- 1Tartu University Hospital, Clinic of Hematology and Oncology, Tartu, Estonia
- 2University of Tartu, Clinic of Hematology and Oncology, Tartu, Estonia
- 3Department of Clinical Genetics, Institute of Clinical Medicine, University of Tartu, Tartu, Estonia
- 4Genetics and Personalized Medicine Clinic, Tartu University Hospital, Tartu, Estonia
- 5Estonian Biobank, Institute of Genomics, University of Tartu, Tartu, Estonia
- 6Institute for Molecular Medicine Finland, Helsinki Institute of Life Science, University of Helsinki, Helsinki, Finland
- 7North-Estonian Medical Center, Oncology and Haematology Clinic, Tallinn, Estonia
- 8Institute of Mathematics and Statistics, University of Tartu, Tartu, Estonia
- 9Tartu University Hospital, Tartu, Estonia
- 10Antegenes, Tartu, Estonia
Although hereditary breast cancer screening and management are well accepted and established in clinical settings, these efforts result in the detection of only a fraction of genetic predisposition at the population level. Here, we describe our experience from a national pilot study (2018–2021) in which 180 female participants of Estonian biobank (of >150,000 participants in total) were re-contacted to discuss personalized clinical prevention measures based on their genetic predisposition defined by 11 breast cancer–related genes. Our results show that genetic risk variants are relatively common in the average-risk Estonian population. Seventy-five percent of breast cancer cases in at-risk subjects occurred before the age of 50 years. Only one-third of subjects would have been eligible for clinical screening according to the current criteria. The participants perceived the receipt of genetic risk information as valuable. Fluent cooperation of project teams supported by state-of-art data management, quality control, and secure transfer can enable the integration of research results to everyday medical practice in a highly efficient, timely, and well-accepted manner. The positive experience in this genotype-first breast cancer study confirms the value of using existing basic genomic data from population biobanks for precise prevention.
Introduction
Pathogenic variants (PV) in known breast cancer (BC)-associated genes have been explored since the identification of the BRCA1 risk variant in 1994 (Miki et al., 1994). Numerous high- and moderate-risk genes associated with BC and ovarian cancer (OC), and specifically hereditary breast and ovarian cancer (HBOC) syndrome, have been described (Weitzel et al., 2019; Angeli, Salvi and Tedaldi, 2020; Yoshida, 2021). Pathogenic variants in the most prevalent BRCA1 and BRCA2 genes (detected in ∼1/400 persons in the general population) dramatically increase the relative risk of BC, particularly among premenopausal women. For example, the cumulative lifetime risk of female BC may be as high as 46–87% for BRCA1 carriers and 38–84% for BRCA2 carriers; the cumulative risks of OC development by the age of 80 years in these carriers are 44 and 17%, respectively (Petrucelli, Daly and Pal, 1993). Male carriers of BRCA1/2 variants are at greater relative risk of developing breast, prostate, pancreatic, and several other malignancies (Kote-Jarai et al., 2011; Moran et al., 2012; Mersch et al., 2015; Mano et al., 2017; Ibrahim et al., 2018; Lee, Lee and Li, 2021; Maccaroni et al., 2021). Pathogenic variants in TP53, STK11, PTEN, and CDH1 genes are rare but highly penetrant and associated with high risk of developing several different cancers including BC (Chen and Lindblom, 2000; Heitzer et al., 2013; Fusco et al., 2020). Moderate risk genes ATM, PALB2, CHEK2, NBN, and NF1 are associated with 2–5 times higher relative risk for breast and other cancers depending on specific gene (Foretová et al., 2019; Hu et al., 2020; Rainville et al., 2020). Today, clinical genetic testing for the determination of BC susceptibility is widely available in most developed countries. However, guidelines restrict such testing to those who meet certain criteria or thresholds for risk variant carriage likelihood based on personal and family backgrounds of cancer, especially at a young age (National Institute for Health and Care Excellence, 2013; NCCN, 2021). Thus, genetic testing is often performed too late, when several cancers have already been diagnosed in individuals’ families and effective, life-saving preventive measures cannot be applied with full potential (King, 2001; Rebbeck et al., 2004; Rebbeck, Kauff and Domchek, 2009; Singer, 2021). In addition, the application of family history (FH)-based testing criteria is known to result in the identification of less than half of disease-causing variant carriers (Møller et al., 2007; Metcalfe et al., 2010; Gabai-Kapara et al., 2014; Manchanda et al., 2015) After more than 25 years of research and knowledge accumulation, along with tremendous progress in technology and biobank development, systematically informed genetic testing for the detection of fatal cancer susceptibility genes is still not taking place in a population-based manner. Estimates suggest that the implementation of such testing in the United Kingdom and United States alone would prevent hundreds of thousands of cancer cases and save lives cost-effectively (Manchanda et al., 2018; Manchanda and Gaba, 2018). In contrast to current practices, a genotype-first approach is needed to reach this goal; the advantages and challenges of such an approach have been described in previous work from the Estonian Biobank (EstBB) (Leitsalu et al., 2021). In this study, we evaluated the genotype-first approach for precise BC prevention in the clinical setting, assessing its feasibility and acceptance by participants. Breast cancer incidence in clinical and family-based cohorts may differ from population cohorts, therefore we added a cumulative incidence analysis to check the justification of genetics-based intervention approach.
We also propose a service model for early cancer screening that includes genetic testing for application at the national healthcare level.
Materials and Methods
This study was designed and performed as an observational feasibility study with clinical implications (Figure 1).
Ethical Approval
The protocol (and further amendments) for this study was approved by the Ethics Review Committee on Human Research of the University of Tartu and Estonian Committee on Bioethics and Human Research (approvals No 282/T-29, 291/M-21, 1.1-12/643, 11.1-12/643, 1.1-12/1,064, 1.1-12/572). The trial has been registered at ClinicalTrials.gov (no. NCT03989258).
Study Cohort
The EstBB is a population-based biobank at the Institute of Genomics, University of Tartu, Estonia. It has now over 200,000 participants, representing about 20% of Estonia’s adult population. All participants have provided broad written consent, allowing the EstBB to follow their cases through linked electronic health records from national registries and to re-contact them (Leitsalu et al., 2021). We used EstBB data to confirm and recontact 180 EstBB female participants aged 22–79-years with monogenic variants conferring BC risk in any of the 11 genes listed in relevant clinical guidelines (National Comprehensive Cancer Network, 2017). High-risk genes were BRCA1, BRCA2, TP53, STK11, PTEN, and CDH1, and moderate-risk genes were ATM, PALB2, CHEK2, NBN, and NF1. BC-associated variants for return of results were identified directly from high-coverage (30×) genome sequencing (n = 2,420) and exome sequencing (n = 2,356). Next generation sequencing (NGS) data included geographically distributed EstBB participants (n = 4,776), of whom 2,245 were female (47%). In addition, we used a subset of array genotyped (Global Screening Array; Illumina Inc. United States) and imputed data available prior to the return of results study (n = 154,201) of whom 100,731 were female participants (65.32%).
To analyze the cumulative incidence of BC, we used combined NGS and array genotyped data available from the full cohort for 136,043 EstBB female participants of whom 449 were with confirmed BRCA1 (n = 153), BRCA2 (n = 92) and CHEK2 (n = 204) pathogenic and likely pathogenic variants. All female participants in the EstBB cohort were included in the analysis of BC risk and cumulative incidence. In case of confirmed diagnosis of BC, participants for whom the date of diagnosis was known, were included.
Variant Detection and Evaluation
The genome and exome sequencing data preparation and quality control workflow has been described elsewhere (Leitsalu et al., 2021). Genotypes from sequenced data were called by GATK (McKenna et al., 2010; Auwera et al., 2013) HaplotypeCaller algorithm. Variants were filtered by GATK Variant Quality Score@ Recalibration (VQSR), call rate <90%, and Hardy-Weinberg equilibrium (HWE) p value < 1e-9. The genotype calling for the microarrays was performed by Illumina’s GenomeStudio v2.0.4 software using Illumina Global Screening Array (GSA) v1.0, v2.0, v2.0_EST and v3.0_est arrays. Individuals were excluded from the analysis if their call-rate was <95% or sex defined based on heterozygosity of X chromosome did not match sex in phenotype data. Variants were filtered by call-rate < 95% and HWE p value < 1e-4 (autosomal variants only). Indirect identification was based on long-range phasing methods (Leitsalu et al., 2021). In addition, an Estonian population-specific reference dataset for high-coverage genome sequencing samples (n = 2,297) was used to impute genotypes for 154,201 EstBB participants genotyped with GSA arrays. Haplotype phasing was performed using Eagle software (ver. 2.3) and imputation was performed using Beagle software (ver. 5.1) (Browning and Browning, 2007; Loh et al., 2016; Mitt et al., 2017). Human reference genome assembly GRCh37 (hg19) was used for all variant analyses.
