- 1Department of Botany, National P.G. College, Lucknow, India
- 2Bangalore, India
In order to meet the global challenges of food security, one of the foremost solutions lies in enhancing the crop productivity. This can be attained by considering key plant hormones such as cytokinins as agrochemicals as cytokinins in particular are known to control the essential processes of the plants. Even though, it has already been established since 1980s that the enzyme, cytokinin oxidase/dehydrogenase (CKO/CKX) deactivates cytokinins; the potential applications of manipulating these enzymes have mostly been speculated to have a high potential in the biotechnology industry and spreads to agriculture, horticulture and agroforestry. The enzyme is critical in maintaining a balanced level of cytokinins in plants. However, it is yet to be fully established that inhibiting this enzyme can be the constant source of improvement in the productivity of plants, even though success has been obtained in some economically important plant species. Furthermore, the impact efficiency of this enzyme may vary from plant to plant, which needs to be evaluated employing tissue culture and other extrinsic applications. This review intends to cover the relevant studies addressing any biological activity of this enzyme in the current context and any associated biotechnological applications specific to enhanced grain yield, abiotic stress tolerance, delayed senescence and in vitro organogenesis among various plants and not only cereals. Moreover, our study will identify the present gaps in research with respect to many important food crops, which will be useful for researchers who are actively involved in providing a foundation for a variety of genetically improved plants achieved through this manner. In addition to this, other ways of engineering the amount of cytokinin levels appropriate for signaling also needs to be analyzed in order to extend the benefits of cytokinin biology to other crops too. The application of these inhibitors can be considered among the best alternates as well as addition to genetically modified plants for overcoming the gaps in crop demand.
Introduction
The exponential rise in human population over the last few decades has forced many ultimate challenges at the basic level in terms of “food, feed, and bioenergy” (Gupta et al., 2021; Nisler et al., 2021), especially for the developing countries, such as India. Moreover, constant human interference has led to environmental imbalance causing poor crop yield. Along with this, various types of abiotic stresses such as drought, salinity, etc., have marred the agricultural production (Aremu et al., 2015). All this has led to scarcity of agricultural land, leaving almost no scope for its expansion to keep pace with the burst to meet the population needs. In order to maintain a sustainable balance between the supply chain of food and demand, it has been strongly realized by the scientists that the solution to this problem lies in focusing on developing ways of enhancing crop productivity of the “existing” agricultural land (Nisler et al., 2021). There are many facets through which the crop yields can be improved; one of such aspect involves controlling the level of plant growth regulators (PGRs) in the crops. It is well known fact that amongst the common PGRs, cytokinins play an indispensable function in plant growth and morphogenesis (Pavlů et al., 2018; Hai et al., 2020). Extensive research on cytokinins have revealed that appropriate levels of cytokinin are necessary for cytokinin governed essential physiological and regulatory responses in different cell types (Gupta et al., 2021) through the “complex network” of cytokinin signaling (Li et al., 2019). These include, controlling the “cell division” involving the expansion, proliferation and development of foliage, branches, root as well as the reproductive organs through “photomorphogenic cell differentiation” (Chiang et al., 2012; Efroni et al., 2013; Bishopp, et al., 2011); non-initiation of lateral roots (Bielach et al., 2012), prolongs stomatal closure (Pospíšilová et al., 2005) and seed fill (Kieber and Schaller, 2014). It has been realized that most of these morphogenetic responses can be directed towards enhancing crop production. Therefore, cytokinins can be employed as “potential agrochemicals” (Koprna et al., 2016; Nisler et al., 2021) for inducing the physiological advantages that can be achieved through enhancing the levels of cytokinins in the plants. Moreover, it has been reported that the increase in cytokinin levels in a plant can enhance seed/crop yields (Bartrina et al., 2011; Jameson and Song, 2016), increase positivity in tillering, improve setting of flowers and seeds (Koprna et al., 2016), impede senescence of the leaf (Zwack and Rashotte, 2015) and mediate their stress tolerance especially in case of drought (Hai et al., 2020; Devireddy et al., 2021), salinity adaptation (Joshi et al., 2018; Li et al., 2019), etc. This review focuses on the current understanding of cytokinin biology in relation to crop improvement. It has been divided into four further sections, commencing with the ways through which the level of cytokinins can be enhanced in the plants, followed by the understanding of the types of the cytokinin inhibitors, their mode of action, then summarizing the various biotechnological responses, especially related to various forms of stress.
Cytokinin Augmentation in Plants
The enhancement of cytokinins in the plants can be achieved through two possible ways, either by the addition of cytokinins that are natural or synthetic in nature or by restricting the cytokinin inhibitors. Strong natural cytokinins such as zeatin can only be applied to the plant as a “single dose at one time point”, which typically gets diluted after some days (Nisler et al., 2021). The positive impact is visible, however, as a short-term effect rather than a long term one and causes variations that are unreproducible and are therefore, unacceptable from the commercial point of view (Koprna et al., 2016). In contrast, synthetic ones such as thidiazuron (TDZ), N-(2-Chloro-4-pyridyl)-N′-phenylurea (CPPU), etc., are ineffective in their signaling aspects and may induce undesirable side effects.
