- Sección Genética Evolutiva, Facultad de Ciencias, UdelaR, Montevideo, Uruguay
The term Annual killifish describes a short-lived and amazing group of vertebrates inhabiting temporary ponds exposed to an extremely variable environment during its short lifespan in South America and Africa, leading to the death of the entire adult population during the dry season. Austrolebias is a specious genus of the family Rivulidae, with ∼58 currently recognized species, extensively distributed in the temperate Neotropical region. Herein, we reviewed different aspects of the evolutionary biology with emphasis on the genome dynamic linked to the burst speciation process in this genus. Austrolebias constitutes an excellent model to study the genomic evolutionary processes underlying speciation events, since all the species of this genus analyzed so far share an unusually large genome size, with an average DNA content of 5.95 ± 0.45 picograms per diploid cell (mean C-value of about 2.98 pg). The drastic nuclear DNA–increasing would be associated with a considerable proportion of transposable elements (TEs) found in the Austrolebias genomes. The genomic proportion of the moderately repetitive DNA in the A. charrua genome represents approximately twice (45%) the amount of the repetitive components of the highly related sympatric and syntopic rivulinae taxon Cynopoecilus melanotaenia (25%), as well as from other rivulids and actinopterygian fish. These events could explain the great genome instability, the high genetic diversity, chromosome variability, as well as the morphological diversity in species of Austrolebias. Thus, species of this genus represent new model systems linking different evolutionary processes: drastic genome increase, massive TEs genomic representation, high chromosome instability, occurrence of natural hybridization between sister species, and burst speciation events.
Introduction
It has been proposed that model organisms are widely used in research as accessible and convenient systems to study a particular area or question in biology (Russell et al., 2017). In recent years they have accelerated the proliferation of experimental approaches, such as high-throughput sequencing, CRISPR gene editing, transgenesis, and other technologies which have enabled new insights, particularly when a trait of interest is most readily observed in a non-traditional model organism (Juntti, 2019).
In this sense, annual fish have been focused on as an important model organism as a consequence of an evolutionary adaption to its extreme habitat (Berois et al., 2014; Reichard and Polačik, 2019). This fish group inhabiting temporary ponds in South America and Africa is a unique, short-lived, vertebrate model presenting developmental, ecological, physiological, genetics, and evolutionary peculiar adaptations (García et al., 2014; Loureiro and de Sá, 2016; Berois et al., 2016, Reichard and Polačik, 2019). In particular, Austrolebias is a specious genus of the family Rivulidae, with ∼58 currently recognized species (Froese and Pauly, 2021), extensively distributed in the temperate Neotropical region in two different South American basins (Figure 1A): La Plata-Paraná-Paraguay-Uruguay basin and Patos-Merin system (Costa, 2006; Loureiro et al., 2011; Volcan et al., 2014). Seasonal ponds inhabited by Austrolebias range from rounded to irregularly shaped shallow depressions in grasslands and seasonal flooded areas adjacent to rivers or large permanent wetlands (Loureiro et al., 2016). Seasonal patches strongly push individuals to accommodate spatially to optimize food supply, metabolic efficiency, mating opportunities, and predator avoidance, all in a few months (Wilbur, 1997). Therefore, they are freshwater teleosts exposed to an extremely variable environment during their short lifespan (Berois et al., 2016). During the rainy season the adults reproduce and generate desiccation-resistant embryos that remain buried in the muddy bottom of the dry ponds (Figures 1B–D). The embryos hatch in the next rainy season since the ponds are flooded, whereas the entire adult population dies during the dry season (Berois et al., 2016). The resulting juveniles reach sexual maturity in a few weeks, until a new reproductive cycle begins (Wourms, 1967; Arezo et al., 2005). Therefore, the survival of the species becomes entirely dependent upon buried embryos and this unique life cycle is correlated to the seasonal environments (Berois et al., 2016).
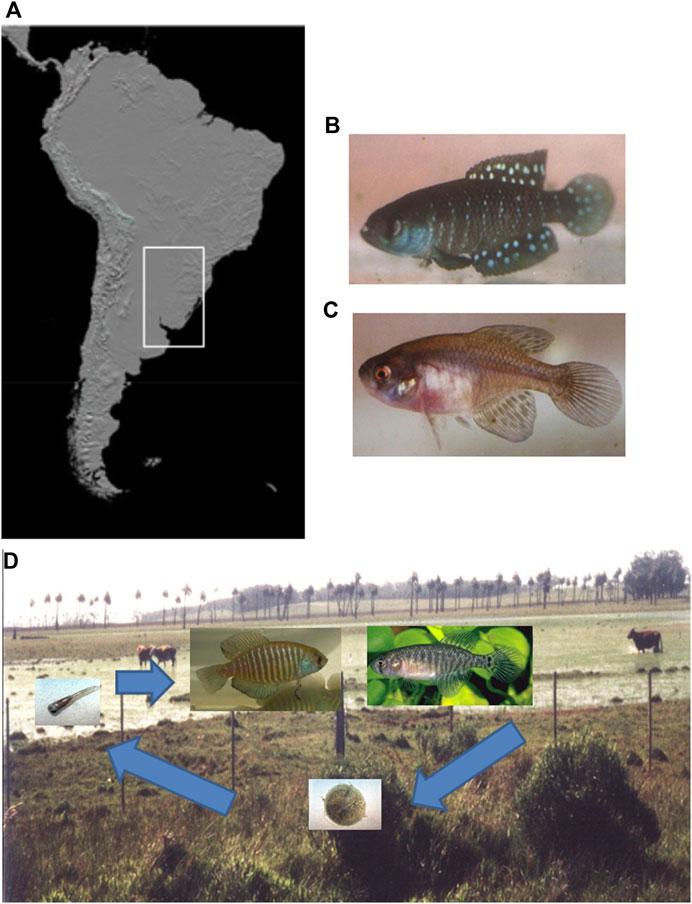
FIGURE 1. Continental occurrence area, specimens, and life cycle of annual fish Austrolebias. (A) Distribution area of the Austrolebias genus in South America (white rectangle). (B) Adult male of the A. affinis species group. (C) Adult female of the A. affinis species group. (D) Life cycle of annual fish Austrolebias in temporary ponds from the R.16, Rocha Department, in Uruguay. A. charrua male (left) and female (right) are shown, the embryos remain in a prehatching stage of developmental arrest and hatch in the next rainy season when the ponds are flooded. The resulting juveniles reach sexual maturity in a few weeks.