A custom pipeline was used to annotate variants in all of the 11 BC-associated genes from sequencing, genotyping and imputed data (Leitsalu et al., 2021). All identified BC-associated variants in EstBB female participants were cross-referenced with the ClinVar database and annotated according to their ClinVar assertions as pathogenic, likely pathogenic, uncertain significance, benign, likely benign, or with conflicting interpretations of pathogenicity. Identified BC-associated variants were then classified as moderate or high risk based on annotated data if their clinical significance was reported to be likely pathogenic and pathogenic, respectively, according to ClinVar database. This set of identified variants were further prioritized and selected for validation, if carrier status was present in both, directly genotyped and imputed dataset and located in high-risk BC genes, e.g., BRCA1/2. Also, a subset of high and moderate risk variants in BRCA1, BRCA2, CHEK2, ATM, NBN, NF1 from sequencing data were selected for validation. All candidate variants were confirmed with Sanger sequencing prior to participant re-contact using DNA samples stored in the EstBB (Figure 2).
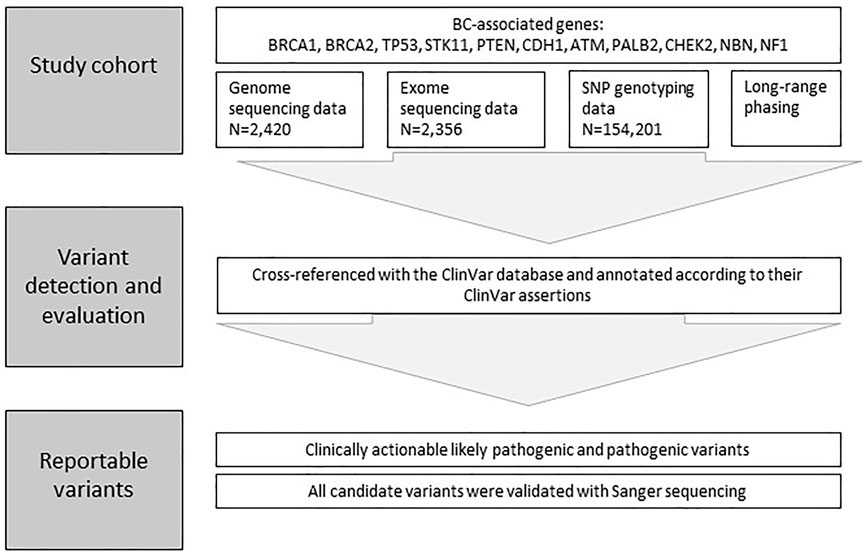
FIGURE 2. Framework for Variant detection and evaluation. A custom pipeline was used to annotate and prioritize variants in all of the 11 BC-associated genes from sequencing, SNP genotyping and long-range phasing data.
Recruitment
We identified and re-contacted female EstBB participants with alleles known to confer high or moderate hereditary BC risk. These individuals were sent invitation letters containing brief information about the scientific project on BC, study visits, and results dissemination (i.e., the opportunity to get personal BC prevention plans). To receive further information and to participate, the individuals were asked to schedule initial visits with clinical geneticists. The invitation contained no information on individuals’ personal genetic risks. One month later, a repeat invitation letter was sent to invited individuals who had not scheduled visits. Another month later, study personnel contacted non-responders by telephone.
Study Actions
The visits were scheduled in the two largest Estonian regional hospitals: Tartu University Hospital and The North Estonia Medical Center. During the first visits, clinical geneticists informed individuals about the study and enrolled only those who consented to receive genetic information. New blood samples were obtained for the second validation with Sanger sequencing, and data on 13 different BC risk factors (American Society of Clinical Oncology (ASCO), 2014; Barnard, Boeke and Tamimi, 2015; Jones et al., 2017; Collaborative Group on Hormonal Factors in Breast Cancer et al., 2019; Zeinomar and Knight, 2019; Zeinomar and Phillips., 2019), cancer FHs, and family pedigrees were recorded.
During the second visits, the geneticists communicated confirmed genetic findings to participants and introduced relevant recommendations from local familial BC prevention and early detection guidelines (based on international guidelines) (National Institute for Health and Care Excellence, 2013; Paluch-Shimon et al., 2016; National Comprehensive Cancer Network, 2017; European Reference Networks and ERN, 2021; NCCN, 2021). Biological relatives of individuals with high genetic risk were mapped and invited for genetic counseling and testing, as part of routine clinical genetic management.
For clinical activities, the geneticists directed participants to clinical oncologists (n = 4). These oncologists identified other known BC risk factors, performed clinical examinations, organized imaging studies and laboratory testing (i.e., for the serum OC markers cancer antigen 125 and human epididymis secretory protein 4), discussed additional preventive options (e.g., surgical and chemoprevention), and created personal (variant-specific) surveillance plans according to local guidelines for familial BC management (based on international guidelines) (National Institute for Health and Care Excellence, 2013; Paluch-Shimon et al., 2016; National Comprehensive Cancer Network, 2017; European Reference Networks and ERN, 2021; NCCN, 2021). All previous cancer diagnoses were confirmed with information from digital health records.
Participant Feedback
Participants’ responses to the receipt of genetic risk information were gathered using two surveys developed based on findings from analogous previous studies (Marteau and Bekker, 1992; Brehaut et al., 2003; Gray et al., 2014; Leitsalu et al., 2016, 2021). The first survey, filled out at the end of the second clinical geneticist visit (immediately after the receipt of genetic risk information) included questions about participants’ satisfaction and solicited self-reported psychological responses to the information received. The second survey, administered ≥6 months later, included questions about decision regret, perceived personal control and coping, psychological adjustment, communication, support, and reported health behavior and healthcare utilization.
Data Analysis
We used the RedCap database (Harris et al., 2009, 2019) hosted by University of Tartu for information storage and basic descriptive statistical analysis. Detailed analysis was performed with R version 4.0.4 or later (R Foundation for Statistical Computing, 2021). We used the genetic testing criteria from the 2018 and 2021 National Comprehensive Cancer Network guidelines (National Comprehensive Cancer Network, 2017; NCCN, 2021) as clinical practice genetic testing eligibility criteria to evaluate participants and their family history to be indicative for genetic testing. We adjusted testing criteria secondarily to investigate if it would have improved genetic testing detection rate. Adjustment consisted of inclusion all pancreatic and prostate cancer in family history and later (up to age 60) BC onset age for close relatives.
The methodology for time to event data analysis (implemented in R package survival) (Terry M Therneau, 2022) was used to analyze cumulative incidence of BC, with Kaplan-Meier method used to obtain the cumulative incidence curves and Cox proportional hazards model was used to obtain the corresponding hazard ratios with 95% confidence Intervals. EstBB performs regular updating of participants’ health data from the national E-Health database. First time breast cancer diagnosis C50 ICD-10 entry in Estonian national E-health database was used for the analysis.
Results
Participation
Of 180 EstBB participants invited to the study, 111 (62%) women scheduled initial visits and 109 (61% of invited, 98% of responders) provided informed consent to study participation. Of these 109 participants, 101 (93%) attended second visits and 100 (92%) met oncologists during the study period.
Findings
The mean age of the participants at the time of study entry was 48 (range, 28–80) years (<40 years, 32%; 40–49 years, 28%; ≥60 years, 23%). The monogenic BC variant distribution among counseled participants was as follows: 54.1% BRCA1, 23% BRCA2, 14.7% CHEK2, 5.5% ATM, 1.8% NBN, and 0.9% NF1. Risk-conferring variants were pathogenic in 95% of cases and likely pathogenic in 5% of cases (Table 1).
Previous Genetic Counselling
Eleven (10%) participants had received formal hereditary BC diagnoses and counseling within the medical healthcare system prior to our study. Genetic testing had been performed previously due to personal histories of cancer in three of them. The majority of these participants (n = 8) did not request additional geneticist and/or oncologist consultations. Participants’ personal histories contained 16 (15%) cancer diagnoses, most prevalently BC (n = 10) and OC/adnexal cancer (n = 3; Table 2).
