- 1Key Laboratory of Green Chemical Engineering Process of Ministry of Education, Hubei Key Laboratory of Novel Reactor and Green Chemical Technology, School of Chemical Engineering and Pharmacy, Wuhan Institute of Technology, Wuhan, China
- 2School of Medicine, Jiujiang University, Jiujiang, China
Head and neck squamous cell carcinoma (HNSCC) represents one of the most malignant and heterogeneous tumors, and the patients have low 5-year survival. Traditional Chinese medicine (TCM) has been demonstrated as an effective complementary and/or alternative therapy for advanced malignancies including HNSCC. It has been noted that several herbs that are used for preparing Yinchen Wuling San (YWLS) have anti-tumor activities, whereas their mechanisms of action remain elusive. In this study, network pharmacology and molecular docking studies were employed to explore the underlying mechanisms of action of YWLS against HNSCC. The 58 active ingredients from six herbs used for YWLS and their 506 potential targets were screened from the traditional Chinese medicine systems pharmacology database and analysis platform (TCMSP) and SwissTargetPrediction database. A total of 2,173 targets associated with HNSCC were mainly identified from the DisGeNET and GeneCards databases. An active components-targets-disease network was constructed in the Cytoscape. Top 20 hub targets, such as AKT1, EGFR, TNF, ESR1, SRC, HSP90AA1, MAPK3, ERBB2, and CCND1, were identified by a degree in the protein–protein interaction (PPI) network. Gene functional enrichment analysis showed that PI3K-AKT, MAPK, Ras, TNF, and EGFR were the main signaling pathways of YWLS in treating HNSCC. There were 48 intersected targets such as EGFR, AKT1, and TNF that were associated with patients’ outcomes by the univariate Cox analysis, and most of them had increased expression in the tumor as compared to normal tissues. The area under curves of receiver operating characteristic indicated their diagnostic potential. Inhibition of these survival-related targets and/or combination with EGFR or AKT inhibitors were promising therapeutic options in HNSCC. The partial active components of YWLS exhibited good binding with the hub targets, and ADME analysis further evaluated the drug-likeness of the active components. These compounds and targets identified in this study might provide novel treatment strategies for HNSCC patients, and the subsequent work is essential to verify the underlying mechanisms of YWLS against HNSCC.
Introduction
Head and neck cancer (HNC) is one of the most common and aggressive human malignancies worldwide and is also one of the most lethal causes of death (Johnson et al., 2020). HNC is characterized by the heterogeneity of primary sites where the tumor originates, including the oral cavity, nasopharynx, oropharynx, larynx, tongue, and hypopharynx (Rasmussen et al., 2019). HNC is understood to be primarily comprised of squamous cell carcinoma, accounting for greater than 90% of cases. Genetic heterogeneity, alcohol consumption, and tobacco abuse are considered the leading carcinogens. Infection with human papillomavirus (HPV) (Chaturvedi et al., 2011) and Epstein–Barr virus (Chien et al., 2001) are also known causes of HNC formation. The standard treatments for HNC with advanced stages are surgery, radiation therapy, chemotherapy, and chemoradiotherapy. The advancement in molecular targeted therapy and immunotherapy has provided promising therapeutic options for patients with metastatic or recurrent HNC (Casasola, 2010). However, the poor outcome of these therapies has not been improved in recent years (Siegel et al., 2022). Therefore, the identification of novel prognostic biomarkers or effective therapeutics is an urgent need.
Most patients with head and neck squamous cell carcinoma (HNSCC) are diagnosed at advanced stages and have a 40–50% 5-year survival rate when receiving standard therapies (Gregoire et al., 2010). The survival of recurrent or metastatic HNSCC was even worse with median overall survival (OS) of 1 year (Argiris et al., 2017). Meanwhile, potentially life-threatening complications or side effects caused by most therapies for HNSCC patients, such as swallowing trouble, nerve damage, dry mouth, substantial toxicity, and hearing loss, are big challenges to be solved (Johnson et al., 2020). Clinical studies have shown that traditional Chinese medicine (TCM) was effective in treating HNSCC and its complications, such as Poria cocos (PC) and Atractylodes macrocephala koidz (AMK) (Meng et al., 2018; Cheng et al., 2021). In addition, it has been reported that Artemisiae scopariae herba (ASH) and Wuling San have anti-tumor efficacy (Lu, 2012). For example, they can be used to decrease chemoradiotherapy-induced diarrhea and ascites (Qu et al., 2016). Yinchen Wuling San (YWLS) prescription is a traditional Chinese medicine from Synopsis of Golden Chamber and consists of six herbal materials including Artemisiae scopariae herba (ASH, Chinese name: Yinchen), Poria cocos (PC, Chinese name: Fuling), Alisma orientale (AO, Chinese name: Zexie), Atractylodes macrocephala koidz (AMK, Chinese name: Baizhu), Polyporus umbellatus (PU, Chinese name: Zhuling), and Cinnamomi ramulus (CR, Chinese name: Guizhi) (Yao et al., 2016). Thus, these Chinese herbs might be potential alternatives or complements for HNSCC. However, the biochemical active components and anti-tumor mechanism of YWLS are unclear and need to be explored.
Network pharmacology as a novel analytical approach has been widely used to predict pharmacological action and potential mechanisms of TCM through integrating drug targets, diseases, and their targets into biomolecular networks (Li and Zhang, 2013) (Sun et al., 2021). In this study, network pharmacology was employed to screen active ingredients of YWLS and their potential targets and to explore the action mechanisms of YWLS against HNSCC. Furthermore, the molecular interactions of identified components with their possible targets were predicted by molecular docking studies. The present study might provide underlying mechanisms of YWLS against HNSCC, and the found targets and therapeutic clues are expected to be validated in further experiments. An analysis workflow of this study is illustrated in Figure 1.
Methods and Materials
Screening of Active Ingredients of Yinchen Wuling San Prescription
YWLS is a common traditional Chinese prescription that includes six herbs: ASH, PC, AO, AMK, CR, and PU. These herbs contain a variety of compounds with the effects of anti-inflammatory, antioxidant, immune regulation, and anti-tumor. ASH has been demonstrated to induce KB epithelioid cell apoptosis through elevated mitochondrial stress and caspase activation mediated by the MAPK-stimulated signaling pathway (Cha et al., 2009). The active ingredients of these herbs were screened from the TCMSP database (http://lsp.nwu.edu.cn/tcmsp.php) (Ru et al., 2014) with parameters of drug-like properties (DL) ≥ 0.18 and oral bioavailability (OB) ≥ 30%. The available pharmacological targets of these ingredients in each herb were obtained from the SwissTargetPrediction database (http://www.swisstargetprediction.ch/) (Daina et al., 2019) since it covers more targets than the TCMSP database. Additionally, unpredicted known targets for active ingredients were added based on published literature. The UniProt database (https://www.uniprot.org/) was used to standardize gene names and target information.
Identification of Potential Targets of Head and Neck Squamous Cell Carcinoma
The DisGeNET (Pinero et al., 2015) and GeneCards (Fishilevich et al., 2017) databases were employed to screen pathological targets of HNSCC. The potential HNSCC-related targets were obtained by merging the two database-derived targets after deleting duplicates. In addition, YWLS and HNSCC-related targets were intersected by the Venn diagram.
Protein–Protein Interaction Network and Topological Analysis
To investigate potential interactions among intersecting targets of YWLS and HNSCC, the PPI network was obtained using the STRING database (Szklarczyk et al., 2021) and visualized in Cytoscape (version 3.8.0) (Shannon et al., 2003). The densely connected modules in the network were identified using the Molecular Complex Detection (MCODE) plugin (Bader and Hogue, 2003) with the default parameters (“Degree Cutoff = 2,” “Node Score Cutoff = 0.2,” “K-Core = 2,” and “Max. Depth = 100.”). The CytoNCA plugin (Tang et al., 2015) was used to calculate the nodes with the highest degree. The hub genes were retrieved by degree using the cytoHubba plugin (Chin et al., 2014).
GO and Kyoto Encyclopedia of Genes and Genomes Enrichment Analysis
To interrogate the potential functions of these intersecting targets of YWLS and HNSCC, gene functional enrichment analysis including biological process (BP), molecular function (MF), and cellular components (CC) was performed using the clusterProfiler package (Yu et al., 2012). The pathway referenced from the Kyoto Encyclopedia of Genes and Genomes pathways (KEGG) was also investigated. Moreover, among these targets, the KEGG pathways of the targets of each herb and immune-related targets involved were also investigated. Additionally, the important targets that were involved in the significantly enriched pathways were visualized in the pathway maps using the Pathview R package (Luo and Brouwer, 2013).
Construction of Active Compounds of Yinchen Wuling San Prescription–Head and Neck Squamous Cell Carcinoma Disease Regulatory Network
In order to illustrate the regulatory network of all active compounds of YWLS and their corresponding targets and HNSCC-related targets, the compound–disease regulatory network was generated by Perl and visualized using the Cytoscape software (Shannon et al., 2003).
Prognostic Effect of Intersecting Targets in Head and Neck Squamous Cell Carcinoma Patients
Gene expression profiles measured by Fragments Per Kilobase of transcript per Million mapped reads (Log2 (FPKM+1)) and clinical information of HNSCC patients were acquired from The Cancer Genome Atlas (TCGA) database (https://portal.gdc.cancer.gov/). The expression matrix of intersecting targets in each HNSCC patient was extracted. The expression levels of these genes between normal and cancerous tissues were compared using Wilcoxon tests and illustrated as a heatmap by the pheatmap R package. To determine their prognostic utility, univariate Cox regression analysis was employed to screen overall survival (OS)-related genes.
An independent HNSCC dataset (GSE42743, n = 103) (Lohavanichbutr et al., 2013) was used to validate the survival-related target expression pattern in tumor and normal tissues.
Molecular Docking
The overlapped genes of the top 20 hub targets and the genes that have a prognostic effect were searched in the RCSB PDB database (https://www.rcsb.org), and their available 3D protein conformations with resolutions less than 3Å as determined by X-ray crystal diffraction were used. The structures of the selected active ingredients of YWLS were downloaded from the PubChem database (SDF.files). SybylX-2.0 software was used to perform energy minimization and optimize geometry in the Tripos force field. SybylX-2.0 software was applied for molecular docking studies. We processed proteins as follows: removing the co-crystalized ligand and water molecules from the structure, adding the H atoms, and fixing the terminal. The Surflex-Dock (SFXC) docking mode was applied, and the obtained total scores usually indicate the binding force.