One of the strengths of using a model organism is the ability to dispose of all possible information, including different sources of data about the analyzed system, improving the interpretation of results. Currently, studies on the evolutionary biology (García et al., 2002, 2009, 2014, 2019, 2020), systematics and conservation (Loureiro et al., 2018), ethology (Passos et al., 2013), ecology (Arim et al., 2011), reproductive strategies (Arezo et al., 2005, 2014), regulation of developmental pathways (Berois et al., 2016), aging (Gutiérrez, 2014), and neurogenesis (Fernández et al., 2020) on several species of Austrolebias are in progress. Herein, we present different findings about the evolutionary biology of annual fish with emphasis on the genome dynamic and the population genomics linked to the speciation process in the genus Austrolebias.
Living in Extreme Environments Displaying High Variability at Different Levels
Ponds in the temperate region of South America are heterogeneous environments that cover a large range of areas. They have variable depths, contain organic matter, are rich in invertebrates, plant diversity, and biomass, and are usually isolated from other ponds (Laufer et al., 2009; Arim et al., 2011). The fragmentary nature of this kind of habitat can induce the occurrence of evolutionary mechanisms such as genetic drift (Gillespie, 2000), bottlenecks, founder effects, and endogamy (Carvalho and Hauser, 1999) in annual fish. Long-term isolation among population also produces local differentiation driving to high levels of genetic and morphological diversity and endemisms in this South American region (García et al., 2002; Loureiro, 2004; Loureiro et al., 2018). However, previous genetic analyses have proposed that the Austrolebias population behave as a metapopulation. During the rainy season, floods mix the populations across large distances yielding a high intrapopulation genetic and morphological variation (García, 2006; García et al., 2009). The wide range of gene flow values revealed by analyses carried out with mitochondrial and nuclear markers indicated that it is not homogeneous among ponds (García et al., 2019, 2020). In fact, the estimates of gene flow reinforced the hypothesis that the populations under study live in a region with a complex geological history and in an irregular rainy regime yielding to chaotic local dynamics, which facilitate the persistence of such a metapopulation (García, 2006). On the other hand, this area has been affected by sea level fluctuations at least since the Miocene, between 15 and 30 million of years ago (Sprechmann, 1978), while the last transgression being five-thousand years ago (García-Rodriguez and Witkowaki, 2003). In this complex geomorphological scenario in the wetlands from South America the proposed explosive speciation events for the genus Austrolebias would be favored (García et al., 2002, 2014). The evolutionary history together with their peculiar development strategy and short generation time (1 year) could also favor high morphologic and molecular variability among and within ponds (García, 2006). Species size ranges from small (max. 35 mm of standard length) to large (more than 200 mm of standard length) (Huber, 1996; Loureiro and de Sá, 2016). Most large species are considered to form a monophyletic group of top predators (García et al., 2002; Costa, 2006, 2010). Moreover, striking sexual dimorphism has been reported for several species of rivulids fish and most Neotropical annual killifish. In Austrolebias, males are usually larger than females (Figures 1B–C). A study on intra- and intersexual selection revealed that a larger body size favors A. charrua males (Passos et al., 2013).
Karytotypic Divergence Among Species of the Genus Austrolebias
In general, chromosome numbers vary little within and among teleost groups (Mank and Avise, 2006) and do not differ greatly from a widely proposed ancestral karyotype of 48 acrocentric chromosomes (Mank and Avise, 2006). This is also the case for some Neotropical rivulid species that include annual species (Elder et al., 1993; García et al., 1993, 1995, 2001). However, among annual killifish, the chromosome numbers range from 2N = 48 to 2N = 28 with arm numbers (NF) as high as 80 (García et al., 1993, 1995, 2001; García, 2016). In particular, chromosome variation at intra- and interspecific level was earlier described in species of the genus Austrolebias (García et al., 1993, 2001). Based on phylogenetic analysis using mitochondrial genes two major clades within Austrolebias could represent different repatterning pathways of the karyotypes (García et al., 2001; García, 2016). This analysis suggested that Clade I, corresponding to the Austrolebias alexandri-affinis species group, has differentiated basally during the late Miocene (García et al., 2014). This clade is characterized by a 2N = 48, different numbers of NF in each species, and the presence of extralarge acrocentric and subtelocentric chromosomes. Therefore, the occurrence of predominantly pericentric inversions, including perhaps heterochromatic loss/addition was proposed. On the other hand, Clade II is composed of all the remaining species groups clustered in four subclades showing the reduction of the diploid number from 2N = 48 to 2N = 34 and the maintenance of NF values nearly 48. Therefore, other rearrangement types could explain the chromosome evolution in these species groups (García et al., 1993, 2001).
