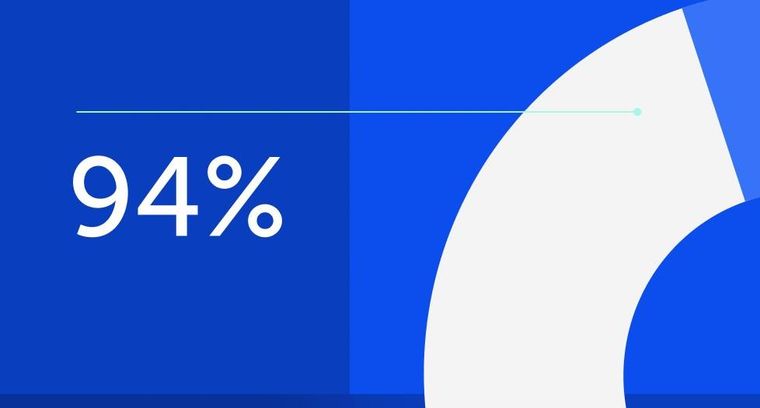
94% of researchers rate our articles as excellent or good
Learn more about the work of our research integrity team to safeguard the quality of each article we publish.
Find out more
ORIGINAL RESEARCH article
Front. Genet., 24 June 2022
Sec. RNA
Volume 13 - 2022 | https://doi.org/10.3389/fgene.2022.875939
This article is part of the Research TopicGenetic and epigenetic aspects of non-coding RNAs in physiology and diseaseView all 11 articles
Cancer development by the human papillomavirus (HPV) infection can occur through the canonical HPV/p53/RB1 pathway mediated by the E2/E6/E7 viral oncoproteins. During the transformation process, HPV inserts its genetic material into host Integration Sites (IS), affecting coding genes and miRNAs. In penile cancer (PeCa) there is limited data on the miRNAs that regulate mRNA targets associated with HPV, such as the TP53 and RB1 genes. Considering the high frequency of HPV infection in PeCa patients in Northeast Brazil, global miRNA expression profiling was performed in high-risk HPV-associated PeCa that presented with TP53 and RB1 mRNA downregulated expression. The miRNA expression profile of 22 PeCa tissue samples and five non-tumor penile tissues showed 507 differentially expressed miRNAs: 494 downregulated and 13 upregulated (let-7a-5p, miR-130a-3p, miR-142-3p, miR-15b-5p miR-16-5p, miR-200c-3p, miR-205-5p, miR-21-5p, miR-223-3p, miR-22-3p, miR-25-3p, miR-31-5p and miR-93-5p), of which 11 were identified to be in HPV16-IS and targeting TP53 and RB1 genes. One hundred and thirty-one and 490 miRNA binding sites were observed for TP53 and RB1, respectively, most of which were in seedless regions. These findings suggest that up-regulation of miRNA expression can directly repress TP53 and RB1 expression by their binding sites in the non-canonical seedless regions.
The TP53 gene is known as the “sentinel gene” due to its ability to identify cell damage and to coordinate complex mechanisms to mediate cell repair, protecting genome stability and, consequently, cell homeostasis. Therefore, it is not surprising that TP53 tumor suppressor is the most frequently mutated gene in human tumors. Most of the alterations described are missense mutations, whereby the protein loses its primary function, or it acquires oncogenetic functions (Datta et al., 2017; Wang and Sun, 2017; Sammons et al., 2020). In addition, the p53 mutant protein may also facilitate the adaptation of tumor cells to the disadvantageous environment that arises as the tumor grows (Mantovani et al., 2019).
The association between human papillomavirus (HPV) and some cancers, including cervical, head and neck, vulvar, anorectal, and penile squamous cell carcinomas (SCC), is well characterized by the canonical mechanism involving the HPV oncogenes E6 and E7 and p53 and RB1 proteins (de Martel et al., 2017). During the transformation process, HPV inserts its genetic material into host human integration sites (IS), which have been identified in regions harboring cancer-related genes, as well as in regions presenting copy number alterations (CNAs) (Busso-Lopes et al., 2015; Macedo et al., 2020; Pinatti et al., 2021). Indeed, integration of DNA-copy number alterations and other omics data have shown that DNA methylation, mRNA, and miRNA expressions alterations affect coding-genes and miRNAs located within or near the HPV common integration sites (Barzon et al., 2014; Groves and Coleman, 2018; Rosa et al., 2019; Pinatti et al., 2021).
In the last decade, it has been also demonstrated that the wild-type p53 protein plays primarily its role as a transcription factor by regulating a large network of protein-coding genes and non-coding RNAs, including miRNAs, both inducing or repressing their targets (Hermeking, 2012; Fischer, 2017). In addition, the p53 protein regulates miRNA processing, primarily through its central DNA-binding domain, a target site of most cancer-specific mutations. Interestingly, miRNAs can also regulate p53 expression by matching in the seed region into the 3′UTR of TP53 mRNA, directly inducing the repression of TP53 or its regulators (Hermeking, 2012; Hermeking et al., 2014). Although several tumor-specific alterations in the p53-miRNA network have been described in different cancers (Hermeking, 2012; Datta et al., 2019), there is no data on miRNAs targeting of TP53 gene in HPV-associated penile cancer.
PeCa is a rare carcinoma in developed countries, but it presents higher incidence rates in South America, Asia, and Africa, where limited economic and social conditions play a large impact leading to delay in diagnosis, and treatment initiation. In Brazil, specifically in the Northeast region that is particularly affected by low socio-economic conditions and educational levels and high frequency of HPV infection, presents a high incidence of PeCa, with patients presenting additional comorbidities, which contributes to a high incidence of mortality rates (Macedo et al., 2020; Silva et al., 2021) However, even in countries that are not impacted by major economic limitations, the incidence and mortality rates of PeCa has increased, mainly among younger patients (Hansen et al., 2018). Hence, the increased occurrence of PeCa, irrespectively of the countries’ socioeconomic conditions, has suggested that HPV infection is possibly the main triggering mechanism for tumor development, in addition to poor hygiene of the genital region, phimosis, uncircumcision, and chronic inflammation (Christodoulidou et al., 2015; Kidd et al., 2017; Adashek et al., 2019).
PeCa treatment options are limited. No effective target therapy is available, mainly due to the scarcity of knowledge on the molecular pathways involved in the development and progression of these tumors. Limited data is available on the role and mechanisms of miRNA deregulation in PeCa, including those that disrupt miRNAs targets that regulate critical genes associated with the action of HPV, such as the TP53 and RB1 genes. Considering the high frequency of HPV infection in patients with advanced PeCa in Maranhão State, in Northeast of Brazil, in the present study miRNAs expression analysis was performed in high-risk HPV-associated PeCa with TP53 and RB1 mRNA downregulated expression, as previously reported by our group in >80 and 60% of the patients, respectively (Macedo et al., 2020).
This study opens the opportunity to better understand the role of TP53 and RB1 transcriptional regulators in HPV-associated penile carcinomas and brings much needed knowledge on the molecular tumorigenesis of this still-neglected tumor.
Fresh PeCa chemotherapy-naive surgical resection tissue specimens were obtained from 22 patients from the Aldenora Hospital, São Luís, Maranhão, Brazil. These patients are a subset of a larger cohort of 37 patients previously investigated for HPV status, gene, and protein expression for TP53 and RB1 (Macedo et al., 2020). All the samples were collected under patients’ written informed consent, approved by the Research Ethics Committee on Humans from the Federal University of Maranhão and by the National Research Ethics Commission (CONEP-Brazil, CAAE: 46371515.5.0000.5087). Tumor and adjacent non-tumor tissues, sampled from 2 cm distant from the tumor site after histopathological assessment, were obtained before any cancer treatment. At the time of the sample collection, the patients had no history of other cancers or sexually transmitted diseases.
