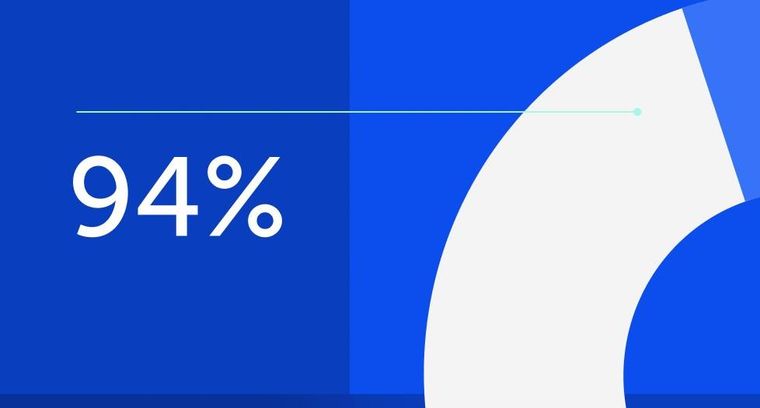
94% of researchers rate our articles as excellent or good
Learn more about the work of our research integrity team to safeguard the quality of each article we publish.
Find out more
ORIGINAL RESEARCH article
Front. Genet., 21 June 2022
Sec. Evolutionary and Population Genetics
Volume 13 - 2022 | https://doi.org/10.3389/fgene.2022.884072
This article is part of the Research TopicApplication of Fishes as Biological Models in Genetic StudiesView all 16 articles
Eukaryotic genomes are usually enriched in repetitive DNA sequences, which can be classified as dispersed or tandemly repeated elements. Satellite DNAs are noncoding monomeric sequences organized in a head-to-tail fashion that are generally located on the subtelomeric and/or pericentromeric heterochromatin. In general, a single species incorporates a diverse group of satellite DNA families, which collection is called satellitome. Here, we characterized three new satellitomes from distinct characid fish (Psalidodon fasciatus, P. bockmanni, and Astyanax lacustris) using a combination of genomic, cytogenetic, and bioinformatic protocols. We also compared our data with the available satellitome of P. paranae. We described 57 satellite DNA (satDNA) families of P. fasciatus (80 variants), 50 of P. bockmanni (77 variants), and 33 of A. lacustris (54 variants). Our analyses demonstrated that several sequences were shared among the analyzed species, while some were restricted to two or three species. In total, we isolated 104 distinctive satDNA families present in the four species, of which 10 were shared among all four. Chromosome mapping revealed that the clustered satDNA was mainly located in the subtelomeric and pericentromeric areas. Although all Psalidodon species demonstrated the same pattern of clusterization of satDNA, the number of clusters per genome was variable, indicating a high dynamism of these sequences. In addition, our results expand the knowledge of the As51 satellite DNA family, revealing that P. bockmanni and P. paranae exhibited an abundant variant of 39 bp, while P. fasciatus showed a variant of 43 bp. The majority of satDNAs in the satellitomes analyzed here presented a common library repetitive sequence in Psalidodon and Astyanax, with abundance variations in each species, as expected for closely related groups. In addition, we concluded that the most abundant satDNA in Psalidodon (As51) passed through a diversification process in this group, resulting in new variants exclusive of Psalidodon.
A significant part of eukaryotic genomes is composed of repetitive DNA sequences (Charlesworth et al., 1994), represented by transposable elements and tandemly arrayed sequences, such as multigene families and satellite DNAs (satDNAs) (Charlesworth et al., 1994; Jurka et al., 2005). SatDNAs are noncoding sequences organized in tandem arrays of up to hundreds of thousands of nucleotides (López-Flores and Garrido-Ramos, 2012; Plohl et al., 2012) that are typically observed in subtelomeric and pericentromeric heterochromatin areas, although short arrays of satDNAs dispersed in euchromatin have been documented (Kuhn, 2015; Ruiz-Ruano et al., 2016; Garrido-Ramos, 2017; Pita et al., 2017; Rodrigues et al., 2019; Montiel et al., 2021). They are originated from duplication of a new sequence by replication slippage or rolling circle replication, with posterior dispersion throughout the genome and massive local amplification (Ruiz-Ruano et al., 2016; Vondrak et al., 2020).
The evolution of satDNAs is characterized by a process called concerted evolution (Elder and Turner, 1995; Lorite et al., 2017). In this sense, satDNAs go through a step of homogenization, following mechanisms such as unequal crossing over and gene conversion (Smith, 1976; Dover, 1986), with homogenized variants fixed in populations by reproduction. In addition, related species may share an ancestral set of different conserved satDNA families (Fry and Salser, 1977; Ruiz-Ruano et al., 2016), according to the library hypothesis. Different variants may be amplified or depleted in each species, generating different collections of detectable satDNAs. Therefore, a part of the library may appear as an abundant satDNA, while others remain at low amounts (Camacho et al., 2022). To name this whole collection of satDNA families observed in a single genome, Ruiz-Ruano et al. (2016) proposed the term satellitome.
The first satellitome of a Neotropical fish was described for Psalidodon paranae, with 45 satDNA families (Silva et al., 2017), and some were detected by Fluorescence in situ hybridization (FISH) in congeneric species, corroboration of the library hypothesis. This identification of common satDNAs in three species of Psalidodon (Silva et al., 2017) and the recent diversification and phylogenetic proximity between P. paranae, P. fasciatus, and P. bockmanni, incites interest in this group for the study of the evolution of satDNAs. Psalidodon was part of the Astyanax, until recently (Terán et al., 2020). Prior to the description of the P. paranae satellitome, the only known satDNA in this group was As51 (Mestriner et al., 2000), which is widely used as a cytogenetic marker, and present in several species of Psalidodon, usually in subtelomeric chromatin regions (Abel et al., 2006; Kantek et al., 2009). In the P. paranae satellitome, As51 represents the most abundant satDNA, corresponding to ApaSat01-51 (Silva et al., 2017).