Giant Genomes and Speciation in the Neotropical Genus Austrolebias
Mank and Avise (2006) proposed that the genomes of ray-finned fishes (Actinopterygii) are well known for their evolutionary dynamism as reflected by drastic alterations in DNA content in general via partial or whole-genome duplication. Also, Mable et al. (2011) reported C-values for teleosts ranging between 0.35 and 4.9 pg, with an average of 1.2 pg. An amazing finding revealed that at least 16 species of Austrolebias show a C-value average of about 5.95 ± 0.45 pg/diploid cell (mean C-value of about 2.98 pg). In the same study, the genome size reported in the putative sister sympatric and syntopic taxon C. melanotaenia was of 2.72 ± 0.06 pg/diploid cell (García et al., 2014). Both C-values were corroborated in more recent findings through NGS of the total genome and de novo assembly and RNA-seq. This last analysis revealed haploid genomes size of 3.4 and 1.0 Gb for A. charrua and C. melanotaenia respectively (Valdivieso et al., 2017). The C. melanotaenia value fell within the range of most other cyprinodontoid and rivulid fish genomes, as in the early study in Anablepsoides urophthalmus (Hinegardner and Rosen, 1972), and more recently as in Austrofundulus limnaeus (Wagner et al., 2018), Orestias ascotanensis (Di Genova et al., 2022), and Nematolebias whitei (Thompson et al., 2022). Also, it is consistent with that of 3.11 pg/diploid cell reported in the African annual killifish Nothobranchius furzeri (Reichwald et al., 2009). Therefore, Austrolebias exhibit larger genomes compared to nearly all other reported diploid, i.e., non-(paleo) polyploid species of actinopterygian fishes (García et al., 2014). Previous analyses based on different mitochondrial genes supported the possible occurrence of burst cladogenetic processes in this genus. The sudden speciation in Austrolebias species, was preceded by events of divergence since a hypothetic ancestral rivulid genome which contained approximately 3.0 pg/diploid nucleus and a basal karyotype constituted by 48 small chromosomes of acrocentric type (García et al., 2001, 2002, 2014). These hypothesized events could be occurred since the Quaternary sharing drastic nuclear DNA increasing in all species analyzed, great genome instability and high levels of chromosomal divergence as previously mentioned (García et al., 2002; García, 2006; 2016). Moreover, they could explain the high morphological diversity described in species of the genus (Loureiro and de Sá, 1998; Loureiro et al., 2018).
Massive Enrichment of Transposable Elements and Genome Instability as an Evolutionary Driving Force in Austrolebias
The extensive genetic variation by means of chromosome rearrangements involving both Robertsonian and non-Robertsonian changes at intra- and interspecific levels provides evidence for the genome instability occurring in Austrolebias (García et al., 1993, 2001). Remarkably, the frequency of these different types of chromosomal rearrangements between the two major clades occurred without statistically significant differences in nuclear DNA content. This finding reinforces the hypothesis that all Austrolebias species share similar nuclear DNA content since a hypothetic common ancestor (García, 2016).
Moreover, the drastic nuclear DNA–increasing events found in Austrolebias would be associated with considerable increase in the proportion of TEs. In fact, a comparative analysis of partial repetitive DNA content by means of NGS (New Generation Sequencing) revealed that the proportion of moderately repetitive DNA in A. charrua (45%) is approximately twice than that of the genus C. melanotaenia (25%) (García et al., 2015; Gutiérrez et al., 2016). Both species inhabit the same ponds and are distributed in the same South America temperate region. Most recently, total NGS genome analyses revealed a high number of repetitive elements present in A. charrua (68.1% of the total genome size), the most repetitive genome reported for a teleost (Valdivieso et al., 2017). Similar to the information reported by Chalopin et al. (2015) and Rhee et al. (2017), almost all classes of repetitive DNA are present in many teleosts groups and in the Rivulidae genomes. In the aforementioned comparative partial genome analysis implemented by García et al. (2015) in A. charrua and C. melanotaenia, retroelements make up most of the repetitive DNA. In particular, the A. charrua genome was predominantly enriched by LINE retroelements of the REX-Babar, Jockey, and L2 type (García et al., 2015; Valdivieso et al., 2017). Moreover, Rhee et al. (2017) reported that approximately one-fourth of the highly related mangrove killifish Kryptolebias marmoratus genome is composed of TEs, corroborating the previous finding in C. melanotaenia. Consistently, the most recent genome analysis of O. ascotanensis revealed that 21% (∼142 Mb) correspond to repetitive sequences, including LINE, LTR, and SINES sequences (Di Genova et al., 2022).
These results are in contrast to those reported in other related rivulid species as in the non-annual genome of mangrove killifish in which DNA transposons (approximately 10–14%) are relatively common (Rhee et al., 2017). TEs insertions can be responsible for the disruption of genes or regulatory sequences, and can also cause chromosomal rearrangements, representing a threat to their host genome integrity (Hedges and Deininger, 2007). The importance of TEs in the structure and evolution of vertebrate genomes and their major impact on genome diversity between and within lineages, were revisited by comparing the mobilomes of 23 vertebrate genomes (Chalopin et al., 2015). It was hypothesized that TE activation could promote or intensify morphological and karyotypical changes, some of which may be potentially important for the process of microevolution, and allow species with plastic genomes to survive as new forms or even as species in times of rapid climatic change (Belyayev, 2014). As proposed by this author, the scenario of events preceding speciation by different models in small marginal populations could be as follows: under the influence of unusual ecological conditions, TEs become active; the mobilization of TEs produces genetic variations, epigenetic alterations, and high rates of karyotypic change (including changes in species-specific chromosomal pattern). All these evolutionary hypothetic scenarios would be taken place in the genus Austrolebias.
Whereas the annotation and characterization of the complex genome sequence of the A. charrua and that of C. melanotaenia are in progress (Valdivieso et al., 2017), the comparative analysis of these genomes and mobilomes could help to interpret their possible association with the extensive phenotypic plasticity detected at all levels in species of the genus Austrolebias, adapted to extreme environmental conditions in temporary ponds.