Cumulative Breast Cancer Incidence
For BRCA1 or BRCA2 variant carriers the hazard of BC is 12.1 (95% CI 9.1–16.0) times higher as compared to the rest of the EstBB cohort. For CHEK2 variant carriers the hazard is 4.4 (95% CI 2.7–10.7) times higher.
The cumulative incidence of BC by the age of 70 was estimated to be 35.3% (95% CI 24,7–44.4%) for BRCA1 or BRCA2 variant carriers. For CHEK2 carriers it was estimated to be 14.4% (95% CI 4.2–23.5%) and for the rest of the EstBB cohort without any BC-associated variants it was 4.3% (95% CI 4.2–4.5%) (Figure 3).
Cancer in Family History
Participants’ FHs revealed cancer among first-degree relatives in 53% of cases and among second-degree relatives in 73% of cases. The most frequent cancer locations were breast (n = 77), stomach (n = 23), and ovaries (n = 16). The remaining 61 cases were primary cancers of seven types (including unknown).
FHs met the 2018 familial BC genetic testing criteria in only 33% (n = 36) of cases. With criteria adjustment (including for pancreatic and prostate cancer histories and later BC onset age), another 20% of participants would have met the criteria, meaning that slightly more than half (53%) of actual risk variant carriers would have been identified by FH-based testing.
Breast Cancer Risk Factors
Information on BC risk factors in the study participants is provided in Table 3. The mean ages of menarche and menopause were 13.3 (range, 10–16) and 49.2 (range, 35–57) years, respectively. The mean numbers of pregnancies and childbirths per participant were 2.55 (range, 0–8) and 1.85 (range, 0–5), respectively; the mean age at the time of first labor was 23.7 (range, 16–40) years. The mean body mass index was 26.4 kg/m2. The most prevalent hormonal treatment was estradiol; in 90% of cases, the exact drug was not known. Half (50%) of the participants used oral contraception, for a mean of almost 7 (range, 0.1–20) years in total and 2.2 (range, 0–20) years before the first childbirth. Almost 70% of participants reported at least some alcohol consumption; 52% consumed <1 unit per week (social drinkers), 23% consumed ≥1 unit per week, and no participant reported daily alcohol consumption. 14% of participants were current regular smokers and 16% were former smokers. The mean number of pack-years was 14. Besides genetic findings, 46% of participants had one and 23% had two additional BC risk factors.
Diagnostic and Physical Examination Results
BC (incident cases) was diagnosed in six participants (5.5%) and in one case it was bilateral. Together with prevalent cases (10), BC was the most frequent (14.7%) malignancy among participants. Physical examination yielded pathological findings in three (3.3%) cases, and the diagnosis of invasive cancer was confirmed in one of these cases. Breast biopsies were performed in five cases. Seventy-three percent of participants underwent digital mammography; findings were pathological in four cases and unclear in two cases.
Breast magnetic resonance imaging and ultrasound examinations were performed for 43 and 6% of participants, respectively. Gynecological examinations and gynecological ultrasound were performed in 27 and 48% of participants, respectively. Serum OC markers were assessed in one-third of cases. Two CHEK2 variant carriers underwent colonoscopy, which yielded the finding of tubular adenoma in one case. One BRCA1 carrier with a personal history of BC underwent a positron emission tomography/computed tomography examination, which revealed no evidence of disease. During follow-up period, a stage I kidney cancer was discovered incidentally with ultrasound in a 47-year-old BRCA1 carrier and later she was successfully operated on.
Information on participants with BC and other cancer diagnoses is provided in Tables 4, 5, respectively.
Risk Reduction Interventions
Sixteen (14.7%) prophylactic salpingo-oophorectomies and 5 (4.6%) mastectomies were performed during the study period; no chemoprevention was performed, except for patients with BC who received adjuvant treatment. The majority (62.5%) of prophylactic salpingo-oophorectomies were performed on participants with personal histories of cancer, and 80% of mastectomies involved the removal of contralateral breast tissue in patients with personal histories of BC. FHs were indicative (e.g., fulfilled the testing criteria) for risk variants in 12 (75%) cases, and BRCA1 or BRCA2 variants were found in 100% of patients undergoing surgical intervention.
Clinical Geneticist Activities With Family Members
The scheduling of the first and second visits with clinical geneticists were partially affected by the COVID-19 pandemic. The close relatives of 106 participants were invited for visits, according to the clinical best practice guidelines. The mean number of relatives recommended for counseling per participant was 4.3. In total, 453 invitations for cascade screening were made. The complete number of relatives actually counseled by geneticists is not known.
Participant Feedback
Response rates for the first and second surveys were 84.4% (n = 92) and 47.7% (n = 52), respectively. Not all participants responded to all survey questions.
After receiving their genetic risk information, the majority (74–88%) of respondents tended to feel calm, content, and relaxed; a minority (11–21%) reported feeling worried, upset, or tense (Figure 4). Most respondents considered the information received to be informative (98.8%), valuable (97.6%), understandable (96.7%), and interesting (93.1%). Almost all (97.7%) respondents appreciated being contacted. Similarly, 97.7% of respondents reported understanding the familial implications of the findings and 82.5% thought that they would be able to explain the meaning of the findings to their relatives. Most (95.5%) respondents reported that they knew who to turn to for further information and support, and 97.8% thought that they had received sufficient information through consultation and counseling.
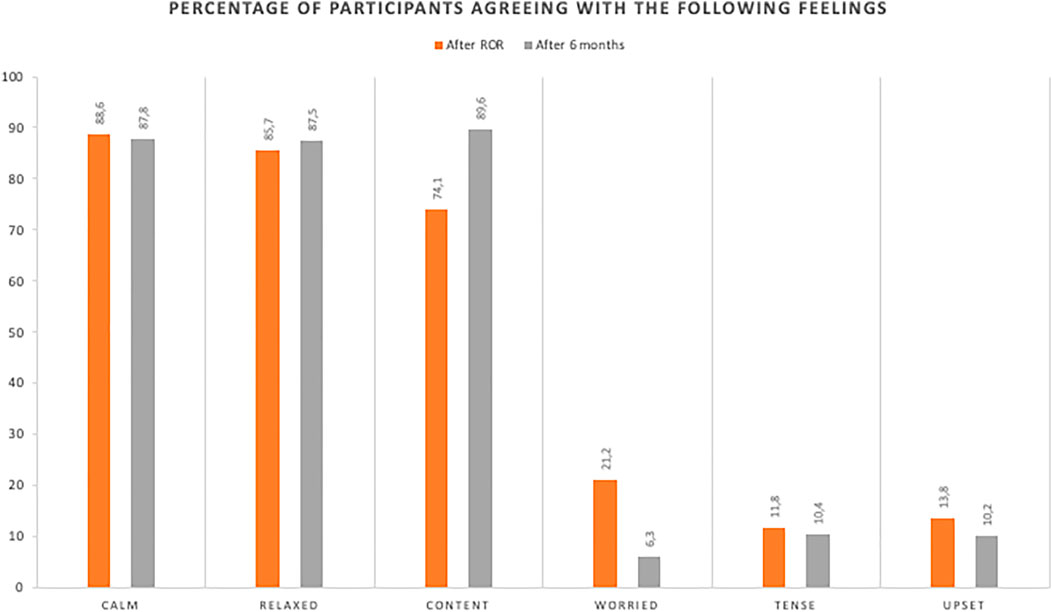
FIGURE 4. Proportions of participants reporting positive and uncomfortable feelings immediately after receiving genetic risk information and 6 months later.
Six or more months later, a greater proportion (87–89%) of respondents tended to feel content and relaxed, and fewer (6–10%) reported feeling worried, upset, or tense.
Most participants (88.2% of 51 individuals) reported that they were coping with having genetic risk factors; one participant disagreed slightly and five (9.8%) were unsure. The majority of respondents considered their decision to participate in the project and receive genetic risk information to be right (96%) and wise (94%), and 92% indicated that they would make the same decision again. The majority of respondents did not consider this decision to have caused them harm (92.1%) and had no regrets (90%). One person regretted the decision. Most (93.9%) of 49 respondents planned to follow the recommended screening and risk management plans.
The participants made some suggestions for improvement. Their comments addressed risk communication “Disclosure of the findings could have been more gentle/considerate”, “The doctor was too abrupt and did not explain much other than the need to hurry and remove my breast and ovaries: (My doctor got me scared so now I have not returned to the doctor for almost a year”), long-term risk management (“More thought on what a person could do moving on, psychologist? Counseling regarding the mastectomy?”) and help with family communication (“Collaboration with family physicians and disclosure to family members through family physicians”).
