.2/GbarCPR5.3, GmusCPR5.2/GmusCPR5.3, GdarCPR5.2/GdarCPR5.3, GlonCPR5.1/GlonCPR5.2. Between different cotton species, the differential splicing sequences shared similarities among amino acid sequence length, subcellular location, and motifs distribution. For example, the amino acid sequence length of GhirCPR5.3 and GbarCPR5.2 both were 401 aa and GmusCPR5.3 was 409 aa. The same results were also seen for the CDS lengths of the genes mentioned above. Meanwhile, the subcellular location suggested that all the genes were located in the nucleus. Combining the gene structure results, the genes located in the nucleus did not possess the motif 2, motif 6, motif 7, and motif 13, which were predicted as four transmembrane features. Previous study suggested that a truncated CPR5 protein could be located at the plant cell nucleus (Perazza et al., 2011), which is consistent with our results.
Many previous studies had indicated that the single copy gene could be used as a molecular marker in understanding the phylogenetic relationships of closely related species (Sudmant et al., 2010; Sun and Komatsuda, 2010; Ananda et al., 2021). In this study, we constructed an unrooted phylogenetic tree by using all 33 CPR5 genes identified from Arabidopsis thaliana, Oryza sativa, Vitis vinifera, Theobroma cacao, and Gossypium species. In this phylogenetic tree, the CPR5 gene of Arabidopsis was present in clade A, the CPR5 genes of Oryza sativa were present in clade B. Furthermore, we found the CPR5 genes of Vitis vinifera, Theobroma cacao, and Gossypium species were grouped in the same clade C. The general evolutionary relationship indicated that Vitis vinifera and Theobroma cacao show a closer relationship with Gossypium as compared with Arabidopsis and Oryza (Chen et al., 2016). These results were consistent with the phylogenetic analysis between the already mentioned four genera. As for the evolutionary relationship within the 26 Gossypium species that were displayed in our phylogenetic tree, the CPR5 genes that were identified from G. rotundifolium (K genome) and G. australe (G genome) showed a closer relationship with Theobroma, which is one of closest genera to Gossypium (Udall et al., 2019). Previous studies on phylogenetic relationships within Gossypium species also supported out results (Tang et al., 2015; Conover et al., 2019). According to these studies, G. herbaceum was closer to G. arboreum, G. barbadense and G. hirsutum were closer to G. darwinii., G. tomentosum, and G. mustelinum (Applequist et al., 2001; Renny-Byfield et al., 2016). The same phenomenon was also found in our study that GheCPR5.1 and GarCPR5.1 were grouped in one branch and GhirCPR5.3, GbarCPR5.4, GtomCPR5.1, GmusCPR5.1, and GdarCPR5.2 were grouped in closer branches. The CPR5 genes which share similar structures and motifs were also grouped together.
In this study, we chose five Gossypium species to display the CPR5 genes chromosome location and the result showed that only one copy of the CPR5 gene was located on chromosome 2 (G. arboreum A02 and G. raimondii D02) for all two diploid cotton species. For tetraploid species, all CPR5 genes were found to be located on chromosomes A02 and D03. Previous study suggested that the tetraploid cotton species were formed by a hybridization process between A genome species and D genome species (Gong et al., 2012; Renny-Byfield and Wendel, 2014). These results indicated that the CPR5 genes number of tetraploid cotton was twice than the number in diploid cotton, resulting in a whole-genome duplication event during the cotton polyploidization process.
The cis-elements including enhancers and promoters have been had their regulating mechanism of gene expression and function proven (Shan et al., 2014; Zhao et al., 2018). By identifying the Cis-element gaining and losing, it was critical to provide the source of morphological evolution influenced by gene function diversity (Li et al., 2020; Mengarelli and Zanor, 2021). In this study, several cis-elements of CPR5 genes were predicted in Gossypium species. The same cis-elements, TCA-element, and TATA-box motifs, showed their core role in regulating CPR5 gene expression. The studies showed that TCA-element is essential to regulating the genes expression to exogenous salicylic acid response in Oryza sativa (Nakshatri and Chambon, 1994; Rai et al., 2004). As for TATA-box, the function to the control transcriptional modulation mediated by miRNA have been identified in plant species (Yang et al., 2017; Lee et al., 2020; Ramalingam et al., 2021). Current results showed that the same CPR5 genes regulate different regulatory mechanisms by various cis-elements distribution in plant species. The salicylic acid response element was mostly found in CPR5 genes of Gossypium species. The CPR5 gene has had its function proven to modulate salicylic acid to regulate pant growth and stress responses (Jirage et al., 2001; Lemarié et al., 2015). Those results revealed that CPR5 would be regulated by multiple hormones, especially the salicylic acid. Gene ontology (GO) enrichment analysis showed that the CPR5 genes were largely related to biological regulation, developmental process, and multicellular organismal process Xiaojing Wang et al., 2021.