Additional Genomic Revolution and Phenotypic Innovations in Natural Hybrid Swarms of a Hybrid Zone Between Two Austrolebias Species
Most recent population genetics analyses have detected other sources of morphological and genetic variability present in a contact area between two parapatric species A. charrua and A. reicherti (Figure 2A). This hybrid zone is the first described among Neotropical killifish and it was located in the Cebolattí River basin in Patos-Merin coastal lagoon system (García et al., 2019, 2020). The RNA-seq-based sequencing of the transcriptomes from pools of individuals of the two parental species and their putative natural hybrids allowed to identified a set of 111,725 SNP (single nucleotide polymorphism) markers, representing presumably fixed allelic differences among the two species (García et al., 2019). From these detected markers the first panel of 106 SNPs, in a single diagnostic multiplex assay to validate their capacity to reconstruct the patterns of the hybrid zone between both taxa, was performed. High-quality transcriptomes and a large set of gene-linked SNPs greatly facilitate functional and population genomics studies in the hybrid zone of these endangered species. Over the previously characterized bimodal hybrid zone (García et al., 2020) the new approach including more extensive sampling individuals and molecular markers, combined with morphological and biogeographic analyses, detected a population structure in which some groups among the hybrid swarms showed different level of introgression towards one or the other parental species according to their geographic distribution. In fact, after 14 years of fieldwork and laboratory analysis, the present hybrid zone remains localized and spatially reduced to two patches: 1) individuals showed intergradation of the morphological and pigmentation patterns towards A. charrua; 2) individuals morphologically A. reicherti-like. New Hybrid analysis (Figure 2B) suggested that the combination of hybrid genotypes and introgression generated new genomic entities, which are different from both parental taxa. Abundant empirical evidence shows that hybridization frequently leads to transgressive phenotypes in plants, animals, and fungi (Rieseberg et al., 1999; Langdon et al., 2019). Natural introgression between sympatric or parapatric sister species could be considered an in situ conservation strategy (Becker et al., 2013) and Hamilton and Miller (2015) have suggested that genetic variation that persists within natural hybrids may have conservation value. The present results reinforce the importance of the natural hybridization and introgression in the analyzed contact zone between A. charrua and A. reicherti to preserve in situ biodiversity by increasing the observed phenotypic variation in each temporal pond where these endangered killifish populations inhabit. Finally, hybridization between species could cause genomic stress, which can lead to several genome reorganizations that seem to be driven by TEs (Fontdevila, 2005; Michalak, 2009; Romero-Soriano et al., 2017). In this sense, earlier, Barbara McClintock (1984) proposed the genomic shock hypothesis in which the hybridization between two species constitutes a source of stress that could disrupt the control mechanisms of TEs and cause their activation.
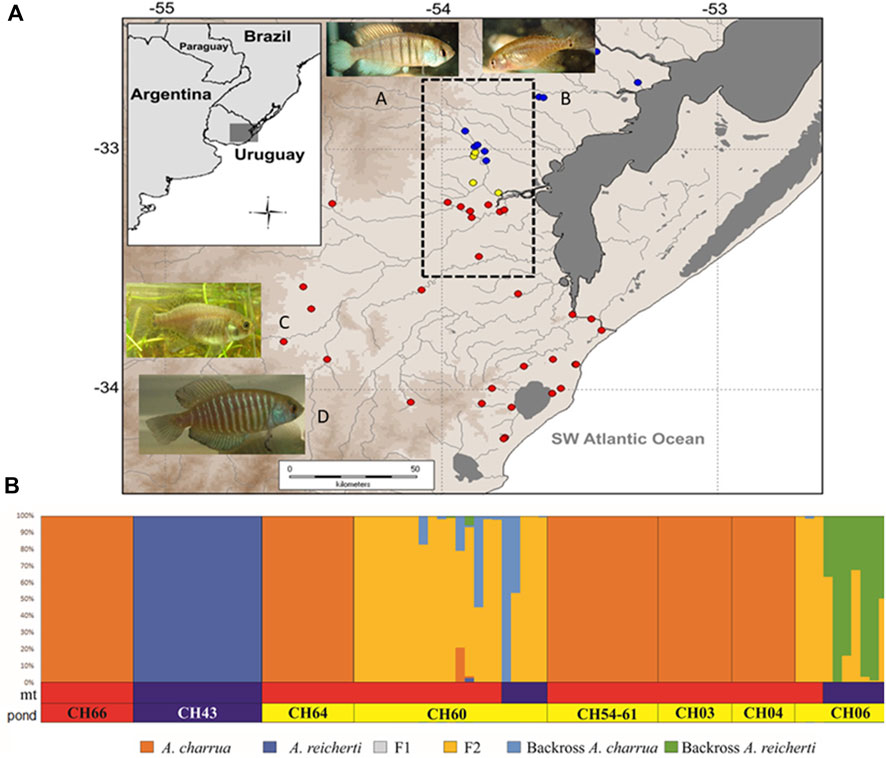
FIGURE 2. Geographic distribution and genotype assignment of individuals sampled in the hybrid zone of the Patos-Merin coastal lagoons basin system, South America. (A) The contact area between both taxa is delimited for the dotted line rectangle. Ponds are represented by circles: A. charrua (red circles), A. reicherti (blue circles) and hybrid populations (yellow circles) In the top, male (A) and female (B) of the parental species A. reicherti; in the bottom, female (C) and male (D) of the parental species A. charrua. (B) Posterior probabilities of the ancestral genotype class estimated with NewHybrids, under Uniform prior assumption. Each individual is represented as a vertical bar divided into six segments. Each color indicates the posterior probability of an individual assignment to pure A. reicherti (blue), pure A. charrua (red), F1 (grey), F2 (yelow), and first generation backcross of a F1 hybrid with a pure A. reicherti (BC1R, green) or with a pure A. charrua (BC1C, sky). Each of the sampled ponds in the hybrid zone are labeled below the bar plots, and named as follows: CH66, CH43, CH64, CH60, CH54-61, CH03, CH04, and CH06. mt (mitochondrial) bars: represent the Cytb haplotype assignation of each individual to A. charrua (red) or A. reicherti (blue) species. Modified from García et al. (2019).