Besides these, another way to increase the cytokinin levels can be through inhibiting the action of cytokinin regulation. Physiologically within the plants, the levels of cytokinins are controlled through the balance of four enzymes; out of which isopentenyl transferase (IPT), which employs the mevalonate as well as methylerythritol phosphate pathway (Wang et al., 2014), is primarily responsible for the cytokinin metabolism in nature (Jameson and Song, 2016), while deactivation of cytokinin is the sole responsibility of the enzyme called cytokinin oxidase/dehydrogenase, CKO/CKX (Chatfield and Armstrong, 1986; Jiang et al., 2016). As the part of the mechanism of action, CKO/CKX enzyme irreversibly inactivates the cytokinins through the removal of N6-isoprene side chain from the cytokinin molecules (Mok and Mok, 2001). It can also be suggested that the CKX enzyme, being a flavoprotein (Gupta et al., 2021), is also involved in the balance as well as regulation of cytokinins, thereby helps in maintaining cytokinin homeostasis (Thu et al., 2017; Hai et al., 2020). This regulatory function has mostly been reported from major cereals such as Hordeum vulgare (Zalewski et al., 2014), Zea mays (Brugière et al., 2003), Oryza sativa (Ashikari et al., 2005) and Triticum aestivum (Song et al., 2012; Zhang et al., 2012; Ogonowska et al., 2019). At the genetic level, the prevalence of CKX gene families in plants has varied from species to species (Nisler et al., 2021) with isoforms differing in “spatial and temporal expression patterns and subcellular localization” with some being localized in the apoplast, vacuoles and cytosols (Joshi et al., 2018; Nisler et al., 2021). The number of genes involved in cytokinin inhibition ranges from seven as found in Arabidopsis thaliana (Werner et al., 2003) and Medicago sativa (Li et al., 2019) to eight in Fragaria vesca (Jiang et al., 2016), eleven in Oryza sativa (Tsai et al., 2012) and Triticum aestivum (Chen et al., 2020), twelve in Malus domestica (Tan et al., 2018), thirteen in Zea mays (Morris et al., 1999) and 23 in Brassica napus (Liu et al., 2013). These genes can be targeted for production of genetically modified plants, which will induce the overexpression of CKX enzyme and can cause drastic changes in the “organ proportions” especially root morphology in barley plants as observed by Mrί;zová et al. (2013). The negative regulation of the cytokinins leads to enhanced crop yield and mediation towards tolerance of abiotic stresses as reported in rice (Yamburenko et al., 2017), Arabidopsis (Werner et al., 2003; Prerostova et al., 2018), barley (Pospíšilová et al., 2016; Holubová et al., 2018). Besides the up regulation of this gene, its down regulation or knocking off has also caused increased yield in rice due to the increase in the quantity of reproductive organs (Ashikari et al., 2005) even during salinity stress (Joshi et al., 2018). Apart from the traditional forms of genetic modification such as selective breeding and crossbreeding, genetic engineering and genome editing are some of the mechanisms through which gene manipulation can be done (US Food and Drug, 2022). It was reported that controlling this enzyme can lead to “tailor made” improvements in the productivity of plants (Ashikari et al., 2005). Moreover, newer techniques for genome editing such as CRISPR/Cas9 (clustered regularly interspaced short palindromic repeat) have been recently used for knocking out of CKX/CKO genes in barley (Holubová et al., 2018; Gasparis et al., 2019) and rice (Mao et al., 2020; Rong et al., 2021). However, none of the mechanism of action has not been fully understood till now (Joshi et al., 2018), even though success has been obtained in some economically important plant species such as apple (Liao et al., 2017), tobacco (Macková et al., 2013), etc. Furthermore, the effectivity of the impact of this enzyme may vary from plant to plant, which needs to be evaluated employing tissue culture and other extrinsic applications (Gupta et al., 2021).