MicroRNAs (miRNAs), that are a group of single-stranded, non-coding micro RNAs, are involved in post-transcriptional gene regulation (Cui et al., 2020). Various miRNAs have been identified via genome-wide analysis that are involved in growth and development in cotton (Zhang et al., 2007; Kwak et al., 2009; Ayubov et al., 2019). The current study identified nine miRNAs belonging to different families (ghr-miR7493, ghr-miR7484a, ghr-miR7484b, ghr-miR7488, ghr-miR7490, ghr-miR7496a, ghr-miR7496b, ghr-miR7499, ghr-miR7504a) targeting 25 Gossypium CPR5 genes (GheCPR5.1, GarCPR5.1, GhirCPR5.1, GbarCPR5.2, GausCPR5.1, GbarCPR5.1, GtomCPR5.1, GbarCPR5.3, GdarCPR5.2, GmusCPR5.2, GmusCPR5.3, GmusCPR5.1, GstuCPR5.1, GdarCPR5.3, GdarCPR5.1, GrotCPR5.1, GthuCPR5.1, GlonCPR5.1, GanoCPR5.1, GlonCPR5.2, GhirCPR5.2, GbarCPR5.4, GtomCPR5.2, GhirCPR5.3, GraiCPR5.1). Discussed miRNAs in the current study are all involved in the cotton plant growth and development and trichome development as reported earlier (Guan et al., 2014; Xue et al., 2014; Li and Zhang, 2016; Wang et al., 2017; Xiaojing Wang et al., 2021). These studies suggest that these Gossypium-miRNAs might play potential roles in plant growth and trichome development by modifying the transcript level of the CPR5 genes in Cotton.
In the current research based on transcriptome analysis of G. arboretum with trichomes and G. herbaceum with no trichome and co-expression network analysis we found that Ghe02G17590 is the hub gene. This gene is also a CPR5 gene and it has higher expressions in G. arboretum with trichomes and G. herbaceum with no trichomes (Yonekura-Sakakibara and Saito, 2013).
Virus induced gene silencing of Ghe02G17590 confirms that this might be the true candidate gene that is involved in the trichome development and elongation. Virus induced gene silencing is an important method to predict the function of a candidate gene and previously many studies on cotton have been published to confirm the role of candidate genes (Zhao et al., 2021).
Keeping in view the importance of CPR5 genes in plant growth and development and its role in trichome development further functional characterizations are needed to understand the molecular and genetics mechanisms of trichome development in cotton.
The CPR5 genes have a significant impact on crop tolerance to biotic and abiotic stress. Currently, we performed the genome wide identification, transcriptome analysis, co-expression, and RT-qPCR profiling and proved that CPR5 genes have potential roles in trichome development. The co-expression network analysis and RT-qPCR results showed that GheCPR5.1 is the hub gene and is involved in trichome development. Virus induced gene silencing of Ghe02G17590 confirms its potential role in trichome development and elongation. This gene might have a positive contribution in trichome development in G. hirsutum. The importance of CPR5 genes in plant growth, development, and trichome formation is summarized in this study and more functional characterization of GheCPR5.1 is needed for conclusive findings at molecular levels.
The datasets presented in this study can be found in online repositories. The name of the repository and accession number can be found below: NCBI; PRJNA833579.
Conceptualization, HW and MU; methodology, HW and MU; software, HW, MU, FL, and XC; validation, HW, MU, FL, and XC; formal analysis, HW, MU, FL, XC, YX, YH, and ZZ; investigation, HW, MU, FL, XC, YX, YH, and ZZ; resources, HW and MU; data curation, HW and MU; writing original draft preparation, HW and MU; review and editing, HW and MU; visualization, HW and MU; supervision, ZZ; project administration, ZZ; funding acquisition, ZZ. All authors have read and agreed to the published version of the manuscript.
This research was supported by the National Natural Science Foundation of China (32171994).
The authors declare that the research was conducted in the absence of any commercial or financial relationships that could be construed as a potential conflict of interest.
All claims expressed in this article are solely those of the authors and do not necessarily represent those of their affiliated organizations, or those of the publisher, the editors and the reviewers. Any product that may be evaluated in this article, or claim that may be made by its manufacturer, is not guaranteed or endorsed by the publisher.
The authors thank all the Wild Cotton Research Group members, Institute of Cotton Research of CAAS.
