- 1Division of Cellular and Molecular Diagnostics (Molecular Biology Group), ICMR- National Institute of Cancer Prevention and Research, Noida, India
- 2Symbiosis School of Biological Sciences, Symbiosis International (Deemed University), Pune, India
- 3Laboratory Oncology Unit, Dr. B. R. A. Institute Rotary Cancer Hospital, All India Institute of Medical Sciences, New Delhi, India
The global cancer cases and mortality rates are increasing and demand efficient biomarkers for accurate screening, detection, diagnosis, and prognosis. Recent studies have demonstrated that variations in epigenetic mechanisms like aberrant promoter methylation, altered histone modification and mutations in ATP-dependent chromatin remodelling complexes play an important role in the development of carcinogenic events. However, the influence of other epigenetic alterations in various cancers was confirmed with evolving research and the emergence of high throughput technologies. Therefore, alterations in epigenetic marks may have clinical utility as potential biomarkers for early cancer detection and diagnosis. In this review, an outline of the key epigenetic mechanism(s), and their deregulation in cancer etiology have been discussed to decipher the future prospects in cancer therapeutics including precision medicine. Also, this review attempts to highlight the gaps in epigenetic drug development with emphasis on integrative analysis of epigenetic biomarkers to establish minimally non-invasive biomarkers with clinical applications.
Introduction
Cancer is a multifactorial disease developed as a result of several genetic as well as epigenetic changes. Epigenetics is a process that involves the alteration of gene expression without changing the DNA sequence. It is a Greek word meaning above or over the genome, which was coined by Conard Waddington in 1942 (Waddington, 2012). The process of epigenetics involves structural modifications within the nucleic acids and histones imparting a different chromatin structure and includes three molecular mechanisms like DNA methylation, histone modification, and nucleosome modelling patterns (Ganesan et al., 2019). These epigenetic modifications involve several chemical alterations which are induced by a group of enzymes, called epigenetic tools or players. The enzymes which participate in chemical addition to DNA or histones are known as “writers” whereas “erasers” are those enzymes that are involved in removing chemical tags. All these modifications are interpreted by a separate group of enzymes called ‘readers’ (Biswas and Rao, 2018). Several processes like DNA repair, replication, transcription, translation, post-transcriptional and post-translational regulation, are controlled by epigenetics (Dawson and Kouzarides, 2012). Thus, aberrant expression patterns or epigenomic alterations can lead to misregulation, culminating in cancers (Lu et al., 2020). The interesting part of studying epigenetics is that it is reversible in nature as compared to genetic changes and they only alter how a DNA sequence is read (Jaenisch and Bird, 2003). There are several factors contributing to epigenetic changes in humans like obesity, diet, lifestyle, alcohol, tobacco use, exposure to electromagnetic radiation and environmental pollutants like chromium, cadmium, nickel, benzene, mercury, and arsenic (Jones and Baylin, 2002; Baccarelli and Bollati, 2009; Metere and Graves, 2020).
Research over a decade has focused on promoter DNA methylation and histone modifications as the two main molecular mechanisms that mediate the process of epigenetic regulation in anticancer therapies and biomarker discovery. Studies have demonstrated that the altered DNA methylation genes or patterns can be potentially used as biomarkers for proper cancer screening, diagnosis, and prognosis (Tost, 2009; Karandish and Mallik, 2016; Grayson et al., 2019). Thus, epigenetic biomarker discovery is crucial for early cancer diagnosis, better cancer therapies, precise treatment and effective clinical outcomes. In spite of continuous growth in the discovery and development of biomarkers, advancement in the clinical validation of the approved biomarker is still demanded (Hussain et al., 2022). Many challenges are being faced in the development of a reliable biomarker with clinical applications. One main issue is the incorporation of clinical trial data into routine practice with affordable cost, which is only possible through interdisciplinary collaboration between researchers, clinicians and diagnostics companies.
In addition, the tumour microenvironment (TME) of the cancer cells contains aberrant epigenetic marks which are known to cause a favorable environment for tumor growth (Lodewijk et al., 2021). The existing literature not only emphasizes research on epigenetic regulation and its role in cancer development but also on the interaction of tumour cells with TME. In the context of the above-mentioned facts, this review has shed light on epigenetic changes and the use of integrated network medicine with epigenetics in the development of epimarkers/epidrugs along with several challenges faced during their development. The administration of such anticancer therapies might lead to reverse epigenetics which could be useful in the treatment and management of cancer patients.
Mechanisms of epigenetic modification
Several molecular mechanisms exist behind epigenetic regulation, including DNA and RNA methylation, histone modifications, and ATP dependent nucleosome remodelling which have been discussed here.
DNA and RNA methylation
DNA methylation is one of the widely studied epigenetic mechanisms in cancer etiopathogenesis. Aberrant methylation leads to DNA hypermethylation or hypomethylation. In DNA hypermethylation, the process of methylation occurs at the cytosine bases present in the promoter region of genes by a group of enzymes called DNA methyltransferases (DNMTs), including DNMT1, DNMT3a, and DNMT3b (Jones and Baylin, 2002). These enzymes convert cytosine residues to 5-methylcytosine eventually leading to decreased gene expression via transcriptional suppression (Fan et al., 2012; Li et al., 2012). On the contrary, DNA hypomethylation indicates overall decrease in the methylation levels as compared to normal cells, and affects the intergenic and intronic regions of the DNA, resulting in chromosomal instability and increased mutation activities (Wilson et al., 2007). The global hypomethylation with hypermethylation of specific gene promoters has already been reported by various studies on cancer (Kurkjian et al., 2008). Thus, inappropriate DNA methylation may lead to altered expression of tumor suppressor genes (deregulation) and/or oncogenes (upregulation) in cancer cells (Kulis and Esteller, 2010). In fact, differences in methylation patterns exist within CpG islands of ∼70% of all mammalian promoters, which have been known to play an important role in transcriptional and post-transcriptional regulation (Robertson, 2005; Tost, 2009). In addition, the introduction of high throughput sequencing has confirmed that 5–10% of abnormally methylated CpG promoter islands are present in various cancer genomes. Also, the hypermethylation of CpG islands in several promoters influences the expression of various noncoding RNAs (ncRNA) as well as messenger RNAs (mRNA), which are known to have a role in cancer progression (Baylin and Jones, 2011). Even, the whole genome sequencing data in several cancers have shown that various somatic mutations exhibit in numerous epigenetic regulators (Forbes et al., 2017).
A less studied epigenetic process is RNA methylation. It is about seven times greater than DNA methylation. These modifications result in mRNA localization and transcript degradation (Zaccara et al., 2019). With the advent of next generation sequencing and the discovery of RNA methylation-related proteins, it is easy to comprehend that methyl modifications at mRNA level may affect the cellular processes resulting in human diseases. Presently, over 150 different RNA modifications have been observed, of which the N6-methyladenosine (m6A) modification is the most abundant and it is recognized by RNA binding proteins that affect many characteristics of mRNA function (Schwartz et al., 2014; Linder et al., 2015). Similar to modifications at the DNA methylation level, alterations at RNA level affect the epigenetic regulation of gene expression (Figure 1).
