- Department of Hand and Foot Surgery, Jilin Provincial People’s Hospital, Changchun, China
Exosomes are nano-extracellular vesicles secreted by a variety of cells. They are composed of a double-layer membrane that can transport a variety of proteins, coding and non-coding genes, and bioactive substances. Exosomes participate in information transmission between cells and regulate processes such as cell proliferation, migration, angiogenesis, and phenotypic transformation. They have broad prospects in the occurrence, development, and treatment of many diseases including orthopedics. Exosomes derived from different types of bone cells such as mesenchymal stem cells, osteoblasts, osteoclasts, and their precursors are recognized to play pivotal roles in bone remodeling processes including osteogenesis, osteoclastogenesis, and angiogenesis. This articlesummarizes the characteristics of exosomes and their research progress in bone remodeling, bone tumors, vascular skeletal muscle injury, spinal cord injury, degenerative disc diseases, cartilage degeneration, osteoarthritis, necrosis of the femoral head, and osteoporosis.
Introduction
Exosomes originate from the invagination of the cell membrane and form multivesicular bodies, which then gradually mature into endosomes. After fusion with the cell membrane, exosomes are actively secreted outside the cells (Cruz and Rocco, 2020). The exosome is usually a lipid bilayer vesicle with a diameter of about 30–150 nm (Batsali et al., 2020; Liu et al., 2020). Its double-layered structure can effectively protect the content of the exosomes, including a variety of proteins, nucleic acids, and lipids, thereby participating in the information transmission between cells (Figure 1). It further regulates cell proliferation (Rezaie, et al., 2019), migration, autophagy (Hassanpour et al., 2020), neovascularization, and phenotypic transformation (He et al., 2018). The type of cells and the environment determines the composition and secretion of exosomes (Table 1). Under stress conditions or stimuli, the secretion of exosomes may rise sharply, and the bioactive substances will vary based on the type of stress. Studies have highlighted the variation in exosomal content based on environmental cues, which aid in their roles in different target cells (Xunian and Kalluri, 2020).
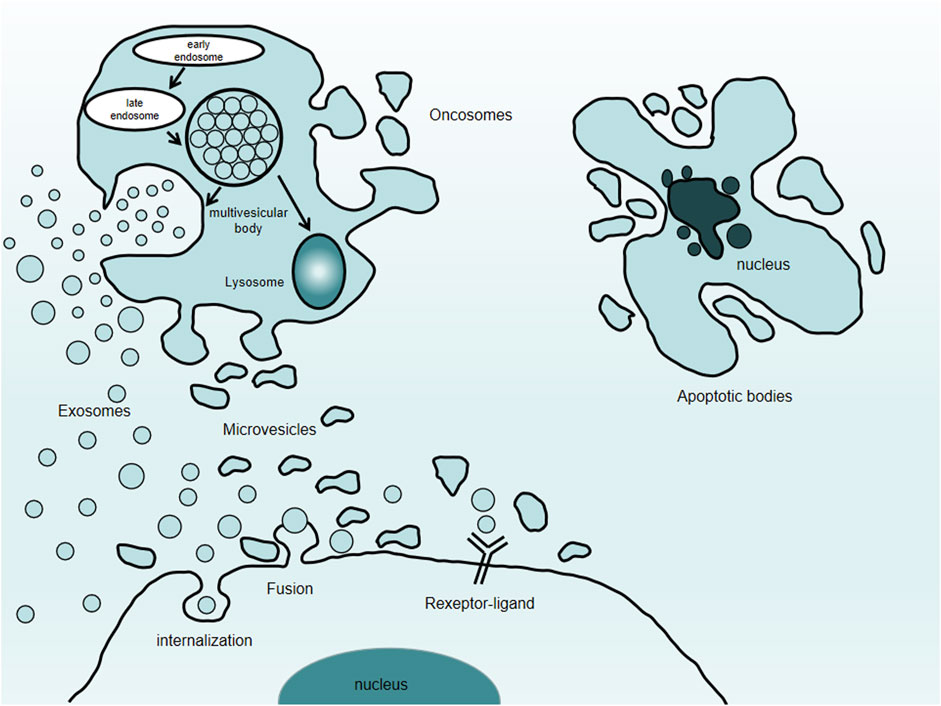
FIGURE 1. Types of extracellular vesicles. Extracellular vesicles comprise exosomes, microvesicles, oncosomes, and apoptotic bodies.
Review of exosomes
Almost all types of alive cells can produce exosomes. There are some differences in the exosomes from different sources in terms of yield, contents, function, and drug loading. For example, mesenchymal stem cells (MSC) exosomes participate in the process of tissue repair and injury and have the potential to act as drug carriers. Macrophage-derived exosomes can affect the tissue microenvironment by regulating immune function. It also has specific surface proteins and tends to accumulate in the cancer cells (Zhang et al., 2020).
The traditional exosome purification involves collecting the cell culture medium for gradient centrifugation. Generally, the supernatant after low-speed centrifugation (300 × g) is taken. At this step, the cells and debris are removed, and the supernatant after further centrifugation cycles, discarding cell fragments (2000 g) and large vesicles (10,000 × g), is collected. This supernatant is subjected to ultracentrifugation (100,000 × g) to obtain the exosomes (Zhang J. et al., 2015). For further purification, exosomes are washed in phosphate-buffered saline, subjected to another ultracentrifugation cycle, collected, and stored at −80°C. This method is widely used for the purification of exosomes from various biological samples such as cell culture medium, urine, and cerebrospinal fluid. However, the use of ultracentrifugation is time-consuming, provides a limited number of purification, and has low efficiency, which limits its wide application in exosome purification. In recent years, ultrafiltration, precipitation, immunoassay, and rapid purification kit of exosomes have been gradually introduced. However, they all have low efficiency with impurities in the exosomal preparation, making them difficult to be popularized. At present, there is no consensus on the separation methods of exosomes, and different purification methods lead to changes in exosomal proteins and bioactive substances contained therein (Liu et al., 2021). Exosomes cannot be stored for a long time after purification from a cell culture medium. The protection techniques mainly include cryopreservation, freeze-drying, and spray-drying (Zhang et al., 2020).
The detection of exosomes was mainly through the assessment of marker proteins and the observation of particle size and morphology of exosomes under the transmission electron microscope. These can be used as markers to detect exosomes from different sources. These proteins include membrane transport fusion protein, heat shock protein, CD9, CD63, and CD81. Protein electrophoresis is one of the methods used to confirm whether the substances extracted from extracellular body fluid and culture medium are exosomes. Combined with the observations of the transmission electron microscope, the exosome purification is confirmed when the extracted substances have a double-layer membrane and cup-shaped morphology, with a size of about 30–150 nm. Nanoparticle tracking analysis (NTA) is a type of optical particle tracking approach that can calculate the concentration and size of the exosomes based on the Brownian motion. The velocity of the particles is analyzed through their movement in liquid, and finally, the particle size and concentration are calculated.