Cytokinin Oxygenase/Dehydrogenase Inhibitors: Types and Mode of Action
The primary approaches to decrease the expression of CKX enzyme can either be through chemical means (Kopecný et al., 2010; Nisler et al., 2021) and molecular approaches (Gouda et al., 2020a; Nguyen et al., 2021). Figure 1 represents a schematic diagram on the mechanism of CKO/CKX control. Nisler et al. (2021) points that inhibition of CKX enzyme by chemicals had been reported long time back which is predated even before the engineering of the genetically modified plants. These chemicals are classified as synthetic cytokinins such as TDZ and its variants (Nisler et al., 2016, Nisler, 2018), diphenyl urea (DPU), chloropyridin phenyl urea (CPPU), N-(2-amino-pyridin-4-yl)-N′-phenylurea (APPU) (Kopecný et al., 2010) or new potent inhibitors derived from CPPU, DPU, and DCPU (Nisler et al., 2021). The findings from Nisler et al. (2016) showed a 15-times decrease in half-maximal inhibitory concentration (IC50) with TDZ for AtCKX2 in Arabidopsis and ZmCKX1 and ZmCKX4a in Zea mays. Along with this, derivatives of 2-X-6-anilinopurine along with 2-chloro-6-(3-methoxy- phenyl) aminopurine (INCYDE) have also been found to be effective inhibitors of CKX enzyme in Arabidopsis (Zatloukal et al., 2008; Prerostova et al., 2020) and tomato (Aremu et al., 2014), respectively. The antioxidant defense mechanism and efficiency of photosynthesis got elevated by the use of these potent compounds (Aremu et al., 2014). The potency of inhibition was found to be higher in the variant of DPU in comparison to DCPPU and the inhibition occurred at the concentration of 10−8 M (Nisler et al., 2021). Similarly, APPU was found to be a better inhibitor as compared to CPPU, TDZ and their derivatives (Kopecný et al., 2010). Moreover, the chemical use of CKX enzyme inhibitors was found to be more advantageous than the application of cytokinin exogenously as a moderate level but “long-term” enhancement in the endogenous levels of cytokinins was observed. Among the molecular approaches, heterogenous nuclear RNA (hRNA-CX3 and -CX5) were used to suppress expression of CKX enzyme in rice (Yeh et al., 2015). An increase in growth, chlorophyll content and grain yield were observed in this case. Recently, one of the molecular approaches applied specific missense single nucleotide polymorphisms (SNPs), namely SNP42, SNP43, SNP44, and SNP46 to reduce the expression of CKX enzyme in rice that led to increase in grain numbers (Gouda et al., 2020a), while another nine SNPs from five genes were demarcated in soybean for enhanced seed yield (Nguyen et al., 2021). In a new approach, computational means has also been followed to study the “structure, function and interaction” of the CKX enzyme from rice plants for the first time (Gouda et al., 2020b). A hypothetical 3-D structure of this enzyme was predicted, which showed the presence of 24 α helix and 13 β strands. This can be extremely useful in understanding the cause of enhanced yield in these plants.
Biotechnological Responses
The decrease in CKX enzyme using various form of inhibitors has manifested a series part of the biotechnological application response or effects. Table 1 summarizes the various studies conducted on the understanding the influence of CKX enzyme inhibitors over abiotic stress tolerance. One of the most common manifestations observed in the genetically modified plant includes the reduction of abiotic stresses and adaptations to drought in Arabidopsis (Prerostova et al., 2018), barley (Pospíšilová et al., 2016; Ramireddy et al., 2018), tobacco (Werner et al., 2010; Macková et al., 2013; Lubovská et al., 2014) and apple (Liao et al., 2017); heat tolerance in Arabidopsis (Prerostova et al., 2020); cold as well as salinity tolerance in tomato (Aremu et al., 2014), in alfalfa (Li et al., 2019), in Arabidopsis (Nisler et al., 2021), etc. Moreover, tolerance towards stresses from heavy metals such as cadmium also can be observed as a result (Gemrotová et al., 2013). Most importantly, there is an increase of antioxidant enzymes (Devireddy et al., 2021). Other outcomes include inducing shoot regeneration, roots and morphogenesis in Chinese water chestnut (Wang et al., 2015), in vitro responses such as organogenesis (Aremu et al., 2015; Werbrouck, 2016; Chen and Wei, 2018; Mazri et al., 2018), callus culture bioassays (Kopecný et al., 2010), delayed senescence (Nisler et al., 2016; Prernostava et al., 2018) and as basic as increasing yield of the cereal crops (Ashikari et al., 2005; Nisler et al., 2021). Moreover, 44% increased zinc levels were present in the seeds of the transgenic barley plant along with drought tolerance. It was construed that the overexpression of CKX enzyme made the plant more nutrient efficient (Ramireddy et al., 2018). In contrast, Gasparis et al. (2019) reported that knocking out the CKX genes may not enhance the grain yield in barley.
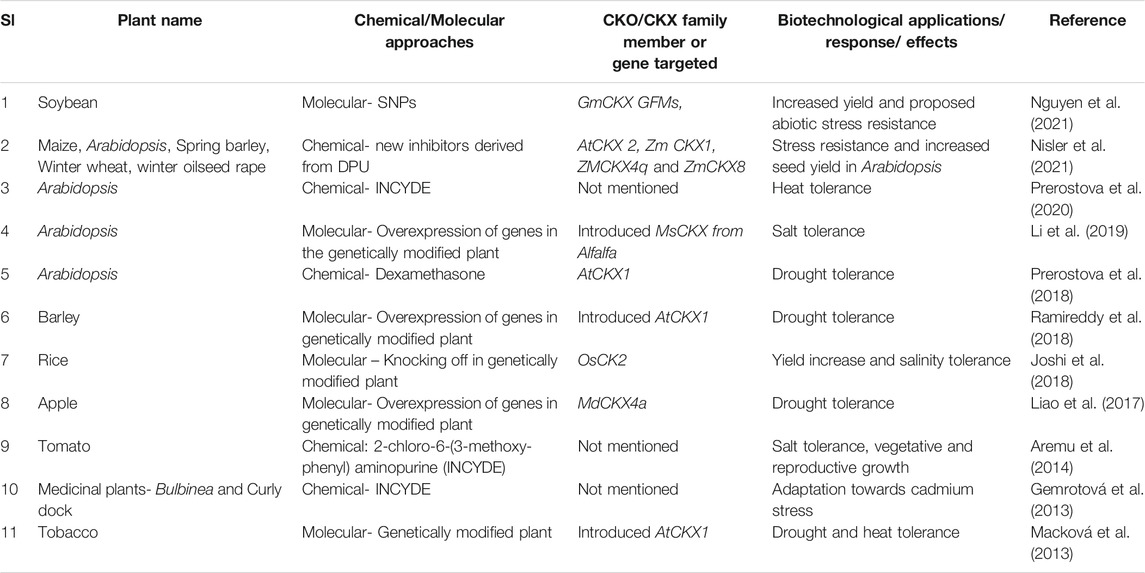
TABLE 1. Biotechnological responses of plants targeted with CKX inhibitors through chemical and molecular approaches.