The satellitome of P. paranae is unique to the genus Psalidodon, although some of its satDNAs are observed in other related species (Silva et al., 2017). Previous evolutionary studies involving the characterization of the entire satellitome of a species are scarce. The objective of this study was to conduct, for the first time, a comparison among the catalogs of satDNAs, involving the satellitome of P. paranae, and three new satellitomes: P. fasciatus, P. bockmanni, and A. lacustris, to expand the knowledge of Psalidodon satDNA families and to describe the Astyanax satellitome for the first time.
We analyzed individuals of P. bockmanni, P. fasciatus, and A. lacustris that were collected from natural populations of the Alambari (22°27′07.4"S; 4914′30.4″W) (P. bockmanni and P. fasciatus), Araras (22°27′49″S; 47°44′44″W) (P. fasciatus), and Batalha (22°23′40.8"S; 4906′34.7″W) (A. lacustris) rivers. Five individuals of each species were collected, with exception of P. fasciatus, in which we collected five individuals of each population analyzed (Alambari and Araras). The samples were collected, maintained, and analyzed following the protocols for the care and use of animals of the Brazilian Society for Laboratory Animal Science (SBCAL), and approved (protocol 1227) by Bioscience Institute/UNESP Ethics Committee on the Use of Animals (CEEAA/IBB/UNESP). The samples were collected with authorization from the relevant organizations (MMA/IBAMA/ICMBio/SISBIO—18884-1, registered with IBAMA No. 2567470). The individuals were deposited at the fish collection of Laboratório de Genética de Peixes, at UNESP, Bauru, São Paulo, Brazil, under the vouchers LGP12524—LGP12529 (P. bockmanni), LGP13006 and LGP14052—LGP14055 (A. lacustris), LGP12554—LGP12558 (P. fasciatus–Alambari), and LGP85536 (P. fasciatus–Araras).
The cytogenetic analysis to obtain mitotic chromosomes followed a protocol previously established (Foresti et al., 1981), using cells of anterior kidney tissue.
We extracted total genomic DNA from the livers of P. bockmanni, P. fasciatus (Alambari, 2n = 46), and A. lacustris using the Wizard Genomic Kit (Promega, Madison, United States), following the manufacturer’s instructions. RNA was removed using RNase A (Invitrogen, Waltham, United States). Genomic DNA sequencing from an individual of each of P. fasciatus, P. bockmanni, and A. lacustris was performed using an Illumina MiSeq (paired-end 2 × 250 bp). In addition, the library of P. paranae was used in our analyses (Silva et al., 2017).
After quality and adapter trimming using Trimmomatic v0.33 (Bolger et al., 2014), we performed a high-throughput analysis of satDNAs within the genomes of P. fasciatus, P. bockmanni, and A. lacustris, using the satMiner bioinformatic protocol (Ruiz-Ruano et al., 2016). We performed a clustering of 2 × 500,000 reads, randomly selected using RepeatExplorer (Novák et al., 2013) with the default options to select clusters with a structure typical of satDNA. A search for contigs with tandem repetitions was performed using the dotplot tool, integrated into Geneious 8.1 software (Biomatters). We used the Deconseq software (Schmieder and Edwards, 2011) to filter the assembled contigs of all clusters designated as in tandem by RepeatExplorer, and the remaining sequences were clustered using RepeatExplorer. These processes were repeated until no new satDNA sequences appeared.
Subseqently, we filtered the obtained sequences and removed other tandemly repeated elements, such as multigene families, and used the software rm_homology (https://github.com/fjruizruano/satminer/blob/master/rm_homology.py) to eliminate redundancies of isolated contigs, and to group the sequences into the same variant, different variants of the same family, or superfamilies (similarities greater than 95, 80, and 50%, respectively), as established by Ruiz-Ruano et al., 2016. The same method was used to compare the satellitomes of P. paranae, P. fasciatus, P. bockmanni, and A. lacustris. The abundance and divergence of each satDNA variant were estimated using RepeatMasker software (Smit et al., 2017), using randomly selected reads (2 × 5,000,000 reads). In RepeatMasker, the reads of species were separately mapped against concatenated monomers of satDNAs consensus sequences (spanning 200 nucleotides). SatDNA families were named according to a previous study (Ruiz-Ruano et al., 2016), with the species abbreviations Pfa, Pbo, and Ala, for P. fasciatus, P. bockmanni, and A. lacustris, respectively, in addition to the term “Sat” and a catalog number in order of decreasing abundance. The catalogs of satDNA families were deposited on the GenBank with accession numbers OM793143-OM793191 (P. bockmanni), OM793192-OM793247 (P. fasciatus), and OM793248-OM793279 (A. lacustris). In addition, we generated repeat landscapes to estimate the average divergence, considering the distances between sequences based on the Kimura-2-parameter model using the script calcDivergenceFromAlign.pl, of the RepeatMasker suite (Supplementary Figures S1–S3).
To better understand the satDNA families observed in all four satellitomes described, we generated variant profiles and coverage depths using the RepeatProfiler pipeline (Negm et al., 2021) to analyze the sequence variation in the genomes studied. In addition, we included a library of A. mexicanus, a species model of the Astyanax genus, obtained in NCBI, SRA database under the access number SRR6386652. We randomly selected 2 × 1,000,000 reads for all five species, and the target satDNA families were concatenated to a minimum of 200 bp. We used dimers when the satDNA were greater than 200 bp. Read mapping was performed with Bowtie2 (Langmead and Salzberg, 2012) with the preset values “-sensitive” and “no-mixed.” We utilized 10 single-copy fish genes to be mapped for single-copy normalization of the read coverage, as described previously (dos Santos et al., 2021). The genes used here were ppfia1 (XM_022685633.1), foxl2 (XM_007232295.3), prospero (XM_017708821.1), msh4 (XM_017711771.1), zdhhc22 (XM_017711775.1), coq6 (XM_017711829.1), znf106 (XM_017711848.1), lactamase (XM_022682177.1), gastrula zinc finger (XM_022685636.1), and tubulin kinase (XM_017711762.1).