The role of exosomes in bone remodeling
Osteoporosis, rheumatoid arthritis, and osteoarthritis (OA) are the most common and complex age-related bone diseases in the world (Sućur et al., 2014). The imbalance between osteoclast bone resorption and osteoblast bone formation leads to bone remodeling imbalance (Sućur et al., 2014) and bone microstructure changes, resulting in bone loss and increased risk of bone fragility and fracture. Bone remodeling is a continuous process of repairing bone microstructure damage and aging bone tissue (Withrow et al., 2016). In the bone microenvironment, the cells involved in bone remodeling regulate the reconstruction process by secreting cytokines (Behera and Tyagi, 2018). The role of exosomes in bone remodeling has attracted attention in recent years. Studies have shown that they play an important role in the communication between the bone microenvironment and the cells. Exosomes released by bone marrow mesenchymal stem cells (BM-MSCs) are involved in bone remodeling, especially in the differentiation of osteoclasts and osteoblasts by transferring bioactive molecules to target cells (Xie et al., 2017). Studies show that MSC-derived exosome microRNA (miRNA) is differentially expressed during the osteogenic differentiation of human BM-MSCs. Exosomes derived from human BM-MSCs have the ability to promote bone regeneration (Martins et al., 2016). Cui et al. (2016) showed that the exosomes of MC3T3-E1 cells can promote the differentiation of bone marrow stromal cells (BMSCs) into osteoblasts. The exosomes inhibit the expression and increase of AXIN1 levels by affecting the miRNA of recipient cells' β-catenin expression, thus activating the Wnt pathway. Other studies confirmed that the exosomes produced by cyc454 cells pretreated with myostatin can be absorbed by osteoblast MC3T3 cells. Here, they significantly reduce the key regulator of osteoblast differentiation Runx2 and reduce osteoblast differentiation by downregulating the Wnt signal pathway. More importantly, this inhibition is regulated by the expression of miRNA carried by exosomes. It is proposed that myostatin inhibits osteoblast differentiation by inhibiting the secretion of exosomal miRNA derived from osteoblasts (Qin et al., 2017). Furthermore, it was found that exosomes derived from C2C12 myoblasts can enter pre-osteoblast MC3T3-E1 and promote osteogenic differentiation through miRNA and other substances carried by them (Xu et al., 2018). Here, miR-27a-3p is the main component of exosome osteogenesis. It changed the expression of antigen-presenting cells and activated the Wnt pathway in MC3T3-E1 cells. This study provides a new perspective to understand musculoskeletal interaction and puts forward the possibility of myogenic exosomes as an indicator of abnormal bone remodeling. Qin et al. (2016a)found that exosomes secreted by BM-MSCs can stimulate osteoblast differentiation, but have no significant effect on the proliferation of human osteoblasts cultured in vitro. In the exosomes derived from BM-MSCs, three highly enriched osteogenic miRNAs, miR-196a, miR-27a, and miR-206, were found, which may be involved in the exosomes derived from stem cells.
Exosomes have the function of secreting and promoting bone regeneration (Wang and Thomsen, 2021). Li et al. (2016) found that the serum exosome miR-214-3p increases significantly in elderly fracture patients. Further research confirmed that miR-214-3p was enriched in exosomes derived from osteoclasts, which can not only inhibit the role of osteoclasts, but also inhibit the formation of osteoblasts. MSCs are widely used in tissue repair and regeneration. However, limited survival after MSC transplantation hinders the use of MSC in tissue repair and regeneration. The exosomes from adipose tissue-derived mesenchymal stem cells can promote the proliferation and differentiation of primitive osteoblasts (Lu Z. et al., 2017). When adipose tissue-derived mesenchymal stem cells are treated with tumor necrosis factor, the content of Wnt-3a in their exosomes increases, which inhibits the signal of Wnt and promotes the expression of osteogenic genes.
In the process of bone regeneration, neovascularization can not only serve as a source of oxygen and nutrition but also provide calcium and phosphate to help mineralization. Although the functions of exosomes during bone angiogenesis remain unclear, the role of exosomes in stimulating angiogenesis of other tissues and organs has been studied. In vitro experiments have shown that placental MSC exosomes can promote endothelial cell proliferation, migration, and tubule formation (Salomon et al., 2013). In addition, exosomes also increase endothelial cell migration by transporting functional enzymes (such as nicotinamide adenine dinucleotide oxidase). Different miR-129 (Vrijsen et al., 2010), miR-136 (Sahoo et al., 2011), and miR-17–92 clusters (Salomon et al., 2013) in exosomes have been shown to regulate endothelial cell proliferation and angiogenesis. Exosomes not only showed angiogenesis in vitro, but also had positive results in vivo. Studies have shown that MSC-derived exosomes have successfully improved angiogenesis in different animal models. For example, caudal vein injection of MSC-derived exosomes reduced myocardial ischemia/reperfusion injury and improved angiogenesis of the ischemic heart (Bian et al., 2014). Exosomes from human umbilical cord MSC can increase blood perfusion of local tissues (Zhang et al., 2012). Additionally, in the study of tissue engineering, researchers incorporated exosomes from Induced pluripotent stem cells into β-tricalcium phosphate scaffolds. These not only have positive effects on MSC migration, proliferation, and differentiation in vitro, but also promote bone regeneration by increasing angiogenesis in vivo (Qi et al., 2016). Exosome secretion significantly promotes angiogenesis, which stimulates bone growth and regeneration. Further exploration of the role of exosomes in angiogenesis will help to develop new bone regeneration treatments.
The role of exosomes in bone tumors
Recent studies found that exosomes are related to tumor progression and metastasis (Weidle et al., 2017). miRNA in exosomes (Table 2) is transmitted as a signal molecule to its target cells by changing information to achieve cell-to-cell communication (Rezaie et al., 2021). Therefore, miRNA can be used as both tumor suppressor and tumor promoter. In excess amounts, it will interfere with the normal cell cycle, affect apoptosis, and promote cell proliferation. Tumor cell invasion and angiogenesis are related to tumor formation and progression. Concurrently, exosomes play an important role in bone development. These functions make exosomes a useful tool for the diagnosis and prognosis of bone tumors (biomarker potential). Osteosarcoma is a common malignant bone tumor in adolescents. The increase in miR-18 levels in exosomes of metastatic osteosarcoma promotes the migration and invasion of non-malignant fibroblasts in vitro. The secretion of miR-675 in exosomes from tumor serum samples increases and inhibits the expression of c-troponin 1 in receptor cells, which is related to the metastatic phenotype of osteosarcoma patients. Therefore, miR-675 in exosomes can be used as a biomarker to monitor the metastasis of osteosarcoma (Gong et al., 2018).
Most tumors such as breast, lung, and prostate cancer could metastasize to the bone. Xu Z. et al. (2018) found that miR-21 in exosomes derived from lung adenocarcinoma cells may be involved in osteoclast formation. Karlsson et al. (2016) reported that exosomes derived from mouse prostate cancer cell line trap-c1l impair the proliferation and differentiation of osteoclasts by affecting the expression of dendritic cell-specific transmembrane proteins, tartrate-resistant acid phosphatase, cathepsin K, and matrix metalloproteinase-9. The levels of exosomal miR-141-3p were found to increase in the serum of patients with prostate cancer (Ye et al., 2017). After a series of in vivo and in vitro experiments, it was proposed that prostate cancer can promote bone metastasis by secreting exosomal miR-141-3p to regulate osteoblast activity.