Discussion
The results of this study support the genotype-first approach to BC screening. It is applicable and feasible in the clinical setting and could thus be integrated into personalized population-based BC screening programs to maximize the benefits of prevention and early detection strategies. During our study, BC was diagnosed in six participants that would have otherwise been missed or discovered late. Of these six patients, five were younger than the current national screening program entry age of 50 years; two of these cases were diagnosed during pregnancy before the age of 40 years (the recommended age for baseline mammography). Most variant carriers had not been tested previously or were not aware of their genetic status. Furthermore, FHs did not fulfill the criteria for genetic testing in more than half of the cases. This might be partially explained by limited knowledge on the family history of the participants, but also a lower than expected cumulative incidence in the population cohort. Cumulative risk analyses were therefor performed on EstBB samples, genetic and breast cancer data. In our population cohort, BRCA1, and BRCA2 pathogenic and likely pathogenic variants have high risk of breast cancer. CHEK2 average cumulative breast cancer risks were even higher than expected from literature, but have large confidence intervals (Vahteristo et al., 2002; Weischer et al., 2007, 2008). Despite the relatively small number of subjects, our findings strongly justify the return of results and clinical interventions. The results are also in line with previously published prospective studies on larger cohorts (Mavaddat et al., 2013; Karoline B and Kuchenbaecker K. B. et al., 2017).
Modifiable Breast Cancer Risk Factors
The most common modifiable risk factor for BC in this study was smoking (current and former), and the second common factor was obesity (BMI>30 kg/m2). According to a recent national health report, the average prevalence of smoking among Estonian females is slightly higher (38%) and that of obesity is slightly lower (21%) than in our study group (Reile and Veideman, 2021). In Europe, only 16% of females are obese (Janssen, Bardoutsos and Vidra, 2020; Eurostat, 2021). Obesity is a serious public health problem associated with the development of several chronic diseases; it is responsible for substantial and increasing direct and indirect healthcare costs as it becomes more common in Europe, globally (its prevalence has almost tripled since 1975), and at younger ages (Eurostat, 2021). However, associations of obesity, smoking, and other risk factors with BC have not been found to be sufficiently strong to warrant the recommendation of sole preventive measures for high-risk allele carriers (King, Marks and Mandell, 2003; Manders et al., 2011; Zeinomar and Knight, 2019; Iyengar et al., 2021). Nevertheless, the provision of scientific information on risk factors during consultations with very young high-risk individuals is important to emphasize what they can do on a personal level to improve their health and minimize the risk of developing cancer before the age at which surgery, the most effective but psychologically most difficult preventive measure, is recommended. A review demonstrated that parity (after four births) and breastfeeding protect against BC and OC in BRCA1 carriers, whereas the opposite is true for BRCA2 carriers, whose risk of BC increases with each birth and for whom breastfeeding has no protective effect (Fishman, 2010).
Oral contraceptive use has also been associated with a reduced risk of OC development, but a potential slightly increased risk of BC development (Iodice et al., 2010). In this study, we identified BC during pregnancy in two BRCA1 carriers with the single additional BC risk factor of hormonal birth control use besides their genetic status. Regarding obesity, studies conducted with unselected populations have shown the opposite effect as those conducted with BRCA1/2 carriers; pre-menopause obesity protects against BC, whereas post-menopause obesity is a risk factor (Karoline B Kuchenbaecker K. B. et al., 2017; Lammert et al., 2018; Manchanda and Menon, 2018; Gallagher et al., 2020; Gao et al., 2021). In this study, we measured the body mass index but did not evaluate diet or physical activity. Data collection on modifiable risk factors was included to enable a longer prospective study on the given cohort and their further inclusion in the complex risk models.
FHs, Guideline Modification, and New Information
We found that a cancer FH, based on suggested testing criteria, is not indicative of the presence of known genetic disease-causing variants in most cases (National Comprehensive Cancer Network, 2017). We modified the criteria to include FHs in close relatives of prostate, pancreatic, and gastric cancers and BC with an onset age of <60 years, which resulted in the detection of slightly more than half of variants. The use of FHs has several limitations, especially in modern societies characterized by individualism, the privacy of health information, mental and/or physical separation of families, and non-biologically related families, which result in the lack of detailed information on family cancers (e.g., age of onset, anatomical location, morphological form, and immunohistochemical properties such as estrogen and progesterone receptor and human epidermal growth factor receptor two status in breast cancer). The retrieval of detailed and complex FHs can be time consuming and still result in imprecise risk assessment and testing decisions. For these reasons, genetic testing would ideally not be restricted to FH-based criteria, but rather be used to aid the assessment of malignancy prevalence likelihoods in variant carriers for the implementation of preventive strategies (Manchanda and Menon, 2018). Another powerful recently developed tool for risk assessment is the polygenic risk score, used in combination with monogenic testing (Karoline B Kuchenbaecker KB. et al., 2017; Gallagher et al., 2020; Gao et al., 2021; Shah, 2021). Zhang et al. (Zhang et al., 2019) reported that population-level genomic screening of all young adults was applicable and cost effective. Guzauskas et al. (Guzauskas et al., 2020) found that a model of genetic screening for HBOC, including cascade influences, in the United States was moderately cost effective when applied to younger (aged 25–30 years), but not older (≥45 years), individuals.
A systematic review revealed that the current evidence, technology, and knowledge support the application of population-level genetic testing for preventive strategy implementation in the near future (Manchanda and Gaba, 2018). Some issues remain to be resolved, including the development, implementation, and thorough evaluation of alternative service-delivery models that involve genetic experts and downstream management pathways. Manchanda and Gaba (Manchanda and Gaba, 2018) proposed six factors to be considered to maximize the effects of such programs: clinical utility, equal access, widening research, robust implementation pathways, cost effectiveness, and consistent coherent messaging. Supported by our findings and literature we suggest hereditary breast cancer risk screening with genetic testing for the whole population of women aged 25–30 and it should be developed, prepared and implemented wise as soon as possible to save more young lives. Moreover, whenever basic genomic information is available, as is the case with biobanks, it should be searched for hereditary cancer predisposing variants, and systematic return of data activities should be planned.
Surgical Procedures
During the limited follow-up period of this study, fewer participants than expected decided to undergo risk-reducing surgeries, the most effective preventive measures. This finding is in line with the previously reported 31% risk-reducing salpingo-oophorectomy uptake and no uptake of prophylactic mastectomy during follow-up among EstBB BRCA1/2 carriers (Leitsalu et al., 2021). It can be explained by the short follow-up period; cultural factors and traditions; the lack of availability of and reimbursement for high-quality breast reconstruction, as well as the inability to consult doctors about such procedures; and several other factors (Scheepens et al., 2021). We did not directly examine these possible explanations for low preventive surgery uptake in this trial. In a prospective study, Chai et al. (Chai et al., 2014)observed risk-reducing salpingo-oophorectomy uptakes of 45% among BRCA1 carriers and 34% among BRCA2 carriers by the age of 40 years; these percentages were 86 and 71%, respectively, by the age of 50 years. Risk-reducing mastectomy uptake was estimated to be 46% by the age of 70 years in both groups-(Chai et al., 2014). In our study, 32% of participants were aged <40 years and 60% were aged <50 years.
Cancers
The cancer burden is going to be a major problem in the next 20 years. Global Cancer Statistics estimates that a 47% increase will occur, with 28.4 million new cancer cases (excluding basal cell carcinoma) diagnosed globally in 2040 (Sung et al., 2021).
Almost 15% of participants and 21% of first-degree relatives in this study had BC diagnoses; one third of diagnoses were made before the age of 40 years, almost half were made at 40–50 years, and only quarter were made in alignment with the current BC screening program age. The estimated prevalence of BRCA risk variants in Estonia is 0.8% (1/124), according to EstBB sequencing data (Leitsalu et al., 2021) but it may be as high as 1/40 among Ashkenazi Jews (Hartge et al., 1999). Simple calculations suggest that the adult population of Estonia contains about 75,000 male and female monogenic variant carriers at risk of developing highly aggressive cancers of which many could be prevented or timely discovered and cured with appropriate personalized screening and handling programs following genetic testing. This study, conducted with only female carriers, confirmed that the BRCA1-associated BC pathological features in this population are typical [triple (estrogen receptor, progesterone receptor, and human epidermal growth factor receptor 2) negative, high grade, and poorly differentiated in the majority of cases], and that BRCA2-induced BC features reflect those of the general BC population more (Mavaddat et al., 2012).