After verifying a satDNA family related to the cytogenetic marker As51 on the satellitome of P. bockmanni (PboSat03-39), characterized by a deletion of 12 bp, we investigated the presence of this variant in the genomes of P. paranae, P. bockmanni, P. fasciatus, A. lacustris, and A. mexicanus. In addition, a variant of 43 bp was observed in the satellitome of P. fasciatus, and we included this sequence in our analysis. We collected monomers of the three variants cited (51 bp, 39 bp, and 43 bp) from the genomes of the five species, using a random selection of 2 × 250,000 reads for each species. We then aligned the isolated reads against each satDNA variant, to only select full reads (Utsunomia et al., 2019). Subsequently, we discarded singletons using CD-HIT software (Li and Godzik, 2006). Due to the large number of monomers obtained, principally in P. paranae, we performed a random proportional selection of monomers for each species using Seqtk software (https://github.com/lh3/seqtk). A total of 2044 monomers were utilized, and information on the monomers obtained for each species and the quantity of monomers utilized in our analyses are shown in Supplementary Table S1. Finally, we constructed a minimum spanning tree (MST) of the pairwise differences and considered the relative abundance of the haplotypes using software PHYLOViZ 2.0 (Nascimento et al., 2017). The images were produced using the Inkscape software.
FISH experiments were performed with eight satDNA families, which were common to the four analyzed species. We utilized primers described by Silva et al. (2017) and probes were labeled with digoxigenin-11dUTP in PCR reactions. FISH experiments were performed following the protocol established by Pinkel et al. (1986), with some modifications (Utsunomia et al., 2017). The metaphasic plate was treated with RNase A (50 μg/ml), for 50 min, with subsequent chromosomal DNA denaturation in 70% formamide/2 × SSC for 2 min, at 70°C. After hybridization, the slides were washed in 0.2 × SSC/15% formamide for 5 min at 42°C, with subsequent washes in 4 × SSC/0.5% Tween-20, at room temperature. Probe detection was performed with anti-digoxigenin-rhodamine (Roche, Basiléia, Switzerland) and the chromosomes were counterstained with DAPI (4ʹ,6-diamino-2-phenylindole, Vector Laboratories, Burlingame, United States). The results were analyzed using an optical microscope (Olympus BX61). Images were captured using the DP Controller software (Olympus®, Hamburg, Germany).
Five individuals from each species were collected. Diploid numbers observed were consistent with the literature, with 2n = 50 for P. bockmanni and A. lacustris. Populations of P. fasciatus demonstrated differential diploid numbers, with 2n = 46 for those from the Alambari River and 2n = 48 for the Araras River. The karyotype formulas were 3m+5sm+6st+11a for P. bockmanni, 3m+5sm+12st+4a for P. fasciatus (Araras), 4m+7sm+9st+3a for P. fasciatus (Alambari), and 3m+9sm+9st+4a for A. lacustris. The species P. fasciatus is part of a “species complex”, with diploid numbers varying between 45 and 49, so our results are consistent with the literature (Kantek et al., 2009; Pasa et al., 2021). None of the analyzed individuals had B chromosomes.
After several iterations with the satMiner toolkit protocol (6 for P. fasciatus, seven for P. bockmanni, and three for A. lacustris), until no satDNA was uncovered, we found 57 families of satDNAs for P. fasciatus (80 variants), 50 for P. bockmanni (77 variants) and 33 for A. lacustris (54 variants). The length distribution of satDNA families revealed the predominance of short satDNAs (<100 bp) for P. fasciatus (42) and P. bockmanni (35), corresponded to 73.9 and 70.0% the satellitomes, respectively. In contrast, long satDNAs predominated in A. lacustris (18), corresponding to 54.5% of the satellitome. The repeat unit length (RUL) ranged from 6 to 286 bp for P. fasciatus (median 82.08); 6 to 584 for P. bockmanni (median 111.3) and 6 to 3028 in A. lacustris (median 316.27). The A + T content varied between 33.9 and 78.2% for P. fasciatus, (median 62.1%); 32.1–72.4% in P. bockmanni (median 57.9%), and 40.0–71.2% in A. lacustris (median 60.4%), indicating a predominance of A + T-rich satDNAs. Complete information on the three new satellitomes is presented in Tables 1–3. The Shapiro-Wilks test demonstrated that only the A + T content showed a normal distribution (W = 0.973, p = 0.235 for P. fasciatus, W = 0.984, p = 0.34 for P. bockmanni, and W = 0.945, p = 0.115 for A. lacustris). Kendall’s rank correlation test demonstrated that the only correlation between traces was a negative correlation between RUL and divergence in A. lacustris (tau = −0.465).
Comparisons between satDNA families for each species detected homology among sequences. Four superfamilies were detected in P. fasciatus, three in P. bockmanni, and only one in A. lacustris (Table 4). In most cases, the variation between sequences involved in a superfamily was caused by nucleotide substitutions. However, deletions of segments from 8 to 10 bp were observed in one of the sequences of SF1 in P. bockmanni and SF1, SF3, and SF4 in P. fasciatus (Supplementary Material).
TABLE 4. Superfamilies characterized in satellitomes of Psalidodon fasciatus, Psalidodon bockmanni, and Astyanax lacustris.
We performed a comparative analysis between the three satellitomes described in this study and that of P. paranae, using the RepeatMasker software. Of a total of 104 satDNA families present in the four species, 10 were observed in all species analyzed. One of them were identified as the telomeric sequence (ApaSat07-06-tel) that was included in other fish satellitomes (Silva et al., 2017; Utsunomia et al., 2019; Stornioli et al., 2021), and other was identified as CharSat01-52 (ApaSat29-52), which was conserved of the several species in Characidae family (dos Santos et al., 2021). Eight other sequences were observed in all four species, with at least 50% similarity (Table 5). In addition, several sequences were detected in only two or three species analyzed, as shown in Table 6, along with their degrees of similarity.
TABLE 5. SatDNA families with at least 50% of similarity in three species of Psalidodon and one species of Astyanax.