It is widely believed that the total score >4.0 indicates that the docking ligands have certain binding activity with the target, the total score >5.0 indicates good binding activity, and the total score >7.0 indicates strong binding activity (Lohning et al., 2017). Meanwhile, export the protein and small-molecule docking file and import the file into PyMOL to visualize the results.
Absorption, Distribution, Metabolism, and Excretion Analysis of Active Molecules
To evaluate potential active ingredients with good ADME characteristics, pharmacokinetic properties, drug-likeness, and medicinal chemistry friendliness of these molecules were predicted using the SwissADME database (http://www.swissadme.ch/) (Daina et al., 2017).
Results
Active Ingredients of Yinchen Wuling San Prescription
A total of 58 active ingredients of YWLS with OB ≥ 30% and DL ≥ 0.18 were acquired in the TCMSP database (Table 1). There were 11 compounds from PU, 10 compounds from AO, 15 compounds from PC, 7 compounds from AMK, 7 compounds from CR, and 13 compounds from ASH, respectively. We noted that CR and AO share the sitosterol ingredient, CR and ASH have the same ingredient beta-sitosterol, and CR and PU share the peroxyergosterol ingredient. Cerevisterol and ergosta-7,22E-dien-3beta-ol were the same compounds from PU and PC.
Target Prediction of Active Ingredients of Yinchen Wuling San Prescription
The targets of these active ingredients in YWLS were predicted in the SwissTargetPrediction database, and 506 potential targets were obtained after the duplicate deletion (Supplementary Table S1). Among these targets, a total of 131 targets are immune-related genes, which are mainly categorized into cytokines and their receptors, BCR signaling pathway, antimicrobials, natural killer cell cytotoxicity, and TCR signaling pathway, suggesting that active components of YWLS might act through modulating immune response (Supplementary Table S1). Furthermore, the most enriched GO terms and KEGG pathways of these immune-related genes are the same. Some signaling pathways related to immune regulation including T cell receptor, VEGF, and Fc epsilon RI signaling corroborated the conjecture (Supplementary Figure S1A, B).
Disease-Related Targets Prediction of Head and Neck Squamous Cell Carcinoma
The keywords “head and neck carcinoma” and “head and neck squamous cell carcinoma” were used to search in DisGeNET and GeneCards databases. A total of 2,173 potential pathological targets related to HNSCC were acquired (Figure 2A, Supplementary Table S2). The related targets of HNSCC and active ingredients of YWLS were intersected using the Venn diagram, and 212 disease- and ingredient-related targets were obtained (Figure 2B). The genes corresponding to these targets were further confirmed by the UniProt database (Table 2).
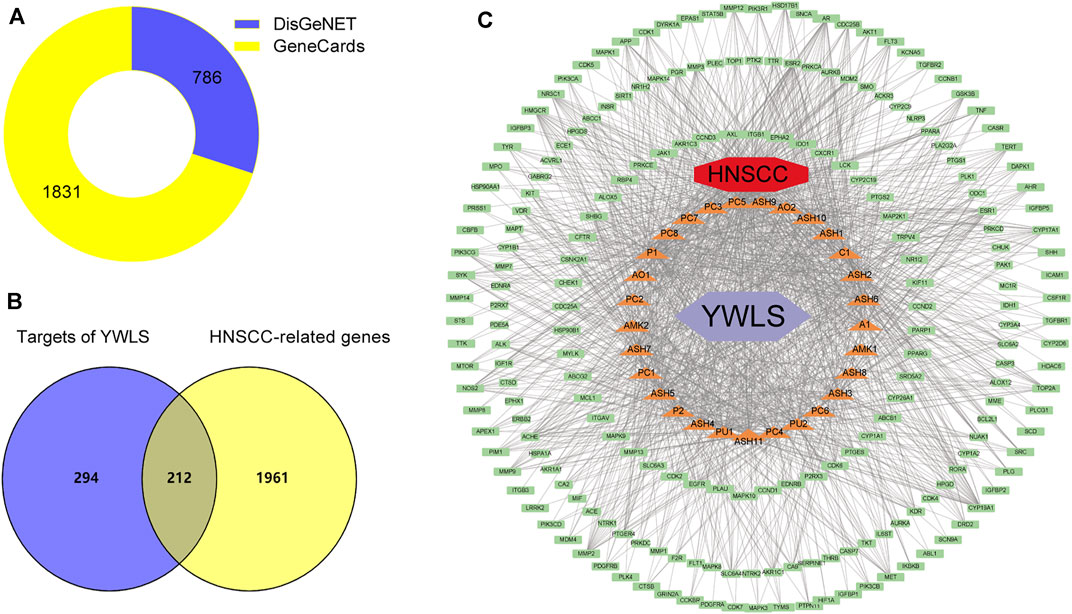
FIGURE 2. Construction of compound-target-disease network. (A). Screening of head and neck squamous cell carcinoma related targets from DisGeNET and GeneCards databases. (B) The Venny plot of 212 potential targets. (C) The active component-target-disease network. The red diamond represented the disease; the light green rectangle represented intersecting targets; the light purple diamond represented YWLS; the orange triangle represented active compounds (C1, MOL000359; AO1, MOL002464; AO2, MOL000862; P1, MOL000279; P2, MOL000282; PU1, MOL000796; PU2, MOL000820; PC1, MOL000273; PC2, MOL000276; PC3, MOL000280; PC4, MOL000285, PC5, MOL000287; PC6, MOL000290; PC7, MOL000291; PC8, MOL000292; AMK1, MOL000072; AMK2, MOL000033; A1, MOL000358; ASH1, MOL000354; ASH2, MOL004609; ASH3, MOL005573; ASH4, MOL007274; ASH5, MOL008039; ASH6, MOL008040; ASH7, MOL008041; ASH8, MOL008043; ASH9, MOL008045; ASH10, MOL008046; and ASH11, MOL000098). The edges represented the connection among active components, targets, and disease.
Construction of the Compound–Disease Regulatory Network
The ingredient-target-disease interaction network was established using Perl and constructed via Cytoscape (Figure 2C), and 242 nodes and 2,640 edges constituted the network. Active compound cerevisterol had the most nodes. (22e,24r)-ergosta-6-en-3beta,5alpha,6beta-triol and polyporusterone E ranked as the secondary and tertiary central nodes, respectively, suggesting they might be the most efficacious components against HNSCC with multiple effects by interacting with different targets (Supplementary Figure S1C, Supplementary Table S3).
PPI Network Analysis
The PPI analysis was performed to investigate the potential interactions of 212 targets. Four significant modules, AKT1, EGFR, TNF, and CYP3A4, were identified in the whole network (Figure 3A). The AKT1 module contained 45 nodes and 342 edges, the EGFR module had 34 nodes and 411 edges, and the TNF module comprised 34 nodes and 97 edges. CYP3A4, CYP2C9, and CYP1A1 were the top three nodes of the CYP3A4 module, which belong to the most common drug-metabolizing enzymes (DME) that contribute significantly to the elimination pathways of new chemical entities (Di, 2014). Furthermore, the top 20 targets ranked by degree in the network were regarded as the hub genes (Figure 3B). Among them, AKT1, EGFR, and TNF were the top 3 hub genes according to the degree. These hub genes might have important implications for the pathogenesis of HNSCC. AKT1 can restrict the invasive capacity of HNC cells through the EGFR-PI3K-AKT-mTOR signaling axis (Brolih et al., 2018) and was involved in acquired cetuximab resistant HNSCC (Zaryouh et al., 2021). Meanwhile, EGFR has been reported as anti-tumor target due to its important role in cell proliferation and survival (Burtness, 2005). Moreover, TNF signaling plays a tumor-promoting role by inducing suppressive tumor immune microenvironment and apoptosis resistance in HNSCC (Sandra et al., 2002; Jackson-Bernitsas et al., 2007; Lu et al., 2011). Blockades of these targets represent potential therapeutics for tumors including HNSCC.
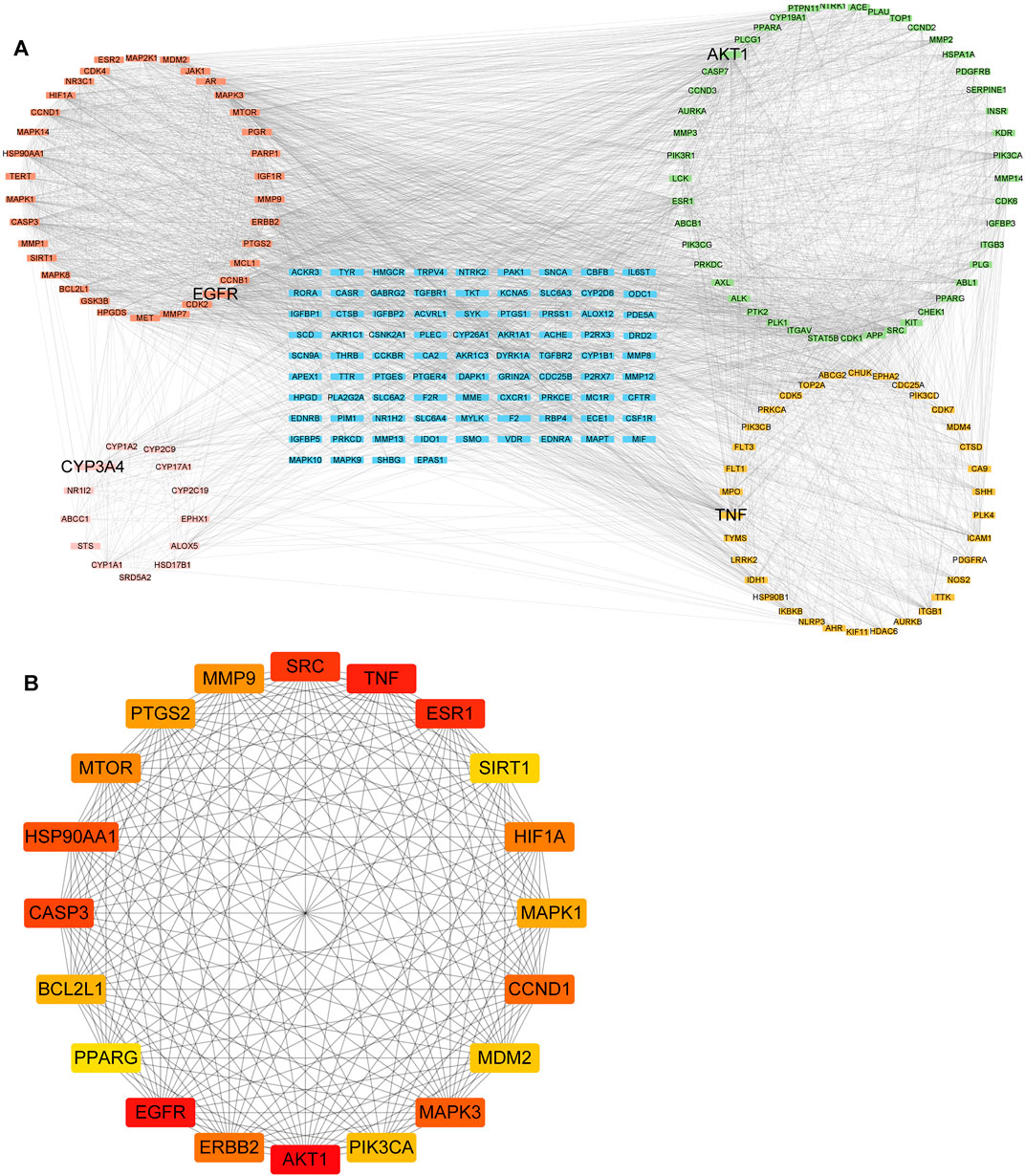
FIGURE 3. The protein–protein network of 212 intersecting targets. (A) Four modules (EGFR, AKT1, TNF, and CYP3A4) were identified from the whole PPI network. (B) The top 20 Core targets are determined by the degree. Color represented the target degree.