General Considerations and Open Questions
Among different genera of annual and non-annual killifish from the New and Old World, giant genomes (∼6 Gb) were only detected in Austrolebias (García et al., 2015; Valdivieso et al., 2017). Genome amplification has not occurred by polyploidization, since Austrolebias species are true diploids (García et al., 2014). Therefore, how can this formidable increase in genome size in the Neotropical genus Autrolebias be explained? Could it be related to the fact that Austrolebias is the unique rivulid genus that inhabit temperate Neotropical region? Could the extremely variable pond environments under different stress conditions have triggered unexpected TEs mobilization events? Are natural hybridization events between para/sympatric species linked to TEs mobilization in the genomes?
Previous findings in other taxa could contribute to our understanding of the possible underlying mechanisms to explain the existence of giant genomes and their dynamics in the genus Austrolebias. For example, the adaptation of Drosophila species to temperate climates was associated to widespread TEs (González et al., 2010). TEs invasion affected putatively genes which were also highly diverse in terms of their molecular and cellular function. Increasing the genome size as well as the timing of the development could have an impact also on its complexity (Gregory, 2005). Finally, an interesting and additional avenue to explore is the parallelism in the genomic size and the proportion of TEs (particularly, the abundance of LINE-type elements) between the human genome associated with different disorders and diseases and the Austrolebias one. For all the aforementioned issues, Austrolebias genus represents an outstanding species model in eco-evo-devo-research linking different evolutionary processes: drastic genome increase, massive presence of TEs, high chromosome instability, occurrence of natural hybridization between sister species, and the burst speciation process.
Author Contributions
GG wrote the manuscript. All authors contributed to different works included in the article and approved the submitted version.
Funding
The research of GG, VG, and NR was supported by SNI (ANII, Agencia Nacional de Investigación e Innovación). Furthermore, NR was supported by DT (UdelaR, Universidad de la República Oriental del Uruguay), and GG was supported by DT_CSIC_ Project (2002–2019) from UdelaR, Uruguay.
Conflict of Interest
The authors declare that the research was conducted in the absence of any commercial or financial relationships that could be construed as a potential conflict of interest.
Publisher’s Note
All claims expressed in this article are solely those of the authors and do not necessarily represent those of their affiliated organizations, or those of the publisher, the editors and the reviewers. Any product that may be evaluated in this article, or claim that may be made by its manufacturer, is not guaranteed or endorsed by the publisher.
Acknowledgments
We are grateful to grants for molecular research to GG, furnished by Killi-Data Organization and Museum National d’Histoire Naturelle of France (2006–2007). Further, we are grateful to PEDECIBA (Programa de Desarrollo de las Ciencias Básicas) and CONICYT (Consejo Nacional de Innovación, Ciencia y Tecnología) Uruguay, for additional financial support. The authors are grateful to reviewers and to the editor for comments and suggestions improving the manuscript.
References
Arezo, M. J., Papa, N., Gutiérrez, V., García, G., and Berois, N. (2014). Sex Determination in Annual fishes: Searching for the Master Sex-Determining Gene in Austrolebias Charrua (Cyprinodontiformes, Rivulidae). Genet. Mol. Bio 37, 364–374. doi:10.1590/S1415-47572014005000009
Arezo, M. J., Pereiro, L., and Berois, N. (2005). Early Development in the Annual Fish Cynolebias Viarius. J. Fish. Bio. 66, 1357–1370. doi:10.1111/j.0022-1112.2005.00688.x
Arim, M., Berazategui, M., Barreneche, J. M., Ziegler, L., Zarucki, M., and Abades, S. R. (2011). Determinants of Density-Body Size Scaling within Food Webs and Tools for Their Detection. Adv. Ecol. Res. 45, 1–43. doi:10.1016/B978-0-12-386475-8.00001-0
Becker, M., Gruenheit, N., Steel, M., Voelcke, C., Deusch, O., Heenan, P. B., et al. (2013). Hybridization May Facilitate in situ Survival of Endemic Species through Periods of Climate Change. Nat. Clim. Chang., 3. 1039–1043. Letters. doi:10.1038/nclimate2027
Belyayev, A. (2014). Bursts of Transposable Elements as an Evolutionary Driving Force. J. Evol. Biol. 27, 2573–2584. doi:10.1111/jeb.12513
Berois, N., Arezo, M. J., and de Sá, R. O. (2014). The Neotropical Genus Austrolebias: An Emerging Model of Annual Killifishes. Annu. Rev. Cell Dev. Biol. 3, 1–9. doi:10.1146/annurev.cellbio.3.1.1
Berois, N., Arezo, M. J., Papa, N. G., and Chalar, C. (2016). “Life Cycle, Reproduction, and Development in Annual Fishes: Cellular and Molecular Aspects,” in Annual Fishes: Life History Strategy, Diversity, and Evolution. Editors N. Berois, G. García, and R. De Sá (Florida: CRC Press: Francis & Taylor Group), 33–45. ISBN 9780367575311.
Carvalho, G. R., and Hauser, L. (1999). Molecular Markers and the Species Concept: New Techniques Toresolve Old Disputes? Rev. Fish. Biol. Fish. 9, 379–382.