TABLE 6. SatDNAs similarity in two or three species analyzed. Similarity in superfamilies level (between 50 and 80%) are highlighted (*).
We generated RepeatProfiler plots of the ten satDNAs shared between the four species. In addition, we included the genome of Astyanax mexicanus for this analysis. We represent profiles of ApaSat12-69, and ApaSat30-50 in Figure 1, and remaining are in Supplementary Material. Our results revealed a high degree of conservation of ApaSat11-22 and ApaSat12-69 for all five species analyzed, with a similar degree of abundance in all groups. As expected, in all cases, the profiles demonstrated greater similarity between species of the Psalidodon than those of the Astyanax (ApaSat03-91, ApaSat29-52, ApaSat30-50, and ApaSat40-189). In addition, the two satDNA families (ApaSat02-236 and ApaSat04-233) observed as centromeric sequences, each demonstrated a large deletion in the Astyanax species, as well as different abundances in the Psalidodon and different mutations fixed on the species of this group.
FIGURE 1. Repeats landscapes of conserved satDNA families conserved between Psalidodon and Astyanax. Alignments and repeat profilers of ApaSat12-69 (A) and Apasat30-50 (B). Repeat landscapes of Apasat12-69 (C), Apasat30-50 (F) demonstrate the abundance and divergence of these satDNAs in the analyzed species. In addition, these satDNAs demonstrated FISH signals in all Psalidodon species (D and G), but not in Astyanax (E and H).
The As51 satDNA family was present in the satellitomes of P. paranae, P. fasciatus, and P. bockmanni, corresponding to the most abundant satDNA in these three species. However, this sequence is part of the superfamilies of P. fasciatus and P. bockmanni. New variants in these two species were produced mainly by deletion of parts of the original sequence, resulting in variants of 39 bp (PboSat03-39) and 43 bp (Pfasat55-43) (Figure 2). We produced a minimum spanning tree (MST) of the As51 satDNA and its variants using monomers extracted from P. paranae, P. fasciatus, P. bockmanni, A. lacustris, and A. mexicanus, excluding the sequence variants found only once (singletons) (Figure 2). The MST of the 39 bp variant was restricted to P. bockmanni and P. paranae, although this sequence was missing from the P. paranae satellitome, with several haplotypes shared between these two species, including the most abundant. The variant of 43 bp was restricted to P. fasciatus, with low abundance. None of the As51 monomers were shared among more than two species, and most only between P. paranae and P. bockmanni or P. paranae and P. fasciatus, corroborating the phylogeny of the group. Monomers of As51 were isolated in the genomes of A. lacustris and A. mexicanus, despite the absence of these satDNAs in the satellitomes of these species and the absence of FISH signals on their chromosomes.
FIGURE 2. Structure and conservation of As51 monomers between Psalidodon and Astyanax. Alignment of As51 variants, with 51 bp, 39 bp, and 43 bp, respectively (A). The emergence and disappearance of each variant is demonstrated in the phylogeny of species (blue = 51 bp, red = 39 bp, green = 43 bp) (B). Stars represents the surging of a variation of As51 and crosses represents the elimination of a variation of As51. Linear MST demonstrating the haplotypes of variants of As51 in Astyanax and Psalidodon species (C). Highlight of the dominance of a haplotype of As51-39 bp shared between Psalidodon bockmanni and Psalidodon paranae, and the most relevant of four haplotypes of As51-51 bp shared between P. bockmanni and P. paranae (1) and P. paranae and Psalidodon fasciatus (3) (C). As51 do not demonstrates FISH signals in Astyanax lacustris (D), but this marker forms greats cluster on chromosomes of P. bockmanni, P. paranae, and P. fasciatus (E–G). Fish images were captured in a magnification of ×1000.
We performed cytogenetic mapping of eight of the conserved satDNA families in the three species (Figure 3), except for the telomeric sequence (Apasat07-6-tel) and Apasat08-35, in which PCR amplification failed. We utilized the metaphase plates of A. lacustris, P. bockmanni, and two cytotypes of P. fasciatus. None of the satDNA families analyzed here demonstrated clusters on the chromosomes of A. lacustris, so we considered them as non-clustered in this species (Supplementary Figure S11). Additionally, Apasat29-52 did not cluster with any individual in our analysis. Clustered satDNAs were mainly present in heterochromatic subtelomeric and centromeric areas. All species demonstrated the same pattern of clusterization of satDNAs, but the number of chromosomes with cluster signals varied. We highlight the following: 1—Apasat02-236 and Apasat04-233 were clustered in pericentromeric regions, with Apasat02-236 present in all chromosomes of P. fasciatus and P. bockmanni, and Apasat04-233 in approximately half of the chromosomes of P. bockmanni and absent only on in par 12 in P. fasciatus; 2—Apasat30-50 demonstrated conserved clustered positions on the short arms of a pair of metacentric chromosomes in P. bockmanni and P. fasciatus; and 3—all other satDNAs demonstrated clusters in subtelomeric regions, with the exception of Apasat11-22 that had clusters in the interstitial regions of a pair of acrocentric chromosomes in P. bockmanni and two pairs of subtelocentric chromosomes in P. fasciatus.
FIGURE 3. Ideogram of clustered conserved satDNAs in Psalidodon bockmanni and two populations of Psalidodon fasciatus. No signal was observed in Astyanax lacustris.