In our cohort we found two kidney cancer cases (1 prevalent and one incident), both with the same BRCA1 PV (rs ID rs80357711) and early onset (before age 50) which is much different (>50 times higher) from the expected average population levels of kindney cancer prevalence, suggesting a possible connection with specific PV of BRCA1 and kidney cancer (chromophobe subtype). However, a recent large (with almost 15,000 BRCA1/2 PV carriers) analysis (Li et al., 2022) did not detect any significant association between kidney cancer and BRCA1/2 variants leaving little room for our data from small numbers of cases and controls to claim the opposite. Otherwise, our data on BRCA1/2 associated cancers beside BC is in line with Li et al. as the second most common cancer (after BC) among first-degree relatives in this study was gastric cancer (Maccaroni et al., 2021; Li et al., 2022).
Moderate and High-Risk Breast Cancer Variant Detection From SNP Genotyping Data
Less than one-quarter of pathogenic genetic variants in our study participants were detected with sequencing data, and most of them were non-BRCA findings; almost all BRCA1/2 findings were discovered from genotype data. As sequencing technology is more expensive, genotyping is currently the most commonly used method for biobank genetic information processing. This situation poses a challenge when attempting to incorporate biobank data into clinical practice, where reliability is extremely important to include and exclude certain pathologies in differential diagnoses. We see that genotyping-based genetic information is sufficiently trustworthy to be made widely available for some personalized medicine applications in clinical practice in the near future. At the same time, not all known high-disease-risk genetic variants are discovered by genotyping only and the high-risk variants found will have to be confirmed by sequencing before returning to participants. The creation of a system or database for genetic information storage and use at the national healthcare system level is underway in Estonia. All genetic information from biobanks could be transferred to such a system upon donors’ request. The broad-scale utility of such approach will be tested in the near future.
Participant Feedback
Only 10% of study participants had previously received counseling on possible hereditary BC, and slightly more than half of the participants had FHs indicative of hereditary BC. Thus, participants did not necessarily expect to discuss the topic of BC or receive news of the genetic risk factors they had. Nevertheless, they tended to feel calm, relaxed, and content; a minority of participants reported uncomfortable feelings, but almost all participants viewed the information they received as valuable and appreciated being contacted.
Although most participants reportedly planned to follow the screening and risk management recommendations, the uptake of risk-reducing surgeries was relatively low. Furthermore, some participants expressed a preference for more subtle and less direct communication when specialists introduced the idea of such surgery.
Limitations
This study has a number of minor limitations. The participation rate was lower than expected (61%), which might be related primarily to the biobank setting. The letter presenting only general information about the study may not have been sufficiently specific to be taken seriously, and difficulties with making contact after long periods (for some, almost 20 years later e.g., wrong addresses, loss of interest) were inherent. In addition, the COVID-19 pandemic occurred during the second part of the trial period and probably also impacted the participation rate, intervals between visits to geneticists, referrals from geneticists to oncologists, and oncologist visits (including rates of prophylactic surgery uptake and performance). Another limitation is that the trial participants did not comprise the full set of HBOC-associated gene carriers in the EstBB cohort; they were a budget-limited, randomly selected sub-population, preferably carrying high-risk gene variants. In addition, the clinical information gathered may not have been complete, as private medicine actions and genetic testing information were not available to oncologists and thus may not reflect the real rates reported. However, private oncological medicine has had a marginal role in Estonia, and the data presented are generally representative. Furthermore, we had difficulty following participants’ relatives due to the lack of registry; they were not involved directly in the study and thus not handled systematically. Finally, oncological FHs are often unclear with respect to specific diagnoses and morphological factors addressed in testing guidelines, such as the metastatic status, intraductal/cribriform morphology of prostate cancer, and diffuse nature of gastric cancer.
Conclusion
This study supports the applicability and feasibility of the genotype-first approach in the clinical setting of HBOC. This approach could be integrated into personalized population-based BC screening programs to maximize the benefits of prevention and early detection strategies.
Data Availability Statement
Restrictions apply to the datasets: The datasets presented in this article are not readily available because include personal information of subjects. Requests to access the datasets should be directed to Ethics Review Committee.
Ethics Statement
The studies involving human participants were reviewed and approved by the Ethics Review Committee on Human Research of the University of Tartu. The patients/participants provided their written informed consent to participate in this study. Written informed consent was obtained from the individual(s) for the publication of any potentially identifiable images or data included in this article.
Author Contributions
PP, NT, KF, KK-K, AM, and TE contributed to conception and design of the study. KB and HJ analyzed RedCap database, statistics. SK and KF performed statistical analysis of cumulative incidence of BC among EstBB female participants according to genetic finding and wrote appropriate section of manuscrcipt. HJ, MN, MP, LL and NT wrote sections of the manuscript. PP, NT, LR, VV, RK and KK consulted study participants and aquired clinical data. MT, PL, KR, ÜM, TK, KÕ, TN and AR acquired, analyzed and interpreted lab data. MK worked on sequencing and imputations, MN and TN validated genetic findings with Sanger sequencing. All authors contributed to manuscript revision, read, and approved the submitted version.
Funding
This research was funded by the Estonian Research Council RITA program, supported by the European Regional Development Fund in accordance with Directive no. 1.1-2/17/15 and by European Union through the European Regional Development Fund project no. 2014-2020.4.01.15-0012 GENTRANSMED. NT and PP are supported by the EIT Health grant BRIGHT (#220720). KO and TK are supported by Estonian Research Council grant PRG471. NT, MP, MN, TN, AR, and MK are supported by the Estonian Research Council grant PRG555. KF and SK were supported by the Estonian Research Council grant PRG1197.
Conflict of Interest
PP was employed by the company Antegenes
The remaining authors declare that the research was conducted in the absence of any commercial or financial relationships that could be construed as a potential conflict of interest.
Publisher’s Note
All claims expressed in this article are solely those of the authors and do not necessarily represent those of their affiliated organizations, or those of the publisher, the editors and the reviewers. Any product that may be evaluated in this article, or claim that may be made by its manufacturer, is not guaranteed or endorsed by the publisher.
Acknowledgments
We express our sincere thanks to the biobank contributors and their relatives for participating in this study. We also acknowledge the work of consortium estPerMed I partners, study personnel, and investigators.
References
American Society of Clinical Oncology (ASCO) (2014). Medical Oncology Self-Evaluation Program (ASCO-SEP) 2014. 4th edn. Alexandria: ASCO Education.
Angeli, D., Salvi, S., and Tedaldi, G. (2020). Genetic Predisposition to Breast and Ovarian Cancers: How Many and Which Genes to Test? Ijms 21 (3), 1128. doi:10.3390/ijms21031128
Auwera, G. A., Carneiro, M. O., Hartl, C., Poplin, R., del Angel, G., Levy‐Moonshine, A., et al. (2013). From FastQ Data to High‐Confidence Variant Calls: The Genome Analysis Toolkit Best Practices Pipeline. Curr. Protoc. Bioinforma. 43 (1), 11101–111033. doi:10.1002/0471250953.bi1110s43
Barnard, M. E., Boeke, C. E., and Tamimi, R. M. (2015). Established Breast Cancer Risk Factors and Risk of Intrinsic Tumor Subtypes. Biochimica Biophysica Acta (BBA) - Rev. Cancer 1856 (1), 73–85. doi:10.1016/j.bbcan.2015.06.002
Brehaut, J. C., O'Connor, A. M., Wood, T. J., Hack, T. F., Siminoff, L., Gordon, E., et al. (2003). Validation of a Decision Regret Scale. Med. Decis. Mak. 23 (4), 281–292. doi:10.1177/0272989X03256005
Browning, S. R., and Browning, B. L. (2007). Rapid and Accurate Haplotype Phasing and Missing-Data Inference for Whole-Genome Association Studies by Use of Localized Haplotype Clustering. Am. J. Hum. Genet. 81 (5), 1084–1097. doi:10.1086/521987
Chai, X., Friebel, T. M., Singer, C. F., Evans, D. G., Lynch, H. T., Isaacs, C., et al. (2014). Use of Risk-Reducing Surgeries in a Prospective Cohort of 1,499 BRCA1 and BRCA2 Mutation Carriers. Breast Cancer Res. Treat. 148 (2), 397–406. doi:10.1007/s10549-014-3134-0
Chen, J., and Lindblom, A. (2000). Germline Mutation Screening of the STK11/LKB1 Gene in Familial Breast Cancer with LOH on 19p. Clin. Genet. 57 (5), 394–397. doi:10.1034/j.1399-0004.2000.570511.x
Collaborative Group on Hormonal Factors in Breast Cancer (2019). Type and Timing of Menopausal Hormone Therapy and Breast Cancer Risk: Individual Participant Meta-Analysis of the Worldwide Epidemiological Evidence. Lancet 394, 1159–1168. doi:10.1016/S0140-6736(19)31709-X
European Reference Networks, G. T. R. S., and Ern, G. (2021) “Care Pathway - Hereditary Breast and Ovarian Cancer (HBOC),” Available at: https://www.genturis.eu/l=eng/Assets/20210914_ERN_GENTURIS_Care_Pathway_HBOC_v2.3_accepted_disclaimer_WM-2030.pdf [Preprint].