The Supplementary Material for this article can be found online at: https://www.frontiersin.org/articles/10.3389/fgene.2022.921096/full#supplementary-material
Aki, T., Konishi, M., Kikuchi, T., Fujimori, T., Yoneyama, T., and Yanagisawa, S. (2007). Distinct Modulations of the Hexokinase1-Mediated Glucose Response and Hexokinase1-Independent Processes by HYS1/CPR5 in Arabidopsis. J. Exp. Bot. 58 (12), 3239–3248. doi:10.1093/jxb/erm169
Ananda, G., Norton, S., Blomstedt, C., Furtado, A., Møller, B., Gleadow, R., et al. (2021). Phylogenetic Relationships in the Sorghum Genus Based on Sequencing of the Chloroplast and Nuclear Genes. Plant Genome 14 (3), e20123. doi:10.1002/tpg2.20123
Anders, S., and Huber, W. (2010). Differential Expression Analysis for Sequence Count Data. Genome Biol. 10, R106. doi:10.1186/gb-2010-11-10-r106
Andino, G. K., Gribskov, M., Anderson, D. L., Evans, J. D., and Hunt, G. J. (2016). Differential Gene Expression in Varroa Jacobsoni Mites Following a Host Shift to European Honey Bees (Apis mellifera). BMC genomics 17 (1), 926–1012. doi:10.1186/s12864-016-3130-3
Anisimova, O. K., Shchennikova, A. V., Kochieva, E. Z., and Filyushin, M. A. (2021). Pathogenesis-Related Genes of PR1, PR2, PR4, and PR5 Families Are Involved in the Response to Fusarium Infection in Garlic (Allium Sativum L). Int. J. Mol. Sci. 22 (13), 6688. doi:10.3390/ijms22136688
Applequist, W. L., Cronn, R., and Wendel, J. F. (2001). Comparative Development of Fiber in Wild and Cultivated Cotton. Evol. Dev. 3 (1), 3–17. doi:10.1046/j.1525-142x.2001.00079.x
Ayubov, M. S., Mirzakhmedov, M. H., Sripathi, V. R., Buriev, Z. T., Ubaydullaeva, K. A., Usmonov, D. E., et al. (2019). Role of MicroRNAs and Small RNAs in Regulation of Developmental Processes and Agronomic Traits in Gossypium Species. Genomics 111 (5), 1018–1025. doi:10.1016/j.ygeno.2018.07.012
Bailey, T. L., Boden, M., Buske, F. A., Frith, M., Grant, C. E., Clementi, L., et al. (2009). MEME SUITE: Tools for motif discovery and searching. Nucl. Acids Res. 37 (Web Server issue), W202 W208. doi:10.1093/nar/gkp335
Bao, Z., and Hua, J. (2014). Interaction of CPR5 with Cell Cycle Regulators UVI4 and OSD1 in Arabidopsis. PLoS One 9 (6), e100347. doi:10.1371/journal.pone.0100347
Benjamini, Y., and Hochberg, Y. (1995). Controlling the False Discovery Rate: a Practical and Powerful Approach to Multiple Testing. J. R. Stat. Soc. Ser. B Methodol. 57 (1), 289–300. doi:10.1111/j.2517-6161.1995.tb02031.x
Borghi, M., Rus, A., and Salt, D. E. (2011). Loss-of-function of Constitutive Expresser of Pathogenesis Related Genes5 Affects Potassium Homeostasis in Arabidopsis thaliana. PLoS One 6 (10), e26360. doi:10.1371/journal.pone.0026360
Bowling, S. A., Clarke, J. D., Liu, Y., Klessig, D. F., and Dong, X. (1997). The Cpr5 Mutant of Arabidopsis Expresses Both NPR1-dependent and NPR1-independent Resistance. Plant Cell 9 (9), 1573–1584. doi:10.1105/tpc.9.9.1573
Campbell, B. W., Hoyle, J. W., Bucciarelli, B., Stec, A. O., Samac, D. A., Parrott, W. A., et al. (2019). Functional Analysis and Development of a CRISPR/Cas9 Allelic Series for a CPR5 Ortholog Necessary for Proper Growth of Soybean Trichomes. Sci. Rep. 9 (1), 14757. doi:10.1038/s41598-019-51240-7
Chen, J., Sui, X., Ma, B., Li, Y., Li, N., Qiao, L., et al. (2022). Arabidopsis CPR5 Plays a Role in Regulating Nucleocytoplasmic Transport of mRNAs in Ethylene Signaling Pathway. Plant Cell. Rep. 41, 1075–1085. doi:10.1007/s00299-022-02838-1
Chen, Z., Feng, K., Grover, C. E., Li, P., Liu, F., Wang, Y., et al. (2016). Chloroplast DNA Structural Variation, Phylogeny, and Age of Divergence Among Diploid Cotton Species. PLoS One 11 (6), e0157183. doi:10.1371/journal.pone.0157183
Chen, C., Chen, H., Zhang, Y., Chen, H. R., Frank, M. H., He, Y., et al. (2020). TBtools: An integrative toolkit developed for interactive analyses of big biological data. Mol. Plant. 13 (8), 1194 1202. doi:10.1016/j.molp.2020.06.009
Choi, Y. E., Lim, S., Kim, H.-J., Han, J. Y., Lee, M.-H., Yang, Y., et al. (2012). Tobacco NtLTP1, a Glandular-specific Lipid Transfer Protein, Is Required for Lipid Secretion from Glandular Trichomes. Plant J. 70 (3), 480–491. doi:10.1111/j.1365-313X.2011.04886.x
Clarke, S. M., Mur, L. A. J., Wood, J. E., and Scott, I. M. (2004). Salicylic Acid Dependent Signaling Promotes Basal Thermotolerance but Is Not Essential for Acquired Thermotolerance inArabidopsis Thaliana. Plant J. 38 (3), 432–447. doi:10.1111/j.1365-313X.2004.02054.x