The role of exosomes in vascular skeletal muscle injury
Orthopedic diseases are often accompanied by vascular skeletal muscle injury. The success of bone remodeling is closely related to vascular regeneration. Many studies have found that vascular and skeletal muscle regeneration is closely related to exosomes (Qin et al., 2016b). In this regard, miR-494 was found to be abundant in exosomes derived from BM-MSCs (Nakamura et al., 2015). miR-494 participates in C2C12 myogenesis and endothelial cell migration and can promote skeletal muscle and vascular regeneration. There are a large number of angiogenic factors (such as vascular endothelial growth factor and interleukin-6) in exosomes derived from BM-MSCs, which contribute to skeletal muscle regeneration (Behera and Tyagi, 2018b). Although the specific mechanism of exosomes and their miRNAs for vascular muscle injury is not clear, the therapeutic potential of exosomes has attracted the attention of many researchers.
The role of exosomes in spinal cord injury
When the spinal cord is injured under physiological conditions, immune cells cannot pass through the blood–cerebrospinal fluid barrier to the spinal cord. Mechanical force directly destroys the nerve tissue and endothelial cell membrane, where immune cells accumulate and penetrate into the lesion site to promote an immune response. The inflammatory response is the most important pathological process and plays an important role in the prognosis of spinal cord injury. Owing to the high incidence rate, complicated cases, and unclear pathogenesis, the treatment of spinal cord injury is a great challenge. Recently, exosomes derived from human umbilical cord mesenchymal stem cells has been shown to effectively trigger bone marrow-derived macrophages to inhibit inflammatory response by downregulating the inflammatory cytokines (Kang and Guo, 2022). Human umbilical cord mesenchymal stem cell-derived exosomes can also reduce inflammation and promote the healing of spinal cord injury, providing a new perspective and treatment strategy for spinal cord recovery (Kang and Guo, 2022).
The role of exosomes in the degenerative disc diseases
Lumbar disc degeneration is the main cause of lumbar disc herniation. Serious degenerative diseases can cause lower back and leg pain and even nerve damage, affecting the ability to work and, consequently, the quality of life. The use of BM-MSCs can differentiate and supplement intervertebral disc cells, promote the proliferation of nucleus pulposus cells, reduce apoptosis, resist inflammation, and have a therapeutic effect on the degradation of the intervertebral disc. However, in clinical application, it is limited by the physiological barrier and long-term survival of cells. Exosomes were reported to play an important role in the treatment of lumbar degenerative diseases by stem cells (Lu K. et al., 2017). Compared with BM-MSCs, exosomes are more stable and easier to store and can survive in a harsh environment, thereby providing a promising treatment for lumbar disc degenerative diseases. Studies showed that miR142-3p in BMSC-derived exosomes could reduce interleukin-1β-induced inflammatory cytokine secretion by targeting mixed lineage kinase 3 (Zhu L. et al., 2020). In addition, mounts of miR-532-5p in BMSC-derived exosomes could inhibit inflammation by targeting RASSF5 (Zhu G. et al., 2020). A vitro study showed that exosomes could result in more than a 50% increase in cell proliferation and a decrease in cellular apoptosis in 3D human degenerative disc cell cultures (Hingert et al., 2020).
The role of exosomes in cartilage degeneration
Degenerative injury of articular cartilage and reactive hyperplasia of subchondral bone led to clinical manifestations such as joint pain, stiffness, and joint swelling. Some miRNAs secreted by exosomes can inhibit chondrocyte inflammation and promote cartilage development, thus inhibiting cartilage degeneration. They have been shown to play pivotal roles in the treatment of arthritis (Sun et al., 2019). In this regard, miR-320c in exosomes could promote the differentiation of BM-MSCs into chondrocytes. Exosomes derived from mouse BM-MSCs were used to stimulate macrophage polarization to develop into an anti-inflammatory phenotype, thereby maintaining chondrocyte homeostasis and protecting the chondrocytes (Ke and Zhu, 2020). Up to now, miRNA-95-5p (Mao et al., 2018a), and miRNA-100-5p (Wu et al., 2019) were also reported to involve in maintaining bone homeostasis during cartilage degeneration. Currently, the most ideal cell source of exosomes for promoting cartilage regeneration remains ambiguous. miR-140 was loaded into exosomes through electroporation, which was more effective in suppressing the progression of cartilage degeneration and in enhancing cartilage regeneration (Liang et al., 2020). miR-135b in exosomes secreted by transforming growth factor-β1-stimulated MSCs could downregulate Sp1 protein expression, leading to better cartilage regeneration in rats with OA (Wang et al., 2018).
The role of exosomes in osteoarthritis
OA is one of the most common arthritis. Its pathological features include cartilage degeneration, subchondral bone sclerosis, osteophyte formation, synovitis, and ligament calcification. At present, the main clinical treatment of OA includes oral non-steroidal anti-inflammatory drugs; hence, scholars are still looking for new treatment strategies. Previous studies have focused on stem cell therapy, such as intra-articular MSCs, which can prevent cartilage degeneration and delay the process of osteoarthritis. Subsequent studies have inferred that the therapeutic function of stem cells comes from its paracrine effect, including its secreted exosomes and particles. Tao et al. (2017a) found that the secretion from synovial mesenchymal stem cells (SMSC) contains Wnt5a and Wnt5b which can activate the Yes-related protein signal transduction pathway, thereby promoting the proliferation and migration of chondrocytes. However, activation of the Yes-related protein signal transduction pathway by Wnt5a and Wnt5b can inhibit the expression of Sox9 and significantly reduce the secretion of the extracellular matrix of chondrocytes. The modified SMSC-derived exosomes with high expression levels of miR-140-5p can eliminate this deficiency, promote the proliferation and migration of articular chondrocytes without affecting the secretion of the extracellular matrix of chondrocytes, and prevent the occurrence of OA in rats. Wnt5a is an atypical Wnt protein. The development of joints, including cartilage, bone, and joint cavity, is highly dependent on the Wnt signal transduction pathway (Ge et al., 2009). Wnt5a has dual functions: in the early stage of cartilage formation, it can activate chondrocyte proliferation and inhibit chondrocyte differentiation, whereas, in the late stage, it can activate matrix metalloproteinase and reduce cartilage formation and extracellular matrix synthesis in mature chondrocytes. BM-MSC-derived exosomes with high expression levels of miR-92a-3p have been reported to target the 3′-untranslated sequence of wnt5a mRNA, inhibit Wnt5a activity, and inhibit cartilage degradation with homeostasis regulating in OA mice (Mao et al., 2018b). In this study, the biological effect of Wnt5a is similar to the results of SMSC-derived exosomes reported by Tao et al. (2017b). The difference between these studies is that the latter inhibits the negative effect of Wnt5a on cartilage degradation through miR-140-5p and retains the protective effect of Wnt5b on cartilage. Human BM-MSC-derived exosomes also contain long-chain noncoding RNA klf3-as1, which can bind to the miR-206 gene as an endogenous competitive RNA inhibitor, affecting the regulation of the miR-206 gene and further improving the expression of G protein–coupled receptor kinase interacting protein-1 (GIT1) (Liu et al., 2018a). Studies have shown that GIT1 can promote chondrocyte proliferation and inhibit chondrocyte apoptosis (Zhang LQ. et al., 2015). Platelet-derived growth factor is shown to promote chondrocyte proliferation and inhibits chondrocyte apoptosis by upregulating GIT1 expression levels (Zhao et al., 2016). Overexpression of miR-206 and knockout of Git1 can reverse the therapeutic effect of BM-MSC-derived exosomes on OA, indicating that these exosomes regulate miR-206/git-1-mediated chondrocyte proliferation and apoptosis and promote cartilage repair through klf3-as1 (Liu et al., 2018b). Furthermore, studies have compared the therapeutic effects of BM-MSC-derived exosomes and particles on OA. The results show that they have similar functions and can reconstruct chondrocyte homeostasis, inhibit chondrocyte apoptosis, and stimulate macrophages to polarization toward an anti-inflammatory phenotype, thereby inhibiting cartilage degradation and blocking OA in mice (Cosenza et al., 2018).