Functional Enrichment Analysis
To investigate the biological functions of 212 potential targets, GO terms analysis showed that they were mainly involved in the biological processes of response to oxidative and chemical stress, peptidyl-serine/tyrosine modification, and protein kinase B signaling pathway. Membrane raft and microdomain, focal adhesion and cell-substrate junction, and protein kinase complex were the main cellular components. Protein kinase activity, growth factor binding activity, and nuclear receptor and ligand-activated transcription factor activity are the top molecular functions (Figure 4A). The pathways referenced from the KEGG database indicated that these targets were enriched in various signaling pathways related to human malignancies, including PI3K-AKT, Ras signaling, MAPK signaling, chemical carcinogenesis, EGFR tyrosine kinase inhibitor resistance, ErbB signaling, and FoxO signaling pathways (Figure 4B). In addition, most of the KEGG pathways that the targets of each herb from YWLS were involved in the similar pathways (Supplementary Table S4). The targets involved in PI3K-AKT and EGFR tyrosine kinase inhibitor resistance were mapped in the pathway (Figures 4C, D). EGFR-targeting inhibitors, such as cetuximab, have been used to treat HNSCC, however, only a small subset of patients showed responsiveness. This might imply that the targets of active ingredients in YWLS are involved in drug resistance (Grandis et al., 1997).
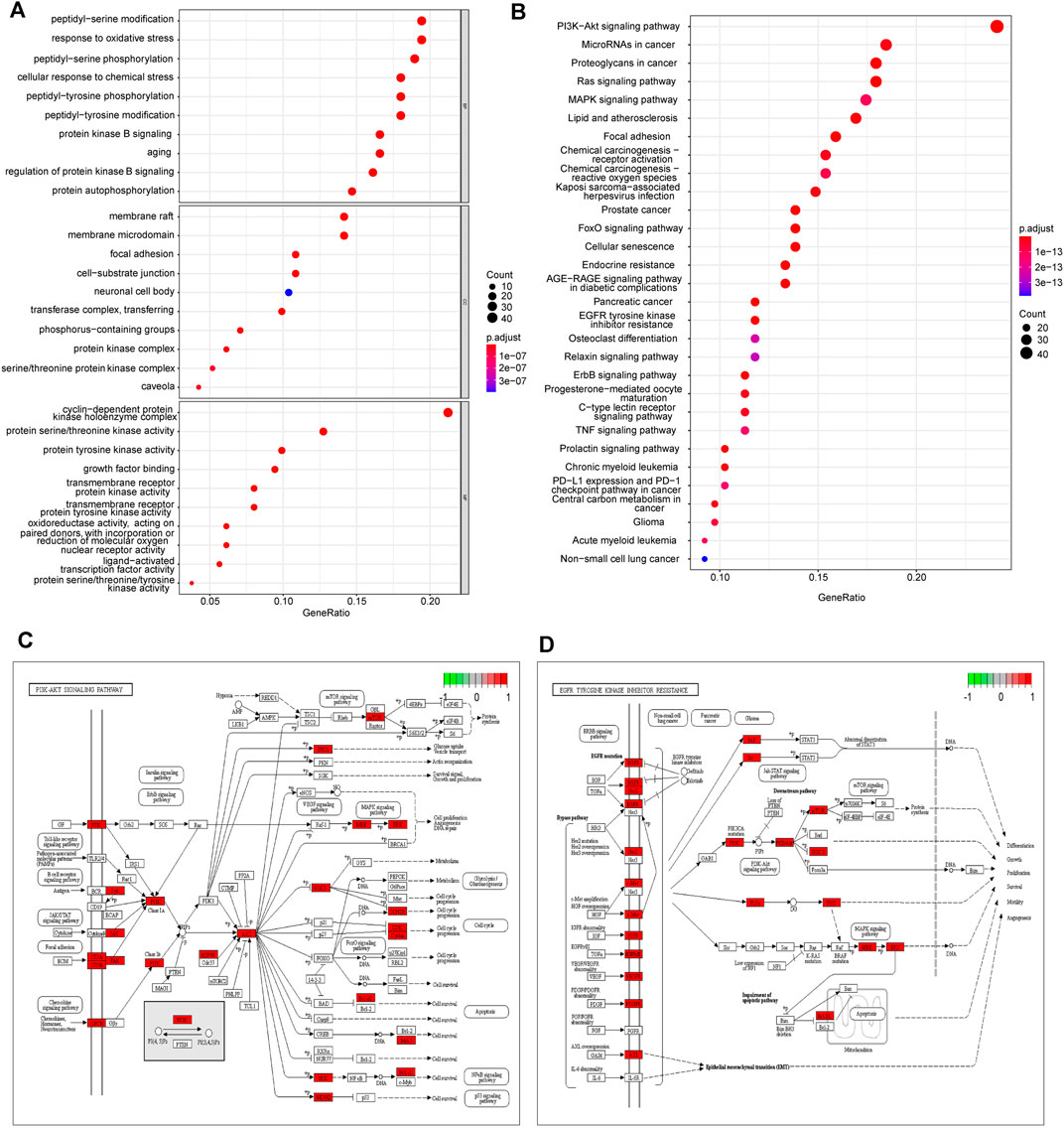
FIGURE 4. GO and KEGG enrichment analysis. (A) The top 10 enriched GO items of BP, CC, and MF. (B) The top 30 enriched KEGG pathways. (C) The important target genes were mainly distributed in the PI3K-AKT pathway. (D) The important target genes were mainly distributed in the EGFR tyrosine kinase inhibitor resistance pathway.
Correlation of Intersected Target Expression With Patients’ Overall Survival
To determine the clinical relevance of 212 intersecting targets in HNSCC patients, the univariate Cox regression analysis showed that 48 targets were significantly correlated with patients’ outcomes (Figure 5A). Among these survival-related targets, high expression of CYP2D6, FLT3, LCK, CASR, ABCB1, and ESR1 were linked to better survival, suggesting they might act as protective factors, whereas increased expression of the other 42 targets were associated with unfavorable prognosis, indicating they might be risk genes. As for the top 20 hub genes, 7 genes were found to be related to decreased survival in HNSCC patients. For example, patients with high AKT1 and EGFR expression had decreased survival, which was consistent with a previous report (Burtness, 2005).
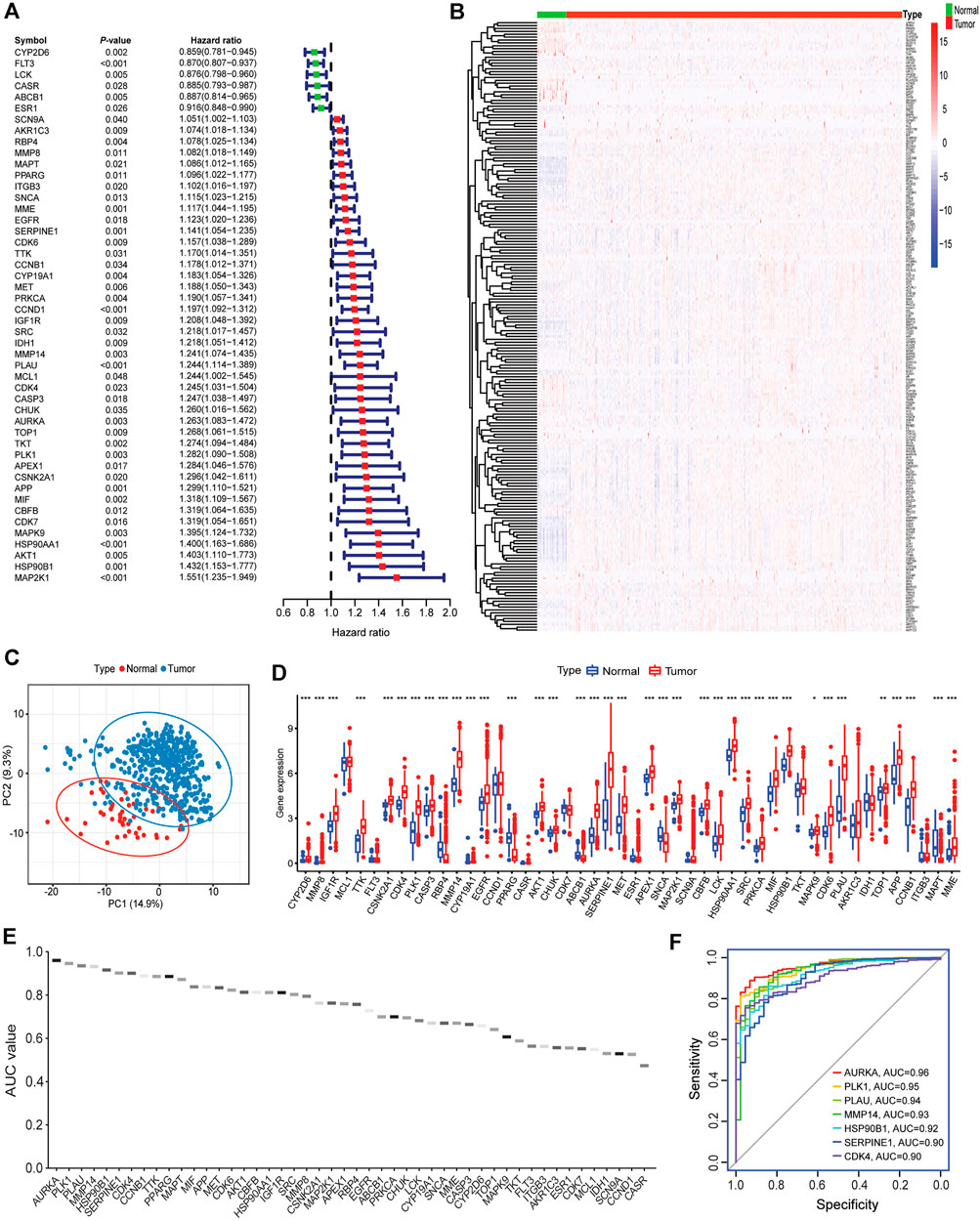
FIGURE 5. Associations of intersecting targets with patients’ outcomes, normal and tumor samples. (A) The forest plot represented the associations of intersecting targets with patients’ outcomes. (B) Heatmap of intersecting targets expression in normal vs. tumor samples. (C) Principal component analysis showed a distinct expression pattern of intersecting targets in normal vs. tumor samples. (D) Expression of 48 survival-related targets in normal vs. tumor samples (Wilcox test, ***: <0.001; **: <0.01; and *: <0.05). (E) AUC values of ROC analysis for 48 survival-related targets. (F) The top 7 targets that AUC values greater than 0.9.