Conover, J. L., Karimi, N., Stenz, N., Ané, C., Grover, C. E., Skema, C., et al. (2019). A Malvaceae Mystery: A Mallow Maelstrom of Genome Multiplications and Maybe Misleading Methods? J. Integr. Plant Biol. 61 (1), 12–31. doi:10.1111/jipb.12746
Cox, N., and Smith, L. M. (2019). A Novel Upstream Regulator of Trichome Development Inhibitors. Plant Physiol. 181 (4), 1398–1400. doi:10.1104/pp.19.01269
Cui, C., Wang, J.-J., Zhao, J.-H., Fang, Y.-Y., He, X.-F., Guo, H.-S., et al. (2020). A Brassica miRNA Regulates Plant Growth and Immunity through Distinct Modes of Action. Mol. Plant 13 (2), 231–245. doi:10.1016/j.molp.2019.11.010
Deuschle, K., Funck, D., Hellmann, H., Däschner, K., Binder, S., and Frommer, W. B. (2001). A Nuclear Gene Encoding Mitochondrial Δ1-pyrroline-5-carboxylate Dehydrogenase and its Potential Role in Protection from Proline Toxicity. Plant J. 27 (4), 345–356. doi:10.1046/j.1365-313x.2001.01101.x
Faisal, M. B., Gechev, T. S., Mueller-Roeber, B., and Dijkwel, P. P. (2020). Putative Alternative Translation Start Site-Encoding Nucleotides of CPR5 Regulate Growth and Resistance. BMC Plant Biol. 20 (1), 295. doi:10.1186/s12870-020-02485-2
Gao, G., Zhang, S., Wang, C., Yang, X., Wang, Y., Su, X., et al. (2011). Arabidopsis CPR5 Independently Regulates Seed Germination and Postgermination Arrest of Development through LOX Pathway and ABA Signaling. PLoS One 6 (4), e19406. doi:10.1371/journal.pone.0019406
Gong, L., Salmon, A., Yoo, M.-J., Grupp, K. K., Wang, Z., Paterson, A. H., et al. (2012). The Cytonuclear Dimension of Allopolyploid Evolution: an Example from Cotton Using Rubisco. Mol. Biol. Evol. 29 (10), 3023–3036. doi:10.1093/molbev/mss110
Gu, Y., Zebell, S. G., Liang, Z., Wang, S., Kang, B.-H., and Dong, X. (2016). Nuclear Pore Permeabilization Is a Convergent Signaling Event in Effector-Triggered Immunity. Cell 166 (6), 1526–1538.e11. doi:10.1016/j.cell.2016.07.042
Guan, X., Song, Q., and Chen, Z. J. (2014). Polyploidy and Small RNA Regulation of Cotton Fiber Development. Trends Plant Sci. 19 (8), 516–528. doi:10.1016/j.tplants.2014.04.007
Heyman, J., Van den Daele, H., De Wit, K., Boudolf, V., Berckmans, B., Verkest, A., et al. (2011). Arabidopsis ULTRAVIOLET-B-INSENSITIVE4 Maintains Cell Division Activity by Temporal Inhibition of the Anaphase-Promoting Complex/Cyclosome. Plant Cell 23 (12), 4394–4410. doi:10.1105/tpc.111.091793
Hu, B., Jin, J., Guo, A. Y., Zhang, H., Luo, J., Gao, G., et al. (2015). GSDS 2.0: An upgraded gene feature visualization server. Bioinformatics 31 (8), 1296–1297. doi:10.1093/bioinformatics/btu817
Hu, H., Wang, M., Ding, Y., Zhu, S., Zhao, G., Tu, L., et al. (2018). Transcriptomic Repertoires Depict the Initiation of Lint and Fuzz Fibres in Cotton (Gossypium Hirsutum L.). Plant Biotechnol. J. 16 (5), 1002–1012. doi:10.1111/pbi.12844
Jing, H.-C., Anderson, L., Sturre, M. J. G., Hille, J., and Dijkwel, P. P. (2007). Arabidopsis CPR5 Is a Senescence-Regulatory Gene with Pleiotropic Functions as Predicted by the Evolutionary Theory of Senescence. J. Exp. Bot. 58 (14), 3885–3894. doi:10.1093/jxb/erm237
Jing, H.-C., and Dijkwel, P. P. (2008). CPR5, a Jack of All Trades in Plants. Plant Signal. Behav. 3 (8), 562–563. doi:10.4161/psb.3.8.5708
Jirage, D., Zhou, N., Cooper, B., Clarke, J. D., Dong, X., and Glazebrook, J. (2001). Constitutive Salicylic Acid-dependent Signaling in Cpr1 and Cpr6 Mutants Requires PAD4. Plant J. 26 (4), 395–407. doi:10.1046/j.1365-313x.2001.2641040.x
Kirik, V., Bouyer, D., Schöbinger, U., Bechtold, N., Herzog, M., Bonneville, J.-M., et al. (2001). CPR5 Is Involved in Cell Proliferation and Cell Death Control and Encodes a Novel Transmembrane Protein. Curr. Biol. 11 (23), 1891–1895. doi:10.1016/s0960-9822(01)00590-5
Kitagawa, M., and Jackson, D. (2022). A Forward Genetic Approach to Identify Plasmodesmal Trafficking Regulators Based on Trichome Rescue. Methods Mol. Biol. 2457, 393–407. doi:10.1007/978-1-0716-2132-5_27
Konarska, A., and Łotocka, B. (2020). Glandular Trichomes of Robinia Viscosa Vent. Var. Hartwigii (Koehne) Ashe (Faboideae, Fabaceae)-Morphology, Histochemistry and Ultrastructure. Planta 252 (6), 102. doi:10.1007/s00425-020-03513-z
Kumar, N., Harashima, H., Kalve, S., Bramsiepe, J., Wang, K., Sizani, B. L., et al. (2015). Functional Conservation in the SIAMESE-RELATED Family of Cyclin-Dependent Kinase Inhibitors in Land Plants. Plant Cell 27 (11), 3065–3080. doi:10.1105/tpc.15.00489