Eurostat (2021). Overweight and Obesity - BMI Statistics European Health Interview Survey 2019. Available at: https://ec.europa.eu/eurostat/statistics-explained/index.php?title=Overweight_and_obesity_-_BMI_statistics#Obesity_in_the_EU:_gender_differences (Accessed October 29, 2021).
Fishman, A. (2010). The Effects of Parity, Breastfeeding, and Infertility Treatment on the Risk of Hereditary Breast and Ovarian Cancer: A Review. Int. J. Gynecol. Cancer 20 (Suppl. 2), S31–S33. doi:10.1111/IGC.0b013e3181f60d4d
Foretová, L., Navrátilová, M., Svoboda, M., Vašíčková, P., Sťahlová Hrabincová, E., Házová, J., et al. (2019). Recommendations for Preventive Care for Women with Rare Genetic Cause of Breast and Ovarian Cancer. Klin. Onkol. 32 (Suppl. 2), 6–13. doi:10.14735/amko2019S6
Fusco, N., Sajjadi, E., Venetis, K., Gaudioso, G., Lopez, G., Corti, C., et al. (2020). PTEN Alterations and Their Role in Cancer Management: Are We Making Headway on Precision Medicine? Genes 11 (7), 719. doi:10.3390/genes11070719
Gabai-Kapara, E., Lahad, A., Kaufman, B., Friedman, E., Segev, S., Renbaum, P., et al. (2014). Population-based Screening for Breast and Ovarian Cancer Risk Due to BRCA1 and BRCA2. Proc. Natl. Acad. Sci. U.S.A. 111 (39), 14205–14210. doi:10.1073/pnas.1415979111
Gallagher, S., Hughes, E., Wagner, S., Tshiaba, P., Rosenthal, E., Roa, B. B., et al. (2020). Association of a Polygenic Risk Score With Breast Cancer Among Women Carriers of High- and Moderate-Risk Breast Cancer Genes. JAMA Netw. Open 3 (7), e208501. doi:10.1001/jamanetworkopen.2020.8501
Gao, C., Polley, E. C., Hart, S. N., Huang, H., Hu, C., Gnanaolivu, R., et al. (2021). Risk of Breast Cancer Among Carriers of Pathogenic Variants in Breast Cancer Predisposition Genes Varies by Polygenic Risk Score. J. Clin. Oncol. 39 (23), 2564–2573. doi:10.1200/JCO.20.01992
Gray, S. W., Martins, Y., Feuerman, L. Z., Bernhardt, B. A., Biesecker, B. B., Christensen, K. D., et al. (2014). Social and Behavioral Research in Genomic Sequencing: Approaches from the Clinical Sequencing Exploratory Research Consortium Outcomes and Measures Working Group. Genet. Med. 16 (10), 727–735. doi:10.1038/gim.2014.26
Guzauskas, G. F., Garbett, S., Zhou, Z., Spencer, S. J., Smith, H. S., Hao, J., et al. (2020). Cost-effectiveness of Population-Wide Genomic Screening for Hereditary Breast and Ovarian Cancer in the United States. JAMA Netw. Open 3 (10), e2022874. doi:10.1001/jamanetworkopen.2020.22874
Harris, P. A., Taylor, R., Minor, B. L., Elliott, V., Fernandez, M., O'Neal, L., et al. (2019). The REDCap Consortium: Building an International Community of Software Platform Partners. J. Biomed. Inf. 95, 103208. doi:10.1016/j.jbi.2019.103208
Harris, P. A., Taylor, R., Thielke, R., Payne, J., Gonzalez, N., and Conde, J. G. (2009). Research Electronic Data Capture (REDCap)-A Metadata-Driven Methodology and Workflow Process for Providing Translational Research Informatics Support. J. Biomed. Inf. 42 (2), 377–381. doi:10.1016/j.jbi.2008.08.010
Hartge, P., Struewing, J. P., Wacholder, S., Brody, L. C., and Tucker, M. A. (1999). The Prevalence of Common BRCA1 and BRCA2 Mutations Among Ashkenazi Jews. Am. J. Hum. Genet. 64 (4), 963–970. doi:10.1086/302320
Heitzer, E., Lax, S., Lafer, I., Müller, S. M., Pristauz, G., Ulz, P., et al. (2013). Multiplex Genetic Cancer Testing Identifies Pathogenic Mutations in TP53 and CDH1in a Patient with Bilateral Breast and Endometrial Adenocarcinoma. BMC Med. Genet. 14 (1), 129. doi:10.1186/1471-2350-14-129
Hu, Z.-Y., Liu, L., Xie, N., Lu, J., Liu, Z., Tang, Y., et al. (2020). Germline PALB2 Mutations in Cancers and Its Distinction From Somatic PALB2 Mutations in Breast Cancers. Front. Genet. 11, 829. doi:10.3389/fgene.2020.00829
Ibrahim, M., Yadav, S., Ogunleye, F., and Zakalik, D. (2018). Male BRCA Mutation Carriers: Clinical Characteristics and Cancer Spectrum. BMC Cancer 18 (1), 179. doi:10.1186/s12885-018-4098-y
Iodice, S., Barile, M., Rotmensz, N., Feroce, I., Bonanni, B., Radice, P., et al. (2010). Oral Contraceptive Use and Breast or Ovarian Cancer Risk in BRCA1/2 Carriers: A Meta-Analysis. Eur. J. Cancer 46 (12), 2275–2284. doi:10.1016/j.ejca.2010.04.018
Iyengar, N. M., Zhou, X. K., Mendieta, H., El-Hely, O., Giri, D. D., Winston, L., et al. (2021). Effects of Obesity on Breast Aromatase Expression and Systemic Metabo-Inflammation in Women with BRCA1 or BRCA2 Mutations. npj Breast Cancer 7 (1), 18. doi:10.1038/s41523-021-00226-8
Janssen, F., Bardoutsos, A., and Vidra, N. (2020). Obesity Prevalence in the Long-Term Future in 18 European Countries and in the USA. Obes. Facts 13 (5), 514–527. doi:10.1159/000511023
Jones, M. E., Schoemaker, M. J., Wright, L. B., Ashworth, A., and Swerdlow, A. J. (2017). Smoking and Risk of Breast Cancer in the Generations Study Cohort. Breast Cancer Res. 19 (1), 118. doi:10.1186/s13058-017-0908-4
King, M.-C., Marks, J. H., and Mandell, J. B. (2003). Breast and Ovarian Cancer Risks Due to Inherited Mutations in BRCA1 and BRCA2. Science 302 (5645), 643–646. doi:10.1126/science.1088759
King, M.-C. (2001). Tamoxifen and Breast Cancer Incidence Among Women With Inherited Mutations in BRCA1 and BRCA2. JAMA 286 (18), 2251. doi:10.1001/jama.286.18.2251
Kote-Jarai, Z., Leongamornlert, D., Saunders, E., Tymrakiewicz, M., Castro, E., Mahmud, N., et al. (2011). BRCA2 Is a Moderate Penetrance Gene Contributing to Young-Onset Prostate Cancer: Implications for Genetic Testing in Prostate Cancer Patients. Br. J. Cancer 105 (8), 1230–1234. doi:10.1038/bjc.2011.383
Kuchenbaecker, K. B., Hopper, J. L., Barnes, D. R., Phillips, K. A., Mooij, T. M., Roos-Blom, M. J., et al. (2017b). Risks of Breast, Ovarian, and Contralateral Breast Cancer for BRCA1 and BRCA2 Mutation Carriers. JAMA 317 (23), 2402–2416. doi:10.1001/jama.2017.7112
Kuchenbaecker, K. B., McGuffog, L., Barrowdale, D., Lee, A., Soucy, P., Dennis, J., et al. (2017a). Evaluation of Polygenic Risk Scores for Breast and Ovarian Cancer Risk Prediction in BRCA1 and BRCA2 Mutation Carriers. J. Natl. Cancer Inst. 109 (7), djw302. doi:10.1093/jnci/djw302
