- 1Center for Molecular Medicine and Biobanking, University of Malta, Msida, Malta
- 2Department of Pathology, Faculty of Medicine and Surgery, University of Malta, Msida, Malta
- 3Department of Dermatology, Mater Dei Hospital, Msida, Malta
- 4Department of Pathology, Mater Dei Hospital, Msida, Malta
Hidradenitis suppurativa is a chronic, suppurative condition of the pilosebaceous unit manifesting as painful nodules, abscesses, and sinus tracts mostly in, but not limited to, intertriginous skin. Great strides have been made at elucidating the pathophysiology of hidradenitis suppurativa, which appears to be the product of hyperkeratinization and inflammation brought about by environmental factors and a genetic predisposition. The identification of familial hidradenitis suppurativa has sparked research aimed at identifying underlying pathogenic variants in patients who harbor them. The objective of this review is to provide a broad overview of the role of genetics in various aspects of hidradenitis suppurativa, specifically the pathophysiology, diagnosis, and clinical application.
Introduction
Hidradenitis suppurativa (HS) is a chronic, suppurative disorder characterized by inflammation and hyperkeratinization at the pilosebaceous unit (PSU). The condition manifests as tender nodules, draining abscesses and sinuses mostly in (but not limited to) intertriginous skin (von Laffert et al., 2011; Danby et al., 2013). The latest estimate of overall HS prevalence is 0.4% (95%CI, 0.26–0.63%) (Jfri et al., 2021). HS can arise as an isolated condition, in a syndromic form or in the setting of other cutaneous conditions (for example Dowling-Degos Disease). The pathophysiology of HS is complex and is strongly determined by environmental and lifestyle factors such as smoking and obesity. These factors interact with specific physical triggers, namely friction, sweat, increased temperature, and changes in the cutaneous microbiome to drive disease risk (Mintoff et al., 2021a).
HS has an underlying genetic etiology. The link between HS and a genetic predisposition has long been suspected. In the 1980s Fitzsimmons and Guilbert published evidence in favor of autosomal dominant or familial HS (fHS) (Fitzsimmons et al., 1984; Fitzsimmons et al., 1985; Fitzsimmons and Guilbert, 1985); however the causative gene remained elusive. Initial studies performed by Gao et al. suggested a possible HS locus at 1p21.1-1q25.3 (Gao et al., 2006). Based on these findings, linkage analysis was performed on HS-patients with a strong family history however, the results obtained failed to identify the causative locus (Saleh Al-Ali et al., 2010). Following the advent of whole exome sequencing (WES), Gao et al later described the c.210_211delAG NCSTN variant which segregated with disease in multiple family members affected by HS (Liu et al., 2011). Since then, several other investigators have provided evidence supporting a monogenic etiology in fHS, mostly involving loci encoding proteins of the γ-secretase complex (GSC) (OMIM #142690, #613736, #613737). These initial discoveries have fueled a strong drive to explore the role of genetic susceptibility in HS with the aim of better characterizing the pathophysiology of the disease.
The mechanisms and extent to which the combination of genomic and non-genomic factors determine disease manifestation, its phenotype and response to therapy are not fully understood. The increasing availability and affordability of genomic sequencing in both clinical and research settings, as well as more robust methods of interrogating the human genome has resulted in a surge of genetic data on HS. This genetic data is supported by in vitro and in vivo functional studies in a minority of cases. Nevertheless, a comprehensive understanding of the role of rare vs. common genetic factors that drive HS is lacking.
This systematic review aims to compile, analyze and present the extant literature pertaining to the genetic architecture of HS. Specifically, we aim to 1) comprehensively describe genetic variants that have been associated with HS phenotypes 2) evaluate the impact of selected missense variants on protein structure and stability using in-silico tools 3) explore genotype-phenotype associations and 4) discuss HS genetics in the context of disease subtypes.