Kusstatscher, P., Wicaksono, W. A., Bergna, A., Cernava, T., Bergau, N., Tissier, A., et al. (2020). Trichomes Form Genotype-specific Microbial Hotspots in the Phyllosphere of Tomato. Environ. Microbiome 15 (1), 17. doi:10.1186/s40793-020-00364-9
Kwak, P. B., Wang, Q. Q., Chen, X. S., Qiu, C. X., and Yang, Z. M. (2009). Enrichment of a Set of microRNAs during the Cotton Fiber Development. BMC Genomics 10 (1), 457–511. doi:10.1186/1471-2164-10-457
Lam, W.-K. F., and Pedigo, L. P. (2001). Effect of Trichome Density on Soybean Pod Feeding by Adult Bean Leaf Beetles (Coleoptera: Chrysomelidae). Econ. Entomol. 94 (6), 1459–1463. doi:10.1603/0022-0493-94.6.1459
Larkin, M. A., Blackshields, G., Brown, N. P., Chenna, R., McGettigan, P. A., McWilliam, H., et al. (2007). Clustal W and Clustal X version 2.0. Bioinformatics 23 (21), 2947–2948. doi:10.1093/bioinformatics/btm404
Lee, J.-S., Lee, J. Y., Song, D. W., Bae, H. S., Doo, H. M., Yu, H. S., et al. (2020). Targeted PMP22 TATA-Box Editing by CRISPR/Cas9 Reduces Demyelinating Neuropathy of Charcot-Marie-Tooth Disease Type 1A in Mice. Nucleic Acids Res. 48 (1), 130–140. doi:10.1093/nar/gkz1070
Lemarié, S., Robert-Seilaniantz, A., Lariagon, C., Lemoine, J., Marnet, N., Jubault, M., et al. (2015). Both the Jasmonic Acid and the Salicylic Acid Pathways Contribute to Resistance to the Biotrophic Clubroot AgentPlasmodiophora Brassicaein Arabidopsis. Plant Cell Physiol. 56 (11), pcv127–2168. doi:10.1093/pcp/pcv127
Lescot, M., Dehais, P., Thijs, G., Marchal, K., Moreau, Y., Van de Peer, Y., et al. (2002). PlantCARE, a database of plant cis-acting regulatory elements and a portal to tools for in silico analysis of promoter sequences. Nucl. Acids Res. 30 (1), 325 327. doi:10.1093/nar/30.1.325
Li, C., and Zhang, B. (2016). MicroRNAs in Control of Plant Development. J. Cell. Physiol. 231 (2), 303–313. doi:10.1002/jcp.25125
Li, P., Fu, J., Xu, Y., Shen, Y., Zhang, Y., Ye, Z., et al. (2022). CsMYB1 Integrates the Regulation of Trichome Development and Catechins Biosynthesis in Tea Plant Domestication. New Phytol. 234 (3), 902–917. doi:10.1111/nph.18026
Li, R., Zhu, F., and Duan, D. (2020). Function Analysis and Stress-Mediated Cis-Element Identification in the Promoter Region of VqMYB15. Plant Signal. Behav. 15 (7), 1773664. doi:10.1080/15592324.2020.1773664
Ghazalli, M. N., Md, S. M., Mat, M., Awang, K., Jaafar, M. A., and Mirad, R. (2021). Leaf Anatomy and Micromorphology Characteristics of Ketum [Mitragyna speciosa (Korth.) Havil.] (Rubiaceae). Trop Life Sci Res. 32 (1), 107–117. doi:10.21315/tlsr2021.32.1.7
Liu, L., Yin, M., Lin, G., Wang, Q., Zhou, P., Dai, S., et al. (2021). Integrating RNA-Seq with Functional Expression to Analyze the Regulation and Characterization of Genes Involved in Monoterpenoid Biosynthesis in Nepeta Tenuifolia Briq. Plant Physiol. Biochem. 167, 31–41. doi:10.1016/j.plaphy.2021.07.026
Meng, Z., Ruberti, C., Gong, Z., and Brandizzi, F. (2017). CPR 5 Modulates Salicylic Acid and the Unfolded Protein Response to Manage Tradeoffs between Plant Growth and Stress Responses. Plant J. 89 (3), 486–501. doi:10.1111/tpj.13397
Mengarelli, D. A., and Zanor, M. I. (2021). Genome-wide Characterization and Analysis of the CCT Motif Family Genes in Soybean (Glycine Max). Planta 253 (1), 15. doi:10.1007/s00425-020-03537-5
Mortazavi, A., Williams, B. A., McCue, K., Schaeffer, L., and Wold, B. (2008). Mapping and Quantifying Mammalian Transcriptomes by RNA-Seq. Nat. Methods. 5 (7), 621–628. doi:10.1038/nmeth.1226
Nakshatri, H., and Chambon, P. (1994). The Directly Repeated RG(G/T)TCA Motifs of the Rat and Mouse Cellular Retinol-Binding Protein II Genes Are Promiscuous Binding Sites for RAR, RXR, HNF-4, and ARP-1 Homo- and Heterodimers. J. Biol. Chem. 269 (2), 890–902. doi:10.1016/s0021-9258(17)42196-x
Okuma, E., Nozawa, R., Murata, Y., and Miura, K. (2014). Accumulation of Endogenous Salicylic Acid Confers Drought Tolerance toArabidopsis. Plant Signal. Behav. 9 (3), e28085. doi:10.4161/psb.28085
Orjuela, J., Deless, E. F. T., Kolade, O., Chéron, S., Ghesquière, A., and Albar, L. (2013). A Recessive Resistance to Rice Yellow Mottle Virus Is Associated with a Rice Homolog of the CPR5 Gene, a Regulator of Active Defense Mechanisms. Mol. Plant-Microbe Interact. 26 (12), 1455–1463. doi:10.1094/MPMI-05-13-0127-R
Papierowska, E., Szatyłowicz, J., Samborski, S., Szewińska, J., and Różańska, E. (2020). The Leaf Wettability of Various Potato Cultivars. Plants 9 (4), 504. doi:10.3390/plants9040504