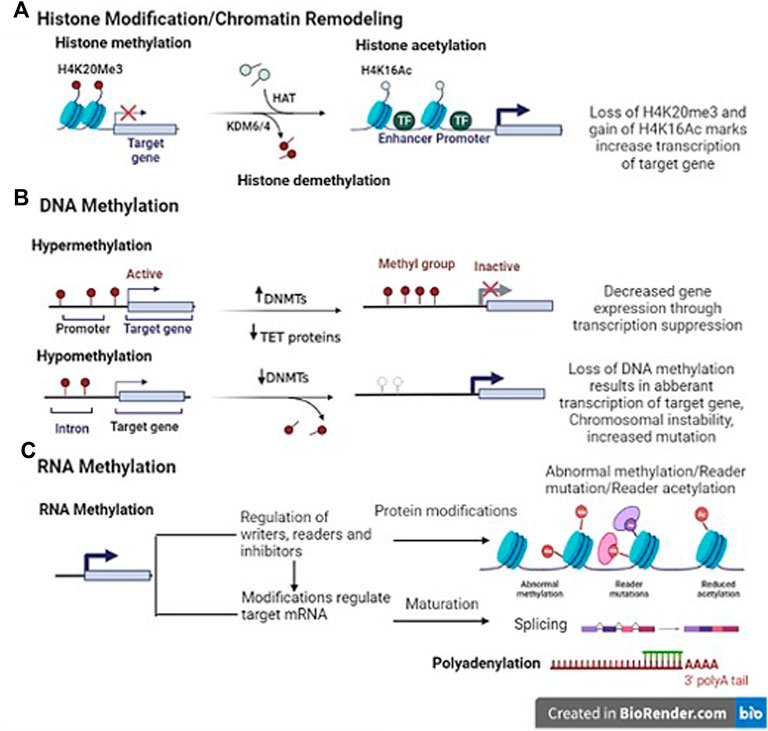
FIGURE 1. Representative image of epigenetic mechanisms (Template was created by free Biorender.com). (A) Histone modification- Histone methyltransferases (HMT) add methyl groups to histones (H4K20Me3). Histone demethylases (HDM)/Lysine demethylase (KDM6/4) remove these methyl groups. It is associated with both gene expression and silencing. Histone acetylation; The addition of an acetyl group on H3K9Ac (lysine 9 histone H3) in enhancer/promoter region by histone acetylase (HAT) enzyme. Histone deacetylase (HDAC) interact with transcriptional repressor (TR) to remove the modifications. (B) DNA methylation- DNA methyltransferases (DNMTs) add methyl group in the promoter region of genes. On the contrary, DNA hypomethylation indicates overall decrease in the methylation levels as compared to normal cells, and affects the intergenic and intronic regions of the DNA, resulting in chromosomal instability and increased mutation events (C) RNA methylation- Indirect translational repression by miRNA causes deadenylation, in which the 3′ poly(A) tail of an mRNA is removed, leading to increased mRNA degradation. The miRNA–mRNA interaction can lead to several modes of direct translational repression.
Histone modification
We are already familiar with the chromatin structure which involves wrapping of DNA on histone octamer -2 subunits each of H2A, H2B, H3 and H4 proteins joined together by H1 proteins. Histone modification takes place at the amino-terminal tail of these histones via the process of acetylation, methylation, phosphorylation, ADP-ribosylation, or ubiquitination. Several enzymes are known to catalyse the above-mentioned processes. The addition of acetyl, methyl, phosphate group etc. to histone amino-terminal tail is performed by histone acetyltransferases (HATs), histone methyltransferases (HMTs), and histone kinases. These epigenetic marks are known as writers and act as transcriptional co-activators. On the contrary, erasers [histone deacetylases (HDACs), histone demethylases (HDMs), phosphatases] function as transcriptional co-repressors by removing these groups from histone end (Li et al., 2020). All these enzymes are involved in the simultaneous opening and closing of chromatin structure, which is necessary for gene expression to occur (Figure 1). Aberrations in these enzymes may lead to altered gene transcription and post-transcriptional modifications, thereby resulting in cancer.
ATP dependent chromatin remodelling
The DNA nucleosome interactions can be modified (histone ejection, removal and incorporation) through chromatin remodelling complexes by ATP hydrolysis. These chromatin-remodelling complexes can be classified into switching defective/sucrose nonfermenting (SWI/SNF), chromodomain-helicase DNA-binding protein (CHD), imitation SWI (ISWI), and INOsitol requiring mutant 80 (INO80) complexes. The catalytic subunit of these complexes performs DNA translocation along with the histone core of the nucleosome (Clapier et al., 2017).
The SWI/SNF complexes are one of the most widely studied ATP dependent chromatin remodelling complexes. They are found to be mutated in 25% of human cancers (Mittal and Roberts, 2020) and are playing an essential role in chromatin remodelling by positioning nucleosomes. Their catalytic activity is known to be associated with SMARCA4/2 proteins. Numerous studies also suggest that SWI/SNF complexes are involved in the regulation of cell progression, cell motility, and nuclear hormone signalling (Wilson and Roberts, 2011). The SWI/SNF complex was found to be altered in 33–42% of pancreatic cancer cases by whole-exome sequencing studies (Shain et al., 2012; Witkiewicz et al., 2015).
The next important complex is ISWI which mobilizes nucleosomes by helping the transcription factors to bind a nucleosome-free DNA. Unlike SWI/SNF complex, ISWI is held to nucleosomes by a SANT and a SLIDE domain (Grüne et al., 2003). As reported in the literature, ISWI complexes have a key role in DNA repair and recombination (Aydin et al., 2014). In humans, two ISWI subunits namely sucrose nonfermenting 2L (SNF2L) and sucrose nonfermenting 2H (SNF2H) ATPases are identified. It has been noticed that SNF2H suppressed the oncogene ras in human cells (Andersen et al., 2006). A tissue microarray study on 78 paraffin wax-embedded prostatic tissues observed a significant increase in ISWI (SNF2L and SNF2H) proteins in prostatic intraepithelial neoplasia and prostate adenocarcinoma (Mohamed et al., 2007). To date, no clinical trials have been performed to unravel the potential of these small molecules as epigenetic biomarkers in cancer therapies.
Epigenetic diagnostic biomarkers
Epigenetic changes like DNA methylation and histone modification detected in early tumorigenesis and cancer progression have been proposed as biomarkers for early cancer detection, tumor prognosis, and treatment response (Figure 2). They are rarely translated into biomarkers for clinical practice, even though there have been major advances in the characterization of cancer. Due to stability in body fluids like urine and serum, which have a great opportunity for assay development to assistance in patient’s treatment, the epigenetic changes act as innovative cancer biomarkers. Recent studies have identified various epigenetic cancer biomarkers that have already been commercialized. However, further validation studies are required to take it to the clinics. Over here, epigenetic diagnostic and prognostic biomarkers that are most promising for the most common cancers have been discussed (Figure 3).
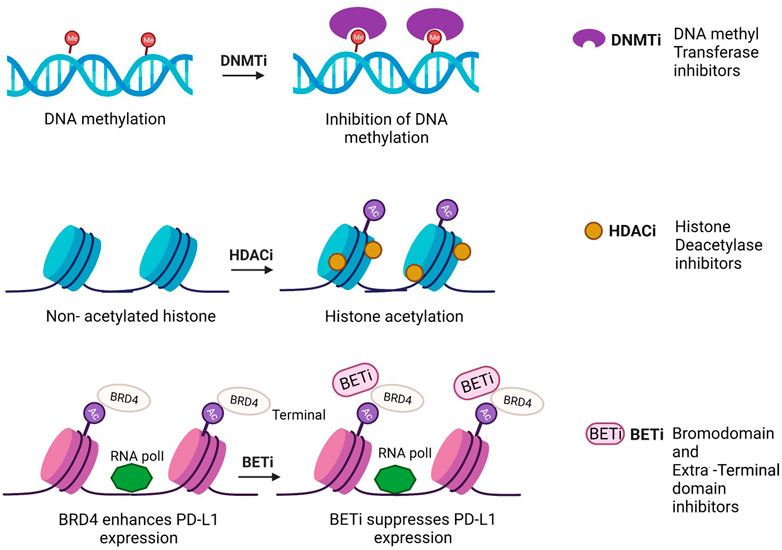
FIGURE 2. Mechanism of action of epidrugs in anticancer therapies [Icons were created by Biorender.com (accessed on February 9th 2022)].