The role of exosomes in necrosis of the femoral head
Glucocorticoid-induced femoral head necrosis is a progressive and disabling joint disease, which is often caused by long-term oral steroids. Apoptosis of BMSCs, osteoblasts, and vascular endothelial cells and the increased differentiation of BMSCs into adipocytes and deformity of bone formation play a key role in this pathological process. Therefore, early prevention of BMSC apoptosis, promotion of BMSC proliferation, and prevention of BMSC differentiation into adipocytes may prevent the progression of glucocorticoid-induced femoral head necrosis. Guo et al. (2016) showed that SMSC-derived exosomes post intravenous injection-induced osteonecrosis of the femoral head in the treated rats. The results indicated that the subchondral bone trabeculae of the femoral head in the treatment group were complete and evenly distributed. Only 20% of the rats had very slight changes in osteonecrosis. The thickness, bone volume (tissue volume), and the number of bone trabeculae in the control group were significantly reduced, resulting in obvious osteonecrosis. The cancellous bone of the femoral head was sparse or even disappeared. This suggests that promoting the proliferation and inhibiting the apoptosis of BMSCs induced by glucocorticoids may be the potential mechanism of SMSC-derived exosomes to prevent femoral head necrosis in rats. Many studies applied various types of exosomes, including mutant hypoxia-inducible factor-1α modified BMSC-derived (Li et al., 2017), multifunctional stem cell-derived (Liu et al., 2017), platelet-rich plasma-derived (Tao et al., 2017a), and BMSC-derived exosomes (Fang et al., 2018), to achieve a better outcome in treating glucocorticoid-induced femoral head necrosis. The principle is to: 1) promote the proliferation of BMSC, osteoblasts, and vascular endothelial cells; 2) promote the osteogenic differentiation of BMSC and angiogenic differentiation of endothelial progenitor cells; 3) resist the apoptosis caused by high glucocorticoid; and 4) activate the cellular protein kinase B/Bad/Bcl-2 and phosphatidylinositol 3-kinase/protein kinase B signal transduction pathway. These different exosomes are effectively prevented by promoting angiogenesis and osteogenesis and the progress of glucocorticoid-induced femoral head necrosis.
The role of exosomes in osteoporosis
As people get older, the osteogenic directional differentiation ability of BMSCs decreases, which is accompanied by the enhancement of adipocyte differentiation tendency. This imbalance can lead to the decline of osteogenic ability and the accumulation of bone marrow adipose tissue, which is considered to be the pathophysiological mechanism of OP (Deng et al., 2021). Studies have shown that BMSCs exosomes can inhibit adipogenic differentiation while promoting osteogenic differentiation and vascularization, which reverses the imbalance between osteogenic and adipogenic conversion (Chen et al., 2017). The Wnt/β- Catenin pathway is an important signal mechanism for inhibiting adipogenic gene expression and restoring osteoblast proliferation and differentiation. Zhao, et al. (2018) co-culture BMSCs exosomes with osteoblasts, and find that the expression of proteins related to the mitogen-activated protein kinase (MAPK) signal pathway increased, and the proportion of the S phase of the cell cycle increased significantly, indicating that the BMSCs exosomes can promote osteoblast proliferation by activating MAPK signal pathway. A recent study showed the differential expression of microRNA in exosomes during osteogenic differentiation in the process of bone metabolism regulation. The expression of miR-218, let-7a, miR-135b, miR-148a, miR-299-5p, miR-219, miR-135b, miR-302b, and miR-299-5p is significantly up-regulated, while the expression of miR-155, miR-320c, miR-885-5p, miR-181a, and miR-221 is significantly down-regulated, revealing that miRNA may be a potential target of OP therapy (Xu et al., 2014). Zhang et al. (2021) showed that miR-935-modified bone marrow mesenchymal stem cells-derived exosomes enhance osteoblast proliferation and differentiation in osteoporotic rats by targeting STAT1, which provides new therapeutic targets for the treatment of osteoporosis. Cui et al. (2021) pointed out that the engineered exosomes BT-Exo-siShn3 could alleviate excessive bone resorption, insufficient bone formation, and inadequate vascularization, which provided a promising therapy for the treatment of osteoporosis.
Clinical significance of exosomes
Owing to the special biological characteristics of exosomes, an increasing number of people have begun to study their characteristics and treatment potential (Figure 2). The composition of exosomes varies with the type of cells, culture conditions, and even separation methods (Li et al., 2018). Bone remodeling is an important bone repair process in human life (Dimitriou et al., 2011). This complex process requires the coordinated activities of many cells to ensure that the process of bone remodeling (bone formation and resorption) occurs sequentially (Raggatt and Partridge, 2010). If the remodeling process goes out of balance, it will cause bone loss and bone microstructure damage, resulting in increased bone fragility and fracture, and the occurrence of osteoporosis (Robling and Bonewald, 2020). BM-MSCs and exosomes can regulate osteoblasts and osteoclasts, thereby promoting the healing of new bone, neovascularization, and skeletal muscle formation. Due to the nanoparticle size, lipid bilayer structure, and specific antibody on the surface of exosomes, they can also play a role in cell communication and have transmission characteristics, making them ideal candidates for drug delivery systems in the treatment of orthopedic diseases. Compared with the popular lipid nanoparticles, the main advantage of exosomes is that they cause low toxicity and immunogenicity, pass through various physiological barriers, and maintain the stability of their contents due to their lipid (Hu et al., 2021). Considering the bone-promoting ability of BM-MSC-derived exosomes, it is an attractive idea to develop exosomes as targeted delivery materials for molecular therapy of bone diseases. Osteogenic exosomes can be used to carry osteogenic molecules to improve bone diseases such as osteoporosis and delayed or nonunion of fractures (Yu et al., 2021). Concurrently, exosomes and their miRNAs can be used as markers for the diagnosis and prognosis of destructive bone diseases and the monitoring of orthopedic cancers (Bravo Vázquez et al., 2021). BM-MSCs have broad prospects in bone regeneration and various tissue regeneration. However, due to the limited number of donors and invasive collection process, they cannot be implemented widely in the treatment of OA. With the in-depth study of its stem cells, increasing evidence confirms that the role of stem cells depends on the exosomes (Li et al., 2018). Compared to the treatment with BM-MSCs, exosomes have the following advantages in orthopedic treatment: 1) as exosomes do not express major histocompatibility complex on the cell surface, they can overcome the shortcomings of cell transplantation, have higher safety, and reduce the problem of immunogenicity; 2) they are easier to preserve and transport; 3) they are generally not limited by various physiological barriers and have higher efficiency; and 4) lack moral and ethical restrictions, which makes it easier to apply them on a large scale. Exosomes can effectively avoid the phagocytosis of mononuclear macrophages in the human body. It can be widely distributed without activation of opsonin and coagulation factors. However, weak targeting and susceptibility to being quickly cleared in the body are the main disadvantages of exosomes. Surface modification of exosomes is a way to overcome its poor stability and rapid elimination. Treating exosomal surface proteins as anchoring devices or affinity tags can meet the requirements of the particle surface by certain means.