Park, J. W., Tokheim, C., Shen, S., and Xing, Y. (2013). Identifying Differential Alternative Splicing Events from RNA Sequencing Data Using RNASeq-MATS. Methods Mol. Biol. 1038, 171–179. doi:10.1007/978-1-62703-514-9_10
Peng, S., Sun, K., Guo, Y., Liu, Y., and Wang, S. (2020). Arabidopsis Nucleoporin CPR5 Controls Trichome Cell Death through the Core Cell Cycle Regulator CKI. Plant Biol. J. 22 (2), 337–345. doi:10.1111/plb.13068
Perazza, D., Laporte, F., Balagué, C., Chevalier, F., Remo, S., Bourge, M., et al. (2011). GeBP/GPL Transcription Factors Regulate a Subset of CPR5-dependent Processes. Plant Physiol. 157 (3), 1232–1242. doi:10.1104/pp.111.179804
Pinel-Galzi, A., Dubreuil-Tranchant, C., Hébrard, E., Mariac, C., Ghesquière, A., and Albar, L. (2016). Mutations in Rice Yellow Mottle Virus Polyprotein P2a Involved in RYMV2 Gene Resistance Breakdown. Front. Plant Sci. 7, 1779. doi:10.3389/fpls.2016.01779
Rai, R., Daugherty, J., Tate, J., Buford, T., and Cooper, T. (2004). Synergistic Operation of Four Cis-Acting Elements Mediate High Level DAL5 Transcription in Saccharomyces cerevisiae. FEMS Yeast Res. 5 (1), 29–41. doi:10.1016/j.femsyr.2004.06.004
Ramalingam, V., Natarajan, M., Johnston, J., and Zeitlinger, J. (2021). TATA and Paused Promoters Active in Differentiated Tissues Have Distinct Expression Characteristics. Mol. Syst. Biol. 17 (2), e9866. doi:10.15252/msb.20209866
Raza, A., Su, W., Gao, A., Mehmood, S. S., Hussain, M. A., Nie, W., et al. (2021). Catalase (CAT) Gene Family in Rapeseed (Brassica Napus L.): Genome-wide Analysis, Identification, and Expression Pattern in Response to Multiple Hormones and Abiotic Stress Conditions. Int. J. Mol. Sci. 22 (8), 4281. doi:10.3390/ijms22084281
Reddy, A. S. N. (2007). Alternative Splicing of Pre-messenger RNAs in Plants in the Genomic Era. Annu. Rev. Plant Biol. 58, 267–294. doi:10.1146/annurev.arplant.58.032806.103754
Rehman, S. u., Schallschmidt, T., Rasche, A., Knebel, B., Stermann, T., Altenhofen, D., et al. (2021). Alternative Exon Splicing and Differential Expression in Pancreatic Islets Reveals Candidate Genes and Pathways Implicated in Early Diabetes Development. Mamm. Genome 32 (3), 153–172. doi:10.1007/s00335-021-09869-1
Renny-Byfield, S., Page, J. T., Udall, J. A., Sanders, W. S., Peterson, D. G., Arick, M. A., et al. (2016). Independent Domestication of Two Old World Cotton Species. Genome Biol. Evol. 8 (6), 1940–1947. doi:10.1093/gbe/evw129
Renny-Byfield, S., and Wendel, J. F. (2014). Doubling Down on Genomes: Polyploidy and Crop Plants. Am. J. Bot. 101 (10), 1711–1725. doi:10.3732/ajb.1400119
Schmittgen, T. D., and Livak, K. J. (2008). Analyzing real-time PCR data by the comparative C(T) method. Nat. Protoc. 3 (6), 1101 1108. doi:10.1038/nprot.2008.73
Shan, C.-M., Shangguan, X.-X., Zhao, B., Zhang, X.-F., Chao, L.-m., Yang, C.-Q., et al. (2014). Control of Cotton Fibre Elongation by a Homeodomain Transcription Factor GhHOX3. Nat. Commun. 5, 5519. doi:10.1038/ncomms6519
Shangguan, X.-X., Xu, B., Yu, Z.-X., Wang, L.-J., and Chen, X.-Y. (2008). Promoter of a Cotton Fibre MYB Gene Functional in Trichomes of Arabidopsis and Glandular Trichomes of Tobacco. J. Exp. Bot. 59 (13), 3533–3542. doi:10.1093/jxb/ern204
Shannon, P., Markiel, A., Ozier, O., Baliga, N. S., Wang, J. T., Ramage, D., et al. (2003). Cytoscape: A Software Environment for Integrated Models of Biomolecular Interaction Networks. Genome Res. 13 (11), 2498–2504. doi:10.1101/gr.1239303
Sudmant, P. H., Kitzman, J. O., Antonacci, F., Alkan, C., Malig, M., Tsalenko, A., et al. (2010). Diversity of Human Copy Number Variation and Multicopy Genes. Science 330 (6004), 641–646. doi:10.1126/science.1197005
Sun, G., and Komatsuda, T. (2010). Origin of the Y Genome in Elymus and its Relationship to Other Genomes in Triticeae Based on Evidence from Elongation Factor G (EF-G) Gene Sequences. Mol. Phylogenetics Evol. 56 (2), 727–733. doi:10.1016/j.ympev.2010.03.037
Tang, M., Chen, Z., Grover, C. E., Wang, Y., Li, S., Liu, G., et al. (2015). Rapid Evolutionary Divergence of Gossypium barbadense and G. Hirsutum Mitochondrial Genomes. BMC Genomics 16, 770. doi:10.1186/s12864-015-1988-0
Tamura, K., Dudley, J., Nei, M., and Kumar, S. (2007). MEGA4: Molecular Evolutionary Genetics Analysis (MEGA) software version 4.0. Mol. Biol. Evol. 24 (8), 1596–1599. doi:10.1093/molbev/msm092
Udall, J. A., Long, E., Ramaraj, T., Conover, J. L., Yuan, D., Grover, C. E., et al. (2019). The Genome Sequence of Gossypioides Kirkii Illustrates a Descending Dysploidy in Plants. Front. Plant Sci. 10, 1541. doi:10.3389/fpls.2019.01541