The clinical and histopathological variables were obtained from patients’ medical records. The mean age of the patients at diagnosis was 64.22 ± 15.63 years, ranging from 32 to 85 years old. The patients declared themselves smokers (41%) and alcoholics (45.5%). All tumors were classified as squamous cell carcinoma (SCC), and the condylomatous and keratinized histological subtypes localized mostly in the glans, corpus cavernosum, and corpus spongiosum were the most frequent, 45.4 and 36.4%, respectively. Tumor grades II and III were the most frequent, present in 54.5 and 27.3% of the patients, respectively. Lymphatic and perineural invasion were positive in 18.2 and 22.8% of the patients, who presented mostly ulcerated lesions (68.2% of the cases), followed by vegetative (18.2%) and verrucous (13.6%). Penectomy (partial and total) was performed in 95.4% of the patients. The primary tumor of each patient was positive for HPV by Nested-PCR and DNA sequencing, as described in Macedo et al. In this subset, the multiple infections were detected in 50.0% of the cases. The HPV16 genotype was the most frequent (72.2%), followed by the 74 (16.6%), 30, 59 and 66 (11%, each) genotypes. Genotypes found in lower frequencies were 6, 18, 30, 35, 44, 53, 58, and 73 (Supplementary Table S1). Four cases were positive for HPV, but not genotyped since the samples did not have sufficient DNA for Nested-PCR and/or DNA sequencing analysis. Table 1 and Supplementary Table S1 present the detailed patients’ clinical-histopathological information.
TABLE 1. Clinical-histopathological profile of patients diagnosed with HPV positive penile carcinoma (n = 22).
Considering our previous study (Macedo et al., 2020) in which we have demonstrated TP53 and RB1 down-regulated expression at both mRNA (by real-time PCR) and protein (by immunohistochemistry) levels (86 and 65% of the cases, respectively), in this study, we investigated the possible mechanisms by which these genes might be repressed in HPV-associated PeCa. For that, a subset of 22 tumors was evaluated for differential miRNA expression in relation to adjacent non-tumor tissues (n = 5). Fifteen of the 22 tumors have data on the expression of TP53 and RB1 (73 and 69% of the tumors are underexpressed, respectively) (Supplementary Table S1). Subsequently, prediction miRNAs binding sites analysis were performed in the TP53 and RB1 gene, followed by a search for molecular pathways potentially involved in penile carcinogenesis in HPV-positive patients.
Total RNA from 22 PeCa tumors and five adjacent non-tumor tissues was isolated using the TRIzol protocol (Invitrogen Carlsbad, CA, United States). RNA concentration and quality were tested by measuring the 260/280 and 260/230 ratios using the Nanodrop 2001 spectrophotometer (Willington, DE, United States). Expression of miRNAs was determined using the nCounter® Human v.3 miRNA expression platform (Nanostring Technologies™, Seattle, Wa, United States), which contains human probes from miRBase v.22 (http://www.mirbase.org) targeting 827 human miRNAs, six positive controls, eight negative controls, three positive binding controls, three negative binding controls, five internal reference genes (ACTB, B2M, GAPDH, RPL19, and RPL0) and five miRNA controls (ath-miR- 159a, cel-miR-248, cel-miR-254, osa-miR-414, and osa-miR-442) as previously reported at the Molecular Oncology Research Center (Pessôa-Pereira et al., 2020; Causin et al., 2021). The raw data were pre-processed and exported as RCC files. The raw data of the study, as well as the clinical information of the patients are available for access from the Gene Expression Omnibus (GEO), under registration GSE197121.
The raw data were normalized and analyzed using the ROSALIND® Nanostring platform (https://rosalind.onramp.bio/). Adjacent non-tumor tissues distant 2 cm for the primary tumor were used as control. Read distribution percentages, identity heatmaps, and sample MDS plots were generated as part of the QC step. The normalization was conducted following the background subtraction based on POS_A probes correction factors (positive control normalization and codeset normalization). For both steps, the geometric mean of each probeset was used to create a normalization factor. The fold changes, p-values for comparisons were calculated using the t-test method (p ≤ 0.05). p-value adjustment was performed using the Benjamini–Hochberg (p ≤ 0.01) method to estimate false discovery rates (FDR). The clustering of miRNAs for the final heatmap was constructed using the PAM (Partitioning Around Medoids) through a method using the FPC R library (Hennig, 2020) that takes into account the direction and type of all signals in a pathway, the position, function, and type of each miRNA identified. Fold change (≥2 for miRNAs upregulated and ≤-2 for miRNAs downregulated), p-value and adjusted p-value were used as selection criteria for miRDE.
The STarMir software (Kanoria et al., 2016) was used to identify the miRNAs binding regions in TP53 and RB1 genes (CLIP-data). The construction design and nucleic acid fold of STarMir are obtained from the Mfold package (Zuker, 2003) and Sfold which contains the Srna module (Ding et al., 2004). Sfold applies a two-step model for hybridization between mRNA and miRNA. In this model, hybridization of the miRNA-target occurs at an accessible target site and then the hybrid elongates to form the complete miRNA-target duplex. The minimum free energy of hybridization was obtained from the RNAhybrid tool (Rehmsmeier et al., 2004; Long et al., 2007). Only interactions in “seed” and “seedless” regions with LogitProb values ≥0.5; ΔGhybrid ≤ -10.00 and site-access ≥ 0.4 were considered.
Pathway’s enrichment analysis was performed by miRPath v.3 - DIANA TOOLS software (Vlachos et al., 2015) using the Tarbase prediction algorithm and considering the p-value threshold ≤0.05. The generated pathways are part of the Kyoto Encyclopedia of Genes and Genomes (KEGG). The TP53 and RB1 genes were used as filters to generate KEGG pathways. The ‘pathways union’ function was used to generate the related top pathways, considering the p-value threshold ≤0.05 and enrichment analysis method by Fisher’s Exact Test.
Differential miRNA expression analysis was performed in the 22 PeCa tissues. The global miRNA expression profile of these tumors showed 507 differentially expressed miRNAs (miRDE) compared to a group of five adjacent non-tumor penile tissues. Among these miRDE, 494 (97.4%) miRNAs were downregulated and 13 (2.6%) upregulated (Figure 1; Table 2). Considering the previously detected lower TP53 mRNA expression (85.7% (12/14) and lower protein expression in 87.5% (14/16) of these cases (Macedo et al., 2020), we further investigated the up-regulated miRNAs in the subset of 22 tumors, of which 73% were downregulated. The miRDEs let-7a-5p, miR-130a-3p, miR-15b-5p, miR-21-5p, and miR-25-3p were overexpressed in 100% of cases. Interestingly, 84.6% (n = 11) of miRDEs were found to be located at HPV integration sites. The HPV integration sites were identified as target regions of the oncogenic HPV16 genotype, the most frequently detected genotype in our study cohort (Supplementary Table S1).
FIGURE 1. The box-plots represent a comparison of the relative expression levels of the 13 miRDE upregulated in tumors (n = 22) versus normal tissue (n = 5); p ≤ 0.05 by t-test and adj.-p ≤ 0.01 by Benjamini–Hochberg; *p ≤ 0.05, **p ≤ 0.01 and ***p ≤ 0.001.
TABLE 2. Thirteen differentially expressed miRNAs observed upregulated in the PeCa patients, and their respective chromosomal location and HPV integration sites (presented by miRNA number).