Bone is another frequent location for tumor metastasis (Rezaie et al., 2022). Communication among bone cells, tumor cells, and the bone matrix leads to the remodeling of the homeostatic bone process and induces sclerotic and/or osteolytic injuries. Examples of some miRNAs contained in exosomes in prostate cancer include miR-409 and miR-141 which are upregulated and can lead to bone metastasis (Rajagopal and Harikumar, 2018). In breast cancer, bone metastases have been associated with the presence of miR-10a and miR-10b (Vičić and Belev, 2021).
Conclusion
At present, the molecular mechanism behind bone remodeling and signal cascade is still unknown. Most exosome research (Table 3) is in the preclinical stage, and the traditional exosome separation and purification methods are not feasible in the clinic. Therefore, one of the biggest challenges in the future is to produce exosomes designed for a wide range of clinical applications, which requires more efficient and accurate qualitative and quantitative acquisition methods of exosomes. Exosomes are more important for the treatment of orthopedic diseases than other gene and cell therapies. Thus, we should pursue research on exosomes, deepen the understanding of exosomes, and find appropriate methods to isolate, purify, and use exosomes to implement them in the diagnosis, monitoring, and treatment of orthopedic diseases.
Data availability statement
The original contributions presented in the study are included in the article/Supplementary Material; further inquiries can be directed to the corresponding author.
Author contributions
CS designed and supervised the study; LZ performed the analysis work; LZ, YL, and XZ organized, designed, and wrote the manuscript. All authors reviewed the final manuscript.
Conflict of interest
The authors declare that the research was conducted in the absence of any commercial or financial relationships that could be construed as a potential conflict of interest.
Publisher’s note
All claims expressed in this article are solely those of the authors and do not necessarily represent those of their affiliated organizations, or those of the publisher, the editors, and the reviewers. Any product that may be evaluated in this article, or claim that may be made by its manufacturer, is not guaranteed or endorsed by the publisher.
Abbreviations
A, osteoarthritis; mscMSCs, mesenchymal stem cells; abrBM-MSCs, bone marrow mesenchymal stem cells; miRNAs, microRNAs; BMSCs, bone marrow stromal cells; SMSC, synovial mesenchymal stem cells; GIT1, G protein coupled receptor kinase interacting protein-1.
References
Batsali, A. K., Georgopoulou, A., Mavroudi, I., Matheakakis, A., Pontikoglou, C. G., Papadaki, H. A., et al. (2020). The role of bone marrow mesenchymal stem cell derived extracellular vesicles (MSC-EVs) in normal and abnormal hematopoiesis and their therapeutic potential. J. Clin. Med. 9 (3), 856. doi:10.3390/jcm9030856
Behera, J., and Tyagi, N. (2018). Exosomes: Mediators of bone diseases, protection, and therapeutics potential. Oncoscience 5 (5-6), 181–195. doi:10.18632/oncoscience.421
Bian, S., Zhang, L., Duan, L., Wang, X., Min, Y., and Yu, H. (2014). Extracellular vesicles derived from human bone marrow mesenchymal stem cells promote angiogenesis in a rat myocardial infarction model.Extracellular vesicles derived from human bone marrow mesenchymal stem cells promote angiogenesis in a rat myocardial infarction model. J. Mol. Med. 92, 387–397. doi:10.1007/s00109-013-1110-5
Bravo Vázquez, L. A., Moreno Becerril, M. Y., Mora Hernández, E. O., Leon Carmona, G. G. d., Aguirre Padilla, M. E., Chakraborty, S., et al. (2021). The emerging role of MicroRNAs in bone diseases and their therapeutic potential. Molecules 27 (1), 211. doi:10.3390/molecules27010211
Chen, C., Wang, D., Moshaverinia, A., Liu, D., Kou, X., Yu, W., et al. (2017). Mesenchymal stem cell transplantation in tight-skin mice identifies miR-151-5p as a therapeutic target for systemic sclerosis. Cell. Res. 27 (4), 559–577. doi:10.1038/cr.2017.11
Cosenza, S., Toupet, K., Maumus, M., Luz-Crawford, P., Blanc-Brude, O., Jorgensen, C., et al. (2018). Mesenchymal stem cells-derived exosomes are more immunosuppressive than microparticles in inflammatory arthritis. Theranostics 8 (5), 1399–1410. doi:10.7150/thno.21072
Cruz, F. F., and Rocco, P. R. M. (2020). The potential of mesenchymal stem cell therapy for chronic lung disease. Expert Rev. Respir. Med. 14 (1), 31–39. doi:10.1080/17476348.2020.1679628
Cui, Y., Guo, Y., Kong, L., Shi, J., Liu, P., Li, R., et al. (2021). A bone-targeted engineered exosome platform delivering siRNA to treat osteoporosis. Bioact. Mat. 10, 207–221. doi:10.1016/j.bioactmat.2021.09.015
Cui, Y., Luan, J., Li, H., Zhou, X., and Han, J. (2016). Exosomes derived from mineralizing osteoblasts promote ST2 cell osteogenic differentiation by alteration of microRNA expression. FEBS Lett. 590 (1), 185–192. doi:10.1002/1873-3468.12024
Deng, P., Yuan, Q., Cheng, Y., Li, J., Liu, Z., Liu, Y., et al. (2021). Loss of KDM4B exacerbates bone-fat imbalance and mesenchymal stromal cell exhaustion in skeletal aging. Cell. Stem Cell. 28 (6), 1057–1073. e7. doi:10.1016/j.stem.2021.01.010
Dimitriou, R., Jones, E., McGonagle, D., and Giannoudis, P. V. (2011). Bone regeneration: Current concepts and future directions. BMC Med. 9, 66. doi:10.1186/1741-7015-9-66
Fang, S., Li, Y., and Chen, P. (2018). Osteogenic effect of bone marrow mesenchymal stem cell-derived exosomes on steroid-induced osteonecrosis of the femoral head. Drug Des. devel. Ther. 13, 45–55. doi:10.2147/DDDT.S178698