Van Dingenen, J. (2022). CPR5 Modulates Plant Immunity via RNA Processing. Plant Cell 34, 1437–1438. doi:10.1093/plcell/koac060
Walford, S.-A., Wu, Y., Llewellyn, D. J., and Dennis, E. S. (2012). Epidermal Cell Differentiation in Cotton Mediated by the Homeodomain Leucine Zipper Gene, GhHD-1. Plant J. 71 (3), no. doi:10.1111/j.1365-313X.2012.05003.x
Wan, Q., Zhang, H., Ye, W., Wu, H., and Zhang, T. (2014). Genome-Wide Transcriptome Profiling Revealed Cotton Fuzz Fiber Development Having a Similar Molecular Model as Arabidopsis Trichome. Plos One 9 (5), e97313. ARTN e97313. doi:10.1371/journal.pone.0097313
Wang, G., Zhao, G.-H., Jia, Y.-H., and Du, X.-M. (2013). Identification and Characterization of Cotton Genes Involved in Fuzz-Fiber Development. J. Integr. Plant Biol. 55 (7), 619–630. doi:10.1111/jipb.12072
Wang, M., Sun, R., Li, C., Wang, Q., and Zhang, B. (2017). MicroRNA Expression Profiles during Cotton (Gossypium Hirsutum L) Fiber Early Development. Sci. Rep. 7, 44454. doi:10.1038/srep44454
Wang, N., Liu, W., Zhang, T., Jiang, S., Xu, H., Wang, Y., et al. (2018). Transcriptomic Analysis of Red-Fleshed Apples Reveals the Novel Role of MdWRKY11 in Flavonoid and Anthocyanin Biosynthesis. J. Agric. Food Chem. 66 (27), 7076–7086. doi:10.1021/acs.jafc.8b01273
Wang, S., Wang, J.-W., Yu, N., Li, C.-H., Luo, B., Gou, J.-Y., et al. (2004). Control of Plant Trichome Development by a Cotton Fiber MYB Gene. Plant Cell 16 (9), 2323–2334. doi:10.1105/tpc.104.024844
Wang, F., Park, Y.-L., and Gutensohn, M. (2021). Glandular Trichome-Derived Mono- and Sesquiterpenes of Tomato Have Contrasting Roles in the Interaction with the Potato Aphid Macrosiphum euphorbiae. J. Chem. Ecol. 47 (2), 204–214. doi:10.1007/s10886-021-01243-4
Wang, X., Shen, C., Meng, P., Tan, G., and Lv, L. (2021). Analysis and Review of Trichomes in Plants. BMC Plant Bio 21 (1), 1–11. doi:10.1186/s12870-021-02840-x
Wei, F., Zhang, Y., Shi, Y., Feng, H., Zhao, L., Feng, Z., et al. (2019). Evaluation of the Biocontrol Potential of Endophytic Fungus Fusarium Solani CEF559 against Verticillium dahliae in Cotton Plant. BioMed Res. Int. 2019, 1–12. doi:10.1155/2019/3187943
Xue, X.-Y., Zhao, B., Chao, L.-M., Chen, D.-Y., Cui, W.-R., Mao, Y.-B., et al. (2014). Interaction between Two Timing microRNAs Controls Trichome Distribution in Arabidopsis. PLoS Genet. 10 (4), e1004266. doi:10.1371/journal.pgen.1004266
Yang, C., and Ye, Z. (2013). Trichomes as Models for Studying Plant Cell Differentiation. Cell. Mol. Life Sci. 70 (11), 1937–1948. doi:10.1007/s00018-012-1147-6
Yang, X., Wang, J., Xia, X., Zhang, Z., He, J., Nong, B., et al. (2021). OsTTG1 , a WD40 Repeat Gene, Regulates Anthocyanin Biosynthesis in Rice. Plant J. 107 (1), 198–214. doi:10.1111/tpj.15285
Yang, Y., Yang, S., Guo, J., Cui, Y., Tang, B., Li, X.-J., et al. (2017). Synergistic Toxicity of Polyglutamine-Expanded TATA-Binding Protein in Glia and Neuronal Cells: Therapeutic Implications for Spinocerebellar Ataxia 17. J. Neurosci. 37 (38), 9101–9115. doi:10.1523/JNEUROSCI.0111-17.2017
Yi, D., Alvim Kamei, C. L., Cools, T., Vanderauwera, S., Takahashi, N., Okushima, Y., et al. (2014). TheArabidopsisSIAMESE-RELATED Cyclin-Dependent Kinase Inhibitors SMR5 and SMR7 Regulate the DNA Damage Checkpoint in Response to Reactive Oxygen Species. Plant Cell 26 (1), 296–309. doi:10.1105/tpc.113.118943
Yonekura-Sakakibara, K., and Saito, K. (2013). Transcriptome coexpression analysis using ATTED-II for integrated transcriptomic/metabolomic analysis. Methods Mol Biol. 1011 (6), 317 326. doi:10.1007/978-1-62703-414-2_25
Yoshida, S., Ito, M., Nishida, I., and Watanabe, A. (2002). Identification of a Novel Gene HYS1/CPR5 that Has a Repressive Role in the Induction of Leaf Senescence and Pathogen-Defence Responses in Arabidopsis thaliana. Plant J. 29 (4), 427–437. doi:10.1046/j.0960-7412.2001.01228.x
Yu, Y.-H., Li, X.-F., Yang, S.-D., Li, S.-Q., Meng, X.-X., Liu, H.-N., et al. (2021). Overexpression of VvPPR1, a DYW-type PPR Protein in Grape, Affects the Phenotype of Arabidopsis thaliana Leaves. Plant Physiol. Biochem. 164, 195–204. doi:10.1016/j.plaphy.2021.04.027
Yuan, F., Leng, B., Zhang, H., Wang, X., Han, G., and Wang, B. (2019). A WD40-Repeat Protein from the Recretohalophyte Limonium Bicolor Enhances Trichome Formation and Salt Tolerance in Arabidopsis. Front. Plant Sci. 10, 1456. doi:10.3389/fpls.2019.01456