In this study, we performed, for the first time for Neotropical fishes, an evolutionary comparison of the complete satellitome in four species. We observed a high retention of satDNAs in Psalidodon and Astyanax, demonstrated by the low number of species-exclusive satDNAs (8 for P. bockmanni, 15 for P. fasciatus, six for A. lacustris, and 16 for P. paranae). According to the library hypothesis (Fry and Salser, 1977), a group of related species should share a common library of satDNAs, and satellitomes can demonstrate quantitative differences among species due to differential amplification. Therefore, in addition to the possibility that these species-specific satDNAs have appeared de novo, future studies could find monomers of these sequences in low abundance on the genome of the other correlated species. A high degree of satDNA families found in the four satellitomes analyzed were shared between the species (Tables 5, 6), supporting the existence of a common library. However, divergences in the abundance of correlated satDNA families were found, as predicted by the library hypothesis (Fry and Salser, 1977). As an example, Alasat07-189 was correlated with Apasat40-189. Changes in satDNA abundance can occur by unequal crossing-over (Garrido-Ramos, 2017), replication slippage (Walsh, 1987; Stephan, 1989; Ruiz-Ruano et al., 2018), replication of extrachromosomal circles of tandem repeats by rolling-circle replication (Cohen et al., 2005; 2010), and transposition element actions (Jurka et al., 2005; Šatović and Plohl, 2013). Comparative analyses of satellitomes of correlated species also found a high degree of shared satDNA families, as in the grasshoppers Locusta migratoria and Oedaleus decorus (Camacho et al., 2022), In this case, association between satDNAs families and transposable elements were observed, as LmiSat02-176 and OdeSat17-176 associated with Helitron TEs (Camacho et al., 2022).
The A + T content was the only characteristic with a normal distribution in the satellitomes of P. bockmanni, P. fasciatus, and A. lacustris, similar to that found in the satellitomes of P. paranae (Silva et al., 2017) and Megaleporinus macrocephalus (Utsunomia et al., 2019). However, no correlations were observed between A + T content and RUL, as identified in P. paranae, or divergence and abundance, as identified in M. macrocephalus.
In addition to the Charsat01-52 and telomeric sequence, we found another eight satDNA families present on the four satellitomes. These satDNAs were maintained from 11.2 mya, when A. lacustris diverged from Psalidodon (Piscor et al., 2019). The maintenance of satDNA families across different species can occur through the biological function of determinate satDNA (Fry and Salser, 1977) or independence of natural selection (Stephan, 1986; Stephan, 1987; Walsh, 1987; Stephan, 1989; Harding et al., 1992). Despite the occurrence of the transcribed monomers of Charsat01-52 in P. paranae (dos Santos et al., 2021), we did not test the transcription of conserved satDNA in our satellitomes. However, the presence of Apasat02-236 and Apasat04-233 in the centromeres of all chromosomes in Psalidodon individuals suggests some structural function of these satDNAs. These sequences were related to Alasat08-236, and no FISH signal was observed in A. lacustris. Evidence in grasshoppers demonstrated that a satDNA family may be involved in centromeric function in one species, but not in other related species, suggesting that the species had replaced the centromeric satDNA during the evolution process (Camacho et al., 2022). It is common that the more abundant satDNAs are probably involved in centromeric function (Melters et al., 2013), as observed in P. paranae (ApaSat02-236 and ApaSat04-233) (Silva et al., 2017); however, in Eumingus monticola, the eighth satDNAs in order of decreasing abundance is located only in the pericentromeric regions (Camacho et al., 2022). In addition, examples of species with different satDNAs present in centromeres are common, such as those of chickens (Shang et al., 2010), plants (Iwata et al., 2013), and fishes (Prochilodus lineatus; Stornioli et al., 2021).
During the description of the satellitome of P. paranae (Silva et al., 2017), those authors obtained FISH signals of P. paranae satDNAs from P. bockmanni and P. fasciatus. Our analyses corroborated their results, with the addition of clustered signals of Apasat12-69 and Apasat40-189 in P. bockmanni and P. fasciatus. However, FISH signals were not observed in A. lacustris. According to the species tree (Silva et al., 2014) P. paranae and P. bockmanni are closely related species. We observed that approximately 50% of the satDNA families of P. bockmanni had some similarity with satDNAs of P. paranae, corroborating these results. In addition, the 39 bp variant of As51 (Apasat01-51) was present only in P. paranae and P. bockmanni.
The As51 satDNA was characterized by digestion of the KpnI restriction enzyme in P. scabripinnis (Mestriner et al., 2000), and is the most commonly used satDNA cytogenetic marker in this group, with FISH signals in P. paranae (Silva et al., 2014), P. scabripinnis (Mestriner et al., 2000), P. fasciatus (Kantek et al., 2009), and several other species. The description of the satellitome of P. paranae revealed that As51 was the most abundant satDNA in this species, corresponding to Apasat01-51. Our results demonstrated that it was the most also abundant in the satellitomes of P. bockmanni and P. fasciatus, despite its absence in A. lacustris satellitome. In addition, variants of this sequence have been described for the first time in P. fasciatus (Afasat55-43) and P. bockmanni (Abosat03-39, also present in P. paranae). Our data suggest the presence of As51 in an ancestor of Psalidodon and Astyanax, due to the identification of As51-51 monomers in the genomes of A. lacustris and A. mexicanus, where it remains as a relic in these species, with the absence of other variants in Astyanax. Therefore, we suggest the emergence of As51-51 in an ancestor of Psalidodon, and Astyanax (11.2 mya), with subsequent amplification and diversification of this satDNA in Psalidodon resulting in variants of 39 bp (6.5 mya) and 43 bp (2 mya). The absence of a 39 bp variant in P. fasciatus may be derived from stochastic processes that have led to significant nucleotide divergence. Similar cases were observed in Drosophila, with 1.688 satDNA conserved in a subgroup of species, with the exception of D. kikkawai and D. leontia (de Lima et al., 2020).
Our results expand the knowledge of the conservation and evolution of satDNAs in Psalidodon and Astyanax, demonstrating a large degree of sharing of sequences between these genera. In addition, we describe the evolutionary history of As51 with expansion and diversification of this sequence in Psalidodon.
The datasets presented in this study can be found in online repositories. The names of the repository/repositories and accession number(s) can be found in the article/Supplementary Material.
The animal study was reviewed and approved by Society for Laboratory Animal ScienceCollege of Animal Experimentation (SBCAL), and approved (protocol 1227) by Bioscience Institute/UNESP Ethics Committee on the Use of Animals (CEEAA/IBB/UNESP).
CG, RU, and FP-F conceived the study and wrote the manuscript. CG, RdS, WA, and DA conduced the experiments. CG, DS, and RU analysed the data. All authors read and approval the final version.