Ge, X., Ma, X., Meng, J., Zhang, C., Ma, K., Zhou, C., et al. (2009). Role of Wnt-5A in interleukin-1beta-induced matrix metalloproteinase expression in rabbit temporomandibular joint condylar chondrocytes. Arthritis Rheum. 60 (9), 2714–2722. doi:10.1002/art.24779
Gong, L., Bao, Q., Hu, C., Wang, J., Zhou, Q., Wei, L., et al. (2018). Exosomal miR-675 from metastatic osteosarcoma promotes cell migration and invasion by targeting CALN1. Biochem. Biophys. Res. Commun. 500 (2), 170–176. doi:10.1016/j.bbrc.2018.04.016
Guo, S. C., Tao, S. C., Yin, W. J., Qi, X., Sheng, J. G., and Zhang, C. Q. (2016). Exosomes from human synovial-derived mesenchymal stem cells prevent glucocorticoid-induced osteonecrosis of the femoral head in the rat. Int. J. Biol. Sci. 12 (10), 1262–1272. doi:10.7150/ijbs.16150
Hassanpour, M., Rezabakhsh, A., Rezaie, J., Nouri, M., and Rahbarghazi, R. (2020). Exosomal cargos modulate autophagy in recipient cells via different signaling pathways. Cell. Biosci. 10, 92. doi:10.1186/s13578-020-00455-7
He, C., Zheng, S., Luo, Y., and Wang, B. (2018). Exosome theranostics: Biology and translational medicine. Theranostics 8 (1), 237–255. doi:10.7150/thno.21945
Hingert, D., Ekström, K., Aldridge, J., Crescitelli, R., and Brisby, H. (2020). Extracellular vesicles from human mesenchymal stem cells expedite chondrogenesis in 3D human degenerative disc cell cultures. Stem Cell. Res. Ther. 11 (1), 323. doi:10.1186/s13287-020-01832-2
Hu, Y., Wang, Y., Chen, T., Hao, Z., Cai, L., Li, J., et al. (2021). Exosome: Function and application in inflammatory bone diseases. Oxid. Med. Cell. Longev. 2021, 6324912. doi:10.1155/2021/6324912
Kang, J., and Guo, Y. (2022). Human umbilical cord mesenchymal stem cells derived exosomes promote neurological function recovery in a rat spinal cord injury model. Neurochem. Res. 8, 1532–1540. doi:10.1007/s11064-022-03545-9
Karlsson, T., Lundholm, M., Widmark, A., and Persson, E. (2016). Tumor cell-derived exosomes from the prostate cancer cell line TRAMP-C1 impair osteoclast formation and differentiation. PLoS One 11 (11), e0166284. doi:10.1371/journal.pone.0166284
Ke, Z., and Zhu, J. (2020). Stem-cell derived exosomes for the treatment of osteoarthritis. Curr. Stem Cell. Res. Ther. 15 (7), 597–601. doi:10.2174/1574888X15666200206104332
Li, D., Liu, J., Guo, B., Liang, C., Dang, L., Lu, C., et al. (2016). Osteoclast-derived exosomal miR-214-3p inhibits osteoblastic bone formation. Nat. Commun. 7, 10872. doi:10.1038/ncomms10872
Li, H., Liu, D., Li, C., Zhou, S., Tian, D., Xiao, D., et al. (2017). Exosomes secreted from mutant-HIF-1α-modified bone-marrow-derived mesenchymal stem cells attenuate early steroid-induced avascular necrosis of femoral head in rabbit. Cell. Biol. Int. 41 (12), 1379–1390. doi:10.1002/cbin.10869
Li, Y., Jin, D., Xie, W., Wen, L., Chen, W., Xu, J., et al. (2018). Mesenchymal stem cells-derived exosomes: A possible therapeutic strategy for osteoporosis. Curr. Stem Cell. Res. Ther. 13 (5), 362–368. doi:10.2174/1574888X13666180403163456
Liang, Y., Xu, X., Li, X., Xiong, J., Li, B., Duan, L., et al. (2020). Chondrocyte-targeted MicroRNA delivery by engineered exosomes toward a cell-free osteoarthritis therapy. ACS Appl. Mat. Interfaces 12 (33), 36938–36947. doi:10.1021/acsami.0c10458
Liu, H., Li, R., Liu, T., Yang, L., Yin, G., Xie, Q., et al. (2020). Immunomodulatory effects of mesenchymal stem cells and mesenchymal stem cell-derived extracellular vesicles in rheumatoid arthritis. Front. Immunol. 11, 1912. doi:10.3389/fimmu.2020.01912
Liu, Q., Piao, H., Wang, Y., Zheng, D., and Wang, W. (2021). Circulating exosomes in cardiovascular disease: Novel carriers of biological information. Biomed. Pharmacother. 135, 111148. doi:10.1016/j.biopha.2020.111148
Liu, X., Li, Q., Niu, X., Hu, B., Chen, S., Song, W., et al. (2017). Exosomes secreted from human-induced pluripotent stem cell-derived mesenchymal stem cells prevent osteonecrosis of the femoral head by promoting angiogenesis. Int. J. Biol. Sci. 13 (2), 232–244. doi:10.7150/ijbs.16951
Liu, Y., Lin, L., Zou, R., Wen, C., Wang, Z., Lin, F., et al. (2018a). MSC-derived exosomes promote proliferation and inhibit apoptosis of chondrocytes via lncRNA-KLF3-AS1/miR-206/GIT1 axis in osteoarthritis. Cell. Cycle 17 (21-22), 2411–2422. doi:10.1080/15384101.2018.1526603
Liu, Y., Zou, R., Wang, Z., Wen, C., Zhang, F., Lin, F., et al. (2018b). Exosomal KLF3-AS1 from hMSCs promoted cartilage repair and chondrocyte proliferation in osteoarthritis. Biochem. J. 475 (22), 3629–3638. doi:10.1042/BCJ20180675
Lu, K., Li, H. Y., Yang, K., Wu, J. L., Cai, X. W., Zhou, Y., et al. (2017b). Exosomes as potential alternatives to stem cell therapy for intervertebral disc degeneration: In-vitro study on exosomes in interaction of nucleus pulposus cells and bone marrow mesenchymal stem cells. Stem Cell. Res. Ther. 8 (1), 108. doi:10.1186/s13287-017-0563-9
Lu, Z., Chen, Y., Dunstan, C., Roohani-Esfahani, S., and Zreiqat, H. (2017a). Priming adipose stem cells with tumor necrosis factor-alpha preconditioning potentiates their exosome efficacy for bone regeneration. Tissue Eng. Part A 23 (21-22), 1212–1220. doi:10.1089/ten.tea.2016.0548
Mao, G., Hu, S., Zhang, Z., Wu, P., Zhao, X., Lin, R., et al. (2018b). Exosomal miR-95-5p regulates chondrogenesis and cartilage degradation via histone deacetylase 2/8. J. Cell. Mol. Med. 22 (11), 5354–5366. doi:10.1111/jcmm.13808
Mao, G., Zhang, Z., Hu, S., Chang, Z., Huang, Z., Liao, W., et al. (2018a). Exosomes derived from miR-92a-3p-overexpressing human mesenchymal stem cells enhance chondrogenesis and suppress cartilage degradation via targeting WNT5A. Stem Cell. Res. Ther. 9 (1), 247. doi:10.1186/s13287-018-1004-0