Chalopin, D., Naville, M., Plard, F., Galiana, D., and Volff, J. N. (2015). Comparative Analysis of Transposable Elements Highlights Mobilome Diversity and Evolution in Vertebrates. Genome Biol. Evol. 7, 567–580. doi:10.1093/gbe/evv005
Costa, W. J. E. M. (2010). Historical Biogeography of Cynolebiasine Annual Killifishes Inferred from Dispersal-Vicariance Analysis. J. Biogeogr. 37, 1995–2004. doi:10.1111/j.1365-2699.2010.02339.x
Costa, W. J. E. M. (2006). The South American Annual Killifish Genus Austrolebias(Teleostei: Cyprinodontiformes: Rivulidae): Phylogenetic Relationships, Descriptive Morphology and Taxonomic Revision. Zootaxa 1213, 1–162. doi:10.11646/zootaxa.1067.1.1
Di Genova, A., Nardocci, G., Maldonado-Agurto, R., Hodar, C., Valdivieso, C., Morales, P., et al. (2022). Genome Sequencing and Transcriptomic Analysis of the Andean Killifish Orestias Ascotanensis Reveals Adaptation to High-Altitude Aquatic Life. Genomics 114, 305–315. doi:10.1016/j.ygeno.2021.12.018
Elder, J. F., Bruce, J. T., Thomerson, J. E., and Taphorn, D. C. (1993). Karyotypes of Nine Venezuel Anannual Killifishes (Cyprinodontidae) with Comments on Karyotype Differentiation in Annual Killifishes. Ichthyol. Explor. Freshwaters 4, 261–268.
Fernández, A. S., Rosillo, J. C., Berrosteguieta, I., Herrera, M. L., and Torres-Pérez, M. (2020). The Ciliary Marginal Zone of the Retina of Austrolebias Charrua Fish is a Highly Proliferative Neurogenic Niche. Microsc. Microanal. 26, 171–172. doi:10.1017/S1431927620001075
Fontdevila, A. (2005). Hybrid Genome Evolution by Transposition. Cytogenet. Genome Res. 110, 49–55. doi:10.1159/000084937
Froese, R., and Pauly, D. (2021). FishBase. World Wide Web Electronic Publication. Available at: www.fishbase.org.version.(08/2021).
García, G., Gutiérrez, V., Ríos, N., Papa, N., Serra, S., and Loureiro, M. (2020). Characterization of a Hybrid Zone between Two Annual Killifish Genus Austrolebias from the Biosphere Reserve and Ramsar Sites in South America. Hydrobiologia 847, 421–442. doi:10.1007/s10750-019-04104-0
García, G., Alvarez-Valin, F., and Gómez, N. (2002). Mitochondrial Genes: Signals and Noise inPhylogenetic Reconstruction within Killifish Genus Cynolebias (Cyprinodontiformes, Rivulidae). Biol. J. Linn. Soc. 76, 49–59. doi:10.1111/j.1095-8312.2002.tb01713.x
García, G. (2016). “Chromosome Evolution, Divergent Meiotic Pathways, and Speciation in the Family Rivulidae,” in Annual Fishes: Life History Strategy, Diversity, and Evolution. Editors N. Berois, G. García, and R. De Sá (Florida: CRC Press: Francis & Taylor Group), 275–287. ISBN 9780367575311.
García, G., Gutiérrez, V., Ríos, N., Turner, B., Santiñaque, F., López-Carro, B., et al. (2014). Burst Speciation Processes and Genomic Expansion in the Neotropical Annual Killifish Genus Austrolebias (Cyprinodontiformes, Rivulidae). Genetica 142, 87–98. doi:10.1007/s10709-014-9756-7
García, G., Lalanne, A. I., Aguirre, G., and Cappetta, M. (2001). Chromosome Evolution in Annual Killifish Genus Cynolebias and Mitochondrial Phylogenetic Analysis. Chromosome Res. 9, 93–100. doi:10.1023/a:1011664009509
García, G., Loureiro, M., Berois, N., Arezo, M. J., Casanova, G., and Olivera, A. (2009). Pattern of Differentiation in the Annual Killifish Genus Austrolebias (Cyprinodontiformes: Rivulidae) from a Biosphere Reserve Site in South America: a Multidisciplinary Approach. Biol. J. Linn. Soc. 98, 620–635. doi:10.1111/j.1095-8312.2009.01303.x
García, G. (2006). Multiple Simultaneous Speciation in Killifishes of the Cynolebias Adloffi Speciescomplex (Cyprinodontiformes, Rivulidae) from Phylogeography and Chromosome Data. J. Zool. Syst. Evol. Res. 44, 75–87. doi:10.1111/j.1439-0469.2005.00346.x
García, G., Ríos, N., and Gutiérrez, V. (2015). Next-generation Sequencing Detects Repetitive Elements Expansion in Giant Genomes of Annual Killifish Genus Austrolebias (Cyprinodontiformes, Rivulidae). Genetica 143, 353–360. doi:10.1007/s10709-015-9834-5
García, G., Ríos, N., Gutiérrez, V., Serra, S., and Loureiro, M. (2019). Transcriptome-Based SNP Discovery and Validation in the Hybrid Zone of the Neotropical Annual Fish Genus Austrolebias. Genes (Basel). 10, 789. doi:10.3390/genes10100789
García, G., Scvortzoff, E., and Hernández, A. (1995). Karyotypic Heterogeneity in South American Annual Killifishes of the Genus Cynolebias (Pisces, Cyprinodontiformes, Rivulidae). Cytologia 60, 103–110.
García, G., Scvortzoff, E., Maspoli, M. C., and Vaz-Ferreira, R. (1993). Analysis of Karyotypic Evolution in Natural Populations of Cynolebias (Pisces: Cyprinodontiformes, Rivulidae) Using Banding Techniques. Cytologia 58, 85–94.