Figure 1 Relative expression of thirteen miRNAs upregulated (tumor vs. non-tumor) in the PeCa studied.
Prediction of miRNA binding sites revealed that all 13 up-regulated miRNAs targeted the TP53 gene, acting as negative regulators of this tumor suppressor gene expression. We found 131 target sites for these miRNAs: 98.5% in the non-canonical seedless regions and two in the seed regions (Supplementary Table S2). Interestingly, TP53 presents 129 seedless sites, in which all 13 differentially expressed miRNAs could bind. The coding region presented the highest number of target seedless regions with 81/129 sites (62.8%), followed by 3′UTR with 32/129 sites (24.8%) and 5′UTR with 16/129 sites (12.4%). Bindings in the gene seed regions were observed to occur with miR-22-3p and let-7a-5p and both interactions were of 8mer-type. The binding between let-7a-5p and TP53 occurred in a canonical 3′UTR region, while the binding of miR-22-3p occurred in a non-canonical coding region (site position: 534–570 (bp); seed position: 564–570 (bp). This region is highly conserved (site conservation = 0.963 and seed conservation = 0.933). Our analysis also revealed that miR-93-5p and let-7a-5p can bind to a higher number of seedless regions, 21 and 20 predicted binding sites, respectively, while miR-15b-5p, miR-16-5p, miR-223-5p, miR-22-5p, and miR-31-5p bind to a lower number of regions, i.e., five predicted sites for each. Considering the size of the TP53 mRNA (2,591 bp; transcript variant 1, NCBI Reference Sequence: NM_000546.5) we observed that the 1,000–1073bp, 2,500–2580bp, and 835–899bp intervals are miRNA binding hotspots regions, harboring a total of 22, 16 and 14 sites, respectively. Figure 2; Table 3 show the ten main binding sites observed in the TP53 gene.
FIGURE 2. From (A–J), hybrid mRNA (TP53)—miRNA upregulated. The upper and lower sequences represent the miRNA in the 3′-5′ sense and 5′-3′ sense hybridization sites in the mRNA, repectively. The numbers at the ends of the mRNA show the starting and ending position of the hybridization sites. Dots between the sequences indicate the paired nucleotides. Nucleotides in red mark the “seed” sequence in miRNA. The energy resulting from the hybridization was calculated by the RNAhybrid algorithm.
Figure 2 Top 10 miRNAs binding site regions identified in the TP53 gene.
The lower expression of RB1 gene was also found in 69% of the tumors. Interestingly, we observed that the thirteen overexpressed miRNAs that down-regulated TP53 also regulated RB1 expression (Figure 3). A total of 490 miRNA binding sites were identified for RB1 (Supplementary Table S3), of which 477 (97.3%) were located in the non-canonical seedless regions, while 13 (2.7%) were in the seed regions. Bindings in the seed regions occurred with seven overexpressed miRNAs (miR-93-5p, let-7a-5p, miR-25-3p, miR-130a-3p, miR-200c-3p, miR-205-5p, and miR-142-3p), most of which were 7mer-A1 (46.2%). Other binding sites identified in the seed regions were offset-6mer (23.0%), 6mer, and 7mer-m8 (15.4%, each) (Supplementary Table S2A). The RB1 gene also presented the highest number of miRNA target sites in seedless regions (490 in total), in which all 13 differentially expressed miRNAs can bind. The RB1 coding region also had the highest number of seedless regions (57.0%), followed by 3′UTR (42.4%) and 5′UTR (0.6%). The miRNA let-7a-5p showed the highest number of seedless bindings (77 predicted sites), followed by miR-93-5p (74 predicted sites). The miRNAs presenting a smaller number of regions were miR-16-5p, miR-205-5p, miR-223-3p (22 predicted sites, each) and miR-22-3p (19 predicted sites). The RB1 gene also presented hotspots regions where several miRNAs can bind. The intervals between 2,202–2297pb and 1906–1997 pb house a total of 21, and 19 sites, respectively (NCBI Reference Sequence: NM_000321.3). Table 3 shows the top 10 binding sites in RB1.
FIGURE 3. From (A–J), hybrid mRNA (RB1)—miRNA upregulated. The upper and lower sequences represent the miRNA in the 3′-5′ sense and 5′-3′ sense hybridization sites in the mRNA, respectively. The numbers at the ends of the mRNA show the starting and ending position of the hybridization sites. Dots between the sequences indicate the paired nucleotides. Nucleotides in red mark the “seed” sequence in miRNA. The energy resulting from the hybridization was calculated by the RNAhybrid algorithm.
Figure 3 Top 10 miRNAs binding site regions identified in the RB1 gene.
KEGG pathway analysis was performed to identify the involvement of the 13 upregulated miRNAs above in disease and signaling pathways. This analysis revealed a total of 13 KEGG pathways (Supplementary Table S3), of which the top was: viral carcinogenesis (hsa05203) (p=<1.00 × 10−325), central carbon metabolism in cancer (hsa05230) (p = 3.39 × 10−06), chronic myeloid leukemia (hsa05220) (p = 1.33 × 10−05), glioma (hsa05214) (p = 0.0064), melanoma (hsa05218) (p = 0.0120) and cell cycle (hsa04110) (p = 0.0224) (Figure 4; Table 4).
FIGURE 4. Top pathways identified by the tarbase algorithm (p < 0.05; DIANA/miRPath v.3). Red colors indicate a stronger role of the miRNAs on a given pathways as compared to the lighter colors.
Figure 4 Unsupervised hierarchical grouping of the 13 miRNAs differentially expressed and top related pathways.
It is well known that the integration of the human papillomavirus (HPV) can occur at or near cancer-related genes (Durst et al., 1987). However, it is not completely understood the mechanisms by which the HPV virus controls its integration into the host cell genome and the molecular consequences that ultimately lead to the development and progression of the HPV infected tumors. Studies have used high-performance technologies to identify virus integration sites in the host genome to better understand the molecular alterations that occur in the host cell, leading to the loss of its genomic stability (Akagi et al., 2014; Bodelon et al., 2016; Liu et al., 2016; Gao et al., 2017; Rosa et al., 2019). The most well-known example is the canonical HPV/TP53/RB1 signaling pathway initiated by the viral E2 disruption. This leads to the loss of the negative feedback control of the viral oncoproteins E6 and E7, mediating ubiquitination and degradation of the p53 and pRb proteins, respectively (Squarzanti et al., 2018). Other authors proposed that HPV integration also directly causes activation of oncogenes or inactivation of tumor suppressors, as reported in HPV-related squamous cell carcinomas (Parfenov et al., 2014; Hu et al., 2015).
In our previous study, we showed downregulated mRNA expression of the TP53 and RB1 genes in 86 and 65% of high-risk HPV-associated PeCa, respectively. In the present subset of cases, we evaluated miRNAs’ expression, and observed that 73 and 69% were downregulated for both genes, respectively, suggesting the existence of other regulatory mechanisms in addition to the canonical HPV/TP53/RB1 pathway (Macedo et al., 2020). Although not all the cases presented with expression alterations in these genes, these results were recently corroborated by Furuya et al., who also described TP53 reduced expression levels in penile tumors.