Martins, M., Ribeiro, D., Martins, A., Reis, R. L., and Neves, N. M. (2016). Extracellular vesicles derived from osteogenically induced human bone marrow mesenchymal stem cells can modulate lineage commitment. Stem Cell. Rep. 6 (3), 284–291. doi:10.1016/j.stemcr.2016.01.001
Nakamura, Y., Miyaki, S., Ishitobi, H., Matsuyama, S., Nakasa, T., Kamei, N., et al. (2015). Mesenchymal-stem-cell-derived exosomes accelerate skeletal muscle regeneration. FEBS Lett. 589 (11), 1257–1265. doi:10.1016/j.febslet.2015.03.031
Qi, X., Zhang, J., Yuan, H., Xu, Z., Li, Q., Niu, X., et al. (2016). Exosomes secreted by human induced pluripotent stem cell derived mesen- chymal stem cel repir critical sized bone defects through enhanced angiogenesis and osteogenesis in Os- teoporotic rats. Int. J. Biol. Sci. 12, 836–849. doi:10.7150/ijbs.14809
Qin, Y., Peng, Y., Zhao, W., Pan, J., Ksiezak-Reding, H., Cardozo, C., et al. (2017). Myostatin inhibits osteoblastic differentiation by suppressing osteocyte-derived exosomal microRNA-218: A novel mechanism in muscle-bone communication. J. Biol. Chem. 292 (26), 11021–11033. doi:10.1074/jbc.M116.770941
Qin, Y., Sun, R., Wu, C., Wang, L., and Zhang, C. (2016b). Exosome: A novel approach to stimulate bone regeneration through regulation of osteogenesis and angiogenesis. Int. J. Mol. Sci. 17 (5), 712. doi:10.3390/ijms17050712
Qin, Y., Wang, L., Gao, Z., Chen, G., and Zhang, C. (2016a). Bone marrow stromal/stem cell-derived extracellular vesicles regulate osteoblast activity and differentiation in vitro and promote bone regeneration in vivo. Sci. Rep. 6, 21961. doi:10.1038/srep21961
Raggatt, L. J., and Partridge, N. C. (2010). Cellular and molecular mechanisms of bone remodeling. J. Biol. Chem. 285 (33), 25103–25108. doi:10.1074/jbc.R109.041087
Rajagopal, C., and Harikumar, K. B. (2018). The origin and functions of exosomes in cancer. Front. Oncol. 8, 66. doi:10.3389/fonc.2018.00066
Rezaie, J., Ahmadi, M., Ravanbakhsh, R., Mojarad, B., Mahbubfam, S., Shaban, S. A., et al. (2022). Tumor-derived extracellular vesicles: The metastatic organotropism drivers. Life Sci. 289, 120216. doi:10.1016/j.lfs.2021.120216
Rezaie, J., Aslan, C., Ahmadi, M., Zolbanin, N. M., Kashanchi, F., Jafari, R., et al. (2021). The versatile role of exosomes in human retroviral infections: From immunopathogenesis to clinical application. Cell. Biosci. 11 (1), 19. doi:10.1186/s13578-021-00537-0
Rezaie, J., Rahbarghazi, R., Pezeshki, M., Mazhar, M., Yekani, F., Khaksar, M., et al. (2019). Cardioprotective role of extracellular vesicles: A highlight on exosome beneficial effects in cardiovascular diseases. J. Cell. Physiol. 234 (12), 21732–21745. doi:10.1002/jcp.28894
Robling, A. G., and Bonewald, L. F. (2020). The osteocyte: New insights. Annu. Rev. Physiol. 82, 485–506. doi:10.1146/annurev-physiol-021119-034332
Sahoo, S., Klychko, E., Thorne, T., Misener, S., Schultz, K. M., Millay, M., et al. (20112011). Exosomes from hu- man CD34 ( +) stem cells mediate their proangiogenic paracrine activity. Circ. Res 109, 724–728. doi:10.1161/CIRCRESAHA.111.253286
Salomon, C., Ryan, J., Sobrevia, L., Kobayashi, M., Ashman, A., Mitchell, M., et al. (2013). Exosomal signaling dur- ing hypoxia mediates microvascular endothelial cell migration and vasculogenesis. PLoS ONE 8, e68451. doi:10.1371/journal.pone.0068451
Sućur, A., Katavić, V., Kelava, T., Jajić, Z., Kovačić, N., Grčević, D., et al. (2014). Induction of osteoclast progenitors in inflammatory conditions: Key to bone destruction in arthritis. Int. Orthop. 38 (9), 1893–1903. doi:10.1007/s00264-014-2386-y
Sun, H., Hu, S., Zhang, Z., Lun, J., Liao, W., Zhang, Z., et al. (2019). Expression of exosomal microRNAs during chondrogenic differentiation of human bone mesenchymal stem cells. J. Cell. Biochem. 120 (1), 171–181. doi:10.1002/jcb.27289
Tao, S. C., Yuan, T., Rui, B. Y., Zhu, Z. Z., Guo, S. C., Zhang, C. Q., et al. (2017b). Exosomes derived from human platelet-rich plasma prevent apoptosis induced by glucocorticoid-associated endoplasmic reticulum stress in rat osteonecrosis of the femoral head via the Akt/Bad/Bcl-2 signal pathway. Theranostics 7 (3), 733–750. doi:10.7150/thno.17450
Tao, S. C., Yuan, T., Zhang, Y. L., Yin, W. J., Guo, S. C., Zhang, C. Q., et al. (2017a). Exosomes derived from miR-140-5p-overexpressing human synovial mesenchymal stem cells enhance cartilage tissue regeneration and prevent osteoarthritis of the knee in a rat model. Theranostics 7 (1), 180–195. doi:10.7150/thno.17133
Vičić, I., and Belev, B. (2021). The pathogenesis of bone metastasis in solid tumors: A review. Croat. Med. J. 62 (3), 270–282. doi:10.3325/cmj.2021.62.270
Vrijsen, K., van Balkom, B. W. M., Noort, W. A., Chamuleau, S. A. J., Sluijter, J. P., Schuchardt, M. W., et al. (2010). Cardiomyo- cyte progenitor cell derived exosomes stimulate migration of endothelial cells. J. Cell. Mol. Med. 14, 1064–1070. doi:10.1111/j.1582-4934.2010.01081.x
Wang, R., Xu, B., and Xu, H. (2018). TGF-β1 promoted chondrocyte proliferation by regulating Sp1 through MSC-exosomes derived miR-135b. Cell. Cycle 17 (24), 2756–2765. doi:10.1080/15384101.2018.1556063
Wang, X., and Thomsen, P. (2021). Mesenchymal stem cell-derived small extracellular vesicles and bone regeneration. Basic Clin. Pharmacol. Toxicol. 128 (1), 18–36. doi:10.1111/bcpt.13478
Weidle, U. H., Birzele, F., Kollmorgen, G., and Rüger, R. (2017). The multiple roles of exosomes in metastasis. Cancer Genomics Proteomics 14 (1), 1–15. doi:10.21873/cgp.20015
Withrow, J., Murphy, C., Liu, Y., Hunter, M., Fulzele, S., Hamrick, M. W., et al. (2016). Extracellular vesicles in the pathogenesis of rheumatoid arthritis and osteoarthritis. Arthritis Res. Ther. 18 (1), 286. doi:10.1186/s13075-016-1178-8