The principal component analysis (PCA) showed that the expression pattern of 212 intersecting genes in the normal tissues was distinct from those in tumor samples (Figure 5B). As illustrated in Figure 5C, most of these genes had increased expression in tumor tissues than in normal tissues and showed evident expression pattern. In addition, 33 survival-related genes were observed to be elevated in tumor tissues, whereas 4 genes (RBP4, ABCB1, SCNA, and MAPT) showed increased expression in normal tissues (Figure 5D). The distinct expression pattern of survival-related targets was verified in an independent HNSCC cohort (GSE42743, Supplementary Figure S2A), and most of these targets were increased in tumors as compared to normal tissues (Supplementary Figure S2B). Receiver operating characteristic (ROC) was performed to further evaluate the diagnostic capacity of these 48 genes in separating normal from tumor samples. The area under the curve (AUC) of ROC ranged from 0.47 to 0.96 (Figure 5E). The top 7 AUCs >0.9 (AURKA, PLK1, PLAU, MMP14, HSP90B1, SERPINE1, and CDK4) are visualized in Figure 5F, indicating they may be promising targets for anti-HNSCC therapy.
Molecular Docking
Molecular docking is a powerful structure-based approach to characterize the binding behavior of small molecules in the target proteins and elucidate fundamental interactions at the atomic level (Meng et al., 2011). We found that 8 genes were overlapped between 20 hub genes and 48 survival-related genes, including AKT1, EGFR, PPARG, CCND1, SRC, CASP3, HSP90AA1, and ESR1. EGFR inhibitors including gefitinib, erlotinib, and lapatinib have shown limited therapeutic efficacy for HNSCC patients due to tumor resistance (Cohen et al., 2003; Soulieres et al., 2004). Inhibition of AKT1/2/3 with cetuximab has been reported as a promising therapeutic strategy for acquired cetuximab resistance in HNSCC patients (Zaryouh et al., 2021). Activation of SRC, one of the non-receptor tyrosine kinase protein family, promotes cell survival, proliferation, and invasion in various human malignancies including lung, colon, and prostate cancer (Dehm and Bonham, 2004). Several SRC-targeting inhibitors have been in clinical trial phases. For instance, dasatinib was approved to treat chronic myeloid leukemia (Breccia et al., 2013), whereas SRC-based therapy for HNSCC is limited (Lang et al., 2018). Overlapped genes were selected to complete molecular docking with their predicted 11 ingredients of YWLS. Among these ingredients, 10 ingredients had comparable binding scores with the selected target proteins excluding MOL000279 (Table 3 and Figures 6A–J). The docking score of MOL000862 with EGFR was 7.10, suggesting this molecule might interact well with the EGFR protein. Molecular dockings of MOL000285 and MOL005573 in PPARG and MOL008039 and MOL000796 in ESR1 also exhibited high performance. A similar high predicted binding potential was seen in AKT1 with MOL000354, MOL008041, MOL000098, and MOL008046. SRC protein with MOL000354, MOL008040, and MOL008041 showed high binding capacity. The data implied that these compounds might be potential drugs for HNSCC.
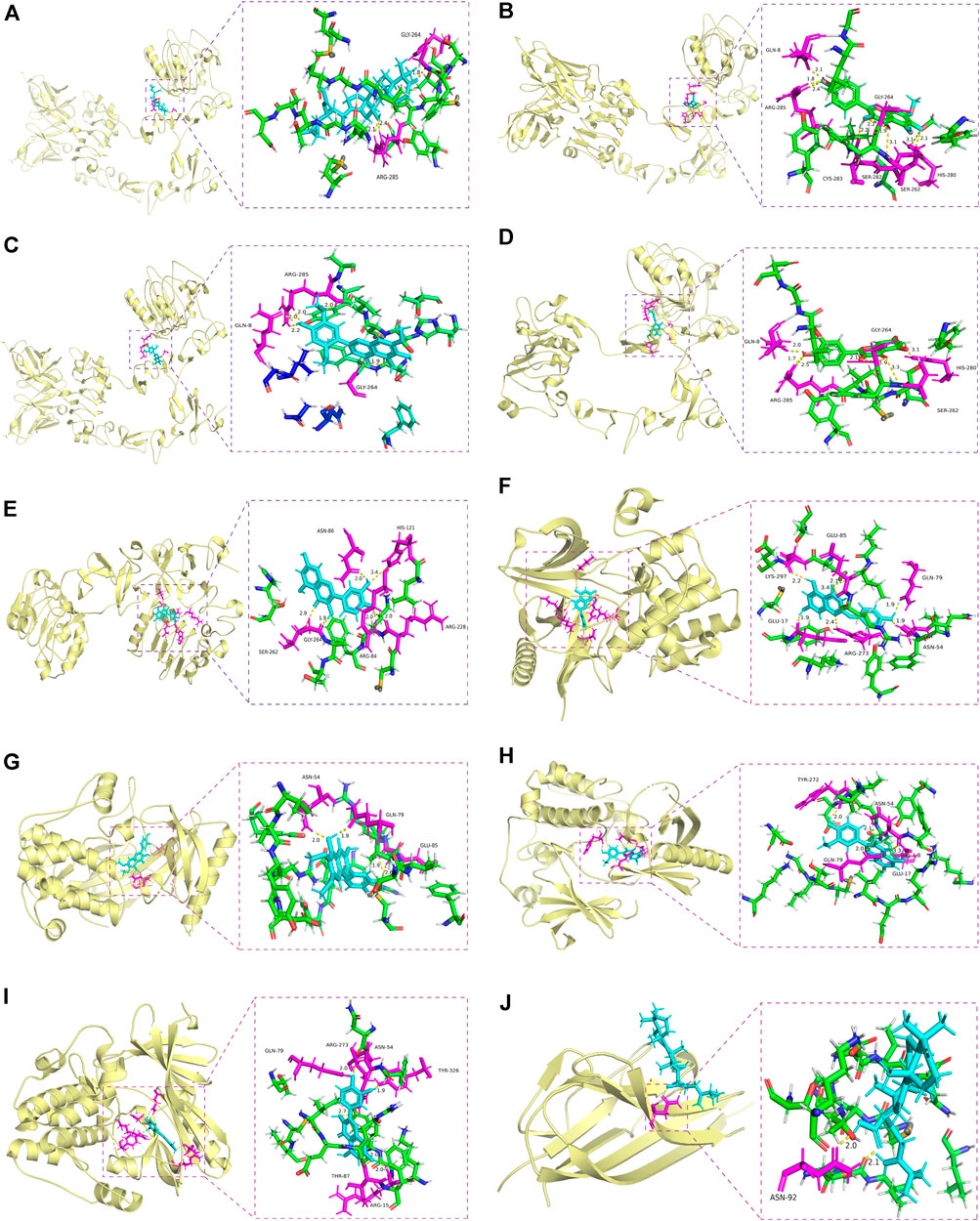
FIGURE 6. Molecular docking of active compounds in core targets. (A) Alisol B 23-acetate-EGFR. (B) Eupalitin-EGFR. (C) Isoarcapillin-EGFR. (D) Isorhamnetin-EGFR. (E) Quercetin-EGFR. (F) Demethoxycapillarisin-AKT1. (G) Eupatolitin-AKT1. (H) Isorhamnetin and AKT1. (I) Quercetin-AKT1. (J) Polyporenic acid C-TNF.
Absorption, Distribution, Metabolism, and Excretion Prediction Analysis
The pharmacokinetics, drug-likeness, and medical chemistry features of 10 active compounds were predicted using SwissADME and were compared to reference clinical drugs for HNSCC patients including methotrexate, hydroxycarbamide, and erlotinib. Gastrointestinal absorption, blood–brain barrier permeability, uptake, and drug-likeness of most compounds were comparable to the current clinical drugs. The five liver drug enzymes in Table 4 are CYP1A2, CYP2C19, CYP2C9, CYP2D6, and CYP3A4. Whether a compound is a substrate of P-gp is the key to evaluating its efflux activity through the biofilms (Montanari and Ecker, 2015). The occurrence of typical multidrug resistance is closely related to drug efflux mediated by multidrug resistance proteins of the ABC transporter family (Locher, 2009). For example, P-gp (P-glycoprotein), ABCB1, and MDR1 (Chen et al., 1986). The drug-likeness index is whether the following requirements are met: Lipinski, Ghose, Veber, Egan, Muegge, and if two or more indexes are satisfied, the drug-likeness is good (Daina et al., 2017). Except for MOL000862, other active ingredients conformed to over two drug-likeness indicators. Among them, MOL008040 can penetrate the blood–brain barrier while MOL008039, MOL008041, and MOL000098 may cause the ache. MOL000285 is a P-gp substrate with low gastrointestinal absorption.