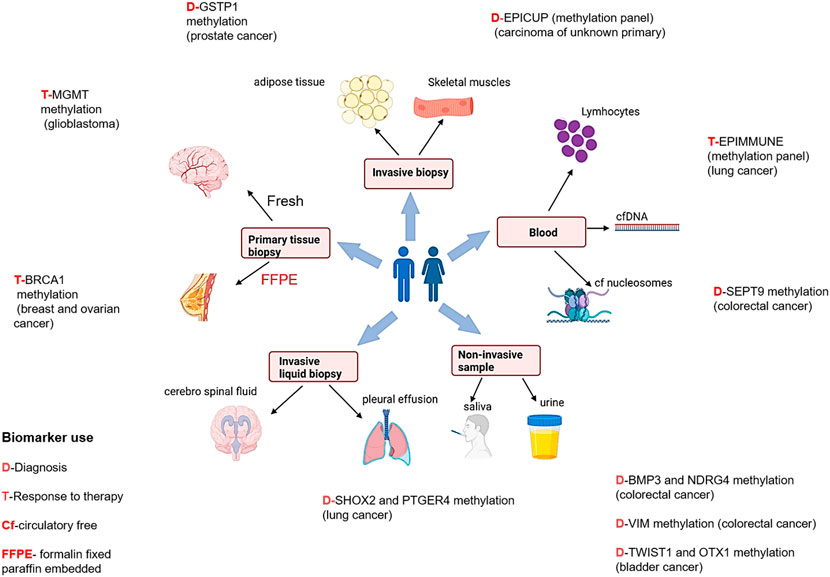
FIGURE 3. Epigenetic biomarkers and different sample types for diagnosis, prognosis, and treatment therapies in different cancers [Icons were created by Biorender.com (accessed on January 28th 2022)].
Prostate cancer
Prostate cancer is the second most common cancer (14.8%) in men and the fourth leading cause of cancer related death (6.6%) (Sung et al., 2021), globally. Prostate cancer is quite heterogenous and lethal disease at clinical level, which makes it impervious not only to diagnose it early but also for evaluating the threat that a given prostate cancer bears to its host (Jain et al., 2014). Therefore, further studies are required to develop epigenetic biomarkers to meet these goals. In 95% of prostate cancer patients, higher expression of PCA3 and ncRNA have extensively been reported in blood samples. Commercially available PROGENSA™, prostate cancer biomarker (Durand et al., 2011) quantifies PCA3 expression ratio normalized as input control for prostate specific antigen (PSA) mRNA. For early detection of prostate cancer by semi non-invasive method, PCA3 testing may be useful, thus also avoiding unnecessary prostate biopsy. The most promising prostate cancer epigenetic biomarkers are DNA methylation and glutathione S-transferase pi gene (GSTP1) promoter hypermethylation (Maldonado et al., 2014).
Glioblastoma
Few studies have reported a correlation of promoter methylation at the methylguanine-DNA methyltransferase (MGMT) gene with favorable treatment outcomes in glioblastoma patients treated with temozolamide, suggesting its possibility to be used as an epigenetic biomarker (Donson et al., 2007; Rosas-Alonso et al., 2021; Kim et al., 2022).
Colorectal cancer
Studies have shown that high levels of hypermethylated DNA exists in colorectal cancer which leads to genomic instability (Toyota et al., 1999). The methylation status of five genes- CACNA1G, IGF2, NEUROG1, RUNX3, and SOCS126 may identify CpG island methylator phenotype (CIMP) positive colon cancers, which are characterized by high incidence of p16 and THBS1 methylation and frequent KRAS and BRAF mutations (Lao and Grady, 2011; Zhang et al., 2021). One of our published studies observed that RASSF1A, FHIT and MGMT gene methylation patterns may be used as markers in diagnosing colorectal cancer (Sinha et al., 2013).
Esophageal cancer
A recent epigenomic study has shown differential methylation patterns in several genes which may account for esophageal cancer development and in future can be realised as diagnostic biomarkers (Lin et al., 2018). An Indian study observed promoter methylation in 52% of histopathologically confirmed tumor tissues and the methylation frequency increased with higher histological grades of the cancer (p = 0.0001) (Salam et al., 2009).
Bladder cancer
In 2021 (Sung et al., 2021), the incidence rate of bladder cancer was 3.3%, and the mortality rate of 2.0% globally. At present no accurate diagnostic or prognostic biomarkers are commercially available. Some biomarkers representing higher sensitivity than cytology (Letelier et al., 2012) have been reported based on methylation. As per some studies, based on genome-wide characterization, bladder cancer cells demonstrated that VIM, GDF15, and TMEFF2 show 94% sensitivity and 100% specificity in urine samples (Costa et al., 2010). The data of other epigenetic alterations like histone modification in bladder cancer is infrequent.
Breast Cancer
Several studies have suggested alterations in histone-modifying enzymes like enhancer of zeste homolog 2(EZH2). This enzyme is encoded by EZH2 gene, which participates in histone-methylation and transcriptional repression. EZH2 has significantly reduced expression of histone in breast cancer (Yomtoubian et al., 2020). Another one is Protein Arginine Methyltransferase (PRMT1). It is also a histone modifying enzyme which plays an important role in the epithelial-to-mesenchymal transformation (EMT) of breast cancer cells (Mathioudaki et al., 2011) in the development of cetuximab sensitivity in triple-negative breast cancer (TNBC) cell lines (Gurdal et al., 2019) with high grade malignancy and poor prognosis (Bianchini et al., 2016). The role of SETD7 (SET Domain Containing 7), lysine methyl transferase in post translational modification of non-histone protein and having prognostic as well as negative effects with tumorigenesis and poor prognosis in patients (Huang et al., 2017) with the expression of lysine methyltransferase SETD7 has been suggested. It is a potential promoter of the antioxidant pathway balancing the cytotoxic effect of oxidative stress. Further validation, for histone modification-based biomarkers is still required.
Stirazaker et al., 2015 divided TNBCs into different epigenetics groups viz. high, intermediate, and low-risk groups based on epigenetic subtypes and the methylated region that is correlated with the progression of the disease. One of the recent studies has also confirmed a correlation between shorter periods of reduction in analysed TNBC-samples (Stirzaker et al., 2015) and hypermethylation of gene regions. Hypermethylation provides explanations and evidence for clinical threat and helps in treatment planning in patients having a higher risk of recurrence.
Ovarian cancer
The worldwide incidence and mortality rates of ovarian cancer in the year 2021 were 1.1% and 2.3%, respectively (Sung et al., 2021). Histone modifications by acetylation with aberrant tubulin protein expression, reduction of PACE3 expression, silencing of survivin (BIRC5), upregulation of pRb tumor suppressor gene and CDKN1 (Cyclin-dependent Kinase) were reported in ovarian tumor formation. Furthermore, the overexpression of Histone Deacetylase Enzymes HDAC3, and loss of H3K27me3 (an epigenetic modification of DNA histone protein H3) was reported to be associated with prognosis (Li et al., 2021) and higher stages of tumor in ovarian cancer. H3K4me3 plays an important role in the transcriptional repression of tumor necrosis factor TNFRSF11B and upregulate the H3K27me3 (Chapman-Rothe et al., 2013). The loss of RNF20 (Ring Finger Protein 20) and H2Bub1 (H2B monoubiquitination) to the progression of ovarian tumors by chromatin remodelling has been reported by a very recent study (Hooda et al., 2019). Tang et al. (2018) revealed that AMPK (Activated Protein Kinase) moderate the repression of H3K27me3 after treatment with metformin and expressed its usefulness in the treatment of ovarian cancer cases. A study also reported that EZH2 facilitates TIMP2 (Tissue Inhibitor of Metalloproteinase 2) and ADP-Ribosylarginine hydrolase 1 (ARH1) by DNA methylation and H3K27me3, which leads to ovarian cancer metastasis. Their inhibitors could thus be used as potential epigenetic biomarkers for the early detection and diagnosis of cancer after proper clinical studies and validation of the same.
Research has recognized the role of epigenetics in developing drug resistance thereby affecting cancer treatment. Cacan et al. (2016) reported that the loss of apoptosis antigen 1 expression impacts drug resistance, which is mediated by histone deacetylase 1 (HDAC1) in chemoresistant ovarian cancer cells (Cacan, 2016). Using ChIP-sequencing, Curry et al. (2018) identified H3K27me3 and H3K4me3 methyltransferases in the promoter region in tumor cases which were acquired resistance for pre and post platinum and showed that these genes are involved in epigenetic silencing during chemotherapies and are prone to hypermethylation thus providing novel awareness to prevent disclosure of drug resistance (Curry et al., 2018).