Compared to other cancers (Santos et al., 2018; Datta et al., 2019; Hussen et al., 2021; Liu et al., 2021), few studies have described epigenetic events in penile tumors, whether evaluating miRNAs (Zhang et al., 2015; Hartz et al., 2016; Kuasne et al., 2017; Peta et al., 2017; Ayoubian et al., 2021; Furuya et al., 2021) or by evaluating methylation patterns (Feber et al., 2015; Kuasne et al., 2015; Marchi et al., 2017). Changes by both mechanisms could justify the downregulation of TP53 and RB1, however only RB1 has been reported to be hypermethylated (Marchi et al., 2017). Additionally, as most pathogenic variants of these genes have been described in coding regions, the mRNA downregulation of TP53 and RB1 does not appear to be due to mutations (Feber et al., 2016; Wang et al., 2019; Chahoud et al., 2021). Furthermore, few studies have evaluated patients’ cohorts with a high incidence of HPV infection, remaining poorly known the impact of HPV infection in disrupting mRNA/miRNA networks in penile tumors (Zhang et al., 2015; Hartz et al., 2016; Kuasne et al., 2017; Ayoubian et al., 2021; Furuya et al., 2021). In the present study, our main goal was to determine whether altered miRNAs expression could be associated with the down-regulation of the TP53 and RB1 expression in the etiopathogenesis of HPV-associated PeCa. This goal is of critical relevance to these particular virus associated with infected tumors, considering that the patient cohort investigated, from the State of Maranhão in Northeastern Brazil, is characterized by advanced PeCa and a high rate of HPV infection (>90%), as we reported previously (Coelho et al., 2018; Macedo et al., 2020). In addition, this study can provide useful information to target HPV-specific molecular pathways in human cancers.
In the present study, all patients were tested for HPV by nested-PCR followed by DNA sequencing. Using two highly sensitive methods we successfully detected HPV infection in 100% of men with PeCa, all of them with high-risk subtypes. Despite the high HPV prevalence in all human populations, occurring as hundreds of types, subtypes, and variants, many of them are not associated with cancer. On the other hand, it is well established the correlation between high-risk HPV and severe dysplasia, in situ and invasive cancer, usually as monoclonal lesions due to clonal selection from less advanced precursors (Pontén and Guo, 1998). This may explain why we were able to detect HPV in 100% of the primary tumor since all of them presented high-risk genotypes.
It is well established that p53 regulates the expression of both protein-coding genes and non-coding RNAs (Hermeking, 2012; Fischer, 2017). TP53-regulated miRNAs can mediate tumor suppression in response to cellular stress; similarly, the expression and activity of p53 can also be under the control of miRNAs (Hermeking, 2012). More than 20 miRNAs have been described to directly regulate p53 via canonical bindings (seed) in 3′UTR (reviewed by Liu et al., 2017). Down regulation of TP53 through seed sequences induce phenotypes that are consistent with loss of p53 function, such as reduced apoptosis, cellular senescence, increased invasion, and growth of tumor cells (Hermeking, 2012; Deng and Sui, 2013; Hermeking et al., 2014). Despite the increasing number of miRNAs that form the TP53 mRNA/miRNAs interaction network, there is no information on TP53-repressor miRNAs in HPV-associated PeCa. Our data revealed a total of 507 differentially expressed miRNA (miRDE) between the tumor and non-tumor tissue of HPV-infected PeCa patients, of which 494 were downregulated and 13 were upregulated. Among the 13 miRDE upregulated, five (let-7a-5p, miR-130a-3p, miR-15b-5p, miR-21-5p and miR-25-3p) were found overexpressed in 100% of the tumors analyzed. Moreover, miR-130a-3p, miR-15b-5p and miR-31-5p were predicted as novel regulator for TP53 gene; while miR-142-3p, miR-200c-3p, miR-205-5p, miR-223-3p, miR-22-3p, miR-25-3p and miR-31-5p for RB1.
Several studies have shown up-regulated expression of these miRNAs in several types of tumors. Overexpression of let-7a-5p has been observed in HCV-related cirrhosis (Petkevich et al., 2021) and liver cancer and ovarian cancer, where it presents a non-invasive diagnostic potential (Liu et al., 2021). Corroborating our data, some studies have also suggested that TP53 is a target of let-7a-5p (Balakrishnan et al., 2014; Pillai et al., 2014; Nunez Lopez et al., 2019; Zhou et al., 2019). MiR-130a-3p is recognized as a miRNA with tumor suppressor action (Kong et al., 2018; Song et al., 2021), that may act directly (Causin et al., 2021) or indirectly (Hu et al., 2021) in cancer progression. On the other hand, miR-15b-5p has generally been described to act on cell proliferation mechanisms, such as the ones involving the LATS2 (Liu et al., 2020), BCL-2 (Zhang et al., 2015), and PTPN4/STAT3 pathways (Liu et al., 2020).
Interestingly, down-regulation of TP53 by miR-25 resulted in a decrease in apoptosis in HCT116 colon cancer cells, A549 cells, NSCLC, and multiple myeloma cells (Kumar et al., 2011). In lung cancer, miR-25 was observed to promote cell proliferation and also inhibit apoptosis by down-regulating the expression of the MOAP1/TP53 axis genes (Wu et al., 2015). Moreover, recent evidence shows that miR-25-3p may also act with LncRNAs on a LINC00858/miR-25/SMAD7 axis modulating TP53-wild expression in colorectal carcinoma (Zhan et al., 2020). (Wang et al., 2021) also observed that exosomal miR-25-3p induced cell proliferation and resistance to temozolomide in glioblastoma through down-regulation of FBXW7, promoting c-Myc and cyclin E expression. MiR-21-5p, also observed up-regulated in this study, was shown by (Huang et al., 2021) to negatively regulate the tumor suppressor PDCD4 and cause resistance to by Osimertinib by interfering with MEK/ERK signaling.
These results are in concordance with the suppressive effect of these miRNAs in the HPV-related genes observed in PeCa in the present study. Our computational analysis revealed that TP53 and RB1 have 131 and 490 target sites for the 13 upregulated miRNAs, respectively. The highest number of miRNA binding sites were identified in coding regions, and not in UTR regions, as reported by (Hafner et al., 2010) Furthermore, 98.5 and 97.3% of the sites in TP53 and RB1, respectively, are in non-canonical seedless regions, presenting high levels of complementarity and conservation. Although most miRNA targets have sites that are perfectly complementary to the seed region, it has been shown that miRNAs can directly interact with seedless binding sequences, even improving their function (Shin et al., 2010). (Lal et al., 2009) for example, presented evidence of cell proliferation control by miR-24 in the E2F2/MYC axis through seedless binding in the 3′UTR region. (Park et al., 2017). also showed that destabilization of miRNAs targets is dramatically increased when binding occurs in non-canonical seedless regions. Therefore, we propose that the 13 miRNAs overexpressed in PeCa directly repress TP53 and RB1 by silencing their messenger RNA at different binding sites, especially in non-canonical seedless regions.
Although a unique miRNA may have a pivotal role in a particular pathway, most miRNAs act targeting multiple mRNAs, affecting the same or several gene pathways. Considering the 13 upregulated miRNAs, we predicted six main pathways by the enrichment analysis. Viral carcinogenesis (hsa05203), in which TP53 and RB1 act, was the main pathway affected (p < 1.00 × 10-325). MiR-205-5p, miR-16-5p, miR-22-3p, miR-93-5p, let-7a-5p, and miR-130a-3p were involved in most of the pathways affected. Glioma and cell cycle pathways, in addition to viral carcinogenesis, were previously shown to be regulated by other miRNAs identified in cytobands affected by CNVs in the same population from Maranhão State (Silva et al., 2021). Thus, these current findings reinforce the involvement of these pathways in HPV-associated penile tumorigenesis.