Wu, J., Kuang, L., Chen, C., Yang, J., Zeng, W. N., Li, T., et al. (2019). miR-100-5p-abundant exosomes derived from infrapatellar fat pad MSCs protect articular cartilage and ameliorate gait abnormalities via inhibition of mTOR in osteoarthritis. Biomaterials 206, 87–100. doi:10.1016/j.biomaterials.2019.03.022
Xie, Y., Chen, Y., Zhang, L., Ge, W., and Tang, P. (2017). The roles of bone-derived exosomes and exosomal microRNAs in regulating bone remodelling. J. Cell. Mol. Med. 21 (5), 1033–1041. doi:10.1111/jcmm.13039
Xu, J. F., Yang, G. H., Pan, X. H., Zhang, S. J., Zhao, C., Qiu, B. S., et al. (2014). Altered microRNA expression profile in exosomes during osteogenic differentiation of human bone marrow-derived mesenchymal stem cells. PLoS One 9 (12), e114627. doi:10.1371/journal.pone.0114627
Xu, Q., Cui, Y., Luan, J., Zhou, X., Li, H., Han, J., et al. (2018a). Exosomes from C2C12 myoblasts enhance osteogenic differentiation of MC3T3-E1 pre-osteoblasts by delivering miR-27a-3p. Biochem. Biophys. Res. Commun. 498 (1), 32–37. doi:10.1016/j.bbrc.2018.02.144
Xu, Z., Liu, X., Wang, H., Li, J., Dai, L., Li, J., et al. (2018b). Lung adenocarcinoma cell-derived exosomal miR-21 facilitates osteoclastogenesis. Gene 666, 116–122. doi:10.1016/j.gene.2018.05.008
Xunian, Z., and Kalluri, R. (2020). Biology and therapeutic potential of mesenchymal stem cell-derived exosomes. Cancer Sci. 111 (9), 3100–3110. doi:10.1111/cas.14563
Ye, Y., Li, S. L., Ma, Y. Y., Diao, Y. J., Yang, L., Su, M. Q., et al. (2017). Exosomal miR-141-3p regulates osteoblast activity to promote the osteoblastic metastasis of prostate cancer. Oncotarget 8 (55), 94834–94849. doi:10.18632/oncotarget.22014
Yu, L., Sui, B., Fan, W., Lei, L., Zhou, L., Yang, L., et al. (2021). Exosomes derived from osteogenic tumor activate osteoclast differentiation and concurrently inhibit osteogenesis by transferring COL1A1-targeting miRNA-92a-1-5p. J. Extracell. Vesicles 10 (3), e12056. doi:10.1002/jev2.12056
Zhang, H. C., Bi, X. Y., Wang, H. X., Xie, L. X., Liu, X., Huang, S., et al. (2012). Microvesicles derived from human umbilical cord mesenchymal stem cells stimulated by hypoxia promote angiogenesis both in vitro and in vivo. Stem Cells Dev. 21, 3289–3297. doi:10.1089/scd.2012.0095
Zhang, J., Li, S., Li, L., Li, M., Guo, C., Yao, J., et al. (2015a). Exosome and exosomal microRNA: Trafficking, sorting, and function. Genomics Proteomics Bioinforma. 13 (1), 17–24. doi:10.1016/j.gpb.2015.02.001
Zhang, L. Q., Zhao, G. Z., Xu, X. Y., Fang, J., Chen, J. M., Li, J. W., et al. (2015b). Integrin-β1 regulates chondrocyte proliferation and apoptosis through the upregulation of GIT1 expression. Int. J. Mol. Med. 35 (4), 1074–1080. doi:10.3892/ijmm.2015.2114
Zhang, Y., Bi, J., Huang, J., Tang, Y., Du, S., and Li, P. (2020). Exosome: A review of its classification, isolation techniques, storage, diagnostic and targeted therapy applications. Int. J. Nanomedicine 15, 6917–6934. doi:10.2147/IJN.S264498
Zhang, Y., Cao, X., Li, P., Fan, Y., Zhang, L., Ma, X., et al. (2021). microRNA-935-modified bone marrow mesenchymal stem cells-derived exosomes enhance osteoblast proliferation and differentiation in osteoporotic rats. Life Sci. 272, 119204. doi:10.1016/j.lfs.2021.119204
Zhao, G. Z., Zhang, L. Q., Liu, Y., Fang, J., Li, H. Z., Gao, K. H., et al. (2016). Effects of platelet-derived growth factor on chondrocyte proliferation, migration and apoptosis via regulation of GIT1 expression. Mol. Med. Rep. 14 (1), 897–903. doi:10.3892/mmr.2016.5291
Zhao, P., Xiao, L., Peng, J., Qian, Y. Q., and Huang, C. C. (2018). Exosomes derived from bone marrow mesenchymal stem cells improve osteoporosis through promoting osteoblast proliferation via MAPK pathway. Eur. Rev. Med. Pharmacol. Sci. 22 (12), 3962–3970. doi:10.26355/eurrev_201806_15280
Zhu, G., Yang, X., Peng, C., Yu, L., and Hao, Y. (2020b). Exosomal miR-532-5p from bone marrow mesenchymal stem cells reduce intervertebral disc degeneration by targeting RASSF5. Exp. Cell. Res. 393 (2), 112109. doi:10.1016/j.yexcr.2020.112109
Zhu, L., Shi, Y., Liu, L., Wang, H., Shen, P., and Yang, H. (2020a). Mesenchymal stem cells-derived exosomes ameliorate nucleus pulposus cells apoptosis via delivering miR-142-3p: Therapeutic potential for intervertebral disc degenerative diseases. Cell. Cycle 19 (14), 1727–1739. doi:10.1080/15384101.2020.1769301
Keywords: exosomes, bone marrow mesenchymal stem cell, bone remodeling, spinal cord injury, osteoarthritis, bone tumor
Citation: Zhang L, Lin Y, Zhang X and Shan C (2022) Research progress of exosomes in orthopedics. Front. Genet. 13:915141. doi: 10.3389/fgene.2022.915141
Received: 07 April 2022; Accepted: 05 July 2022;
Published: 23 August 2022.
Edited by:
Reza Rahbarghazi, Tabriz University of Medical Sciences, IranReviewed by:
Dehao Fu, Shanghai General Hospital, ChinaNaresh Kumar, The Ohio State University, United States
Qin Han, Chinese Academy of Medical Sciences and Peking Union Medical College, China
Copyright © 2022 Zhang, Lin, Zhang and Shan. This is an open-access article distributed under the terms of the Creative Commons Attribution License (CC BY). The use, distribution or reproduction in other forums is permitted, provided the original author(s) and the copyright owner(s) are credited and that the original publication in this journal is cited, in accordance with accepted academic practice. No use, distribution or reproduction is permitted which does not comply with these terms.
*Correspondence: Chen Shan, c2hhbmNoZW4xOTg1QDEyNi5jb20=