A recent study has described hypomethylation of developmental genes MSX1, DAXX and TMEM88. mRNA expression of these developmental genes is associated with platinum resistance and inversely correlated with promoter methylation in ovarian cancer patients by treatment with Guadecitabine (DNA methyl transferase inhibitor) and cisplatin (Bonito et al., 2016; de Leon et al., 2016).
DNA methylation regulates epithelial mesenchymal transition (EMT) by lncRNA (HOTAIR) and it is a sign of resistance to carboplatin (Singh et al., 2019). A study has also described how DNA methylation targeted genome scale strategies could prevent the formation of tumors, for example Guadecitabine facilitated inducing hypomethylation, activates tumor suppressor genes and affects metabolic and immune responses to contributing platinum drug desensitization in ovarian cancer. It may help in improving of patients survival outcomes with ovarian cancer (Fang et al., 2018). An epigenetic study described that the methylation of Zinc Finger protein 671 (ZNF671) can serve as a prognosticator for the early relapse of ovarian tumorigenesis and correlates with disease aggressiveness and progression (Zhang et al., 2019). Epigenetic inhibitors used for combinational therapies, would possibly be most effective by repair of pathways associated with drug response for chemo-desensitization of resistant tumors and would consequently implicate improved survival outcomes as well as personalized treatment for various cancers.
Epigenome-targeted therapies
Quite a few epidrugs are approved for the treatment of several cancers. These epidrugs are the inhibitors of DNA methyltransferase (DNMTi) and histone deacetylase (HDACi) enzymes (Figure 4). The first US-FDA approved epigenetic drug is 5- azacitidine (Azacitidine), a DNMTi which is used in the treatment of myelodysplastic syndromes (MDS) and acute myeloid leukaemia (AML). Even combination therapies including both DNMTi and HDACi are widely inspected in the treatment of MDS, AML and chronic myelomonocytic leukaemia (CMML) (Blagitko-Dorfs et al., 2019). However, clinical results for such a combination of inhibitors are controversial (Thurn et al., 2011). The major reason being the lack of large sized cohort studies. Now, the research on epidrug development has expanded its boundary to targeted therapy, shifting the focus on the presence of activating mutations in epigenetic players, especially histone methyltransferases. It has been found that the evolutionarily conserved histone modifier EZH2 is mutated in several cancers. Another inhibitor of EZH2, Tazemetostat (TAZVERIK, Epizyme, Inc.) was approved by US- FDA in June 2020 for treating adult patients with relapsed or follicular lymphoma with EZH2 positive mutations.
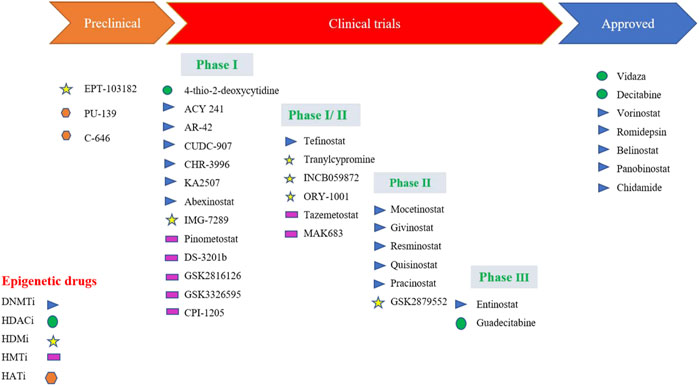
FIGURE 4. Epidrugs in preclinical and clinical trials for cancer therapy [Icons were created by Biorender.com]. (DNMTi - DNA methyl Transferase; HDACi—Histone deacetylase inhibitor; - HMTi -Histone methyltransferase inhibitors; HDMi- Histone demethylase inhibitors and HATi -Histone acetyltransferase inhibitors).
One of the main problems in the application of epidrugs is that the drug binds to other targets rather than its own target. This is called “off-target effects” in epigenetic therapy. Growing epi-research has shown that the use of synthetic lethal approaches might result in apoptosis. In this approach, two inactive genes which synergistically result in apoptosis are selected and combined. These epidrugs are delivered to target synthetic lethal partners having genetic mutations in cancer cells. However, these drugs are less/not toxic to non-cancerous cells with no mutations, resulting in more precise therapy. One such epidrug is the inhibitor of the histone methyltransferase DOT1L (disrupter of telomere silencing 1-like), Pinometostat which specifically kills the MLL-fusion leukaemia cells (Marcos-Villar and Nieto, 2019). Another epidrug used in the treatment of lung cancer with a specific DNA hypomethylation is GSK2879552. It is an inhibitor of lysine-specific histone demethylase 1A (LSD1) (Smitheman et al., 2019). GSK2879552 treatment results in the increase of H3K4 methylation, thereby reducing tumour potential (Fang et al., 2019).
Interestingly, DNA methylation biomarker technology is being employed in circulating free DNA present in body fluids to detect cancers. In the year 2017, “Epi proLung®” assay has received the Conformité Européenne (CE) mark as In Vitro Diagnostic (IVD) test for lung cancer diagnosis. It is based on methylation analysis of SHOX2 (Short Stature Homeobox 2) and PTGER4 (the prostaglandin E receptor 4) genes (Beltrán-García et al., 2019). Numerous reports have shown increased promoter methylation of SEPT9, Vimentin, and NDRG4 gene in colorectal cancer. The US- Food and Drug Administration (FDA) has also approved non-invasive DNA methylation tests of these genes for early colorectal cancer screening programmes (Ned et al., 2011; Lamb and Dhillon, 2017).
Another CE-IVD marked test, miRpredX-31-3p kit (IntegraGen S.A., France) is based on the quantification of miR-31-3p expression levels. It is used to recognise metastatic colorectal cancer patients who can benefit from anti-EGFR (epidermal growth factor receptor) therapy (Ramon et al., 2018). Thus, an effective evolution of epidrugs in cancer therapeutics can be seen from inhibitors to combination therapies to non-invasive diagnostic assays. However, the area of epigenetics still needs to be explored in precision oncology for effective cancer treatment and management.
Epigenetics and integrated network medicine
The future of epidrug development involves the use of integrated network medicine with epigenetics, where several analytical methods like protein-protein interaction (PPI) networks, correlation-based networks and gene regulatory networks are utilized to roll out key genes, relevant regulatory and co-regulatory networks in causing disease pathogenesis (Silverman et al., 2020; Sarno et al., 2021). A group at Stanford University, United States has developed the Genomic Regions Enrichment of Annotations Tool (GREAT) for functional enrichment analysis of DNA binding events across the entire genome, which is useful in identifying gene-regulatory networks and subnetworks in epigenomics data analysis (McLean et al., 2010). Another integrative epigenome-transcriptome-interactome tool called Functional Epigenetic Modules (FEM), identified HAND2 methylation as an important epigenetic alteration in the development of endometrium cancer (Jones et al., 2013; Jiao et al., 2014). In addition, integrative analysis on epigenetic modifications and their effect on gene expression can be performed using Epigenetic Module based on Differential Networks (EMDN) algorithm (Ma et al., 2017). These frameworks could be utilized directly from epigenomic data to unravel co-regulatory networks responsible for causing the disease.
Zheng et al. (2020), used deep neural network (DNN) algorithm to predict cancer diagnosis in the DNA methylation data of 7,339 patients of 18 different cancer origins from The Cancer Genome Atlas (TCGA) (Zheng and Xu, 2020). Recently, weighted correlation network analysis (WGCNA) of 201 patients in a TCGA prostate cancer dataset revealed hypermethylation of FOXD1 might promote poor prognosis (Zhang et al., 2020). Another study yielded 13 genes epigenetic signature that stratified breast cancer patients into low and high-risk groups by using WGCNA analysis and single sample gene set enrichment analysis (ssGSEA) (Bao et al., 2019). One more tool named SWItchMiner (SWIM) is being used to identify potential therapeutic targets when applied to large panel of cancer datasets from TCGA (Paci et al., 2017).