Methods
Literature Search
A systematic literature search pertaining to hidradenitis suppurativa and genetic variation was conducted in PubMed/MEDLINE, Science Direct and Google Scholar databases. A comprehensive search strategy was based on the following combinations of free text keywords and Medical Subject Heading (MeSH) terms: “hidradenitis”, “suppurativa”, “acne”, “inversa”, “genetic”, “familial”, “secretase”, “nicastrin”, “NCSTN”, “PSENEN”, “presenilin”, “PSEN”, “APH”, “NOD2”, “PSTPIP1”, “MEFV”, “Syndrome”, “PASH”, “PAPASH”, “Pyoderma”, “SAPHO”, “PASS”, “Dowling-Degos”, “Mediterranean”. The Boolean operators used were “AND” and “OR”. The search covered articles published between January 1980 and January 2022, and was restricted to articles published in the English, Italian and Spanish languages. Furthermore, handsearching and citation review of relevant studies was also conducted to identify studies that were not captured by the electronic database search. Published original studies investigating the genetics of HS in its isolated or syndromic forms through both targeted and untargeted genomic approaches were eligible for inclusion. We excluded studies with 1) absent genetic data 2) duplicate data pertaining to the same proband in separate publications 3) conference proceedings, reviews, editorial letters or comments and 4) studies not directly investigating the association of specific genes with HS phenotypes.
Data Extraction
Articles identified from the literature search were screened for duplicates. All studies deemed to be potentially eligible for inclusion were reviewed and data extracted by the investigators. Any discrepancies were resolved by consensus.
The following information was extracted from each eligible article 1) primary author and year of publication 2) locus, the specific genetic variant identified and segregation data when available 3) age at presentation of first lesion 4) primary anatomic sites affected and 5) data on comorbid risk factors, specifically obesity and smoking status.
Data Analysis
Identified variants were categorized as missense, nonsense, frameshift indels, splice-site and non-coding regions according to their effect on translation. VarSeq software (Golden Helix) was used for variant interpretation and annotation (Kopanos et al., 2019). Variant pathogenicity was classified according to guidelines from the American College of Medical Genetics/Association for Molecular Pathology (ACMG/AMP) (Richards et al., 2015). These guidelines standardize variant classification by stratification into five categories (pathogenic, likely pathogenic, uncertain significance, likely benign, benign) based on a combination of computational, population, functional and segregation data. Rare variants were considered to be of uncertain significance (VUS) if there is limited or contradictory clinical or functional evidence for pathogenicity. The Human Gene Mutation Database, (HGMD), dbSNP and ClinVar databases (ncbi.nlm.nih.gov/clinvar/) were also interrogated to identify any respective studies and ontologies. To explore genotype-phenotype associations, variants in the GSC genes were considered as an aggregate category and contrasted against variants in genes not forming part of this complex.
Protein Structure Analysis and Molecular Modelling
The impact of missense variants on protein structure was evaluated through molecular modelling. The DynaMut webserver was used to predict the effects of amino acid substitutions on protein stability, flexibility and to analyze interactions amongst amino acid residues (Rodrigues et al., 2018). The predicted change in stability between the wild type and variant structures derived from SDM, DUET, and mCSM algorithms is reported as ΔΔG in kcal/mol, with negative values indicative of destabilizing variants. Dynamut also reports vibrational entropy changes (ΔΔSVib in kcal mol−1K−1) calculated by ENCoM using normal-mode analysis to depict the substitution’s effect on structure flexibility or rigidity. To further categorize the structural impact of missense variants, the Missense3D and HOPE webservers were used. Missense3D assesses 17 different structural features that are essential for protein conformation and stability, such as stearic clashes and disallowed phi/psi angles (Venselaar et al., 2010; Ittisoponpisan et al., 2019). A comprehensive graphical summary of identified GSC variants was generated using ProteinPaint (Zhou C et al., 2016).