Discussion
HNC is the sixth most prevalent human cancer worldwide, which originates from the head and neck sites including the lips, pharynx, larynx, and tongue (Puram and Rocco, 2015). Rare specific diagnostic and prognostic-related markers for patients with HNC have been identified due to genetic heterogeneity and tumor diversity (Hammerman et al., 2015). Although there are current advancements in combined treatments for HNC patients, especially for metastatic and/or recurrent patients, the HNC patients’ outcomes have not changed much in recent years. Complications or side effects also aggravated the deterioration in patients’ life quality. Identification of safe and effective drugs to treat HNC is an urgent need. TCM that has been used as an alternative or complementary therapy in human malignancies showed high safety and efficacy.
In this study, we investigated the main active ingredients of YWLS and their potential mechanisms in treating HNSCC through network pharmacology and molecular docking studies. In the component-target-disease network, cerevisterol, (22e,24r)-ergosta-6-en-3beta,5alpha,6beta-triol, polyporusterone E, genkwanin, and polyporenic acid C were the top 5 components that have relatively high degrees, which were 228, 119, 113, 108, and 105, respectively. This suggests that they might be the main active ingredients for treating HNSCC. Cerevisterol and (22e,24r)-ergosta-6-en-3beta,5alpha,6beta-triol belong to steroids. Studies have found that steroids have anti-inflammatory, immunomodulatory (Calpe-Berdiel et al., 2007), and anti-cancer activities (Imanaka et al., 2008) in breast (Grattan, 2013), gastric (De Stefani et al., 2000), and lung (Mendilaharsu et al., 1998). An epidemiological study indicated that cancer risk reduction was positively correlated with plant sterol intake (Grattan, 2013). Cerevisterol has been reported to inhibit DNA polymerase alpha (Mizushina et al., 1999) and act as a potent inhibitor of NF-kappa B signaling activation (Kim et al., 2008). It was revealed that the transcription factor NF-κB is constitutively expressed in HNSCC tissues, which results in cancer cell proliferation, survival, invasion, metastasis, and poor survival of patients (Monisha et al., 2017). This indicated that cerevisterol might be a promising drug candidate to treat HNSCC, but further in vitro and in vivo experiments’ validation are necessary. Polyporusterone E which is isolated from PU belongs to cytotoxic steroids. Pharmacological studies showed that steroids exert anti-tumor effects mainly by preventing cancer cell proliferation and inducing cancer cell apoptosis (XiaoMei et al., 2017). Polyporusterone E has a dose-independent inhibitory effect in the cell proliferation of leukemia L-1210 (Ohsawa et al., 1992). As one of the major non-glycosylated flavonoids in many herbs, genkwanin exhibited a variety of pharmacological functions, such as anti-inflammatory, chemopreventive, and antibacterial activities. It exerted an anti-inflammatory effect by the regulation of the miR-101/MKP-1/MAPK signaling pathway and the downregulation of proinflammatory mediators such as TNF-a, IL-1B, and IL-6 (Gao et al., 2014). Polyporenic acid C is one of the lanostane-type triterpenoids, and it can induce cell apoptosis in human lung cancer cells through the death receptor-mediated apoptotic pathway and is a promising agent for lung cancer therapy (Ling et al., 2009). These data implied that the active ingredients might be the potential candidates against HNSCC.
We intersected the potential targets of active ingredients of YWLS and HNSCC-related genes. Four modules named AKT1, EGFR, TNF, and CYP3A4, respectively, were identified in the PPI network of the overlapped genes. Additionally, AKT1, EGFR, and TNF are the top 3 hub genes ranked by degrees. AKT1 is one of the serine-threonine protein kinase families and is a downstream target of phosphoinositide 3-kinase (PI3K). It was a key regulator in various cell processes including cell proliferation, survival, and angiogenesis in normal and tumor cells (Vivanco and Sawyers, 2002). Activated AKT was a frequent event in many cancers such as HNSCC (Marquard and Juecker, 2020). Constitutively phosphorylated AKT and elevated kinase activity were observed in a large fraction of HNSCC (Amornphimoltham et al., 2004), suggesting AKT signaling represented a clinically relevant target. Several AKT-targeting inhibitors have been developed. An AKT inhibitor, capivasertib (AZD5363), showed significant responses in patients with tumors that carried AKT1 E17K mutation (Kalinsky et al., 2021). Two distinct AKT inhibitors, ATP-competitive and allosteric inhibitors, are in clinical development, while the allosteric inhibitor MK-2206 has failed in single-agent activity in many clinical trials (Jsb and Uba, 2017). Another inhibitor, miransertib (ARQ 092), showed promising anti-tumor effects in early phase studies (Harb et al., 2015). We noted that AKT1 is a potential target for several active ingredients from AO, PU, and CR.
Increased EGFR expression, amplification, and low frequencies of single nucleotide variations/indels have been observed in HNSCC (Xu et al., 2017; Liu et al., 2020). The overexpression of EGFR is associated with decreased survival for patients (Rubin Grandis et al., 1998). The activation of EGFR acted as a stimulator of Ras-Raf-MAPK, PI3K/AKT/mTOR, and JAK-STAT signaling pathways that promote carcinogenesis through increased cell proliferation and survival (Zimmermann et al., 2006). Targeted therapy that is directed toward EGFR for HNSCC has attracted interest. Current anti-EGFR therapeutic strategies are to target the extracellular domain of the receptor with monoclonal antibodies such as cetuximab and panitumumab (Troiani et al., 2016) and the intracellular domain using tyrosine kinase inhibitors (TKIs) such as gefitinib, erlotinib, osimertinib, and afatinib (Fasano et al., 2014). However, the low rates of response or resistance are the main challenges (Chong and Jaenne, 2013). Recently, a crucial semisynthetic derivative of artemisinin named dihydroartemisinin (DHA) combined with osimertinib showed in vitro and in vivo cytotoxicity in HNSCC (Chaib et al., 2019). This might lead to a novel strategy of EGFR inhibitors combined with TCM due to less than 5% of HNSCC patients carrying EGFR mutations. EGF-stimulated recycling of EGFR can induce AKT phosphorylation through activating downstream signaling. EGFR and AKT1 have been revealed to play a synergistic tumor-promoting role to aggravate tumor progression in human lung cancer (Nishimura et al., 2015). In addition, TNF signaling has been shown to act as a tumor accomplice in HNSCC by decreasing tumor cell apoptosis or promoting an immune-suppressive tumor microenvironment (Sandra et al., 2002; Lu et al., 2011). For example, TNF-α was proved to promote invasion and metastasis by the NF-κB pathway in oral squamous cell carcinoma (Tang et al., 2017). TNF-α can also inhibit apoptosis by activation of AKT serine/threonine kinase in HNSCC (Sandra et al., 2002). It was noted that several ingredients of YWLS might target these proteins simultaneously to result in inhibitory effects in HNSCC, but further verification will make it convincing.
The KEGG pathway analysis indicated that these 212 targets were mainly enriched in PI3K-AKT, MAPK, RAS, EGFR tyrosine kinase inhibitor resistance, ErbB, PD-L1 expression, PD-1 checkpoint pathway in cancer, and TNF signaling pathways. Previous reports demonstrated that activation of these pathways is highly correlated with cell proliferation, survival, and metastasis in HNC carcinogenesis (Tang et al., 2017) (Marquard and Juecker, 2020) and drug resistance (Picon and Guddati, 2020). These pathways are potential therapeutic targets for HNSCC patients such as EGFR and PI3K/AKT signaling. Accordingly, pathway analysis indicated that the targets of each herb in YWLS were enriched in these signaling pathways. We found that increased expression of 42 genes was associated with decreased survival, which was consistent with previous evidence, such as EGFR, AKT1, SERPINE1, HSP90AA1, HSP90B1 (Fan et al., 2020), PLAU (Li et al., 2021), MAP2K1 (Jain et al., 2019), and CCND1 (Feng et al., 2011). Among these survival-related genes, 33 genes had higher expression in tumor tissues than in normal tissues like EGFR, AKT1, and HSP family genes, suggesting they could serve as diagnostic markers to distinguish normal from tumoral samples. An independent verification analysis has shown the consistent expression pattern of these targets in tumors versus normal tissues. In addition, ROC analysis showed that the AUC values of AURKA, PLK1, PLAU, MMP14, HSP90B1, SERPINE1, and CDK4 genes are greater than 0.9, exhibiting good performance. Several survival-related genes have been reported to be pharmacologic targets for solid tumors including HNSCC. CDK4/CDK6 inhibitors have been approved to treat breast and small cell lung cancer (Riess et al., 2021). CDK 4/6/7 inhibitors for HNSCC have been in preclinical and clinical applications. For example, palbociclib and ribociclib specifically inhibit CDK4 and CDK6, and abemaciclib selectively targets CDK4. CCND1 mutations and CDKN2A/B were the predictive biomarkers of response. Dual inhibition of EGFR and MAPK/CDK4/6 prevented oesophageal squamous cell carcinoma (OSCC) progression (Zhou et al., 2017). Therapeutic targeting of MAP2K1 in the MAPK pathway was a promising strategy for EGFR inhibitor (erlotinib)-resistant HNSCC patients (Jain et al., 2019). It was reported that Aurora kinases were potential targets to overcome EGFR inhibitor resistance in HNSCC, indicating that Aurora kinase A (AURKA) blockade might be a promising approach (Kim and Bandyopadhyay, 2021). PLK1 inhibitor could induce pyroptosis in OSCC to elevate cisplatin chemosensitivity (Wu et al., 2019). Inhibition of apoptosis signaling through BCL-xL and MCL-1 in HNSCC was a potential therapeutic option (Ow et al., 2020). These findings elucidated that the combination therapy with EGFR inhibitors might synergistically enhance the anti-HNSCC capacity and attenuate the resistance to EGFR therapy, and further experimental work is needed to verify this hypothesis.
The molecular docking study was used to validate the interactions between eight survival-related hub targets and their possible active components of YWLS. The compounds showed good binding scores with the corresponding targets such as AKT1, EGFR, PPARG, ESR1, and SRC. The ADME analysis was conducted to further assess the drug potentials of these compounds for HNSCC patients. Partial components exhibited comparable pharmacological characteristics with current clinical agents. These data indicated that these compounds might be used as potential therapeutic drugs to treat HNSCC.