Thus, network medicine might advance the field of epigenomics as it is possible to rule out the co-regulatory networks of DNA methylation (Figure 5). However, its clinical application is still lacking accompanied by the challenges of integration of epigenomics data in multi-omics.
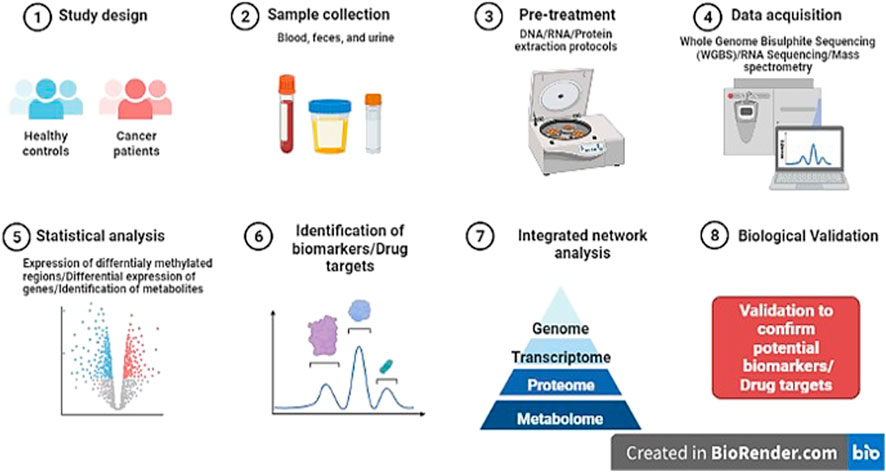
FIGURE 5. Diagrammatic representation of research pipeline on the discovery of novel potential epi-markers in human cancers (Template was created by free Biorender.com).
Challenges and way forward for epidrug development
Epidrug development is accompanied by its own set of challenges that needs to be addressed for the establishment of locus-specific, highly sensitive and cost-effective biomarkers. The main obstacle is to comprehend the “casualty” of epigenetics, meaning that whether the epigenetic abnormality is a result of malignancy or malignancy itself is caused due to these variations. It is crucial to decipher the link between epigenetic differences and cancer progression to establish a biomarker with potential utility in the cancer clinics. This is only possible by pursuing special cohort studies where epigenome profiling can be maintained before the start and after the end of the disease. The high cost of such studies limits their application.
Another challenge is the lack of locus specificity which might cause epigenome-wide “off-target” effects leading to the loss of important gene function and can be resolved using new epigenome editing approaches (Du et al., 2015). With the introduction of proteolysis targeting chimera (PROTAC) drug design approach, epidrugs specific to genetically altered chromatin players can be developed, thereby offering precise cancer therapies approach in treatment (Majchrzak-Celinska et al., 2021).
It is understandable that the epigenetic changes, especially the methylation patterns are very informative in establishing both diagnostic and prognostic biomarkers. But the problem lies in the complex assay systems, imprecise reproducibility, inadequate clinical validation, and false discovery of these biomarkers (Lorincz, 2011). Therefore, implementing clinical epigenetics for the benefit of public health is the main goal of epigenetics research. However, it is restricted due to the variable cellular composition of epigenomic profiles of bulk cell populations. To resolve this issue, single-cell methods should be undertaken to provide resolution of DNA at single-cell level like 5-methylcytosine or 5-hydroxymethylcytosine. Also, computational algorithms can be used to correct variable cellular composition by comparisons to reference epigenomes. Another biological challenge is the limited knowledge of complex epigenome with small sized studies. In addition to the epigenome, epitranscriptome should be studied to rule out potentially modified RNA molecules in cancer, whose potential as epigenetic marks can be exploited before clinical application (Salam et al., 2009). As epigenetic modifications are dynamic, it is vital to consider all epigenetics layers using multiomics approaches along with integrated network medicine for epidrug development.
Conclusion
It is well established that there is a link between cancer and epigenetics. Some epigenetic drugs have already been approved by US-FDA and many more epidrugs are under development for appropriate cancer detection and treatment. Furthermore, there is a scope for epigenetics-based cancer therapies delineating the tumor heterogeneity in different cancers with precision, that should focus on cell-cell behaviour in TME. In addition, epigenetic research should focus on network and precision medicine approaches for the discovery of novel biomarkers so that they can be safely translated to the clinic after proper clinical trials. Thus, proper identification of the epigenetic landscape behind the cancer progression and establishing therapeutic drugs is the future of epigenetics in cancer without forgetting to overcome the challenges faced in effective epidrug development.
Author contributions
ST wrote the first draft of the manuscript. MA wrote the section “Epigenetic biomarkers for cancer diagnosis”. SS made the figures. AK Literature search and checked plagiarism via iThenticate software. AC made the figures. PT contributed to conception and design of the study. SH contributed to conception and design of the study. All authors contributed to manuscript revision and approved the submitted version.
Acknowledgments
The authors are grateful to the Indian Council of Medical Research (ICMR), New Delhi, India (Sanction No. 3/2/3/21/2020/NCD-III) for providing fellowship to ST.
Conflict of interest
The authors declare that the research was conducted in the absence of any commercial or financial relationships that could be construed as a no conflict of interest.
Publisher’s note
All claims expressed in this article are solely those of the authors and do not necessarily represent those of their affiliated organizations, or those of the publisher, the editors and the reviewers. Any product that may be evaluated in this article, or claim that may be made by its manufacturer, is not guaranteed or endorsed by the publisher.
References
Andersen, E. C., Lu, X., and Horvitz, H. R. (2006). C. elegans ISWI and NURF301 antagonize an Rb-like pathway in the determination of multiple cell fates. Development 133 (14), 2695–2704. doi:10.1242/dev.02444
Aydin, Ö. Z., Vermeulen, W., and Lans, H. (2014). ISWI chromatin remodeling complexes in the DNA damage response. Cell Cycle 13 (19), 3016–3025. doi:10.4161/15384101.2014.956551
Baccarelli, A., and Bollati, V. (2009). Epigenetics and environmental chemicals. Curr. Opin. Pediatr. 21 (2), 243–251. doi:10.1097/mop.0b013e32832925cc
Bao, X., Anastasov, N., Wang, Y., and Rosemann, M. (2019). A novel epigenetic signature for overall survival prediction in patients with breast cancer. J. Transl. Med. 17 (1), 380. doi:10.1186/s12967-019-2126-6
Baylin, S. B., and Jones, P. A. (2011). A decade of exploring the cancer epigenome - biological and translational implications. Nat. Rev. Cancer 11 (10), 726–734. doi:10.1038/nrc3130
Beltrán-García, J., Osca-Verdegal, R., Mena-Mollá, S., and García-Giménez, J. L. (2019). Epigenetic IVD tests for personalized precision medicine in cancer. Front. Genet. 10, 621. doi:10.3389/fgene.2019.00621
Biswas, S., and Rao, C. M. (2018). Epigenetic tools (The Writers, the Readers and the Erasers) and their implications in cancer therapy. Eur. J. Pharmacol. 837, 8–24. doi:10.1016/j.ejphar.2018.08.021
Bianchini, G., Balko, J. M., Mayer, I. A., Sanders, M. E., and Gianni, L. (2016). Triple-negative breast cancer: Challenges and opportunities of a heterogeneous disease. Nat. Rev. Clin. Oncol. 13 (11), 674–690. doi:10.1038/nrclinonc.2016.66
Blagitko-Dorfs, N., Schlosser, P., Greve, G., Pfeifer, D., Meier, R., Baude, A., et al. (2019). Combination treatment of acute myeloid leukemia cells with DNMT and HDAC inhibitors: Predominant synergistic gene downregulation associated with gene body demethylation. Leukemia 33 (4), 945–956. doi:10.1038/s41375-018-0293-8
Bonito, N. A., Borley, J., Wilhelm-Benartzi, C. S., Ghaem-Maghami, S., and Brown, R. (2016). Epigenetic regulation of the homeobox gene MSX1 associates with platinum-resistant disease in high-grade serous epithelial ovarian cancer. Clin. Cancer Res. 22 (12), 3097–3104. doi:10.1158/1078-0432.CCR-15-1669
Cacan, E. (2016). Histone deacetylase-1-mediated suppression of FAS in chemoresistant ovarian cancer cells. Anticancer Res. 36 (6), 2819–2826.