Lammert, J., Lubinski, J., Gronwald, J., Huzarski, T., Armel, S., Eisen, A., et al. (2018). Physical Activity during Adolescence and Young Adulthood and the Risk of Breast Cancer in BRCA1 and BRCA2 Mutation Carriers. Breast Cancer Res. Treat. 169 (3), 561–571. doi:10.1007/s10549-018-4694-1
Lee, Y.-C., Lee, Y.-L., and Li, C.-Y. (2021). BRCA Genes and Related Cancers: A Meta-Analysis from Epidemiological Cohort Studies. Medicina 57 (9), 905. doi:10.3390/medicina57090905
Leitsalu, L., Alavere, H., Jacquemont, S., Kolk, A., Maillard, A. M., Reigo, A., et al. (2016). Reporting Incidental Findings of Genomic Disorder-Associated Copy Number Variants to Unselected Biobank Participants. Pers. Med. 13 (4), 303–314. doi:10.2217/pme-2016-0009
Leitsalu, L., Palover, M., Sikka, T. T., Reigo, A., Kals, M., Pärn, K., et al. (2021). Genotype-first Approach to the Detection of Hereditary Breast and Ovarian Cancer Risk, and Effects of Risk Disclosure to Biobank Participants. Eur. J. Hum. Genet. 29 (3), 471–481. doi:10.1038/s41431-020-00760-2
Li, S., Silvestri, V., Leslie, G., Rebbeck, T. R., Neuhausen, S. L., Hopper, J. L., et al. (2022). Cancer Risks Associated With BRCA1 and BRCA2 Pathogenic Variants. J. Clin. Oncol. 40, 1529–1541. doi:10.1200/JCO.21.02112
Loh, P.-R., Danecek, P., Palamara, P. F., Fuchsberger, C., A Reshef, Y., K Finucane, H., et al. (2016). Reference-based Phasing Using the Haplotype Reference Consortium Panel. Nat. Genet. 48 (11), 1443–1448. doi:10.1038/ng.3679
Maccaroni, E., Giampieri, R., Lenci, E., Scortichini, L., Bianchi, F., Belvederesi, L., et al. (2021). BRCA Mutations and Gastrointestinal Cancers: When to Expect the Unexpected? Wjco 12 (7), 565–580. doi:10.5306/wjco.v12.i7.565
Manchanda, R., and Gaba, F. (2018). Population Based Testing for Primary Prevention: A Systematic Review. Cancers 10 (11), 424. doi:10.3390/cancers10110424
Manchanda, R., Loggenberg, K., Sanderson, S., Burnell, M., Wardle, J., Gessler, S., et al. (2015). Population Testing for Cancer Predisposing BRCA1/BRCA2 Mutations in the Ashkenazi-Jewish Community: A Randomized Controlled Trial. JNCI J. Natl. Cancer Inst. 107 (1), dju379. doi:10.1093/jnci/dju379
Manchanda, R., and Menon, U. (2018). Setting the Threshold for Surgical Prevention in Women at Increased Risk of Ovarian Cancer. Int. J. Gynecol. Cancer 28 (1), 34–42. doi:10.1097/IGC.0000000000001147
Manchanda, R., Patel, S., Gordeev, V. S., Antoniou, A. C., Smith, S., Lee, A., et al. (2018). Cost-effectiveness of Population-Based BRCA1, BRCA2, RAD51C, RAD51D, BRIP1, PALB2 Mutation Testing in Unselected General Population Women. JNCI J. Natl. Cancer Inst. 110 (7), 714–725. doi:10.1093/jnci/djx265
Manders, P., Pijpe, A., Hooning, M. J., Kluijt, I., Vasen, H. F. A., Hoogerbrugge, N., et al. (2011). Body Weight and Risk of Breast Cancer in BRCA1/2 Mutation Carriers. Breast Cancer Res. Treat. 126 (1), 193–202. doi:10.1007/s10549-010-1120-8
Mano, R., Benjaminov, O., Kedar, I., Bar, Y., Sela, S., Ozalvo, R., et al. (2017). PD07-10 MALIGNANCIES IN MALE BRCA MUTATION CARRIERS - RESULTS FROM A PROSPECTIVELY SCREENED COHORT OF PATIENTS ENROLLED TO A DEDICATED MALE BRCA CLINIC. J. Urology 197 (4S), e131–e132. doi:10.1016/j.juro.2017.02.385
Marteau, T. M., and Bekker, H. (1992). The Development of a Six‐item Short‐form of the State Scale of the Spielberger State-Trait Anxiety Inventory (STAI). Br. J. Clin. Psychol. 31 (3), 301–306. doi:10.1111/j.2044-8260.1992.tb00997.x
Mavaddat, N., Barrowdale, D., Andrulis, I. L., Domchek, S. M., Eccles, D., Nevanlinna, H., et al. (2012). Pathology of Breast and Ovarian Cancers Among BRCA1 and BRCA2 Mutation Carriers: Results from the Consortium of Investigators of Modifiers of BRCA1/2 (CIMBA). Cancer Epidemiol. Biomarkers Prev. 21 (1), 134–147. doi:10.1158/1055-9965.EPI-11-0775
Mavaddat, N., Peock, S., Frost, D., Ellis, S., Platte, R., Fineberg, E., et al. (2013). Cancer Risks for BRCA1 and BRCA2 Mutation Carriers: Results From Prospective Analysis of EMBRACE. J. Natl. Cancer Inst. 105 (11), 812–822. doi:10.1093/jnci/djt095
McKenna, A., Hanna, M., Banks, E., Sivachenko, A., Cibulskis, K., Kernytsky, A., et al. (2010). The Genome Analysis Toolkit: A MapReduce Framework for Analyzing Next-Generation DNA Sequencing Data. Genome Res. 20 (9), 1297–1303. doi:10.1101/gr.107524.110
Mersch, J., Jackson, M. A., Park, M., Nebgen, D., Peterson, S. K., Singletary, C., et al. (2015). Cancers Associated withBRCA1andBRCA2mutations Other Than Breast and Ovarian. Cancer 121 (2), 269–275. doi:10.1002/cncr.29041
Metcalfe, K. A., Poll, A., Royer, R., Llacuachaqui, M., Tulman, A., Sun, P., et al. (2010). Screening for Founder Mutations in BRCA1 and BRCA2 in Unselected Jewish Women. J. Clin. Oncol. 28 (3), 387–391. doi:10.1200/JCO.2009.25.0712
Miki, Y., Swensen, J., Shattuck-Eidens, D., Futreal, P. A., Harshman, K., Tavtigian, S., et al. (1994). A Strong Candidate for the Breast and Ovarian Cancer Susceptibility Gene BRCA1. Science 266 (5182), 66–71. doi:10.1126/science.7545954
Mitt, M., Kals, M., Pärn, K., Gabriel, S. B., Lander, E. S., Palotie, A., et al. (2017). Improved Imputation Accuracy of Rare and Low-Frequency Variants Using Population-specific High-Coverage WGS-Based Imputation Reference Panel. Eur. J. Hum. Genet. 25 (7), 869–876. doi:10.1038/ejhg.2017.51
Møller, P., Hagen, A. I., Apold, J., Maehle, L., Clark, N., Fiane, B., et al. (2007). Genetic Epidemiology of BRCA Mutations – Family History Detects Less Than 50% of the Mutation Carriers. Eur. J. Cancer 43 (11). doi:10.1016/j.ejca.2007.04.023
Moran, A., O’Hara, C., Khan, S., Shack, L., Woodward, E., Maher, E. R., et al. (2012). Risk of Cancer Other Than Breast or Ovarian in Individuals with BRCA1 and BRCA2 Mutations. Fam. Cancer 11 (2), 235–242. doi:10.1007/s10689-011-9506-2
National Comprehensive Cancer Network (2017). NCCN Guidelines. Genetic/Familial High-Risk Assessment: Breast and Ovarian, Genetic/Familial High-Risk Assessment: Breast and Ovarian. Plymouth Meeting: National Comprehensive Cancer Network. Version 1. 2018.