Statistics
The characteristics of the cohort are summarized using descriptive statistics. Normality of age at first lesion was assessed by the Shapiro–Wilk and Kolmogorov-Smirnoff tests. This exhibited a skewed non-normal distribution; non-parametric statistics with medians and interquartile ranges are presented. To compare differences in quantitative variables between two categories, the independent-samples Mann–Whitney U test was applied. The chi-square test was used to compare categorical variables. A p-value of <0.05 was considered statistically significant.
Results
In total, the literature search identified 88 published variants implicated in HS. Approximately 83% of published variants involve the four genes encoding protein subunits of the multimeric GSC (NCSTN 54.5%, PSENEN 24%, PSEN 3.4% and APH 1%) (Figure 1). The remaining variants localized to PSTPIP1, MEFV, NLRP3, IL1RN, NOD2 and POFUT loci, which have been variably associated with non-syndromic forms of HS. A summary of the identified variants, their pathogenicity classifiers and phenotypes are provided in Table 1. The reported median age at first lesion was 17 years (min 10 years—max 67 years). The axillae and groin were the most frequently reported affected sites. Clear segregation with the HS phenotype was documented by 78% of reports. The identified variants were classified as pathogenic or likely pathogenic in 66% of cases, and as VUS or VUS leaning pathogenic in 18% of cases according to the ACMG-AMP consensus criteria. At the protein level, variants were classified as missense (29.5%), nonsense (24%), frameshift indels (28.4%), splice donor/acceptor site (15.9%) and in non-coding regions (2.3%). Lifestyle factors that associate with HS predisposition and outcome, such as obesity and smoking status were inconsistently reported in the literature.
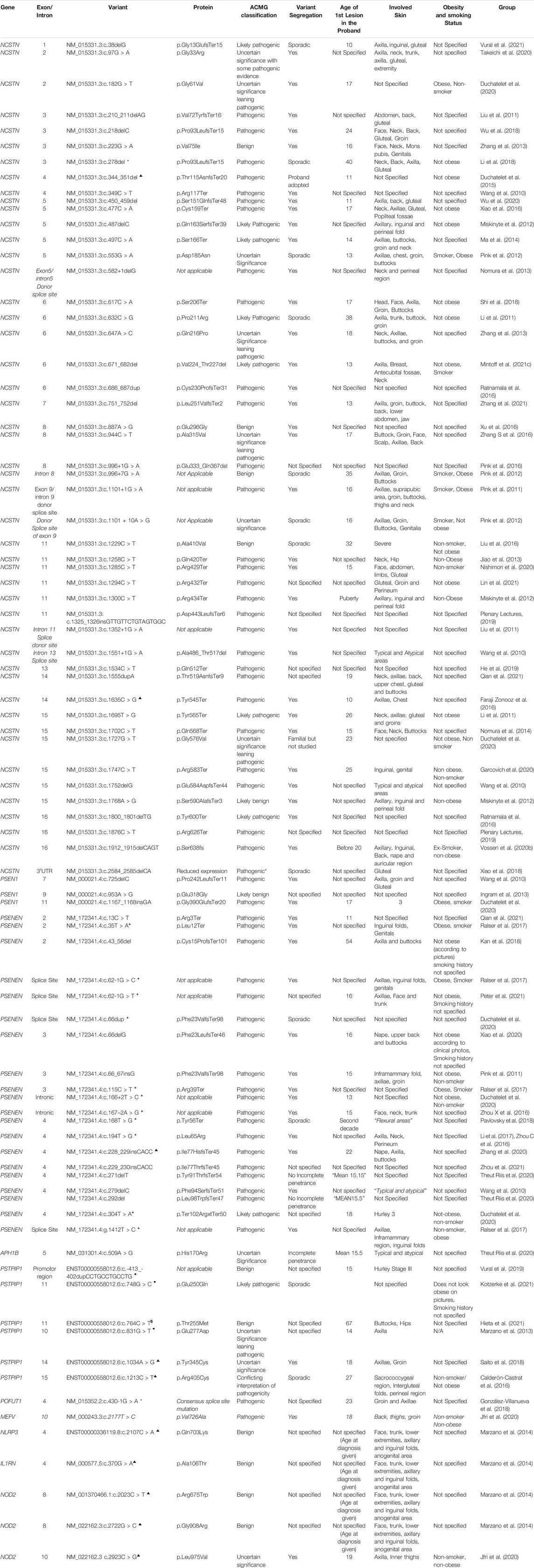
TABLE 1. A summary of described genetic variants, their protein effect, ACMG classification and phenotypic associations. *SAPHO ▲ PASH • PAPASH $PPHSF +HS-DDD.