Chapman-Rothe, N., Curry, E., Zeller, C., Liber, D., Stronach, E., Gabra, H., et al. (2013). Chromatin H3K27me3/H3K4me3 histone marks define gene sets in high-grade serous ovarian cancer that distinguish malignant, tumour-sustaining and chemo-resistant ovarian tumour cells. Oncogene 32 (38), 4586–4592. doi:10.1038/onc.2012.477
Clapier, C. R., Iwasa, J., Cairns, B. R., and Peterson, C. L. (2017). Mechanisms of action and regulation of ATP-dependent chromatin-remodelling complexes. Nat. Rev. Mol. Cell Biol. 18 (7), 407–422. doi:10.1038/nrm.2017.26
Costa, V. L., Henrique, R., Danielsen, S. A., Duarte-Pereira, S., Eknaes, M., Skotheim, R. I., et al. (2010). Three epigenetic biomarkers, GDF15, TMEFF2, and VIM, accurately predict bladder cancer from DNA-based analyses of urine samples. Clin. Cancer Res. 16 (23), 5842–5851. doi:10.1158/1078-0432.CCR-10-1312
Curry, E., Zeller, C., Masrour, N., Patten, D. K., Gallon, J., Wilhelm-Benartzi, C. S., et al. (2018). Genes predisposed to DNA hypermethylation during acquired resistance to chemotherapy are identified in ovarian tumors by bivalent chromatin domains at initial diagnosis. Cancer Res. 78 (6), 1383–1391. doi:10.1158/0008-5472.CAN-17-1650
Dawson, M. A., and Kouzarides, T. (2012). Cancer epigenetics: From mechanism to therapy. Cell 150 (1), 12–27. doi:10.1016/j.cell.2012.06.013
de Leon, M., Cardenas, H., Vieth, E., Emerson, R., Segar, M., Liu, Y., et al. (2016). Transmembrane protein 88 (TMEM88) promoter hypomethylation is associated with platinum resistance in ovarian cancer. Gynecol. Oncol. 142 (3), 539–547. doi:10.1016/j.ygyno.2016.06.017
Donson, A. M., Addo-Yobo, S. O., Handler, M. H., Gore, L., and Foreman, N. K. (2007). MGMT promoter methylation correlates with survival benefit and sensitivity to temozolomide in pediatric glioblastoma. Pediatr. Blood Cancer 48 (4), 403–407. doi:10.1002/pbc.20803
Du, Q., Luu, P. L., Stirzaker, C., and Clark, S. J. (2015). Methyl-CpG-binding domain proteins: Readers of the epigenome. Epigenomics 7 (6), 1051–1073. doi:10.2217/epi.15.39
Durand, X., Moutereau, S., Xylinas, E., and De La Taille, A. (2011). Progensa™ PCA3 test for prostate cancer. Expert Rev. Mol. Diagn. 11 (2), 137–144. doi:10.1586/erm.10.122
Fan, R., Zhang, L. Y., Wang, H., Yang, B., Han, T., Zhao, X. L., et al. (2012). Methylation of the CpG island near SOX7 gene promoter is correlated with the poor prognosis of patients with myelodysplastic syndrome. Tohoku J. Exp. Med. 227 (2), 119–128. doi:10.1620/tjem.227.119
Fang, F., Cardenas, H., Huang, H., Jiang, G., Perkins, S. M., Zhang, C., et al. (2018). Genomic and epigenomic signatures in ovarian cancer associated with Re-sensitization to platinum drugs. Cancer Res. 78 (3), 631–644. doi:10.1158/0008-5472.CAN-17-1492
Fang, Y., Liao, G., and Yu, B. (2019). LSD1/KDM1A inhibitors in clinical trials: Advances and prospects. J. Hematol. Oncol. 12 (1), 129–214. doi:10.1186/s13045-019-0811-9
Forbes, S. A., Beare, D., Boutselakis, H., Bamford, S., Bindal, N., Tate, J., et al. (2017). COSMIC: Somatic cancer genetics at high-resolution. Nucleic Acids Res. 45 (D1), D777-D783–83. doi:10.1093/nar/gkw1121
Ganesan, A., Arimondo, P. B., Rots, M. G., Jeronimo, C., and Berdasco, M. (2019). The timeline of epigenetic drug discovery: From reality to dreams. Clin. Epigenetics 11 (1), 174. doi:10.1186/s13148-019-0776-0
Grayson, K., Gregory, E., Khan, G., and Guinn, B-A. (2019). Urine biomarkers for the early detection of ovarian cancer - are we there yet? Biomark. Cancer 11, 1179299X19830977. doi:10.1177/1179299X19830977
Grüne, T., Brzeski, J., Eberharter, A., Clapier, C. R., Corona, D. F. V., Becker, P. B., et al. (2003). Crystal structure and functional analysis of a nucleosome recognition module of the remodeling factor ISWI. Mol. Cell 12 (2), 449–460. doi:10.1016/s1097-2765(03)00273-9
Gurdal, H., Tuglu, M. M., Bostanabad, S. Y., and Dalkiliç, B. (2019). Partial agonistic effect of cetuximab on epidermal growth factor receptor and Src kinase activation in triple-negative breast cancer cell lines. Int. J. Oncol. 54 (4), 1345–1356. doi:10.3892/ijo.2019.4697
Hooda, J., Novak, M., Salomon, M. P., Matsuba, C., Ramos, R. I., MacDuffie, E., et al. (2019). Early loss of histone H2B monoubiquitylation alters chromatin accessibility and activates key immune pathways that facilitate progression of ovarian cancer. Cancer Res. 79 (4), 760–772. doi:10.1158/0008-5472.CAN-18-2297
Huang, R., Li, X., Yu, Y., Ma, L., Liu, S., Zong, X., et al. (2017). SETD7 is a prognosis predicting factor of breast cancer and regulates redox homeostasis. Oncotarget 8 (55), 94080–94090. doi:10.18632/oncotarget.21583
Hussain, S., Tulsyan, S., Dar, S. A., Sisodiya, S., Abiha, U., Kumar, R., et al. (2022). Role of epigenetics in carcinogenesis: Recent advancements in anticancer therapy. Semin. Cancer Biol. 83, 441–451. doi:10.1016/j.semcancer.2021.06.023
Jaenisch, R., and Bird, A. (2003). Epigenetic regulation of gene expression: How the genome integrates intrinsic and environmental signals. Nat. Genet. 33 (3), 245–254. doi:10.1038/ng1089
Jain, S., Saxena, S., and Kumar, A. (2014). Epidemiology of prostate cancer in India. Meta Gene 2, 596–605. doi:10.1016/j.mgene.2014.07.007
Jiao, Y., Widschwendter, M., and Teschendorff, A. E. (2014). A systems-level integrative framework for genome-wide DNA methylation and gene expression data identifies differential gene expression modules under epigenetic control. Bioinformatics 30 (16), 2360–2366. doi:10.1093/bioinformatics/btu316
Jones, A., Teschendorff, A. E., Li, Q., Hayward, J. D., Kannan, A., Mould, T., et al. (2013). Role of DNA methylation and epigenetic silencing of HAND2 in endometrial cancer development. PLoS Med. 10 (11), e1001551. doi:10.1371/journal.pmed.1001551
Jones, P. A., and Baylin, S. B. (2002). The fundamental role of epigenetic events in cancer. Nat. Rev. Genet. 3 (6), 415–428. doi:10.1038/nrg816
Karandish, F., and Mallik, S. (2016). Biomarkers and targeted therapy in pancreatic cancer. Biomark. Cancer 8 (1), 27–35. doi:10.4137/BiC.s34414
Kim, M., Yoo, J., Chang, J. H., and Kim, S. H. (2022). Association of MGMT gene promoter methylation with clinicopathological parameters in patients with wild-type IDH glioblastoma. Anticancer Res. 42 (1), 335–341. doi:10.21873/anticanres.15490