It is worth highlighting that 84.6% of miRDE were observed to be located in HPV integration sites (HPV-IS), including the five miRDE overexpressed in 100% of cases (sites at 3q25.33, 7q22.1, 9q22.32, 11q12.1, and 17q23.1). Several viruses mediate tumorigenesis by expressing viral oncogenes or activating host oncogenes through the integration of viral DNA into the human genome (Lee and Dutta, 2009; Tuna and Amos, 2017). We have recently shown that chromosomal regions with gene copy number alterations (CNA) are present in HPV-IS, such as 2p12-p11.2 and 14q32.33 (observed in 100% of PeCa patients), which can also affect the expression of miRNAs located in these regions (Macedo et al., 2020; Silva et al., 2021). These regions were also described in other HPV-associated tumors (Wentzensen et al., 2004; Kumar Gupta and Kumar, 2015; Holmes et al., 2016; Liu et al., 2016). This data shows the close connection of CNAs and miRNA deregulation located in HPV-IS. Altogether, our present data, support that miRNAs located in HPV-IS can directly repress genes related to HPV infection, such as TP53 and RB1, highlighting HPV insertion as one of the factors that trigger epigenetic mechanisms.
In this study, we suggest that the HPV-related genes, TP53 and RB1, are directly down-regulated by 13 miRNAs located in high-risk HPV integration sites, notably for the HPV16 subtype, present in 72% of the PeCa patients studied. Considering that the expression and activity of TP53 and RB1 can be under the control of miRNAs, our findings provide a new understanding of the role of high-risk HPV infection in penile tumorigenesis through an epigenetic mechanism.
The datasets presented in this study can be found in online repositories. The names of the repository/repositories and accession number(s) can be found below: https://www.ncbi.nlm.nih.gov/geo/, GSE197121.
The studies involving human participants were reviewed and approved by Research Ethics Committee on Humans from the Federal University of Maranhão and by the National Research Ethics Commission (CONEP-Brazil, CAAE: 46371515.5.0000.5087). The patients/participants provided their written informed consent to participate in this study.
Conceptualization, SP and JS; Methodology, SP, JS, RR, AL, LS, and CC; Formal Analysis, SP, AK, IF, and JS; Investigation, JS and SP; Resources, SP and AK; Writing—Original Draft Preparation, SP and JS; Writing—Review and Editing, SP and LC; Visualization, LC and AK; Supervision, SP; Project Administration, SP; Funding Acquisition, SP and AK.
This research was funded by Fundação de Amparo à Pesquisa e ao Desenvolvimento Científico e Tecnológico do Maranhão (FAPEMA)—Grant number IECT-05551/18 and Uniscience—nanoString miRNA Grant for SP, and by Comissão de Aperfeiçoamento de Pessoal do Nível Superior (CAPES; code 001) and for providing scholarship for JS. “The APC was funded by PROPESP-FEDERAL UNIVERSITY OF PARÁ”.
The authors declare that the research was conducted in the absence of any commercial or financial relationships that could be construed as a potential conflict of interest.
All claims expressed in this article are solely those of the authors and do not necessarily represent those of their affiliated organizations, or those of the publisher, the editors and the reviewers. Any product that may be evaluated in this article, or claim that may be made by its manufacturer, is not guaranteed or endorsed by the publisher.
The Supplementary Material for this article can be found online at: https://www.frontiersin.org/articles/10.3389/fgene.2022.875939/full#supplementary-material
Adashek, J. J., Necchi, A., and Spiess, P. E. (2019). Updates in the Molecular Epidemiology and Systemic Approaches to Penile Cancer. Urologic Oncol. Seminars Orig. Investigations 37 (7), 403–408. doi:10.1016/j.urolonc.2019.04.012
Akagi, K., Li, J., Broutian, T. R., Padilla-Nash, H., Xiao, W., Jiang, B., et al. (2014). Genome-wide Analysis of HPV Integration in Human Cancers Reveals Recurrent, Focal Genomic Instability. Genome Res. 24 (2), 185–199. doi:10.1101/gr.164806.113
Ayoubian, H., Heinzelmann, J., Hölters, S., Khalmurzaev, O., Pryalukhin, A., Loertzer, P., et al. (2021). MiRNA Expression Characterizes Histological Subtypes and Metastasis in Penile Squamous Cell Carcinoma. Cancers 13 (6), 1480. doi:10.3390/cancers13061480
Balakrishnan, I., Yang, X., Brown, J., Ramakrishnan, A., Torok-Storb, B., Kabos, P., et al. (2014). Genome-Wide Analysis of miRNA-mRNA Interactions in Marrow Stromal Cells. Stem Cells 32 (3), 662–673. doi:10.1002/stem.1531
Barzon, L., Cappellesso, R., Peta, E., Militello, V., Sinigaglia, A., Fassan, M., et al. (2014). Profiling of Expression of Human Papillomavirus-Related Cancer miRNAs in Penile Squamous Cell Carcinomas. Am. J. Pathology 184 (12), 3376–3383. doi:10.1016/j.ajpath.2014.08.004
Bodelon, C., Untereiner, M. E., Machiela, M. J., Vinokurova, S., and Wentzensen, N. (2016). Genomic Characterization of Viral Integration Sites in HPV-Related Cancers. Int. J. Cancer 139 (9), 2001–2011. doi:10.1002/ijc.30243
Busso-Lopes, A. F., Marchi, F. A., Kuasne, H., Scapulatempo-Neto, C., Trindade-Filho, J. C. S., de Jesus, C. M. N., et al. (2015). Genomic Profiling of Human Penile Carcinoma Predicts Worse Prognosis and Survival. Cancer Prev. Res. 8 (2), 149–156. doi:10.1158/1940-6207.CAPR-14-0284
Causin, R. L., da Silva, L. S., Evangelista, A. F., Leal, L. F., Souza, K. C. B., Pessôa-Pereira, D., et al. (2021). MicroRNA Biomarkers of High-Grade Cervical Intraepithelial Neoplasia in Liquid Biopsy. BioMed Res. Int. 2021, 1–9. doi:10.1155/2021/6650966
Chahoud, J., Gleber-Netto, F. O., McCormick, B. Z., Rao, P., Lu, X., Guo, M., et al. (2021). Whole-exome Sequencing in Penile Squamous Cell Carcinoma Uncovers Novel Prognostic Categorization and Drug Targets Similar to Head and Neck Squamous Cell Carcinoma. Clin. Cancer Res. 27 (9), 2560–2570. doi:10.1158/1078-0432.CCR-20-4004
Christodoulidou, M., Sahdev, V., Houssein, S., and Muneer, A. (2015). Epidemiology of Penile Cancer. Curr. Problems Cancer 39 (3), 126–136. doi:10.1016/j.currproblcancer.2015.03.010