Conclusion
In summary, the potential therapeutic targets of active ingredients of YWLS for treating HNSCC were predicted by the network pharmacology studies, and molecular docking predicted the interactions between the active compounds and the related targets, and the drug-likeness properties of these compounds were further evaluated by the ADME analysis. The underlying mechanism of YWLS against HNSCC might be associated with PI3K-AKT, MAPK, and EGFR signaling pathways. These compounds might provide novel treatment strategies for HNSCC themselves or in combination with current molecular targeted therapies, and further verification by subsequent experiments is imperative.
Data Availability Statement
The original contributions presented in the study are included in the article/Supplementary Material; further inquiries can be directed to the corresponding authors. The data analyzed in this study are available in the following repositories: 1. TCGA: https://portal.gdc.cancer.gov/ 2. TCMSP: https://old.tcmsp-e.com/tcmsp.php 3. DisGeNET: https://www.disgenet.org/ 4. GeneCards: https://www.genecards.org/ 5. STRING: https://string-db.org/ 6. RCSB PDB: https://www.rcsb.org 7. PubChem: https://pubchem.ncbi.nlm.nih.gov/ 8. SwissTargetPrediction: http://www.swisstargetprediction.ch/ 9. SwissADME: http://www.swissadme.ch/.
Ethics Statement
This study did not involve experiments on humans or animals and thus did not require approval from an ethics committee.
Author Contributions
Conceptualization and design: XH, BZ; data acquisition: BZ; methodology: BZ and XH; data analysis and interpretation: BZ; writing (original draft): BZ; writing (review and editing): XW, GL, and XH. All authors contributed to the article and approved the submitted version.
Conflict of Interest
The authors declare that the research was conducted in the absence of any commercial or financial relationships that could be construed as a potential conflict of interest.
Publisher’s Note
All claims expressed in this article are solely those of the authors and do not necessarily represent those of their affiliated organizations, or those of the publisher, the editors, and the reviewers. Any product that may be evaluated in this article, or claim that may be made by its manufacturer, is not guaranteed or endorsed by the publisher.
Acknowledgments
We are grateful to all contributors to the public databases used in this study.
Supplementary Material
The Supplementary Material for this article can be found online at: https://www.frontiersin.org/articles/10.3389/fgene.2022.914646/full#supplementary-material
Abbreviations
HNC, head and neck cancer; HNSCC, head and neck squamous cell carcinoma; YWLS, Yinchen Wuling San; PU, Polyporus umbellatus; AO, Alisma orientale; PC, Poria cocos; AMK, Atractylodes macrocephala koidz; CR, Cinnamomi ramulus; ASH, Artemisiae scopariae herba; DEGs, differentially expressed genes; TCGA, The Cancer Genome Atlas; FPKM, Fragments Per Kilobase of transcript per Million mapped reads; TCMSP, traditional Chinese medicine systems pharmacology database and analysis platform; PPI, protein–protein interaction; MCODE. molecular complex detection; BP, biological process; CC, cellular component; MF, molecular function; KEGG, Kyoto Encyclopedia of Genes and Genomes; OS, overall survival; ADME, absorption, distribution, metabolism, and excretion. OSCC, oral squamous cell carcinoma; PCA, principal component analysis; ROC, receiver operating characteristic; and AUC, area under the curve
References
Amornphimoltham, P., Sriuranpong, V., Patel, V., Benavides, F., Conti, C. J., and Sauk, J. (2004). Persistent Activation of the Akt Pathway in Head and Neck Squamous Cell Carcinoma: a Potential Target for UCN-01. Clin. cancer Res. 10 (12 Pt 1), 4029–4037. doi:10.1158/1078-0432.ccr-03-0249
Argiris, A., Harrington, K. J., Tahara, M., Schulten, J., Chomette, P., Ferreira Castro, A., et al. (2017). Evidence-Based Treatment Options in Recurrent And/or Metastatic Squamous Cell Carcinoma of the Head and Neck. Front. Oncol. 7, 72. doi:10.3389/fonc.2017.00072
Bader, G. D., and Hogue, C. W. V. (2003). An Automated Method for Finding Molecular Complexes in Large Protein Interaction Networks. BMC Bioinforma. 4, 2. doi:10.1186/1471-2105-4-2
Breccia, M., Salaroli, A., Molica, M., and Alimena, G. (2013). Systematic Review of Dasatinib in Chronic Myeloid Leukemia. Oncotargets Ther. 6, 257–265. doi:10.2147/ott.s35360
Brolih, S., Parks, S. K., Vial, V., Durivault, J., Mostosi, L., Pouyssegur, J., et al. (2018). AKT1 Restricts the Invasive Capacity of Head and Neck Carcinoma Cells Harboring a Constitutively Active PI3 Kinase Activity. Bmc Cancer 18. doi:10.1186/s12885-018-4169-0
Burtness, B. (2005). The Role of Cetuximab in the Treatment of Squamous Cell Cancer of the Head and Neck. Expert Opin. Biol. Ther. 5 (8), 1085–1093. doi:10.1517/14712598.5.8.1085
Calpe-Berdiel, L., Escolà-Gil, J. C., Benítez, S., Bancells, C., González-Sastre, F., Palomer, X., et al. (2007). Dietary Phytosterols Modulate T-Helper Immune Response but Do Not Induce Apparent Anti-inflammatory Effects in a Mouse Model of Acute, Aseptic Inflammation. Life Sci. 80 (21), 1951–1956. doi:10.1016/j.lfs.2007.02.032
Casasola, R. J. (2010). Head and Neck Cancer. J. R. Coll. Physicians Edinb. 40 (4), 343–345. doi:10.4997/jrcpe.2010.423
Cha, J.-D., Moon, S.-E., Kim, H.-Y., Cha, I.-H., and Lee, K.-Y. (2009). Essential Oil of Artemisia Capillaris Induces Apoptosis in KB Cells via Mitochondrial Stress and Caspase Activation Mediated by MAPK-Stimulated Signaling Pathway. J. Food Sci. 74 (9), T75–T81. doi:10.1111/j.1750-3841.2009.01355.x
Chaib, I., Cai, X., Llige, D., Santarpia, M., Jantus-Lewintre, E., Filipska, M., et al. (2019). Osimertinib and Dihydroartemisinin: a Novel Drug Combination Targeting Head and Neck Squamous Cell Carcinoma. Ann. Transl. Med. 7 (22). doi:10.21037/atm.2019.10.80
Chaturvedi, A. K., Engels, E. A., Pfeiffer, R. M., Hernandez, B. Y., Xiao, W., Kim, E., et al. (2011). Human Papillomavirus and Rising Oropharyngeal Cancer Incidence in the United States. J. Clin. Oncol. 29 (32), 4294–4301. doi:10.1200/jco.2011.36.4596
Chen, C. J., Chin, J. E., Ueda, K., Clark, D. P., Pastan, I., Gottesman, M. M., et al. (1986). Internal Duplication and Homology with Bacterial Transport Proteins in the Mdr1 (P-Glycoprotein) Gene from Multidrug-Resistant Human Cells. Cell 47 (3), 381–389. doi:10.1016/0092-8674(86)90595-7
Cheng, B., Fan, J., Liu, J., Su, R., Zhou, F., Huang, R., et al. (2021). Network Pharmacology Analysis and Verification of the Material Basis and Potential Mechanism of Fuling (Poria) Against Nasopharyngeal Carcinoma. Journal of hu University of Chinese medicine 41 (9), 1381–1388.