Kulis, M., and Esteller, M. (2010). DNA methylation and cancer. Adv. Genet. 70 (C), 27–56. doi:10.1016/B978-0-12-380866-0.60002-2
Kurkjian, C., Kummar, S., and Murgo, A. J. (2008). DNA methylation: Its role in cancer development and therapy. Curr. Probl. Cancer 32 (5), 187–235. doi:10.1016/j.currproblcancer.2008.08.002
Lamb, Y. N., and Dhillon, S. (2017). Epi proColon ® 2.0 CE: A blood-based screening test for colorectal cancer. Mol. Diagn. Ther. 21 (2), 225–232. doi:10.1007/s40291-017-0259-y
Lao, V. V., and Grady, W. M. (2011). Epigenetics and colorectal cancer. Nat. Rev. Gastroenterol. Hepatol. 8 (12), 686–700. doi:10.1038/nrgastro.2011.173
Letelier, P., Brebi, P., Tapia, O., and Roa, J. C. (2012). DNA promoter methylation as a diagnostic and therapeutic biomarker in gallbladder cancer. Clin. Epigenetics 4 (1), 11. doi:10.1186/1868-7083-4-11
Li, G., Tian, Y., and Zhu, W. G. (2020). The roles of histone deacetylases and their inhibitors in cancer therapy. Front. Cell Dev. Biol. 8, 576946. doi:10.3389/fcell.2020.576946
Li, M., Shi, M., Xu, Y., Qiu, J., and Lv, Q. (2021). Histone methyltransferase KMT2D regulates H3K4 methylation and is involved in the pathogenesis of ovarian cancer. Cell Transpl. 30, 9636897211027521. doi:10.1177/09636897211027521
Li, X., Cheung, K. F., Ma, X., Tian, L., Zhao, J., Go, M. Y. Y., et al. (2012). Epigenetic inactivation of paired box gene 5, a novel tumor suppressor gene, through direct upregulation of p53 is associated with prognosis in gastric cancer patients. Oncogene 31 (29), 3419–3430. doi:10.1038/onc.2011.511
Lin, D. C., Wang, M. R., and Koeffler, H. P. (2018). Genomic and epigenomic aberrations in esophageal squamous cell carcinoma and implications for patients. Gastroenterology 154 (2), 374–389. doi:10.1053/j.gastro.2017.06.066
Linder, B., Grozhik, A. V., Olarerin-George, A. O., Meydan, C., Mason, C. E., and Jaffrey, S. R. (2015). Single-nucleotide-resolution mapping of m6A and m6Am throughout the transcriptome. Nat. Methods 12 (8), 767–772. doi:10.1038/nmeth.3453
Lodewijk, I., Nunes, S. P., Henrique, R., Jerónimo, C., Dueñas, M., and Paramio, J. M. (2021). Tackling tumor microenvironment through epigenetic tools to improve cancer immunotherapy. Clin. Epigenetics 13 (1), 63. doi:10.1186/s13148-021-01046-0
Lorincz, A. T. (2011). The promise and the problems of epigenetics biomarkers in cancer. Expert Opin. Med. Diagn. 5 (5), 375–379. doi:10.1517/17530059.2011.590129
Lu, Y., Chan, Y. T., Tan, H. Y., Li, S., Wang, N., and Feng, Y. (2020). Epigenetic regulation in human cancer: The potential role of epi-drug in cancer therapy. Mol. Cancer 19 (1), 79–16. doi:10.1186/s12943-020-01197-3
Ma, X., Liu, Z., Zhang, Z., Huang, X., and Tang, W. (2017). Multiple network algorithm for epigenetic modules via the integration of genome-wide DNA methylation and gene expression data. BMC Bioinforma. 18 (1), 72. doi:10.1186/s12859-017-1490-6
Majchrzak-Celinska, A., Warych, A., and Szoszkiewicz, M. (2021). Novel approaches to epigenetic therapies: From drug combinations to epigenetic editing. Genes 1212 (2), 208. doi:10.3390/genes12020208
Maldonado, L., Brait, M., Loyo, M., Sullenberger, L., Wang, K., Peskoe, S. B., et al. (2014). GSTP1 promoter methylation is associated with recurrence in early stage prostate cancer. J. Urol. 192 (5), 1542–1548. doi:10.1016/j.juro.2014.04.082
Marcos-Villar, L., and Nieto, A. (2019). The DOT1L inhibitor Pinometostat decreases the host-response against infections: Considerations about its use in human therapy. Sci. Rep. 9 (1), 16862–16868. doi:10.1038/s41598-019-53239-6
Mathioudaki, K., Scorilas, A., Ardavanis, A., Lymberi, P., Tsiambas, E., Devetzi, M., et al. (2011). Clinical evaluation of PRMT1 gene expression in breast cancer. Tumour Biol. 32 (3), 575–582. doi:10.1007/s13277-010-0153-2
McLean, C. Y., Bristor, D., Hiller, M., Clarke, S. L., Schaar, B. T., Lowe, C. B., et al. (2010). GREAT improves functional interpretation of cis-regulatory regions. Nat. Biotechnol. 28 (5), 495–501. doi:10.1038/nbt.1630
Metere, A., and Graves, C. E. (2020). Factors influencing epigenetic mechanisms: Is there A role for bariatric surgery? High. Throughput. 9 (1), E6. doi:10.3390/ht9010006
Mittal, P., and Roberts, C. W. M. (2020). The SWI/SNF complex in cancer - biology, biomarkers and therapy. Nat. Rev. Clin. Oncol. 17 (7), 435–448. doi:10.1038/s41571-020-0357-3
Mohamed, M. A., Greif, P. A., Diamond, J., Sharaf, O., Maxwell, P., Montironi, R., et al. (2007). Epigenetic events, remodelling enzymes and their relationship to chromatin organization in prostatic intraepithelial neoplasia and prostatic adenocarcinoma. BJU Int. 99 (4), 908–915. doi:10.1111/j.1464-410X.2006.06704.x
Ned, R. M., Melillo, S., and Marrone, M. (2011). Fecal DNA testing for colorectal cancer screening: The ColoSure™ test. PLoS Curr. 3, RRN1220. doi:10.1371/currents.RRN1220
Paci, P., Colombo, T., Fiscon, G., Gurtner, A., Pavesi, G., and Farina, L. (2017). Erratum: SWIM: A computational tool to unveiling crucial nodes in complex biological networks. Sci. Rep. 7, 46843. doi:10.1038/srep46843
Ramon, L., David, C., Fontaine, K., Lallet, E., Marcaillou, C., Martin-Lannerée, S., et al. (2018). Technical validation of a reverse-transcription quantitative polymerase chain reaction in Vitro diagnostic test for the determination of MiR-31-3p expression levels in formalin-fixed paraffin-embedded metastatic colorectal cancer tumor specimens. Biomark. Insights 13, 1177271918763357. doi:10.1177/1177271918763357
Robertson, K. D. (2005). DNA methylation and human disease. Nat. Rev. Genet. 6 (8), 597–610. doi:10.1038/nrg1655
Rosas-Alonso, R., Colmenarejo-Fernandez, J., Pernia, O., Rodriguez-Antolín, C., Esteban, I., Ghanem, I., et al. (2021). Clinical validation of a novel quantitative assay for the detection of MGMT methylation in glioblastoma patients. Clin. Epigenetics 13 (1), 52. doi:10.1186/s13148-021-01044-2
Salam, I., Hussain, S., Mir, M. M., Dar, N. A., Abdullah, S., Siddiqi, M. A., et al. (2009). Aberrant promoter methylation and reduced expression of p16 gene in esophageal squamous cell carcinoma from kashmir valley: A high-risk area. Mol. Cell. Biochem. 332 (1–2), 51–58. doi:10.1007/s11010-009-0173-7