This study was supported by the FAPERJ (Fundação de Amparo à Pesquisa do Estado do Rio de Janeiro, grants 201.289/2021 and 211.475/2019), FAPESP (Fundação de Amparo à Pesquisa do Estado de São Paulo, grant 2018/03365-3), CAPES, and CNPq.
The authors declare that the research was conducted in the absence of any commercial or financial relationships that could be construed as a potential conflict of interest.
All claims expressed in this article are solely those of the authors and do not necessarily represent those of their affiliated organizations, or those of the publisher, the editors and the reviewers. Any product that may be evaluated in this article, or claim that may be made by its manufacturer, is not guaranteed or endorsed by the publisher.
We would like to thank the financial support of FAPESP, CAPES, CNPq, and FAPERJ, and Francisco Ruiz-Ruano and Juan Pedro Camacho to support to this work.
The Supplementary Material for this article can be found online at: https://www.frontiersin.org/articles/10.3389/fgene.2022.884072/full#supplementary-material
Abel, L. D. D. S., Mantovani, M., and Moreira-Filho, O. (2006). Chromosomal Distribution of the As51 Satellite DNA in Two Species Complexes of the Genus Astyanax (Pisces, Characidae). Genet. Mol. Biol. 29 (3), 448–452. doi:10.1590/s1415-47572006000300008
Bolger, A. M., Lohse, M., and Usadel, B. (2014). Trimmomatic: A Flexible Trimmer for Illumina Sequence Data. Bioinformatics 30 (15), 2114–2120. doi:10.1093/bioinformatics/btu170
Camacho, J. P. M., Cabrero, J., López-León, M. D., Martín-Peciña, M., Perfectti, F., Garrido-Ramos, M. A., et al. (2022). Satellitome Comparison of Two Oedipodine Grasshoppers Highlights the Contingent Nature of Satellite DNA Evolution. BMC Biol. 20, 36. doi:10.1186/s12915-021-01216-9
Charlesworth, B., Sniegowski, P., and Stephan, W. (1994). The Evolutionary Dynamics of Repetitive DNA in Eukaryotes. Nature 371 (6494), 215–220. doi:10.1038/371215a0
Cohen, S., Agmon, N., Sobol, O., and Segal, D. (2010). Extrachromosomal Circles of Satellite Repeats and 5S Ribosomal DNA in Human Cells. Mob. DNA 1 (1), 11. doi:10.1186/1759-8753-1-11
Cohen, S., Agmon, N., Yacobi, K., Mislovati, M., and Segal, D. (2005). Evidence for Rolling Circle Replication of Tandem Genes in Drosophila. Nucleic Acids Res. 33 (14), 4519–4526. doi:10.1093/nar/gki764
de Lima, L. G., Hanlon, S. L., and Gerton, J. L. (2020). Origins and Evolutionary Patterns of the 1.688 Satellite DNA Family in Drosophila Phylogeny. G3-Genes. Genom. Genet. 10 (11), 4129–4146. doi:10.1534/g3.120.401727
dos Santos, R. Z., Calegari, R. M., Silva, D. M. Z. A., Ruiz-Ruano, F. J., Melo, S., Oliveira, C., et al. (2021). A Long-Term Conserved Satellite DNA that Remains Unexpanded in Several Genomes of Characiformes Fish Is Actively Transcribed. Genome Biol. Evol. 13 (2), evab002. doi:10.1093/gbe/evab002
Dover, G. A. (1986). Molecular Drive in Multigene Families: How Biological Novelties Arise, Spread and are Assimilated. Trends. Genet. 2, 159–165. doi:10.1016/0168-9525(86)90211-8
Elder, J. F., and Turner, B. J. (1995). Concerted Evolution of Repetitive DNA Sequences in Eukaryotes. Q. Rev. Biol. 70 (3), 297–320. doi:10.1086/419073
Foresti, F., Almeida Toledo, L. F., and Toledo-Filho, S. A. (1981). Polymorphic Nature of Nucleolus Organizer Regions in Fishes. Cytogenet. Genome Res. 31 (3), 137–144. doi:10.1159/000131639
Fry, K., and Salser, W. (1977). Nucleotide Sequences of HS-α Satellite DNA from Kangaroo Rat Dipodomys Ordii and Characterization of Similar Sequences in Other Rodents. Cell 12 (4), 1069–1084. doi:10.1016/0092-8674(77)90170-2
Garrido-Ramos, M. (2017). Satellite DNA: An Evolving Topic. Genes 8 (9), 230. doi:10.3390/genes8090230
Harding, R. M., Boyce, A. J., and Clegg, J. B. (1992). The Evolution of Tandemly Repetitive DNA: 1137 Recombination Rules. Genetics 132 (3), 847–859. doi:10.1093/genetics/132.3.847
Iwata, A., Tek, A. L., Richard, M. M., Abernathy, B., Fonsêca, A., Schmutz, J., et al. (2013). Identification and Characterization of Functional Centromeres of the Common Bean. Plant J. 76 (1), 47–60. doi:10.1111/tpj.12269
Jurka, J., Kapitonov, V. V., Pavlicek, A., Klonowski, P., Kohany, O., and Walichiewicz, J. (2005). Repbase Update, a Database of Eukaryotic Repetitive Elements. Cytogenet. Genome Res. 110 (1–4), 462–467. doi:10.1159/000084979
Kantek, D. L. Z., Vicari, M. R., Peres, W. A. M., Cestari, M. M., Artoni, R. F., Bertollo, L. A. C., et al. (2009). Chromosomal Location and Distribution of As51 Satellite DNA in Five Species of the Genus Astyanax (Teleostei, Characidae, Incertae Sedis). J. Fish. Biol. 75 (2), 408–421. doi:10.1111/j.1095-8649.2009.02333.x
Kuhn, G. C. S. (2015). Satellite DNA Transcripts Have Diverse Biological Roles in Drosophila. Heredity 115 (1), 1–2. doi:10.1038/hdy.2015.12