Yuan, Y., Xu, X., Luo, Y., Gong, Z., Hu, X., Wu, M., et al. (2021). R2R3 MYB‐dependent Auxin Signalling Regulates Trichome Formation, and Increased Trichome Density Confers Spider Mite Tolerance on Tomato. Plant Biotechnol. J. 19 (1), 138–152. doi:10.1111/pbi.13448
Zhang, B., Wang, Q., Wang, K., Pan, X., Liu, F., Guo, T., et al. (2007). Identification of Cotton microRNAs and Their Targets. Gene 397 (1-2), 26–37. doi:10.1016/j.gene.2007.03.020
Zhang, F., Zuo, K., Zhang, J., Liu, X., Zhang, L., Sun, X., et al. (2010). An L1 Box Binding Protein, GbML1, Interacts with GbMYB25 to Control Cotton Fibre Development. J. Exp. Bot. 61 (13), 3599–3613. doi:10.1093/jxb/erq173
Zhang, H., Li, W., Niu, D., Wang, Z., Yan, X., Yang, X., et al. (2019). Tobacco Transcription Repressors NtJAZ: Potential Involvement in Abiotic Stress Response and Glandular Trichome Induction. Plant Physiol. Biochem. 141, 388–397. doi:10.1016/j.plaphy.2019.06.021
Zhang, X., Dyachok, J., Krishnakumar, S., Smith, L. G., and Oppenheimer, D. G. (2005). IRREGULAR TRICHOME BRANCH1 in Arabidopsis Encodes a Plant Homolog of the Actin-Related Protein2/3 Complex Activator Scar/WAVE that Regulates Actin and Microtubule Organization. Plant Cell 17 (8), 2314–2326. doi:10.1105/tpc.104.028670
Zhang, Y., He, P., Yang, Z., Huang, G., Wang, L., Pang, C., et al. (2017). A Genome-Scale Analysis of the PIN Gene Family Reveals its Functions in Cotton Fiber Development. Front. Plant Sci. 8, 461. doi:10.3389/fpls.2017.00461
Zhao, B., Cao, J.-F., Hu, G.-J., Chen, Z.-W., Wang, L.-Y., Shangguan, X.-X., et al. (2018). Corecis-element Variation Confers Subgenome-Biased Expression of a Transcription Factor that Functions in Cotton Fiber Elongation. New Phytol. 218 (3), 1061–1075. doi:10.1111/nph.15063
Zhao, L., Gao, L., Wang, H., Chen, X., Wang, Y., Yang, H., et al. (2013). The R2R3-MYB, bHLH, WD40, and Related Transcription Factors in Flavonoid Biosynthesis. Funct. Integr. Genomics. 13 (1), 75–98. doi:10.1007/s10142-012-0301-4
Zhao, M., Li, J., Zhu, L., Chang, P., Li, L., and Zhang, L. (2019). Identification and Characterization of MYB-bHLH-WD40 Regulatory Complex Members Controlling Anthocyanidin Biosynthesis in Blueberry Fruits Development. Genes 10 (7), 496. doi:10.3390/genes10070496
Zhao, Y., Jing, H., Zhao, P., Chen, W., Li, X., Sang, X., et al. (2021). GhTBL34 is associated with verticillium wilt resistance in cotton. Int. J. Mol. Sci. 22 (17). doi:10.3390/ijms22179115
Zhou, Z., An, L., Sun, L., Zhu, S., Xi, W., Broun, P., et al. (2011). Zinc Finger Protein5 Is Required for the Control of Trichome Initiation by Acting Upstream of Zinc Finger Protein8 in Arabidopsis. Plant Physiol. 157 (2), 673–682. doi:10.1104/pp.111.180281
Keywords: cotton, CPR5 genes, co-expression network analysis, gene expression, trichome development
Citation: Wang H, Umer MJ, Liu F, Cai X, Zheng J, Xu Y, Hou Y and Zhou Z (2022) Genome-Wide Identification and Characterization of CPR5 Genes in Gossypium Reveals Their Potential Role in Trichome Development. Front. Genet. 13:921096. doi: 10.3389/fgene.2022.921096
Received: 15 April 2022; Accepted: 10 May 2022;
Published: 08 June 2022.
Edited by:
Ali Raza, Fujian Agriculture and Forestry University, ChinaReviewed by:
Sajid Fiaz, The University of Haripur, PakistanCopyright © 2022 Wang, Umer, Liu, Cai, Zheng, Xu, Hou and Zhou. This is an open-access article distributed under the terms of the Creative Commons Attribution License (CC BY). The use, distribution or reproduction in other forums is permitted, provided the original author(s) and the copyright owner(s) are credited and that the original publication in this journal is cited, in accordance with accepted academic practice. No use, distribution or reproduction is permitted which does not comply with these terms.
*Correspondence: Yanchao Xu, eHV5YW5jaGFvMjAxNkAxNjMuY29t; Yuqing Hou, aG91eXVxaW5nMThAMTYzLmNvbQ==; Zhongli Zhou, emhvdXpsQGNyaWNhYXMuY29tLmNu
†These authors have contributed equally to this work
Disclaimer: All claims expressed in this article are solely those of the authors and do not necessarily represent those of their affiliated organizations, or those of the publisher, the editors and the reviewers. Any product that may be evaluated in this article or claim that may be made by its manufacturer is not guaranteed or endorsed by the publisher.
Research integrity at Frontiers
Learn more about the work of our research integrity team to safeguard the quality of each article we publish.