Sarno, F., Benincasa, G., List, M., Barabasi, A. L., Baumbach, J., Ciardiello, F., et al. (2021). Clinical epigenetics settings for cancer and cardiovascular diseases: Real-life applications of network medicine at the bedside. Clin. Epigenetics 13 (1), 66. doi:10.1186/s13148-021-01047-z
Schwartz, S., Mumbach, M. R., Jovanovic, M., Wang, T., Maciag, K., Bushkin, G. G., et al. (2014). Perturbation of m6A writers reveals two distinct classes of mRNA methylation at internal and 5′ sites. Cell Rep. 8 (1), 284–296. doi:10.1016/j.celrep.2014.05.048
Shain, A. H., Giacomini, C. P., Matsukuma, K., Karikari, C. A., Bashyam, M. D., Hidalgo, M., et al. (2012). Convergent structural alterations define SWItch/Sucrose NonFermentable (SWI/SNF) chromatin remodeler as a central tumor suppressive complex in pancreatic cancer. Proc. Natl. Acad. Sci. U. S. A. 109 (5), E252–E259. doi:10.1073/pnas.1114817109
Silverman, E. K., Schmidt, H. H. H. W., Anastasiadou, E., Altucci, L., Angelini, M., Badimon, L., et al. (2020). Molecular networks in network medicine: Development and applications. Wiley Interdiscip. Rev. Syst. Biol. Med. 12 (6), e1489. doi:10.1002/wsbm.1489
Singh, A., Gupta, S., and Sachan, M. (2019). Epigenetic biomarkers in the management of ovarian cancer: Current prospectives. Front. Cell Dev. Biol. 7, 182. doi:10.3389/fcell.2019.00182
Sinha, R., Hussain, S., Mehrotra, R., Kumar, R. S., Kumar, K., Pande, P., et al. (2013). Kras gene mutation and RASSF1A, FHIT and MGMT gene promoter hypermethylation: Indicators of tumor staging and metastasis in adenocarcinomatous sporadic colorectal cancer in Indian population. PLoS One 8 (4), e60142. doi:10.1371/journal.pone.0060142
Smitheman, K. N., Severson, T. M., Rajapurkar, S. R., McCabe, M. T., Karpinich, N., Foley, J., et al. (2019). Lysine specific demethylase 1 inactivation enhances differentiation and promotes cytotoxic response when combined with all-trans retinoic acid in acute myeloid leukemia across subtypes. Haematologica 104 (6), 1156–1167. doi:10.3324/haematol.2018.199190
Stirzaker, C., Zotenko, E., Song, J. Z., Qu, W., Nair, S. S., Locke, W. J., et al. (2015). Methylome sequencing in triple-negative breast cancer reveals distinct methylation clusters with prognostic value. Nat. Commun. 6, 5899. doi:10.1038/ncomms6899
Sung, H., Ferlay, J., Siegel, R. L., Laversanne, M., Soerjomataram, I., Jemal, A., et al. (2021). Global cancer statistics 2020: GLOBOCAN estimates of incidence and mortality worldwide for 36 cancers in 185 countries. Ca. Cancer J. Clin. 71 (3), 209–249. doi:10.3322/caac.21660
Thurn, K. T., Thomas, S., Moore, A., and Munster, P. N. (2011). Rational therapeutic combinations with histone deacetylase inhibitors for the treatment of cancer. Future Oncol. 7 (2), 263–283. doi:10.2217/fon.11.2
Tost, J. (2009). DNA methylation: An introduction to the biology and the disease-associated changes of a promising biomarker. Methods Mol. Biol. 507, 3–20. doi:10.1007/978-1-59745-522-0_1
Toyota, M., Ahuja, N., Ohe-Toyota, M., Herman, J. G., Baylin, S. B., and Issa, J. P. J. (1999). CpG island methylator phenotype in colorectal cancer. Proc. Natl. Acad. Sci. U. S. A. 96 (15), 8681–8686. doi:10.1073/pnas.96.15.8681
Waddington, C. H. (2012). The epigenotype. 1942. Int. J. Epidemiol. 41 (1), 10–13. doi:10.1093/ije/dyr184
Wilson, A. S., Power, B. E., and Molloy, P. L. (2007). DNA hypomethylation and human diseases. Biochim. Biophys. Acta 1775 (1), 138–162. doi:10.1016/j.bbcan.2006.08.007
Wilson, B. G., and Roberts, C. W. M. (2011). SWI/SNF nucleosome remodellers and cancer. Nat. Rev. Cancer 11 (7), 481–492. doi:10.1038/nrc3068
Witkiewicz, A. K., McMillan, E. A., Balaji, U., Baek, G. H., Lin, W. C., Mansour, J., et al. (2015). Whole-exome sequencing of pancreatic cancer defines genetic diversity and therapeutic targets. Nat. Commun. 6, 6744. doi:10.1038/ncomms7744
Yomtoubian, S., Lee, S. B., Verma, A., Izzo, F., Markowitz, G., Choi, H., et al. (2020). Inhibition of EZH2 catalytic activity selectively targets a metastatic subpopulation in triple-negative breast cancer. Cell Rep. 30 (3), 755–770. e6. doi:10.1016/j.celrep.2019.12.056
Zheng, C., and Xu, R. (2020). Predicting cancer origins with a DNA methylation-based deep neural network model. PLoS One 15 (5), e0226461. doi:10.1371/journal.pone.0226461
Zaccara, S., Ries, R. J., and Jaffrey, S. R. (2019). Reading, writing and erasing mRNA methylation. Nat. Rev. Mol. Cell Biol. 20 (10), 608–624. doi:10.1038/s41580-019-0168-5
Zhang, E., Hou, X., Hou, B., Zhang, M., and Song, Y. (2020). A risk prediction model of DNA methylation improves prognosis evaluation and indicates gene targets in prostate cancer. Epigenomics 12 (4), 333–352. doi:10.2217/epi-2019-0349
Zhang, H., Zhang, R. H., Liao, X. M., Yang, D., Wang, Y. C., Zhao, Y. L., et al. (2021). Discovery of β-carboline derivatives as a highly potent cardioprotectant against myocardial ischemia-reperfusion injury. J. Med. Chem. 64 (13), 9166–9181. doi:10.1021/acs.jmedchem.1c00384
Keywords: epigenetics, cancer, DNA methylation, histone modification, chromatin remodelling, cancer therapies
Citation: Tulsyan S, Aftab M, Sisodiya S, Khan A, Chikara A, Tanwar P and Hussain S (2022) Molecular basis of epigenetic regulation in cancer diagnosis and treatment. Front. Genet. 13:885635. doi: 10.3389/fgene.2022.885635
Received: 28 February 2022; Accepted: 19 July 2022;
Published: 24 August 2022.
Edited by:
Ángeles Carlos Reyes, Instituto Nacional de Enfermedades Respiratorias-México (INER), MexicoReviewed by:
Giuditta Benincasa, University of Campania Luigi Vanvitelli, ItalySamriddhi Shukla, Central Drug Research Institute (CSIR), India
Varish Ahmad, King Abdulaziz University, Saudi Arabia
Copyright © 2022 Tulsyan, Aftab, Sisodiya, Khan, Chikara, Tanwar and Hussain. This is an open-access article distributed under the terms of the Creative Commons Attribution License (CC BY). The use, distribution or reproduction in other forums is permitted, provided the original author(s) and the copyright owner(s) are credited and that the original publication in this journal is cited, in accordance with accepted academic practice. No use, distribution or reproduction is permitted which does not comply with these terms.
*Correspondence: Showket Hussain, c2hvd2tldC5odXNzYWluQGdvdi5pbg==; Pranay Tanwar, cHJhbmF5dGFud2FyQGdtYWlsLmNvbQ==