Coelho, R. W. P., Pinho, J. D., Moreno, J. S., Garbis, D. V. e. O., do Nascimento, A. M. T., Larges, J. S., et al. (2018). Penile Cancer in Maranhão, Northeast Brazil: the Highest Incidence Globally? BMC Urol. 18 (1), 50. doi:10.1186/s12894-018-0365-0
Datta, A., Das, P., Dey, S., Ghuwalewala, S., Ghatak, D., Alam, S. K., et al. (2019). Genome-Wide Small RNA Sequencing Identifies MicroRNAs Deregulated in Non-small Cell Lung Carcinoma Harboring Gain-Of-Function Mutant P53. Genes 10 (11), 852. doi:10.3390/genes10110852
Datta, A., Ghatak, D., Das, S., Banerjee, T., Paul, A., Butti, R., et al. (2017). p53 Gain‐of‐function Mutations Increase Cdc7‐dependent Replication Initiation. EMBO Rep. 18 (11), 2030–2050. doi:10.15252/embr.201643347
de Martel, C., Plummer, M., Vignat, J., and Franceschi, S. (2017). Worldwide Burden of Cancer Attributable to HPV by Site, Country and HPV Type. Int. J. Cancer 141 (4), 664–670. doi:10.1002/ijc.30716
Deng, G., and Sui, G. (2013). Noncoding RNA in Oncogenesis: A New Era of Identifying Key Players. Ijms 14 (9), 18319–18349. doi:10.3390/ijms140918319
Ding, Y., Chan, C. Y., and Lawrence, C. E. (2004). Sfold Web Server for Statistical Folding and Rational Design of Nucleic Acids. Nucleic Acids Res. 32, W135–W141. doi:10.1093/nar/gkh449
Dürst, M., Croce, C. M., Gissmann, L., Schwarz, E., and Huebner, K. (1987). Papillomavirus Sequences Integrate Near Cellular Oncogenes in Some Cervical Carcinomas. Proc. Natl. Acad. Sci. U.S.A. 84 (4), 1070–1074. doi:10.1073/pnas.84.4.1070
Feber, A., Arya, M., de Winter, P., Saqib, M., Nigam, R., Malone, P. R., et al. (2015). Epigenetics Markers of Metastasis and HPV-Induced Tumorigenesis in Penile Cancer. Clin. Cancer Res. 21 (5), 1196–1206. doi:10.1158/1078-0432.CCR-14-1656
Feber, A., Worth, D. C., Chakravarthy, A., de Winter, P., Shah, K., Arya, M., et al. (2016). CSN1 Somatic Mutations in Penile Squamous Cell Carcinoma. Cancer Res. 76 (16), 4720–4727. doi:10.1158/0008-5472.CAN-15-3134
Fischer, M. (2017). Census and Evaluation of P53 Target Genes. Oncogene 36 (28), 3943–3956. doi:10.1038/onc.2016.502
Furuya, T. K., Murta, C. B., Murillo Carrasco, A. G., Uno, M., Sichero, L., Villa, L. L., et al. (2021). Disruption of miRNA-mRNA Networks Defines Novel Molecular Signatures for Penile Carcinogenesis. Cancers 13 (19), 4745. doi:10.3390/cancers13194745
Gao, G., Johnson, S. H., Vasmatzis, G., Pauley, C. E., Tombers, N. M., Kasperbauer, J. L., et al. (2017). Common Fragile Sites (CFS) and Extremely Large CFS Genes Are Targets for Human Papillomavirus Integrations and Chromosome Rearrangements in Oropharyngeal Squamous Cell Carcinoma. Genes Chromosom. Cancer 56 (1), 59–74. doi:10.1002/gcc.22415
Groves, I. J., and Coleman, N. (2018). Human Papillomavirus Genome Integration in Squamous Carcinogenesis: what Have Next-Generation Sequencing Studies Taught Us? J. Pathol. 245 (1), 9–18. doi:10.1002/path.5058
Hafner, M., Landthaler, M., Burger, L., Khorshid, M., Hausser, J., Berninger, P., et al. (2010). Transcriptome-wide Identification of RNA-Binding Protein and MicroRNA Target Sites by PAR-CLIP. Cell. 141 (1), 129–141. doi:10.1016/j.cell.2010.03.009
Hansen, B. T., Orumaa, M., Lie, A. K., Brennhovd, B., and Nygård, M. (2018). Trends in Incidence, Mortality and Survival of Penile Squamous Cell Carcinoma in Norway 1956-2015. Int. J. Cancer 142 (8), 1586–1593. doi:10.1002/ijc.31194
Hartz, J. M., Engelmann, D., Fürst, K., Marquardt, S., Spitschak, A., Goody, D., et al. (2016). Integrated Loss of miR-1/miR-101/miR-204 Discriminates Metastatic from Nonmetastatic Penile Carcinomas and Can Predict Patient Outcome. J. Urology 196 (2), 570–578. doi:10.1016/j.juro.2016.01.115
Hennig, C. (2020). Fpc: Flexible Procedures for Clustering. Fpc: Flexible Procedures for Clustering. AvaliableAt: https://CRAN.R-project.org/package=fpc (Accessed August 5, 2021).
Hermeking, H. (2012). MicroRNAs in the P53 Network: Micromanagement of Tumour Suppression. Nat. Rev. Cancer 12 (9), 613–626. doi:10.1038/nrc3318
Hermeking, H., Rokavec, M., Li, H., and Jiang, L. (2014). The p53/microRNA Connection in Gastrointestinal Cancer. Ceg 395, 395. doi:10.2147/CEG.S43738
Holmes, A., Lameiras, S., Jeannot, E., Marie, Y., Castera, L., Sastre-Garau, X., et al. (2016). Mechanistic Signatures of HPV Insertions in Cervical Carcinomas. npj Genomic Med. 1 (1), 16004. doi:10.1038/npjgenmed.2016.4
Hu, W., Zheng, X., Liu, J., Zhang, M., Liang, Y., and Song, M. (2021). MicroRNA MiR-130a-3p Promotes Gastric Cancer by Targeting Glucosaminyl N-Acetyl Transferase 4 (GCNT4) to Regulate the TGF-Β1/smad3 Pathway. Bioengineered 12 (2), 11634–11647. doi:10.1080/21655979.2021.1995099
Hu, Z., Zhu, D., Wang, W., Li, W., Jia, W., Zeng, X., et al. (2015). Genome-wide Profiling of HPV Integration in Cervical Cancer Identifies Clustered Genomic Hot Spots and a Potential Microhomology-Mediated Integration Mechanism. Nat. Genet. 47 (2), 158–163. doi:10.1038/ng.3178
Huang, W.-C., Yadav, V. K., Cheng, W.-H., Wang, C.-H., Hsieh, M.-S., Huang, T.-Y., et al. (2021). The MEK/ERK/miR-21 Signaling Is Critical in Osimertinib Resistance in EGFR-Mutant Non-small Cell Lung Cancer Cells. Cancers 13 (23), 6005. doi:10.3390/cancers13236005
Hussen, B. M., Ahmadi, G., Marzban, H., Fard Azar, M. E., Sorayyayi, S., Karampour, R., et al. (2021). The Role of HPV Gene Expression and Selected Cellular MiRNAs in Lung Cancer Development. Microb. Pathog. 150, 104692. doi:10.1016/j.micpath.2020.104692
Kanoria, S., Rennie, W., Liu, C., Carmack, C. S., Lu, J., and Ding, Y. (2016). STarMir Tools for Prediction of microRNA Binding Sites. Methods Mol. Biol. 1490, 73–82. doi:10.1007/978-1-4939-6433-8_6
Kidd, L. C., Chaing, S., Chipollini, J., Giuliano, A. R., Spiess, P. E., and Sharma, P. (2017). Relationship between Human Papillomavirus and Penile Cancer-Implications for Prevention and Treatment. Transl. Androl. Urol. 6 (5), 791–802. doi:10.21037/tau.2017.06.27