Chien, Y. C., Chen, J. Y., Liu, M. Y., Yang, H. I., Hsu, M. M., Chen, C. J., et al. (2001). Serologic Markers of Epstein-Barr Virus Infection and Nasopharyngeal Carcinoma in Taiwanese Men. N. Engl. J. Med. 345 (26), 1877–1882. doi:10.1056/NEJMoa011610
Chin, C.-H., Chen, S.-H., Wu, H.-H., Ho, C.-W., Ko, M.-T., and Lin, C.-Y. (2014). cytoHubba: Identifying Hub Objects and Sub-networks from Complex Interactome. Bmc Syst. Biol. 8. doi:10.1186/1752-0509-8-s4-s11
Chong, C. R., and Jaenne, P. A. (2013). The Quest to Overcome Resistance to EGFR-Targeted Therapies in Cancer. Nat. Med. 19 (11), 1389–1400. doi:10.1038/nm.3388
Cohen, E. E. W., Rosen, F., Stadler, W. M., Recant, W., Stenson, K., Huo, D., et al. (2003). Phase II Trial of ZD1839 in Recurrent or Metastatic Squamous Cell Carcinoma of the Head and Neck. J. Clin. Oncol. 21 (10), 1980–1987. doi:10.1200/jco.2003.10.051
Daina, A., Michielin, O., and Zoete, V. (2019). Swiss Target Prediction: Updated Data and New Features for Efficient Prediction of Protein Targets of Small Molecules. Nucleic Acids Res. 47 (W1), W357–W364. doi:10.1093/nar/gkz382
Daina, A., Michielin, O., and Zoete, V. (2017). SwissADME: a Free Web Tool to Evaluate Pharmacokinetics, Drug-Likeness and Medicinal Chemistry Friendliness of Small Molecules. Sci. Rep. 7. doi:10.1038/srep42717
De Stefani, E., Boffetta, P., Ronco, A. L., Brennan, P., Deneo-Pellegrini, H., Carzoglio, J. C., et al. (2000). Plant Sterols and Risk of Stomach Cancer: a Case-Control Study in Uruguay. Nutr. cancer 37 (2), 140–144. doi:10.1207/s15327914nc372_4
Dehm, S. M., and Bonham, K. (2004). SRC Gene Expression in Human Cancer: the Role of Transcriptional Activation. Biochem. Cell Biol. = Biochimie Biol. Cell. 82 (2), 263–274. doi:10.1139/o03-077
Di, L. (2014). The Role of Drug Metabolizing Enzymes in Clearance. Expert Opin. Drug Metabolism Toxicol. 10 (3), 379–393. doi:10.1517/17425255.2014.876006
Fan, G., Tu, Y., Wu, N., and Xiao, H. (2020). The Expression Profiles and Prognostic Values of HSPs Family Members in Head and Neck Cancer. Cancer Cell Int. 20 (1). doi:10.1186/s12935-020-01296-7
Fasano, M., Della Corte, C. M., Califano, R., Capuano, A., Troiani, T., Martinelli, E., et al. (2014). Type III or Allosteric Kinase Inhibitors for the Treatment of Non-small Cell Lung Cancer. Expert Opin. Investigational Drugs 23 (6), 809–821. doi:10.1517/13543784.2014.902934
Feng, Z., Guo, W., Zhang, C., Xu, Q., Zhang, P., Sun, J., et al. (2011). CCND1 as a Predictive Biomarker of Neoadjuvant Chemotherapy in Patients with Locally Advanced Head and Neck Squamous Cell Carcinoma. Plos One 6 (10). doi:10.1371/journal.pone.0026399
Fishilevich, S., Nudel, R., Rappaport, N., Hadar, R., Plaschkes, I., Stein, T. I., et al. (2017). GeneHancer: Genome-wide Integration of Enhancers and Target Genes in GeneCards. Database-the J. Biol. Databases Curation 2017, bax028. doi:10.1093/database/bax028
Gao, Y., Liu, F., Fang, L., Cai, R., Zong, C., and Qi, Y. (2014). Genkwanin Inhibits Proinflammatory Mediators Mainly through the Regulation of miR-101/MKP-1/MAPK Pathway in LPS-Activated Macrophages. Plos One 9 (5). doi:10.1371/journal.pone.0096741
Grandis, J. R., Chakraborty, A., Melhem, M. F., Zeng, Q., and Tweardy, D. J. (1997). Inhibition of Epidermal Growth Factor Receptor Gene Expression and Function Decreases Proliferation of Head and Neck Squamous Carcinoma but Not Normal Mucosal Epithelial Cells. Oncogene 15 (4), 409–416. doi:10.1038/sj.onc.1201188
Grattan, B. J. (2013). Plant Sterols as Anticancer Nutrients: Evidence for Their Role in Breast Cancer. Nutrients 5 (2), 359–387. doi:10.3390/nu5020359
Gregoire, V., Lefebvre, J. L., Licitra, L., Felip, E., and Group, E.-E.-E. G. W. (2010). Squamous Cell Carcinoma of the Head and Neck: EHNS-ESMO-ESTRO Clinical Practice Guidelines for Diagnosis, Treatment and Follow-Up. Ann. Oncol. 21 (Suppl. 5), v184–186. doi:10.1093/annonc/mdq185
Hammerman, P. S., Hayes, D. N., and Grandis, J. R. (2015). Therapeutic Insights from Genomic Studies of Head and Neck Squamous Cell Carcinomas. Cancer Discov. 5 (3), 239–244. doi:10.1158/2159-8290.cd-14-1205
Harb, W., Saleh, M. N., Papadopoulos, K. P., Chai, F., Larmar, M., Abbadessa, G., et al. (2015). Clinical Trial Results from the Subgroup of Lymphoma/CLL in a Phase 1 Study of ARQ 092, a Novel Pan AKT-Inhibitor. Blood 126 (23). doi:10.1182/blood.v126.23.5116.5116
Imanaka, H., Koide, H., Shimizu, K., Asai, T., Shimizu, N. K., Ishikado, A., et al. (2008). Chemoprevention of Tumor Metastasis by Liposomal Beta-Sitosterol Intake. Biol. Pharm. Bull. 31 (3), 400–404. doi:10.1248/bpb.31.400
Jackson-Bernitsas, D. G., Ichikawa, H., Takada, Y., Myers, J. N., Lin, X. L., Darnay, B. G., et al. (2007). Evidence that TNF-TNFR1-TRADD-TRAF2-RIP-TAK1-IKK Pathway Mediates Constitutive NF-kappaB Activation and Proliferation in Human Head and Neck Squamous Cell Carcinoma. Oncogene 26 (10), 1385–1397. doi:10.1038/sj.onc.1209945
Jain, A. P., Patel, K., Pinto, S., Radhakrishnan, A., Nanjappa, V., Kumar, M., et al. (2019). MAP2K1 Is a Potential Therapeutic Target in Erlotinib Resistant Head and Neck Squamous Cell Carcinoma. Sci. Rep. 9. doi:10.1038/s41598-019-55208-5
Johnson, D. E., Burtness, B., Leemans, C. R., Lui, V. W. Y., Bauman, J. E., and Grandis, J. R. (2020). Head and Neck Squamous Cell Carcinoma. Nat. Rev. Dis. Prim. 6 (1). doi:10.1038/s41572-020-00224-3
Jsb, A., and Uba, B. (2017). Maximising the Potential of AKT Inhibitors as Anti-cancer Treatments. Pharmacol. Ther. 172, 101–115.
Kalinsky, K., Hong, F., McCourt, C. K., Sachdev, J. C., Mitchell, E. P., Zwiebel, J. A., et al. (2021). Effect of Capivasertib in Patients With an AKT1 E17K-Mutated Tumor: NCI-MATCH Subprotocol EAY131-Y Nonrandomized Trial. JAMA Oncology 7 (2), 271–278.
Kim, D., and Bandyopadhyay, S. (2021). Aurora Kinases Shed Light on Resistance to EGFR Inhibition in Head and Neck Cancer. Ebiomedicine 65. doi:10.1016/j.ebiom.2021.103257
Kim, J. A., Tay, D., and de Blanco, E. C. (2008). NF-kappaB Inhibitory Activity of Compounds Isolated from Cantharellus Cibarius. Phytother. Res. 22 (8), 1104–1106. doi:10.1002/ptr.2467
Lang, L., Shay, C., Xiong, Y., Thakkar, P., Chemmalakuzhy, R., Wang, X., et al. (2018). Combating Head and Neck Cancer Metastases by Targeting Src Using Multifunctional Nanoparticle-Based Saracatinib. J. Hematol. Oncol. 11. doi:10.1186/s13045-018-0623-3
Li, S., and Zhang, B. (2013). Traditional Chinese Medicine Network Pharmacology: Theory, Methodology and Application. Chin. J. Nat. Med. 11 (2), 110–120. doi:10.1016/s1875-5364(13)60037-0
Li, Z., Chen, C., Wang, J., Wei, M., Liu, G., Qin, Y., et al. (2021). Overexpressed PLAU and its Potential Prognostic Value in Head and Neck Squamous Cell Carcinoma. Peerj 9. doi:10.7717/peerj.10746
Ling, H., Zhou, L., Jia, X., Gapter, L. A., Agarwal, R., and Ng, K.-y. (2009). Polyporenic Acid C Induces Caspase-8-Mediated Apoptosis in Human Lung Cancer A549 Cells. Mol. Carcinog. 48 (6), 498–507. doi:10.1002/mc.20487
Liu, H., Zhang, B., and Sun, Z. (2020). Spectrum of EGFR Aberrations and Potential Clinical Implications: Insights from Integrative Pan-Cancer Analysis. Cancer Commun. 40 (1), 43–59. doi:10.1002/cac2.12005
Locher, K. P. (2009). Review. Structure and Mechanism of ATP-Binding Cassette Transporters. Philos. Trans. R. Soc. Lond B Biol. Sci. 364 (1514), 239–245. doi:10.1098/rstb.2008.0125
Lohavanichbutr, P., Mendez, E., Holsinger, F. C., Rue, T. C., Zhang, Y., Houck, J., et al. (2013). A 13-gene Signature Prognostic of HPV-Negative OSCC: Discovery and External Validation. Clin. Cancer Res. 19 (5), 1197–1203. doi:10.1158/1078-0432.CCR-12-2647
Lohning, A. E., Levonis, S. M., Williams-Noonan, B., and Schweiker, S. S. (2017). A Practical Guide to Molecular Docking and Homology Modelling for Medicinal Chemists. Curr. Top. Med. Chem. 17 (18), 2023–2040. doi:10.2174/1568026617666170130110827
Lu, H., Yang, X., Duggal, P., Allen, C. T., Yan, B., Cohen, J., et al. (2011). TNF-alpha Promotes C-REL/DeltaNp63alpha Interaction and TAp73 Dissociation from Key Genes that Mediate Growth Arrest and Apoptosis in Head and Neck Cancer. Cancer Res. 71 (21), 6867–6877. doi:10.1158/0008-5472.CAN-11-2460
Luo, W., and Brouwer, C. (2013). Pathview: an R/Bioconductor Package for Pathway-Based Data Integration and Visualization. Bioinformatics 29 (14), 1830–1831. doi:10.1093/bioinformatics/btt285
Lu, S. (2012). Traditional Chinese Medicine Preparation for Treating Cancer and Preparation Method Thereof. P.R. China: Patent No CN101670071A Beijing: P.R. China Internal Intellectual Property Administration.
Marquard, F. E., and Juecker, M. (2020). PI3K/AKT/mTOR Signaling as a Molecular Target in Head and Neck Cancer. Biochem. Pharmacol. 172. doi:10.1016/j.bcp.2019.113729
Mendilaharsu, M., De Stefani, E., Deneo-Pellegrini, H., Carzoglio, J., and Ronco, A. (1998). Phytosterols and Risk of Lung Cancer: a Case-Control Study in Uruguay. Lung cancer (Amsterdam, Neth. 21 (1), 37–45. doi:10.1016/s0169-5002(98)00044-0
Meng, L. I., Shang, J., Yang, B., and Zhao, R. X. (2018). Effects of Zhenqi Fuzheng Granule on Curative Effect and T Lymphocyte Subsets in Postoperative Patients with Breast Cancer Undergoing Chemotherapy. Beijing, China: Medical & Pharmaceutical Journal of Chinese People's Liberation Army. Available at: http://www.plamj.org/index.php/plamj/index
Meng, X.-Y., Zhang, H.-X., Mezei, M., and Cui, M. (2011). Molecular Docking: A Powerful Approach for Structure-Based Drug Discovery. Curr. Computer-Aided Drug Des. 7 (2), 146–157. doi:10.2174/157340911795677602
Mizushina, Y., Takahashi, N., Hanashima, L., Koshino, H., Esumi, Y., Uzawa, J., et al. (1999). Lucidenic Acid O and Lactone, New Terpene Inhibitors of Eukaryotic DNA Polymerases from a Basidiomycete, Ganoderma Lucidum. Bioorg. Med. Chem. 7 (9), 2047–2052. doi:10.1016/s0968-0896(99)00121-2
Monisha, J., Roy, N. K., Bordoloi, D., Kumar, A., Golla, R., Kotoky, J., et al. (2017). Nuclear Factor Kappa B: A Potential Target to Persecute Head and Neck Cancer. Curr. Drug Targets 18 (2), 232–253. doi:10.2174/1389450117666160201112330
Montanari, F., and Ecker, G. F. (2015). Prediction of Drug-ABC-Transporter Interaction - Recent Advances and Future Challenges. Adv. Drug Deliv. Rev. 86, 17–26. doi:10.1016/j.addr.2015.03.001
Nishimura, Y., Takiguchi, S., Ito, S., and Itoh, K. (2015). EGF-stimulated AKT Activation Is Mediated by EGFR Recycling via an Early Endocytic Pathway in a Gefitinib-Resistant Human Lung Cancer Cell Line. Int. J. Oncol. 46 (4), 1721–1729. doi:10.3892/ijo.2015.2871
Ohsawa, T., Yukawa, M., Takao, C., Murayama, M., and Bando, H. (1992). Studies on Constituents of Fruit Body of Polyporus Umbellatus and Their Cytotoxic Activity. Chem. Pharm. Bull. 40 (1), 143–147. doi:10.1248/cpb.40.143
Ow, T. J., Thomas, C., Fulcher, C. D., Chen, J., Lopez, A., Reyna, D. E., et al. (2020). Apoptosis Signaling Molecules as Treatment Targets in Head and Neck Squamous Cell Carcinoma. Laryngoscope 130 (11), 2643–2649. doi:10.1002/lary.28441
Picon, H., and Guddati, A. K. (2020). Mechanisms of Resistance in Head and Neck Cancer. Am. J. Cancer Res. 10 (9), 2742–2751.