Conclusion, Perspectives and Future Scope of Research
The application of inhibitors of CKX enzyme as a successful and capable tool for tolerance of abiotic stresses is evident from this study, which has a great potential for crop improvement in a variety of crops, including cereals. The present study reviews relevant research pertaining to the biological activity of the CKX enzyme in the context of adapting towards abiotic stresses along with improved grain yield. This can also be extended as the source of providing benefits to various crops through cytokinin biology. Other biotechnological responses of this enzyme also include delayed senescence and inducing organogenesis through tissue culture. In addition to this, other ways of manipulating the level of cytokinin suitable for signaling was also explained and the present gaps in this research area has been identified from this study.
The comparison of CKX enzyme inhibitors reveals that the use of chemicals is more popular over the molecular approaches. Therefore, it is anticipated that these chemicals can work as an alternate to genetically modified crops (Nisler et al., 2021). This will be extremely advantageous for mankind as any legal hassles towards acceptance of genetically modified organisms (GMOs) can be easily avoided using this approach, implying a wider reach among many varieties of plants across countries. However, an appropriate dosage level as well as the “cost effectiveness” of these chemicals is yet to be assessed at a commercial level, thereby warranting immediate attention from the researchers in this field. Recent studies demonstrate the emergence of successful genetic approaches (Wang et al., 2020; Nguyen et al., 2021; Nisler et al., 2021; and many others), emphasizing that modulating CKX enzymes can open up multiple paths for developing “tailor made” stress resistant and nutrition rich crops which will be useful in the long-term breeding programs (Ramireddy et al., 2018). These will be developed as a means of sustainable agriculture through unravelling the signaling network of the cytokinins (Pavlů et al., 2018). From this review, it was also realized that both up- and downregulation of the CKX gene can be instrumental in improving the economic needs, even though it seems to vary from plant to plant and even within a plant species. This ambiguity opens up a wide scope for further molecular research. In future, CKX inhibitors can be treated as part of plant defense regulators and studies can focus on comprehending the molecular mechanism of the interaction of CKX enzyme with other plant defense regulators such as jasmonic acid, salicylic acid, ethylene, abscisic acid (ABA) and others in order to develop a better understanding towards abiotic stresses.
Author Contributions
KA and SS were equally involved in the conception of the review, literature search, drafting the article and critical revision of the manuscript.
Conflict of Interest
The authors declare that the research was conducted in the absence of any commercial or financial relationships that could be construed as a potential conflict of interest.
Publisher’s Note
All claims expressed in this article are solely those of the authors and do not necessarily represent those of their affiliated organizations, or those of the publisher, the editors and the reviewers. Any product that may be evaluated in this article, or claim that may be made by its manufacturer, is not guaranteed or endorsed by the publisher.
Acknowledgments
We would like to thank the entire Frontiers editorial team for providing us the opportunity to write this review.
References
Aremu, A. O., Masondo, N. A., Sunmonu, T. O., Kulkarni, M. G., Zatloukal, M., Spichal, L., et al. (2014). A Novel Inhibitor of Cytokinin Degradation (INCYDE) Influences the Biochemical Parameters and Photosynthetic Apparatus in NaCl-Stressed Tomato Plants. Planta 240, 877–889. doi:10.1007/s00425-014-2126-y
Aremu, A. O., Stirk, W. A., Masondo, N. A., Plačková, L., Novák, O., Pěnčík, A., et al. (2015). Dissecting the Role of Two Cytokinin Analogues (INCYDE and PI-55) on In Vitro Organogenesis, Phytohormone Accumulation, Phytochemical Content and Antioxidant Activity. Plant Sci. 238, 81–94. doi:10.1016/j.plantsci.2015.05.018
Ashikari, M., Sakakibara, H., Lin, S., Yamamoto, T., Takashi, T., Nishimura, A., et al. (2005). Cytokinin Oxidase Regulates Rice Grain Production. Science 309, 741–745. doi:10.1126/science.1113373
Bartrina, I., Otto, E., Strnad, M., Werner, T., and Schmülling, T. (2011). Cytokinin Regulates the Activity of Reproductive Meristems, Flower Organ Size, Ovule Formation, and Thus Seed Yield inArabidopsis Thaliana. Plant Cell 23, 69–80. doi:10.1105/tpc.110.079079