Kong, X., Zhang, J., Li, J., Shao, J., and Fang, L. (2018). MiR-130a-3p Inhibits Migration and Invasion by Regulating RAB5B in Human Breast Cancer Stem Cell-like Cells. Biochem. Biophysical Res. Commun. 501 (2), 486–493. doi:10.1016/j.bbrc.2018.05.018
Kuasne, H., Barros-Filho, M. C., Busso-Lopes, A., Marchi, F. A., Pinheiro, M., Muñoz, J. J. M., et al. (2017). Integrative miRNA and mRNA Analysis in Penile Carcinomas Reveals Markers and Pathways with Potential Clinical Impact. Oncotarget 8 (9), 15294–15306. doi:10.18632/oncotarget.14783
Kuasne, H., Cólus, I. M. d. S., Busso, A. F., Hernandez-Vargas, H., Barros-Filho, M. C., Marchi, F. A., et al. (2015). Genome-wide Methylation and Transcriptome Analysis in Penile Carcinoma: Uncovering New Molecular Markers. Clin. Epigenet 7 (1), 46. doi:10.1186/s13148-015-0082-4
Kumar Gupta, A., and Kumar, M. (2015). HPVbase - a Knowledgebase of Viral Integrations, Methylation Patterns and microRNAs Aberrant Expression: As Potential Biomarkers for Human Papillomaviruses Mediated Carcinomas. Sci. Rep. 5 (1), 12522. doi:10.1038/srep12522
Kumar, M., Lu, Z., Takwi, A. A. L., Chen, W., Callander, N. S., Ramos, K. S., et al. (2011). Negative Regulation of the Tumor Suppressor P53 Gene by microRNAs. Oncogene 30 (7), 843–853. doi:10.1038/onc.2010.457
Lal, A., Navarro, F., Maher, C. A., Maliszewski, L. E., Yan, N., O'Day, E., et al. (2009). miR-24 Inhibits Cell Proliferation by Targeting E2F2, MYC, and Other Cell-Cycle Genes via Binding to "Seedless" 3′UTR MicroRNA Recognition Elements. Mol. Cell. 35 (5), 610–625. doi:10.1016/j.molcel.2009.08.020
Lee, Y. S., and Dutta, A. (2009). MicroRNAs in Cancer. Annu. Rev. Pathol. Mech. Dis. 4 (1), 199–227. doi:10.1146/annurev.pathol.4.110807.092222
Liu, J., Yoo, J., Ho, J. Y., Jung, Y., Lee, S., Hur, S. Y., et al. (2021). Plasma-derived Exosomal miR-4732-5p Is a Promising Noninvasive Diagnostic Biomarker for Epithelial Ovarian Cancer. J. Ovarian Res. 14 (1), 59. doi:10.1186/s13048-021-00814-z
Liu, J., Zhang, C., Zhao, Y., and Feng, Z. (2017). MicroRNA Control of P53. J. Cell. Biochem. 118 (1), 7–14. doi:10.1002/jcb.25609
Liu, X., Dong, Y., and Song, D. (2020a). Inhibition of microRNA-15b-5p Attenuates the Progression of Oral Squamous Cell Carcinoma via Modulating the PTPN4/STAT3 Axis. Cmar Vol. 12, 10559–10572. doi:10.2147/CMAR.S272498
Liu, Y., Lu, Z., Xu, R., and Ke, Y. (2016). Comprehensive Mapping of the Human Papillomavirus (HPV) DNA Integration Sites in Cervical Carcinomas by HPV Capture Technology. Oncotarget 7 (5), 5852–5864. doi:10.18632/oncotarget.6809
Liu, Z.-J., Liu, S.-H., Li, J.-R., Bie, X.-C., and Zhou, Y. (2020b). MiR-15b-5b Regulates the Proliferation of Prostate Cancer PC-3 Cells via Targeting LATS2. Cmar Vol. 12, 10669–10678. doi:10.2147/CMAR.S266421
Long, D., Lee, R., Williams, P., Chan, C. Y., Ambros, V., and Ding, Y. (2007). Potent Effect of Target Structure on microRNA Function. Nat. Struct. Mol. Biol. 14 (4), 287–294. doi:10.1038/nsmb1226
Macedo, J., Silva, E., Nogueira, L., Coelho, R., Silva, J., Santos, A., et al. (2020). Genomic Profiling Reveals the Pivotal Role of hrHPV Driving Copy Number and Gene Expression Alterations, Including mRNA Downregulation of TP53 and RB1 in Penile Cancer. Mol. Carcinog. 59 (6), 604–617. doi:10.1002/mc.23185
Mantovani, F., Collavin, L., and Del Sal, G. (2019). Mutant P53 as a Guardian of the Cancer Cell. Cell. Death Differ. 26 (2), 199–212. doi:10.1038/s41418-018-0246-9
Marchi, F. A., Martins, D. C., Barros-Filho, M. C., Kuasne, H., Busso Lopes, A. F., Brentani, H., et al. (2017). Multidimensional Integrative Analysis Uncovers Driver Candidates and Biomarkers in Penile Carcinoma. Sci. Rep. 7 (1), 6707. doi:10.1038/s41598-017-06659-1
Nunez Lopez, Y. O., Retnakaran, R., Zinman, B., Pratley, R. E., and Seyhan, A. A. (2019). Predicting and Understanding the Response to Short-Term Intensive Insulin Therapy in People with Early Type 2 Diabetes. Mol. Metab. 20, 63–78. doi:10.1016/j.molmet.2018.11.003
Parfenov, M., Pedamallu, C. S., Gehlenborg, N., Freeman, S. S., Danilova, L., Bristow, C. A., et al. (2014). Characterization of HPV and Host Genome Interactions in Primary Head and Neck Cancers. Proc. Natl. Acad. Sci. U.S.A. 111 (43), 15544–15549. doi:10.1073/pnas.1416074111
Park, J. H., Shin, S.-Y., and Shin, C. (2017). Non-canonical Targets Destabilize microRNAs in Human Argonautes. Nucleic Acids Res. 45, 1569–1583. doi:10.1093/nar/gkx029
Pessôa-Pereira, D., Evangelista, A. F., Causin, R. L., da Costa Vieira, R. A., Abrahão-Machado, L. F., Santana, I. V. V., et al. (2020). MiRNA Expression Profiling of Hereditary Breast Tumors from BRCA1- and BRCA2-Germline Mutation Carriers in Brazil. BMC Cancer 20 (1), 143. doi:10.1186/s12885-020-6640-y
Peta, E., Cappellesso, R., Masi, G., Sinigaglia, A., Trevisan, M., Grassi, A., et al. (2017). Down-regulation of microRNA-146a Is Associated with High-Risk Human Papillomavirus Infection and Epidermal Growth Factor Receptor Overexpression in Penile Squamous Cell Carcinoma. Hum. Pathol. 61, 33–40. doi:10.1016/j.humpath.2016.10.019
Petkevich, A. A., Abramov, A. A., Pospelov, V. I., Malinina, N. A., Kuhareva, E. I., Mazurchik, N. V., et al. (2021). Exosomal and Non-exosomal miRNA Expression Levels in Patients with HCV-Related Cirrhosis and Liver Cancer. Oncotarget 12 (17), 1697–1706. doi:10.18632/oncotarget.28036
Pillai, M. M., Gillen, A. E., Yamamoto, T. M., Kline, E., Brown, J., Flory, K., et al. (2014). HITS-CLIP Reveals Key Regulators of Nuclear Receptor Signaling in Breast Cancer. Breast Cancer Res. Treat. 146 (1), 85–97. doi:10.1007/s10549-014-3004-9
Pinatti, L. M., Gu, W., Wang, Y., Elhossiny, A., Bhangale, A. D., Brummel, C. V., et al. (2021). SearcHPV: A Novel Approach to Identify and Assemble Human Papillomavirus-Host Genomic Integration Events in Cancer. Cancer 127 (19), 3531–3540. doi:10.1002/cncr.33691
Rehmsmeier, M., Steffen, P., Höchsmann, M., and Giegerich, R. (2004). Fast and Effective Prediction of microRNA/target Duplexes. RNA 10 (10), 1507–1517. doi:10.1261/rna.5248604