National Institute for Health and Care Excellence (2013). NICE Clinical Guideline CG164. Familial Breast Cancer: Classification and Care of People at Risk of Familial Breast Cancer and Management of Breast Cancer and Related Risks in People with a Family History of Breast Cancer. London, UK: National Institute for Health and Care Excellence.
NCCN (2021). NCCN Guidelines. Genetic/Familial High-Risk Assessment: Breast, Ovarian and Pancreatic. [Preprint] National Comprehensive Cancer Network Available at: https://www.nccn.org/professionals/physician_gls/pdf/genetics_bop.pdf (Accessed October 25, 2021).
Paluch-Shimon, S., Cardoso, F., Sessa, C., Balmana, J., Cardoso, M. J., Gilbert, F., et al. (2016). Prevention and Screening in BRCA Mutation Carriers and Other Breast/ovarian Hereditary Cancer Syndromes: ESMO Clinical Practice Guidelines for Cancer Prevention and Screening. Ann. Oncol. 27, v103–v110. doi:10.1093/annonc/mdw327
Petrucelli, N., Daly, M. B., and Pal, T. (1993). BRCA1- and BRCA2-Associated Hereditary Breast and Ovarian Cancer. Available at: https://www.ncbi.nlm.nih.gov/books/NBK1247/(Accessed October 25, 2021).
R Foundation for Statistical Computing (2021). R: A Language and Environment for Statistical Computing. Vienna, Austria: R Core Team.
Rainville, I., Hatcher, S., Rosenthal, E., Larson, K., Bernhisel, R., Meek, S., et al. (2020). High Risk of Breast Cancer in Women with Biallelic Pathogenic Variants in CHEK2. Breast Cancer Res. Treat. 180 (2), 503–509. doi:10.1007/s10549-020-05543-3
Rebbeck, T. R., Friebel, T., Lynch, H. T., Neuhausen, S. L., van ’t Veer, L., Garber, J. E., et al. (2004). Bilateral Prophylactic Mastectomy Reduces Breast Cancer Risk in BRCA1 and BRCA2 Mutation Carriers: The PROSE Study Group. J. Clin. Oncol. 22 (6), 1055–1062. doi:10.1200/JCO.2004.04.188
Rebbeck, T. R., Kauff, N. D., and Domchek, S. M. (2009). Meta-analysis of Risk Reduction Estimates Associated With Risk-Reducing Salpingo-Oophorectomy in BRCA1 or BRCA2 Mutation Carriers. J. Natl. Cancer Inst. 101 (2), 80–87. doi:10.1093/jnci/djn442
Reile, R., and Veideman, T. (2021). Health Behavior Among Estonian Adult Population Study 2020. Estonia: Tallinn.
Scheepens, J. C. C., Veer, L. v. t., Esserman, L., Belkora, J., and Mukhtar, R. A. (2021). Contralateral Prophylactic Mastectomy: A Narrative Review of the Evidence and Acceptability. Breast 56, 61–69. doi:10.1016/j.breast.2021.02.003
Shah, P. D. (2021). Polygenic Risk Scores for Breast Cancer-Can They Deliver on the Promise of Precision Medicine? JAMA Netw. Open 4 (8), e2119333. doi:10.1001/jamanetworkopen.2021.19333
Singer, C. F. (2021). Nonsurgical Prevention Strategies in BRCA1 and BRCA2 Mutation Carriers. Breast Care 16 (2), 144–148. doi:10.1159/000507503
Sung, H., Ferlay, J., Siegel, R. L., Laversanne, M., Soerjomataram, I., Jemal, A., et al. (2021). Global Cancer Statistics 2020: GLOBOCAN Estimates of Incidence and Mortality Worldwide for 36 Cancers in 185 Countries. CA A Cancer J. Clin. 71 (3), 209–249. doi:10.3322/caac.21660
TerryTherneau, M. (2022). A Package for Survival Analysis in R. Available at: https://CRAN.R-project.org/package=survival (Accessed June 4, 2022).
Vahteristo, P., Bartkova, J., Eerola, H., Syrjäkoski, K., Ojala, S., Kilpivaara, O., et al. (2002). A CHEK2 Genetic Variant Contributing to a Substantial Fraction of Familial Breast Cancer. Am. J. Hum. Genet. 71 (2), 432–438. doi:10.1086/341943
Weischer, M., Bojesen, S. E., Ellervik, C., Tybjærg-Hansen, A., and Nordestgaard, B. G. (2008). CHEK2*1100delC Genotyping for Clinical Assessment of Breast Cancer Risk: Meta-Analyses of 26,000 Patient Cases and 27,000 Controls. J. Clin. Oncol. 26 (4), 542–548. doi:10.1200/JCO.2007.12.5922
Weischer, M., Bojesen, S. E., Tybjærg-Hansen, A., Axelsson, C. K., and Nordestgaard, B. G. (2007). Increased Risk of Breast Cancer Associated With CHEK2*1100delC. J. Clin. Oncol. 25 (1), 57–63. doi:10.1200/JCO.2005.05.5160
Weitzel, J. N., Neuhausen, S. L., Adamson, A., Tao, S., Ricker, C., Maoz, A., et al. (2019). Pathogenic and Likely Pathogenic Variants in PALB2 , CHEK2 , and Other Known Breast Cancer Susceptibility Genes Among 1054 BRCA -negative Hispanics with Breast Cancer. Cancer 125 (16), 2829–2836. doi:10.1002/cncr.32083
Yoshida, R. (2021). Hereditary Breast and Ovarian Cancer (HBOC): Review of its Molecular Characteristics, Screening, Treatment, and Prognosis. Breast Cancer 28 (6), 1167–1180. doi:10.1007/s12282-020-01148-2
Zeinomar, N., Knight, J. A., Knight, J. A., Genkinger, J. M., Phillips, K.-A., Daly, M. B., et al. (2019). Alcohol Consumption, Cigarette Smoking, and Familial Breast Cancer Risk: Findings from the Prospective Family Study Cohort (ProF-SC). Breast Cancer Res. 21 (1), 128. doi:10.1186/s13058-019-1213-1
Zeinomar, N., Phillips, K. A., Daly, M. B., Milne, R. L., Dite, G. S., MacInnis, R. J., et al. (2019). Benign Breast Disease Increases Breast Cancer Risk Independent of Underlying Familial Risk Profile: Findings from a Prospective Family Study Cohort. Int. J. Cancer 145 (2), 370–379. doi:10.1002/ijc.32112
Keywords: genotype-first approach, return of results to biobank participants, research findings/results in healthcare, clinical practice personalized medicine, precision screening
Citation: Jürgens H, Roht L, Leitsalu L, Nõukas M, Palover M, Nikopensius T, Reigo A, Kals M, Kallak K, Kütner R, Budrikas K, Kuusk S, Valvere V, Laidre P, Toome K, Rekker K, Tooming M, Ülle Murumets , Kahre T, Kruuv-Käo K, Õunap K, Padrik P, Metspalu A, Esko T, Fischer K and Tõnisson N (2022) Precise, Genotype-First Breast Cancer Prevention: Experience With Transferring Monogenic Findings From a Population Biobank to the Clinical Setting. Front. Genet. 13:881100. doi: 10.3389/fgene.2022.881100
Received: 22 February 2022; Accepted: 21 June 2022;
Published: 22 July 2022.
Edited by:
Kelvin Yuen-Kwong Chan, Hong Kong Genome Institute (HKGI), Hong Kong, SAR ChinaReviewed by:
Valentina Silvestri, Sapienza University of Rome, ItalyQi Guo, Benevolent AI, United Kingdom
Copyright © 2022 Jürgens, Roht, Leitsalu, Nõukas, Palover, Nikopensius, Reigo, Kals, Kallak, Kütner, Budrikas, Kuusk, Valvere, Laidre, Toome, Rekker, Tooming, Ülle Murumets, Kahre, Kruuv-Käo, Õunap, Padrik, Metspalu, Esko, Fischer and Tõnisson. This is an open-access article distributed under the terms of the Creative Commons Attribution License (CC BY). The use, distribution or reproduction in other forums is permitted, provided the original author(s) and the copyright owner(s) are credited and that the original publication in this journal is cited, in accordance with accepted academic practice. No use, distribution or reproduction is permitted which does not comply with these terms.
*Correspondence: Hannes Jürgens, hannes.jurgens@kliinikum.ee