Bielach, A., Podlešáková, K., Marhavý, P., Duclercq, J., Cuesta, C., Müller, B., et al. (2012). Spatiotemporal Regulation of Lateral Root Organogenesis in Arabidopsis by Cytokinin. Plant Cell 24, 3967–3981. doi:10.1105/tpc.112.103044
Bishopp, A., Help, H., El-Showk, S., Weijers, D., Scheres, B., Friml, J., et al. (2011). A Mutually Inhibitory Interaction between Auxin and Cytokinin Specifies Vascular Pattern in Roots. Curr. Biol. 21, 917–926. doi:10.1016/j.cub.2011.04.017
Brugière, N., Jiao, S., Hantke, S., Zinselmeier, C., Roessler, J. A., Niu, X., et al. (2003). Cytokinin Oxidase Gene Expression in maize Is Localized to the Vasculature, and Is Induced by Cytokinins, Abscisic Acid, and Abiotic Stress. Plant Physiol. 132, 1228–1240. doi:10.1104/pp.102.017707
Chatfield, J. M., and Armstrong, D. J. (1986). Regulation of Cytokinin Oxidase Activity in Callus Tissues of Phaseolus vulgaris L. Cv Great Northern. Plant Physiol. 80, 493–499. doi:10.1104/pp.80.2.493
Chen, J., and Wei, X. (2018). “Thidiazuron in Micropropagation of Aroid Plants,” in Thidiazuron: From Urea Derivative to Plant Growth Regulator (Singapore: Springer), 95–113. doi:10.1007/978-981-10-8004-3_4
Chen, L., Zhao, J., Song, J., and Jameson, P. E. (2020). Cytokinin Dehydrogenase: a Genetic Target for Yield Improvement in Wheat. Plant Biotechnol. J. 18, 614–630. doi:10.1111/pbi.13305
Chiang, Y.-H., Zubo, Y. O., Tapken, W., Kim, H. J., Lavanway, A. M., Howard, L., et al. (2012). Functional Characterization of the GATA Transcription Factors GNC and CGA1 Reveals Their Key Role in Chloroplast Development, Growth, and Division in Arabidopsis. Plant Physiol. 160, 332–348. doi:10.1104/pp.112.198705
Devireddy, A. R., Zandalinas, S. I., Fichman, Y., and Mittler, R. (2021). Integration of Reactive Oxygen Species and Hormone Signaling during Abiotic Stress. Plant J. 105, 459–476. doi:10.1111/tpj.15010
Efroni, I., Han, S.-K., Kim, H. J., Wu, M.-F., Steiner, E., Birnbaum, K. D., et al. (2013). Regulation of Leaf Maturation by Chromatin-Mediated Modulation of Cytokinin Responses. Dev. Cel 24, 438–445. doi:10.1016/j.devcel.2013.01.019
Gasparis, S., Przyborowski, M., Kała, M., and Nadolska-Orczyk, A. (2019). Knockout of the HvCKX1 or HvCKX3 Gene in Barley (Hordeum Vulgare L.) by RNA-Guided Cas9 Nuclease Affects the Regulation of Cytokinin Metabolism and Root Morphology. Cells 8, 782. doi:10.3390/cells8080782
Gemrotová, M., Kulkarni, M. G., Stirk, W. A., Strnad, M., Van Staden, J., and Spíchal, L. (2013). Seedlings of Medicinal Plants Treated with Either a Cytokinin Antagonist (PI-55) or an Inhibitor of Cytokinin Degradation (INCYDE) Are Protected against the Negative Effects of Cadmium. Plant Growth Regul. 71, 137–145. doi:10.1007/s10725-013-9813-8
Gouda, G., Gupta, M. K., Donde, R., Kumar, J., Parida, M., Mohapatra, T., et al. (2020a). Characterization of Haplotypes and Single Nucleotide Polymorphisms Associated with Gn1a for High Grain Number Formation in rice Plant. Genomics 112, 2647–2657. doi:10.1016/j.ygeno.2020.02.016
Gouda, G., Gupta, M. K., Donde, R., Kumar, J., Vadde, R., Mohapatra, T., et al. (2020b). Computational Approach towards Understanding Structural and Functional Role of Cytokinin Oxidase/dehydrogenase 2 (CKX2) in Enhancing Grain Yield in rice Plant. J. Biomol. Struct. Dyn. 38, 1158–1167. doi:10.1080/07391102.2019.1597771
Gupta, M. K., Gouda, G., Donde, R., Vadde, R., and Behera, L. (2021). Novel Cytokinin Oxidase/dehydrogenase Inhibitors for Enhancing Grain Yield in Crop Plants and Potential Applications in the Biotechnology Industry. J. Exp. Bot. 72, 153–156. doi:10.1093/jxb/eraa445
Hai, N. N., Chuong, N. N., Tu, N. H. C., Kisiala, A., Hoang, X. L. T., and Thao, N. P. (2020). Role and Regulation of Cytokinins in Plant Response to Drought Stress. Plants (Basel) 9, 10–12. doi:10.3390/plants9040422
Holubová, K., Hensel, G., Vojta, P., Tarkowski, P., Bergougnoux, V., and Galuszka, P. (2018). Modification of Barley Plant Productivity through Regulation of Cytokinin Content by Reverse-Genetics Approaches. Front. Plant Sci. 9, 1676. doi:10.3389/fpls.2018.01676
Jameson, P. E., and Song, J. (2016). Cytokinin: A Key Driver of Seed Yield. Exbotj 67 (3), 593–606. doi:10.1093/jxb/erv461
Jiang, Y., Mi, X., Lin, Y., Wu, H., Gu, T., Ding, J., et al. (2016). Evolution and Expression Patterns of Cytokinin Oxidase Genes in Fragaria Vesca. Scientia Horticulturae 212, 115–125. doi:10.1016/j.scienta.2016.09.037
Joshi, R., Sahoo, K. K., Tripathi, A. K., Kumar, R., Gupta, B. K., Pareek, A., et al. (2018). Knockdown of an Inflorescence Meristem-specific Cytokinin Oxidase - OsCKX2 in rice Reduces Yield Penalty under Salinity Stress Condition. Plant Cel Environ 41, 936–946. doi:10.1111/pce.12947
Kieber, J. J., and Schaller, G. E. (2014). The Arabidopsis Book/American Society of Plant Biologists. Cytokinins 12.