Pinero, J., Queralt-Rosinach, N., Bravo, A., Deu-Pons, J., Bauer-Mehren, A., Baron, M., et al. (2015). DisGeNET: a Discovery Platform for the Dynamical Exploration of Human Diseases and Their Genes. Database-the J. Biol. Databases Curation 2015, bav028. doi:10.1093/database/bav028
Puram, S. V., and Rocco, J. W. (2015). Molecular Aspects of Head and Neck Cancer Therapy. Hematology-Oncology Clin. N. Am. 29 (6), 971. doi:10.1016/j.hoc.2015.07.003
Qu, L., Deng, Y., Song, Y., and Jiang, Y. (2016). Combined With Modern Research Discussion Zhongjing Prescription of Tumor. Journal of Liaoning University of Traditional Chinese Medicine.
Rasmussen, J. H., Lelkaitis, G., Hakansson, K., Vogelius, I. R., Johannesen, H. H., Fischer, B. M., et al. (2019). Intratumor Heterogeneity of PD-L1 Expression in Head and Neck Squamous Cell Carcinoma. Br. J. Cancer 120 (10), 1003–1006. doi:10.1038/s41416-019-0449-y
Riess, C., Irmscher, N., Salewski, I., Strueder, D., Classen, C.-F., Grosse-Thie, C., et al. (2021). Cyclin-dependent Kinase Inhibitors in Head and Neck Cancer and Glioblastoma-Backbone or Add-On in Immune-Oncology? Cancer Metastasis Rev. 40 (1), 153–171. doi:10.1007/s10555-020-09940-4
Ru, J., Li, P., Wang, J., Zhou, W., Li, B., Huang, C., et al. (2014). TCMSP: a Database of Systems Pharmacology for Drug Discovery from Herbal Medicines. J. Cheminformatics 6. doi:10.1186/1758-2946-6-13
Rubin Grandis, J., Melhem, M. F., Gooding, W. E., Day, R., Holst, V. A., Wagener, M. M., et al. (1998). Levels of TGF-Alpha and EGFR Protein in Head and Neck Squamous Cell Carcinoma and Patient Survival. J. Natl. Cancer Inst. 90 (11), 824–832. doi:10.1093/jnci/90.11.824
Sandra, F., Matsuki, N. A., Takeuchi, H., Ikebe, T., Kanematsu, T., Ohishi, M., et al. (2002). TNF Inhibited the Apoptosis by Activation of Akt Serine/threonine Kinase in the Human Head and Neck Squamous Cell Carcinoma. Cell Signal 14 (9), 771–778. doi:10.1016/s0898-6568(02)00025-6
Shannon, P., Markiel, A., Ozier, O., Baliga, N. S., Wang, J. T., Ramage, D., et al. (2003). Cytoscape: a Software Environment for Integrated Models of Biomolecular Interaction Networks. Genome Res. 13 (11), 2498–2504. doi:10.1101/gr.1239303
Siegel, R. L., Miller, K. D., Fuchs, H. E., and Jemal, A. (2022). Cancer Statistics, 2022. Ca-a Cancer J. Clin. 72 (1), 7–33. doi:10.3322/caac.21708
Soulieres, D., Senzer, N. N., Vokes, E. E., Hidalgo, M., Agarwala, S. S., and Siu, L. L. (2004). Multicenter Phase II Study of Erlotinib, an Oral Epidermal Growth Factor Receptor Tyrosine Kinase Inhibitor, in Patients with Recurrent or Metastatic Squamous Cell Cancer of the Head and Neck. J. Clin. Oncol. 22 (1), 77–85. doi:10.1200/jco.2004.06.075
Sun, J., Han, T., Yang, T., Chen, Y., and Huang, J. (2021). Interpreting the Molecular Mechanisms of Yinchenhao Decoction on Hepatocellular Carcinoma through Absorbed Components Based on Network Pharmacology. Biomed Res. Int. 2021. doi:10.1155/2021/6616908
Szklarczyk, D., Gable, A. L., Nastou, K. C., Lyon, D., Kirsch, R., Pyysalo, S., et al. (2021). The STRING Database in 2021: Customizable Protein-Protein Networks, and Functional Characterization of User-Uploaded Gene/measurement Sets. Nucleic Acids Res. 49 (D1), D605–D612. doi:10.1093/nar/gkaa1074
Tang, D., Tao, D., Fang, Y., Deng, C., Xu, Q., and Zhou, J. (2017). TNF-alpha Promotes Invasion and Metastasis via NF-Kappa B Pathway in Oral Squamous Cell Carcinoma. Med. Sci. Monit. basic Res. 23, 141–149. doi:10.12659/msmbr.903910
Tang, Y., Li, M., Wang, J., Pan, Y., and Wu, F.-X. (2015). CytoNCA: A Cytoscape Plugin for Centrality Analysis and Evaluation of Protein Interaction Networks. Biosystems 127, 67–72. doi:10.1016/j.biosystems.2014.11.005
Troiani, T., Napolitano, S., Della Corte, C. M., Martini, G., Martinelli, E., Morgillo, F., et al. (2016). Therapeutic Value of EGFR Inhibition in CRC and NSCLC: 15 Years of Clinical Evidence. ESMO open 1 (5), e000088. doi:10.1136/esmoopen-2016-000088
Vivanco, I., and Sawyers, C. L. (2002). The Phosphatidylinositol 3-Kinase AKT Pathway in Human Cancer. Nat. Rev. Cancer 2 (7), 489–501. doi:10.1038/nrc839
Wu, M., Wang, Y., Yang, D., Gong, Y., Rao, F., Liu, R., et al. (2019). A PLK1 Kinase Inhibitor Enhances the Chemosensitivity of Cisplatin by Inducing Pyroptosis in Oesophageal Squamous Cell Carcinoma. Ebiomedicine 41, 244–255. doi:10.1016/j.ebiom.2019.02.012
XiaoMei, C., LiXia, T., and ShunXing, G. (2017). Research Progress on Chemical Constituents and Pharmacological Effects of Sclerotia of Polyporus umbellatus (Polyporaceae, Basidiomycota). Mycosystema 36 (1), 35–47.
Xu, M. J., Johnson, D. E., and Grandis, J. R. (2017). EGFR-targeted Therapies in the Post-genomic Era. Cancer Metastasis Rev. 36 (3), 463–473. doi:10.1007/s10555-017-9687-8
Yao, H., Zhang, Z., Wang, J., Zuo, J., Chen, Y., Zhu, L., et al. (2016). Efficacy and Safety of Yinchenwuling Powder for Hyperlipidemia: a Systematic Review and Meta-Analysis. J. Traditional Chin. Med. 36 (2), 135–143. doi:10.1016/s0254-6272(16)30019-x
Yu, G., Wang, L.-G., Han, Y., and He, Q.-Y. (2012). clusterProfiler: an R Package for Comparing Biological Themes Among Gene Clusters. Omics-a J. Integr. Biol. 16 (5), 284–287. doi:10.1089/omi.2011.0118
Zaryouh, H., De Pauw, I., Baysal, H., Pauwels, P., Peeters, M., Vermorken, J. B., et al. (2021). The Role of Akt in Acquired Cetuximab Resistant Head and Neck Squamous Cell Carcinoma: An In Vitro Study on a Novel Combination Strategy. Front. Oncol. 11. doi:10.3389/fonc.2021.697967
Zhou, J., Wu, Z., Wong, G., Pectasides, E., Nagaraja, A., Stachler, M., et al. (2017). CDK4/6 or MAPK Blockade Enhances Efficacy of EGFR Inhibition in Oesophageal Squamous Cell Carcinoma. Nat. Commun. 8. doi:10.1038/ncomms13897
Keywords: Yinchen Wuling San, head and neck squamous cell carcinoma, network pharmacology, target, molecular docking
Citation: Zhang B, Liu G, Wang X and Hu X (2022) Identification of Molecular Targets and Potential Mechanisms of Yinchen Wuling San Against Head and Neck Squamous Cell Carcinoma by Network Pharmacology and Molecular Docking. Front. Genet. 13:914646. doi: 10.3389/fgene.2022.914646
Received: 07 April 2022; Accepted: 02 May 2022;
Published: 06 July 2022.
Edited by:
Gengming Cai, Fujian Medical University, ChinaReviewed by:
Jing Lijun, First Affiliated Hospital of Zhengzhou University, ChinaQi-zhuang Lv, Yulin Normal University, China
Copyright © 2022 Zhang, Liu, Wang and Hu. This is an open-access article distributed under the terms of the Creative Commons Attribution License (CC BY). The use, distribution or reproduction in other forums is permitted, provided the original author(s) and the copyright owner(s) are credited and that the original publication in this journal is cited, in accordance with accepted academic practice. No use, distribution or reproduction is permitted which does not comply with these terms.
*Correspondence: Xuelei Hu, MDQwMDIxNDhAd2l0LmVkdS5jbg==; Xin Wang, d2FuZ3hpbjAwNzJAMTI2LmNvbQ==