Langmead, B., and Salzberg, S. L. (2012). Fast Gapped-Read Alignment with Bowtie 2. Nat. Methods 9 (4), 357–359. doi:10.1038/nmeth.1923
Li, W., and Godzik, A. (2006). Cd-hit: A Fast Program for Clustering and Comparing Large Sets of Protein or Nucleotide Sequences. Bioinformatics 22 (13), 1658–1659. doi:10.1093/bioinformatics/btl158
López-Flores, I., and Garrido-Ramos, M. A. (2012). “The Repetitive DNA Content of Eukaryotic Genomes,” in Repetitive DNA. Editor M.A. Garrido-Ramos (Basel: Karger), 7, 1–28. doi:10.1159/000337118
Lorite, P., Muñoz-López, M., Carrillo, J. A., Sanllorente, O., Vela, J., Mora, P., et al. (2017). Concerted Evolution, a Slow Process for Ant Satellite DNA: Study of the Satellite DNA in the Aphaenogaster Genus (Hymenoptera, Formicidae). Org. Divers. Evol. 17 (3), 595–606. doi:10.1007/s13127-017-0333-7
Melters, D. P., Bradnam, K. R., Young, H. A., Telis, N., May, M. R., Ruby, J., et al. (2013). Comparative Analysis of Tandem Repeats from Hundreds of Species Reveals Unique Insights into Centromere Evolution. Genome Biol. 14 (1), R10. doi:10.1186/gb-2013-14-1-r10
Mestriner, C. A., Galetti, P. M., Valentini, S. R., Ruiz, I. R. G., Abel, L. D. S., Moreira-Filho, O., et al. (2000). Structural and Functional Evidence that a B Chromosome in the Characid Fish Astyanax Scabripinnis Is an Isochromosome. Heredity 85, 1–9. doi:10.1046/j.1365-2540.2000.00702.x
Montiel, E. E., Panzera, F., Palomeque, T., Lorite, P., and Pita, S. (2021). Satellitome Analysis of Rhodnius Prolixus, One of the Main Chagas Disease Vector Species. Int. J. Mol. Sci. 22 (11), 6052. doi:10.3390/ijms22116052
Nascimento, M., Sousa, A., Ramirez, M., Francisco, A. P., Carriço, J. A., and Vaz, C. (2017). PHYLOViZ 2.0: Providing Scalable Data Integration and Visualization for Multiple Phylogenetic Inference Methods. Bioinformatics 33 (1), 128–129. doi:10.1093/bioinformatics/btw582
Negm, S., Greenberg, A., Larracuente, A. M., and Sproul, J. S. (2021). RepeatProfiler: A Pipeline for Visualization and Comparative Analysis of Repetitive DNA Profiles. Mol. Ecol. Resour. 21 (3), 969–981. doi:10.1111/1755-0998.13305
Novák, P., Neumann, P., Pech, J., Steinhaisl, J., and Macas, J. (2013). RepeatExplorer: A Galaxy-Based Web Server for Genome-Wide Characterization of Eukaryotic Repetitive Elements from Next-Generation Sequence Reads. Bioinformatics 29 (6), 792–793. doi:10.1093/bioinformatics/btt054
Pasa, R., Menegídio, F. B., Rodrigues-Oliveira, I. H., da Silva, I. B., de Campos, M. L. C. B., Rocha-Reis, D. A., et al. (2021). Ten Complete Mitochondrial Genomes of Gymnocharacini (Stethaprioninae, Characiformes). Insights into Evolutionary Relationships and a Repetitive Element in the Control Region (D-Loop). Front. Ecol. Evol. 9, 456. doi:10.3389/fevo.2021.650783
Pinkel, D., Straume, T., and Gray, J. W. (1986). Cytogenetic Analysis Using Quantitative, High-Sensitivity, Fluorescence Hybridization. Proc. Natl. Acad. Sci. U. S. A. 83 (9), 2934–2938. doi:10.1073/pnas.83.9.2934
Piscor, D., Pozzobon, A. P. B., Fernandes, C. A., Centofante, L., and Parise-Maltempi, P. P. (2019). Molecular Clock as Insight to Estimate the Evolutionary History and Times of Divergence for 10 Nominal Astyanax Species (Characiformes, Characidae): An Evolutionary Approach in Species with 2n= 36, 46, 48, and 50 Chromosomes. Zebrafish 16 (1), 98–105. doi:10.1089/zeb.2018.1647
Pita, S., Panzera, F., Mora, P., Vela, J., Cuadrado, Á., Sánchez, A., et al. (2017). Comparative Repeatome Analysis on Triatoma Infestans Andean and Non-Andean Lineages, Main Vector of Chagas Disease. PLoS One 12 (7), e0181635. doi:10.1371/journal.pone.0181635
Plohl, M., Meštrović, N., and Mravinac, B. (2012). “Satellite DNA Evolution,” in Repetitive DNA. Editor M. A. Garrido-Ramos (Basel: Karger Publishers), 7, 126–152. doi:10.1159/000337122
Rodrigues, P. H. D. M., dos Santos, R. Z., Silva, D. M. Z. D. A., Goes, C. A. G., Oliveira, C., Foresti, F., et al. (2019). Chromosomal and Genomic Dynamics of Satellite DNAs in Characidae (Characiformes, Teleostei) Species. Zebrafish 16 (4), 408–414. doi:10.1089/zeb.2019.1738
Ruiz-Ruano, F. J., Castillo-Martínez, J., Cabrero, J., Gómez, R., Camacho, J. P. M., and López-León, M. D. (2018). High-throughput Analysis of Satellite DNA in the Grasshopper Pyrgomorpha Conica Reveals Abundance of Homologous and Heterologous Higher-Order Repeats. Chromosoma 127 (3), 323–340. doi:10.1007/s00412-018-0666-9
Ruiz-Ruano, F. J., López-León, M. D., Cabrero, J., and Camacho, J. P. M. (2016). High-Throughput Analysis of the Satellitome Illuminates Satellite DNA Evolution. Sci. Rep. 6 (1), 28333. doi:10.1038/srep28333
Šatović, E., and Plohl, M. (2013). Tandem Repeat-Containing MITEs in the Clam Donax Trunculus. Genome Biol. Evol. 5 (12), 2549–2559. doi:10.1093/gbe/evt202