Kopecný, D., Briozzo, P., Popelková, H., Sebela, M., Koncitíková, R., Spíchal, L., et al. (2010). Phenyl- and Benzylurea Cytokinins as Competitive Inhibitors of Cytokinin Oxidase/dehydrogenase: a Structural Study. Biochimie 92, 1052–1062. doi:10.1016/j.biochi.2010.05.006
Koprna, R., De Diego, N., Dundálková, L., and Spíchal, L. (2016). Use of Cytokinins as Agrochemicals. Bioorg. Med. Chem. 24, 484–492. doi:10.1016/j.bmc.2015.12.022
Li, S., An, Y., Hailati, S., Zhang, J., Cao, Y., Liu, Y., et al. (2019). Overexpression of the Cytokinin Oxidase/dehydrogenase (CKX) from Medicago Sativa Enhanced Salt Stress Tolerance of Arabidopsis. J. Plant Biol. 62, 374–386. doi:10.1007/s12374-019-0141-z
Liao, X., Guo, X., Wang, Q., Wang, Y., Zhao, D., Yao, L., et al. (2017). Overexpression ofMsDREB6.2results in Cytokinin-Deficient Developmental Phenotypes and Enhances Drought Tolerance in Transgenic Apple Plants. Plant J. 89, 510–526. doi:10.1111/tpj.13401
Liu, Z., Lv, Y., Zhang, M., Liu, Y., Kong, L., Zou, M., et al. (2013). Identification, Expression, and Comparative Genomic Analysis of the IPT and CKX Gene Families in Chinese Cabbage (Brassica Rapa Ssp. Pekinensis). BMC Genomics 14, 594. doi:10.1186/1471-2164-14-594
Lubovská, Z., Dobrá, J., Štorchová, H., Wilhelmová, N., and Vanková, R. (2014). Cytokinin Oxidase/dehydrogenase Overexpression Modifies Antioxidant Defense against Heat, Drought and Their Combination in Nicotiana Tabacum Plants. J. Plant Physiol. 171, 1625–1633. doi:10.1016/j.jplph.2014.06.021
Macková, H., Hronková, M., Dobrá, J., Turečková, V., Novák, O., Lubovská, Z., et al. (2013). Enhanced Drought and Heat Stress Tolerance of Tobacco Plants with Ectopically Enhanced Cytokinin Oxidase/dehydrogenase Gene Expression. J. Exp. Bot. 64, 2805–2815. doi:10.1093/jxb/ert131
Mao, C., He, J., Liu, L., Deng, Q., Yao, X., Liu, C., et al. (2020). OsNAC2 Integrates Auxin and Cytokinin Pathways to Modulate rice Root Development. Plant Biotechnol. J. 18 (2), 429–442. doi:10.1111/pbi.13209
Mazri, M. A., Belkoura, I., and Meziani, R. (2018). “Use of TDZ for Micropropagation of Some Mediterranean Crop Species,” in Thidiazuron: From Urea Derivative to Plant Growth Regulator (Singapore: Springer), 115–137. doi:10.1007/978-981-10-8004-3_5
Mok, D. W., and Mok, M. C. (2001). Cytokinin Metabolism and Action. Annu. Rev. Plant Physiol. Plant Mol. Biol. 52, 89–118. doi:10.1146/annurev.arplant.52.1.89
Morris, R. O., Bilyeu, K. D., Laskey, J. G., and Cheikh, N. N. (1999). Isolation of a Gene Encoding a Glycosylated Cytokinin Oxidase from maize. Biochem. biophysical Res. Commun. 255 (2), 328–333.