García-Rodriguez, F., and Witkowaki, A. (2003). Inferring Sea Level Variation from Relative Percentages of Pseudopodosira Kosugii in Rocha Lagoon, SE Uruguay. Diatom. Res. 18, 49–59. doi:10.1080/0269249X.2003.9705572
Gillespie, J. H. (2000). Genetic Drift in an Infinite Population: the Pseudohitchhiking Model. Genetics 155, 909–919. doi:10.1093/genetics/155.2.909
González, J., Karasov, T. L., Messer, P. W., and Petrov, D. A. (2010). Genome-Wide Patterns of Adaptation to Temperate Environments Associated with Transposable Elements in Drosophila. PLOS Genet. 6, e1000905. doi:10.1371/journal.pgen.1000905
Gregory, T. R. (2005). Synergy between Sequence and Size in Largescale Genomics. Nat. Rev. Genet. 6, 699–708. doi:10.1038/nrg1674
Gutiérrez, V. (2014). Patterns of Molecular Senescence in the Neotropical Annnual Killifish Genus Austrolebias. UdelaR, Uruguay: Facultad de Ciencias. Umpublished Ph.D. Dissertation Thesis PEDECIBA.
Gutiérrez, V., Ríos, N., and García, G. (2016). “Genome of the Annual Fishes Austrolebias Dynamized byTransposable Elements,” in Annual Fishes: Life History Strategy, Diversity, and Evolution. Editors N. Berois, G. García, and R. De Sá (Florida: CRC Press: Francis & Taylor Group), 245–251. ISBN 9780367575311.
Hamilton, J. A., and Miller, J. M. (2015). Adaptive Introgression as a Resource for Management and Geneticconservation in a Changing Climate. Conserv. Biol. 30, 33–41. doi:10.1111/cobi.12574
Hedges, D. J., and Deininger, P. L. (2007). Inviting Instability: Transposable Elements, Double-Strand Breaks, and the Maintenance of Genome Integrity. Mutat. Research/Fundamental Mol. Mech. Mutagen. 616, 46–59. doi:10.1016/j.mrfmmm.2006.11.021
Hinegardner, R., and Rosen, D. E. (1972). Cellular DNA Content and Evolution of Teleostean Fishes. Am. Nat. 106, 621–644.
Huber, J. H. (1996). Updated Checklist of Taxonomic Names, Collecting Localities and Bibliographic References of Oviparous Cyprinodont Fishes, Bibliographic References of Oviparous Cyprinodont Fishes (Atherinomorpha, Pisces). Killi-Data 1996. Paris, France: Société Française d'Ichtyologie, Muséum National d'Histoire Naturelle, 399.
Juntti, S. (2019). The Future of Gene-Guided Neuroscience Research in Non-Traditional ModelOrganisms. Brain Behav. Evol. 93, 108–121. doi:10.1159/000500072
Langdon, Q. K., Peris, D., Baker, E. P., Opulente, D. A., Nguyen, H. V., Bond, U., et al. (2019). Fermentation Innovation through Complex Hybridization of Wild and Domesticated Yeasts. Nat. Ecol. Evol. 3, 1576–1586. doi:10.1038/s41559-019-0998-8
Laufer, G., Arim, M., Loureiro, M., Piñeiro-Guerra, J. M., Clavijo-Baquet, S., and Fagúndez, C. (2009). Diet of Four Annual Killifishes: An Intra and Interspecific Comparison. Neotrop. Ichthyol. 7, 77–86. doi:10.1590/S1679-62252009000100010
Loureiro, M., Borthagaray, A., Hernández, D., Duarte, A., Pinelli, V., and Arim, M. (2016). “Austrolebias in Space Scaling from Ponds to Biogeographical Regions,” in Annual Fishes: Life History Strategy, Diversity, and Evolution. Editors N. Berois, G. García, and R. De Sá (Florida: CRC Press: Francis & Taylor Group), 111–132. ISBN 9780367575311.
Loureiro, M., de Sá, R. O., Berois, N., García, G., and De Sá, R. (2016). “Diversity of Aplocheiloidei,” in Annual Fishes: Life History Strategy, Diversity, and Evolution (Florida: CRC Press: Francis & Taylor Group), 3–31. ISBN 9780367575311.
Loureiro, M., and de Sá, R. O. (1998). Osteological Analysis of the Killifish Genus Cynolebias (Cyprinodontiformes: Rivulidae). J. Morphol. 238, 109–262.
Loureiro, M., de Sá, R. O., Serra, S. W., Alonso, F., Krause Lanés, L. E., Volcan, M. V., et al. (2018). Review of the Family Rivulidae (Cyprinodontiformes, Aplocheiloidei) and a Molecular and Morphological Phylogeny of the Annual Fish Genus Austrolebias Costa 1998. Neotrop. Ichthyol. 16, e180007. doi:10.1590/1982-0224-20180007
Loureiro, M., Duarte, A., and Zarucki, M. (2011). A New Species of Austrolebias Costa (Cyprinodontiformes: Rivulidae) from Northeastern Uruguay, with Comments on Distribution Patterns. Neotrop. Ichthyol. 9, 335–342. doi:10.1590/S1679-62252011000200010
Loureiro, M. (2004). Sistemática y biogeografía de los peces anuales de la subtribu Cynolebiatina(Cyprinodontiformes: Rivulidae: Cynolebiatinae). Montevideo: Universidad de la República, 119. Unpublished Ph.D. Thesis, PEDECIBA.