Schmieder, R., and Edwards, R. (2011). Quality Control and Preprocessing of Metagenomic Datasets. Bioinformatics 27 (6), 863–864. doi:10.1093/bioinformatics/btr026
Shang, W.-H., Hori, T., Toyoda, A., Kato, J., Popendorf, K., Sakakibara, Y., et al. (2010). Chickens Possess Centromeres with Both Extended Tandem Repeats and Short Non-Tandem-Repetitive Sequences. Genome Res. 20 (9), 1219–1228. doi:10.1101/gr.106245.110
Silva, D. M. Z. D. A., Pansonato-Alves, J. C., Utsunomia, R., Araya-Jaime, C., Ruiz-Ruano, F. J., Daniel, S. N., et al. (2014). Delimiting the Origin of a B Chromosome by FISH Mapping, Chromosome Painting and DNA Sequence Analysis in Astyanax Paranae (Teleostei, Characiformes). PLoS One 9 (4), e94896. doi:10.1371/journal.pone.0094896
Silva, D. M. Z. D. A., Utsunomia, R., Ruiz-Ruano, F. J., Daniel, S. N., Porto-Foresti, F., Hashimoto, D. T., et al. (2017). High-Throughput Analysis Unveils a Highly Shared Satellite DNA Library Among Three Species of Fish Genus Astyanax. Sci. Rep. 7, 12726. Available at: https://www.nature.com/articles/s41598-017-12939-7. doi:10.1038/s41598-017-12939-7
Smith, G. P. (1976). Evolution of Repeated DNA Sequences by Unequal Crossover. Science 191 (4227), 528–535. doi:10.1126/science.1251186
Stephan, W. (1987). Quantitative Variation and Chromosomal Location of Satellite DNAs. Genet. Res. 50 (1), 41–52. doi:10.1017/S0016672300023326
Stephan, W. (1986). Recombination and the Evolution of Satellite DNA. Genet. Res. 47, 167–174. doi:10.1017/S0016672300023089
Stephan, W. (1989). Tandem-Repetitive Noncoding DNA: Forms and Forces. Mol. Biol. Evol. 6 (2), 198–212. doi:10.1093/oxfordjournals.molbev.a040542
Stornioli, J. H. F., Goes, C. A. G., Calegari, R. M., dos Santos, R. Z., Giglio, L. M., Foresti, F., et al. (2021). The B Chromosomes of Prochilodus Lineatus (Teleostei, Characiformes) Are Highly Enriched in Satellite DNAs. Cells 10 (6), 1527. doi:10.3390/cells10061527
Terán, G. E., Benitez, M. F., and Mirande, J. M. (2020). Opening the Trojan Horse: Phylogeny of Astyanax, Two New Genera and Resurrection of Psalidodon (Teleostei: Characidae). Zool. J. Linn. Soc. 190 (4), 1217–1234. doi:10.1093/zoolinnean/zlaa019
Utsunomia, R., Ruiz-Ruano, F. J., Silva, D. M. Z. A., Serrano, E. A., Rosa, I. F., Scudeler, P. E. S., et al. (2017). A Glimpse into the Satellite DNA Library in Characidae Fish (Teleostei, Characiformes). Front. Genet. 8, 103. doi:10.3389/fgene.2017.00103
Utsunomia, R., Silva, D. M. Z. A., Ruiz-Ruano, F. J., Goes, C. A. G., Melo, S., Ramos, L. P., et al. (2019). Satellitome Landscape Analysis of Megaleporinus Macrocephalus (Teleostei, Anostomidae) Reveals Intense Accumulation of Satellite Sequences on the Heteromorphic Sex Chromosome. Sci. Rep. 9 (1), 1–10. doi:10.1038/s41598-019-42383-8
Vondrak, T., Ávila Robledillo, L., Novák, P., Koblížková, A., Neumann, P., and Macas, J. (2020). Characterization of Repeat Arrays in Ultra‐Long Nanopore Reads Reveals Frequent Origin of Satellite DNA from Retrotransposon‐Derived Tandem Repeats. Plant J. 101 (2), 484–500. doi:10.1111/tpj.14546
Keywords: satellitome, satDNA evolution, characiforms, fish, neotropical fishes
Citation: Goes CAG, dos Santos RZ, Aguiar WRC, Alves DCV, Silva DMZdA, Foresti F, Oliveira C, Utsunomia R and Porto-Foresti F (2022) Revealing the Satellite DNA History in Psalidodon and Astyanax Characid Fish by Comparative Satellitomics. Front. Genet. 13:884072. doi: 10.3389/fgene.2022.884072
Received: 25 February 2022; Accepted: 31 May 2022;
Published: 21 June 2022.
Edited by:
Tony Silveira, Federal University of Rio Grande, BrazilReviewed by:
Érica Ramos, Serviço Social da Indústria (SESI), BrazilCopyright © 2022 Goes, dos Santos, Aguiar, Alves, Silva, Foresti, Oliveira, Utsunomia and Porto-Foresti. This is an open-access article distributed under the terms of the Creative Commons Attribution License (CC BY). The use, distribution or reproduction in other forums is permitted, provided the original author(s) and the copyright owner(s) are credited and that the original publication in this journal is cited, in accordance with accepted academic practice. No use, distribution or reproduction is permitted which does not comply with these terms.
*Correspondence: Fabio Porto-Foresti, ZnAuZm9yZXN0aUB1bmVzcC5icg==
Disclaimer: All claims expressed in this article are solely those of the authors and do not necessarily represent those of their affiliated organizations, or those of the publisher, the editors and the reviewers. Any product that may be evaluated in this article or claim that may be made by its manufacturer is not guaranteed or endorsed by the publisher.
Research integrity at Frontiers
Learn more about the work of our research integrity team to safeguard the quality of each article we publish.