Mrízová, K., Jiskrová, E., Vyroubalová, Š., Novák, O., Ohnoutková, L., Pospíšilová, H., et al. (2013). Overexpression of Cytokinin Dehydrogenase Genes in Barley (Hordeum Vulgare Cv. Golden Promise) Fundamentally Affects Morphology and Fertility. PLoS ONE 8, e79029. doi:10.1006/bbrc.1999.0199
Nguyen, H. N., Kambhampati, S., Kisiala, A., Seegobin, M., and Emery, R. J. N. (2021). The Soybean (Glycine max L.) Cytokinin Oxidase/dehydrogenase Multigene Family; Identification of Natural Variations for Altered Cytokinin Content and Seed Yield. Plant Direct 5, e00308–15. doi:10.1002/pld3.308
Nisler, J., Kopečný, D., Končitíková, R., Zatloukal, M., Bazgier, V., Berka, K., et al. (2016). Novel Thidiazuron-Derived Inhibitors of Cytokinin Oxidase/dehydrogenase. Plant Mol. Biol. 92, 235–248. doi:10.1007/s11103-016-0509-0
Nisler, J., Kopečný, D., Pěkná, Z., Končitíková, R., Koprna, R., Murvanidze, N., et al. (2021). Diphenylurea-derived Cytokinin Oxidase/dehydrogenase Inhibitors for Biotechnology and Agriculture. J. Exp. Bot. 72, 355–370. doi:10.1093/jxb/eraa437
Nisler, J. (2018). “TDZ: Mode of Action, Use and Potential in Agriculture,” in Thidiazuron: From Urea Derivative to Plant Growth Regulator (Singapore: Springer), 37–59. doi:10.1007/978-981-10-8004-3_2
Ogonowska, H., Barchacka, K., Gasparis, S., Jablonski, B., Orczyk, W., Dmochowska-Boguta, M., et al. (2019). Specificity of Expression of TaCKX Family Genes in Developing Plants of Wheat and Their Co-operation within and Among Organs. PLoS ONE 14 (4), e0214239. doi:10.1371/journal.pone.0214239
Pavlů, J., Novák, J., Koukalová, V., Luklová, M., Brzobohatý, B., and Černý, M. (2018). Cytokinin at the Crossroads of Abiotic Stress Signalling Pathways. Int. J. Mol. Sci. 19 (8), 2450. doi:10.3390/ijms19082450
Pospíšilová, H., Jiskrova, E., Vojta, P., Mrizova, K., Kokáš, F., Čudejková, M. M., et al. (2016). Transgenic Barley Overexpressing a Cytokinin Dehydrogenase Gene Shows Greater Tolerance to Drought Stress. New Biotechnol. 33 (5), 692–705. doi:10.1016/j.nbt.2015.12.005
Pospíšilová, J., Vagner, M., Malbeck, J., Travnickova, A., and Batkova, P. (2005). Interactions between Abscisic Acid and Cytokinins during Water Stress and Subsequent Rehydration. Biol. Plant 49 (4), 533–540. doi:10.1007/s10535-005-0039-0
Prerostova, S., Dobrev, P. I., Gaudinova, A., Knirsch, V., Körber, N., Pieruschka, R., et al. (2018). Cytokinins: Their Impact on Molecular and Growth Responses to Drought Stress and Recovery in Arabidopsis. Front. Plant Sci. 9, 655. doi:10.3389/fpls.2018.00655
Prerostova, S., Dobrev, P. I., Kramna, B., Gaudinova, A., Knirsch, V., Spichal, L., et al. (2020). Heat Acclimation and Inhibition of Cytokinin Degradation Positively Affect Heat Stress Tolerance of Arabidopsis. Front. Plant Sci. 11, 87–14. doi:10.3389/fpls.2020.00087
Ramireddy, E., Hosseini, S. A., Eggert, K., Gillandt, S., Gnad, H., von Wirén, N., et al. (2018). Root Engineering in Barley: Increasing Cytokinin Degradation Produces a Larger Root System, Mineral Enrichment in the Shoot and Improved Drought Tolerance. Plant Physiol. 177 (July), 1078–1095. doi:10.1104/pp.18.00199
Rong, C., Liu, Y., Chang, Z., Liu, Z., Ding, Y., and Ding, C. (2021). Cytokinin Oxidase/dehydrogenase Family Genes Exhibit Functional Divergence and Overlap in Ricegrowth and Development, Especially in Control of Tillering. bioRxiv. doi:10.1101/2021.05.09.443313
Song, J., Jiang, L., and Jameson, P. E. (2012). Co-ordinate Regulation of Cytokinin Gene Family Members during Flag Leaf and Reproductive Development in Wheat. BMC Plant Biol. 12 (1), 78–17. doi:10.1186/1471-2229-12-78
Tan, M., Li, G., Qi, S., Liu, X., Chen, X., Ma, J., et al. (2018). Identification and Expression Analysis of the IPT and CKX Gene Families during Axillary Bud Outgrowth in Apple ( Malus Domestica Borkh.). Gene 651, 106–117. doi:10.1016/j.gene.2018.01.101
Thu, N. B. A., Hoang, X. L. T., Truc, M. T., Sulieman, S., Thao, N. P., and Tran, L.-S. P. (2017). Cytokinin Signaling in Plant Response to Abiotic Stresses. Mechanism Plant Horm. signaling under Stress Vol. 1, 71–100. doi:10.1002/9781118889022.ch4
Tsai, Y.-C., Weir, N. R., Hill, K., Zhang, W., Kim, H. J., Shiu, S.-H., Schaller, G. E., and Kieber, J. J. (2012). Characterization of Genes Involved in Cytokinin Signaling and Metabolism from Rice. Plant Physiol. 158 (4), 1666–1684. doi:10.1104/pp.111.192765
U.S. Food and Drug (2022). Types of Genetic Modification Methods for Crops. . https://www.fda.gov/food/agricultural-biotechnology/types-genetic-modification-methods-crops (Accessed February 28, 2022).