Mabble, B. K., Alexandrou, M. A., and Taylor, M. I. (2011). Genome Duplication in Amphibians and Fish: An Extended Synthesis. J. Zoology 284, 151–182. doi:10.1111/j.1469-7998.2011.00829.x
Mank, J. E., and Avise, J. C. (2006). Cladogenetic Correlates of Genomic Expansions in the Recent Evolution of Actinopterygiian Fishes. Proc. R. Soc. B 273, 33–38. doi:10.1098/rspb.2005.3295
McClintock, B. (1984). The Significance of Responses of the Genome to Challenge. Science 226, 792–801. doi:10.1126/science.15739260
Michalak, P. (2009). Epigenetic, Transposon and Small RNA Determinants of Hybrid Dysfunctions. Heredity 102, 45–50. doi:10.1038/hdy.2008.48
Passos, C., Tassino, B., Loureiro, M., and Rosenthal, G. G. (2013). Intra- and Intersexual Selection on Male Body Size in the Annual Killifish Austrolebias Charrua. Behav. Process 96, 20–26. doi:10.1016/j.beproc.2013.01.008
Reichard, M., and Polacik, M. (2019). Nothobranchius Furzeri, an ’instant’ Fish from an Ephemeral Habitat. eLife 8, e41548. doi:10.7554/eLife.41548
Reichwald, K., Lauber, C., Nanda, I., Kirschner, J., Hartmann, N., Schories, S., et al. (2009). High Tandem Repeat Content in the Genome of the Shortlived Annual FIsh Nothobranchius Furzeri: A New Vertebrate Model for Aging Research. Genome Biol. 10, R16. doi:10.1186/gb-2009-10-2-r16
Rhee, J.-S., Choi, B.-S., Kim, J., Kim, B.-M., Lee, Y.-M., Kim, I.-C., et al. (2017). Diversity, Distribution, and Significance of Transposable Elements in the Genome of the Only Selfing Hermaphroditic Vertebrate Kryptolebias Marmoratus. Sci. Rep. 7, 40121. doi:10.1038/srep40121
Rieseberg, L., Archer, M., and Wayne, R. (1999). Transgressive Segregation, Adaptation and Speciation. Heredity 83, 363–372 .doi:10.1038/sj.hdy.688617
Romero-Soriano, V., Modolo, L., Lopez-Maestre, H., Mugat, B., Pessia, E., Chambeyron, S., et al. (2017). Transposable Element Misregulation Is Linked to the Divergence between Parental piRNA Pathways in Drosophila Hybrids. Genome Biol. Evol. 9, 1450–1470. doi:10.1093/gbe/evx091
Russell, J. J., Theriot, J. A., Sood, P., Marshall, W. F., Landweber, L. F., Fritz-Laylin, L., et al. (2017). Non-model Model Organisms. BMC Biol. 15, 55. doi:10.1186/s12915-017-0391-5
Sprechman, P. (1978). The Paleoecology and Paleogeography and Stratigraphy of the Uruguayan Coastal área during the Neogene and Quaternary. Zitteliana 4, 3–72.
Thompson, A. W., Wojtas, H., Davoll, M., and Braasch, I. (2022). Genome of the Rio Pearlfish (Nematolebias Whitei), a Bi-annual Killifish Model for Eco-Evo-Devo in Extreme Environments. G3, Genes,Genomes, Genet. 12 (5), jkac045. doi:10.1093/g3journal/jkac045
Valdivieso, C., Pereiro, L., Di Genova, A., Gajardo, F., García, G., Arezo, M. J., et al. (2017). “The Genome Sequence of the Annual Killifish Austrolebias Charrua Reveals Mechanisms of Genomic Expansion via Retrolelements,” in IX Latin American Society for Developmental Biology Meeting (Colombia: Medellín), 192.)
Volcan, M. V., Gonçalves, A. C., and Lanés, L. E. K. (2014). Austrolebias Quirogai (Actinopterygii: Cyprinodontiformes: Rivulidae) in Brazil: Occurence, Population Parameters, Habitat Characteristics, and Conservation Status. Acta Ichtyol. Pisc. 44, 37–44. doi:10.3750/AIP2014.44.1.05
Wagner, J. T., Singh, P. P., Romney, A. L., Riggs, C. L., Minx, P., Woll, S. C., et al. (2018). The Genome of Austrofundulus Limnaeus Offers Insights into Extreme Vertebrate Stress Tolerance and Embryonic Development. BMC Genomics 19, 155. doi:10.1186/s12864-018-4539-7
Wilbur, H. M. (1997). Experimental Ecology of Food Webs: Complex Systems in Temporary Ponds. Ecology 78, 2279–2302. doi:10.2307/2265892
Keywords: neotropical, killifish, Austrolebias, giant genomes, evolutionary model
Citation: García G, Gutiérrez V and Ríos N (2022) Living in Temporary Ponds Loading Giant Genomes: The Neotropical Annual Killifish Genus Austrolebias as New Outstanding Evolutionary Model. Front. Genet. 13:903683. doi: 10.3389/fgene.2022.903683
Received: 24 March 2022; Accepted: 05 May 2022;
Published: 20 June 2022.
Edited by:
Tony Silveira, Federal University of Rio Grande, BrazilReviewed by:
Daiana Kaster Garcez, Federal University of Santa Maria, BrazilLuis Esteban Krause Lanés, Instituto Pró-Pampa, Brazil
Copyright © 2022 García, Gutiérrez and Ríos. This is an open-access article distributed under the terms of the Creative Commons Attribution License (CC BY). The use, distribution or reproduction in other forums is permitted, provided the original author(s) and the copyright owner(s) are credited and that the original publication in this journal is cited, in accordance with accepted academic practice. No use, distribution or reproduction is permitted which does not comply with these terms.
*Correspondence: Graciela García, Z2dhcmNpYUBmY2llbi5lZHUudXk=
†Present Address: Verónica Gutiérrez, Departamento de Biodiversidad y Genética, Instituto de Investigaciones Biológicas Clemente Estable, Ministerio de Educación y Cultura, Montevideo, Uruguay