Rosa, M. N., Evangelista, A. F., Leal, L. F., De Oliveira, C. M., Silva, V. A. O., Munari, C. C., et al. (2019). Establishment, Molecular and Biological Characterization of HCB-514: A Novel Human Cervical Cancer Cell Line. Sci. Rep. 9 (1), 1913. doi:10.1038/s41598-018-38315-7
Sammons, M. A., Nguyen, T.-A. T., McDade, S. S., and Fischer, M. (2020). Tumor Suppressor P53: From Engaging DNA to Target Gene Regulation. Nucleic Acids Res. 48 (16), 8848–8869. doi:10.1093/nar/gkaa666
Santos, J., Peixoto da Silva, S., Costa, N., Gil da Costa, R., and Medeiros, R. (2018). The Role of MicroRNAs in the Metastatic Process of High-Risk HPV-Induced Cancers. Cancers 10 (12), 493. doi:10.3390/cancers10120493
Shin, C., Nam, J.-W., Farh, K. K.-H., Chiang, H. R., Shkumatava, A., and Bartel, D. P. (2010). Expanding the MicroRNA Targeting Code: Functional Sites with Centered Pairing. Mol. Cell. 38 (6), 789–802. doi:10.1016/j.molcel.2010.06.005
Silva, J. d., Nogueira, L., Coelho, R., Deus, A., Khayat, A., Marchi, R., et al. (2021). HPV-associated Penile Cancer: Impact of Copy Number Alterations in miRNA/mRNA Interactions and Potential Druggable Targets. Cbm 32, 147–160. doi:10.3233/CBM-210035
Song, G.-L., Xiao, M., Wan, X.-Y., Deng, J., Ling, J.-D., Tian, Y.-G., et al. (2021). MiR-130a-3p Suppresses Colorectal Cancer Growth by Targeting Wnt Family Member 1 (WNT1). Bioengineered 12 (1), 8407–8418. doi:10.1080/21655979.2021.1977556
Squarzanti, D. F., Sorrentino, R., Landini, M. M., Chiesa, A., Pinato, S., Rocchio, F., et al. (2018). Human Papillomavirus Type 16 E6 and E7 Oncoproteins Interact with the Nuclear P53-Binding Protein 1 in an In Vitro Reconstructed 3D Epithelium: New Insights for the Virus-Induced DNA Damage Response. Virol. J. 15 (1), 176. doi:10.1186/s12985-018-1086-4
Tang, D., Li, B., Xu, T., Hu, R., Tan, D., Song, X., et al. (2020). VISDB: A Manually Curated Database of Viral Integration Sites in the Human Genome. Nucleic Acids Res. 48 (D1), D633–D641. doi:10.1093/nar/gkz867
Tuna, M., and Amos, C. I. (2017). Next Generation Sequencing and its Applications in HPVassociated Cancers. Oncotarget 8 (5), 8877–8889. doi:10.18632/oncotarget.12830
Vlachos, I. S., Zagganas, K., Paraskevopoulou, M. D., Georgakilas, G., Karagkouni, D., Vergoulis, T., et al. (2015). DIANA-miRPath v3.0: Deciphering microRNA Function with Experimental Support. Nucleic Acids Res. 43 (W1), W460–W466. doi:10.1093/nar/gkv403
Wang, J., Li, T., and Wang, B. (2021). Exosomal Transfer of miR-25-3p P-romotes the P-roliferation and T-emozolomide R-esistance of G-lioblastoma C-ells by T-argeting FBXW7. Int. J. Oncol. 59 (2), 64. doi:10.3892/ijo.2021.5244
Wang, X., and Sun, Q. (2017). TP53 Mutations, Expression and Interaction Networks in Human Cancers. Oncotarget 8 (1), 624–643. doi:10.18632/oncotarget.13483
Wang, Y., Wang, K., Chen, Y., Zhou, J., Liang, Y., Yang, X., et al. (2019). Mutational Landscape of Penile Squamous Cell Carcinoma in a Chinese Population. Int. J. Cancer 145 (5), 1280–1289. doi:10.1002/ijc.32373
Wentzensen, N., Vinokurova, S., and Doeberitz, M. v. K. (2004). Systematic Review of Genomic Integration Sites of Human Papillomavirus Genomes in Epithelial Dysplasia and Invasive Cancer of the Female Lower Genital Tract. Cancer Res. 64 (11), 3878–3884. doi:10.1158/0008-5472.CAN-04-0009
Wu, T., Chen, W., Kong, D., Li, X., Lu, H., Liu, S., et al. (2015). MiR-25 Targets the Modulator of Apoptosis 1 Gene in Lung Cancer. Carcin 36 (8), 925–935. doi:10.1093/carcin/bgv068
Zhan, J., Tong, J., and Fu, Q. (2020). Long Non-coding RNA LINC00858 P-romotes TP53-wild-type C-olorectal C-ancer P-rogression by R-egulating the microRNA-25-3p/SMAD7 axis. Oncol. Rep. 43, 1267–1277. doi:10.3892/or.2020.7506
Zhang, L., Wei, P., Shen, X., Zhang, Y., Xu, B., Zhou, J., et al. (2015a). MicroRNA Expression Profile in Penile Cancer Revealed by Next-Generation Small RNA Sequencing. Plos One 10 (7), e0131336. doi:10.1371/journal.pone.0131336
Zhang, Y., Huang, F., Wang, J., Peng, L., and Luo, H. (2015b). MiR-15b Mediates Liver Cancer Cells Proliferation through Targeting BCL-2. Int. J. Clin. Exp. Pathol. 7, 15677–15683.
Zhou, X., Zhang, Z., and Liang, X. (2019). Regulatory Network Analysis to Reveal Important miRNAs and Genes in Non-small Cell Lung Cancer. Cell. J. 21 (4), 459–466. doi:10.22074/cellj.2020.6281
Keywords: penile cancer, tumor suppressor repression, miRNA, HPV, TP53, Rb1
Citation: da Silva J, da Costa CC, de Farias Ramos I, Laus AC, Sussuchi L, Reis RM, Khayat AS, Cavalli LR and Pereira SR (2022) Upregulated miRNAs on the TP53 and RB1 Binding Seedless Regions in High-Risk HPV-Associated Penile Cancer. Front. Genet. 13:875939. doi: 10.3389/fgene.2022.875939
Received: 14 February 2022; Accepted: 26 May 2022;
Published: 24 June 2022.
Edited by:
Ticiana D. J. Farias, University of Colorado, United StatesReviewed by:
Hin Fung Tsang, Hong Kong Adventist Hospital, Hong Kong SAR, ChinaCopyright © 2022 da Silva, da Costa, de Farias Ramos, Laus, Sussuchi, Reis, Khayat, Cavalli and Pereira. This is an open-access article distributed under the terms of the Creative Commons Attribution License (CC BY). The use, distribution or reproduction in other forums is permitted, provided the original author(s) and the copyright owner(s) are credited and that the original publication in this journal is cited, in accordance with accepted academic practice. No use, distribution or reproduction is permitted which does not comply with these terms.
*Correspondence: Silma Regina Pereira, c2lsbWEucGVyZWlyYUB1Zm1hLmJy
Disclaimer: All claims expressed in this article are solely those of the authors and do not necessarily represent those of their affiliated organizations, or those of the publisher, the editors and the reviewers. Any product that may be evaluated in this article or claim that may be made by its manufacturer is not guaranteed or endorsed by the publisher.
Research integrity at Frontiers
Learn more about the work of our research integrity team to safeguard the quality of each article we publish.