Wang, J., Xu, H., Li, N., Fan, F., Wang, L., Zhu, Y., et al. (2015). Artificial Selection of Gn1a Plays an Important Role in Improving rice Yields across Different Ecological Regions. Rice (N Y) 8 (1), 37–10. doi:10.1186/s12284-015-0071-4
Wang, L.-X., Xu, Y.-P., Liu, J.-Q., Luo, X., Zhang, S.-S., Zheng, L.-W., et al. (2020). Cytokinins Affect Shoot Regeneration and Modulate the Expression of IPT and CKX Genes of In Vitro Cultured Eleocharis Dulcis (Brum.f.) Trin. J. Hortic. Sci. Biotechnol. 96 (3), 330–337. doi:10.1080/14620316.2020.1829503
Wang, L., Guo, Y., Jia, L., Chu, H., Zhou, S., Chen, K., et al. (2014). Hydrogen Peroxide Acts Upstream of Nitric Oxide in the Heat Shock Pathway in Arabidopsis Seedlings. Plant Physiol. 164, 2184–2196. doi:10.1104/pp.113.229369
Werbrouck, S. P. O. (2016). Topolin-derived Cytokinins and Cytokinin Oxidase Inhibitors Offer New Tools to Study In Vitro Plant Development. IX Int. Symp. vitro Cult. Hortic. Breed. 1187, 101–106. doi:10.17660/ActaHortic.2017.1187.12
Werner, T., Motyka, V., Laucou, V., Smets, R., Van Onckelen, H., and Schmülling, T. (2003). Cytokinin-deficient Transgenic Arabidopsis Plants Show Multiple Developmental Alterations Indicating Opposite Functions of Cytokinins in the Regulation of Shoot and Root Meristem Activity. Plant Cell 15 (11), 2532–2550. doi:10.1105/tpc.014928
Werner, T., Nehnevajova, E., Köllmer, I., Novák, O., Strnad, M., Krämer, U., et al. (2010). Root-Specific Reduction of Cytokinin Causes Enhanced Root Growth, Drought Tolerance, and Leaf Mineral Enrichment in Arabidopsis and Tobacco. Plant Cell 22 (12), 3905–3920. doi:10.1105/tpc.109.072694
Yamburenko, M. V., Kieber, J. J., and Schaller, G. E. (2017). Dynamic Patterns of Expression for Genes Regulating Cytokinin Metabolism and Signaling during rice Inflorescence Development. PLoS ONE 12 (4), e0176060–18. doi:10.1371/journal.pone.0176060
Yeh, S.-Y., Chen, H.-W., Ng, C.-Y., Lin, C.-Y., Tseng, T.-H., Li, W.-H., et al. (2015). Down-regulation of Cytokinin Oxidase 2 Expression Increases Tiller Number and Improves rice Yield. Rice 8, 36. doi:10.1186/s12284-015-0070-5
Zalewski, W., Gasparis, S., Boczkowska, M., Rajchel, I. K., Kała, M., Orczyk, W., et al. (2014). Expression Patterns of HvCKX Genes Indicate Their Role in Growth and Reproductive Development of Barley. PLoS ONE 9 (12), e115729. doi:10.1371/journal.pone.0115729
Zatloukal, M., Gemrotová, M., Doležal, K., Havlíček, L., Spíchal, L., and Strnad, M. (2008). Novel Potent Inhibitors of A. Thaliana Cytokinin Oxidase/dehydrogenase. Bioorg. Med. Chem. 16 (20), 9268–9275. doi:10.1016/j.bmc.2008.09.008
Zhang, L., Zhao, Y. L., Gao, L. F., Zhao, G. Y., Zhou, R. H., Zhang, B. S., et al. (2012). TaCKX6‐D1 , the Ortholog of rice OsCKX2 , Is Associated with Grain Weight in Hexaploid Wheat. New Phytol. 195, 574–584. doi:10.1111/j.1469-8137.2012.04194.x
Keywords: cytokinins, cytokinin oxygenase/dehydrogenase, inhibitors, stress tolerance, overexpression
Citation: Arora K and Sen S (2022) Cytokinin Oxygenase/Dehydrogenase Inhibitors: An Emerging Tool in Stress Biotechnology Employed for Crop Improvement. Front. Genet. 13:877510. doi: 10.3389/fgene.2022.877510
Received: 16 February 2022; Accepted: 04 March 2022;
Published: 24 March 2022.
Edited by:
Jyoti mathur, Banasthali University, IndiaReviewed by:
Md Mahmudul Hassan, Patuakhali Science and Technology University, BangladeshCopyright © 2022 Arora and Sen. This is an open-access article distributed under the terms of the Creative Commons Attribution License (CC BY). The use, distribution or reproduction in other forums is permitted, provided the original author(s) and the copyright owner(s) are credited and that the original publication in this journal is cited, in accordance with accepted academic practice. No use, distribution or reproduction is permitted which does not comply with these terms.
*Correspondence: Kavita Arora, ZHJrYXJvcmExN0BnbWFpbC5jb20=; Sangeeta Sen, c2Vuc2FuZ2VldGFAZ21haWwuY29t
†These authors share first authorship