No significant difference in the reported age at first lesion was identified between carriers of variants in GSC and other loci (17 vs. 19 years, p = 0.112). Similarly, no difference in age at first lesion between NCSTN variant carriers and non-NCSTN variant carriers was identified (17 vs. 18 years, p = 0.345). Carriers of pathogenic variants had a marginally lower age at first lesion than carriers of VUS or benign variants (16 vs. 18 years p = 0.048). A significantly higher proportion of pathogenic variants was located in GSC genes compared to other loci (74 vs. 26.7%, χ2 = 12.1, p = 0.001). As expected, variants that disrupt translation through nonsense, frameshift or splice-altering effects are more likely to be pathogenic than missense variants (91.4% vs. 8.6%, χ2 = 42.2, p < 0.01). No significant difference in obesity or smoking status was noted when probands carrying pathogenic variants were compared to those carrying non-pathogenic variants, although these parameters were inconstantly reported in the literature. No association between the primary anatomic sites involved by HS lesions and gene (NCSTN vs. non-NCSTN) or the ACMG pathogenicity classifier (pathogenic vs. non-pathogenic variants) was noted. Table 2 contrasts the salient clinical and genetic features between GSC variant carriers and non-carriers.
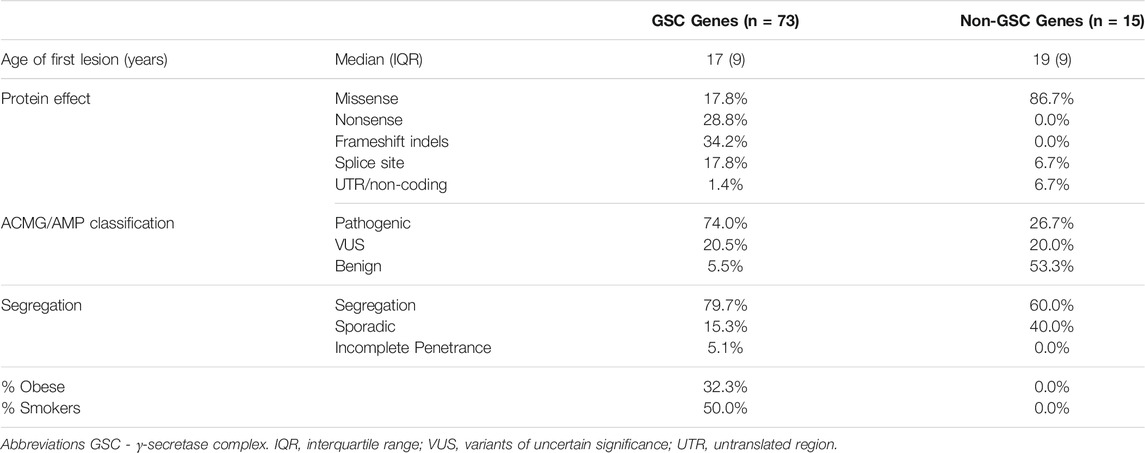
TABLE 2. A comparison of the salient clinical and genetic findings between HS cases bearing variants in γ-secretase complex genes and HS cases with variants at other loci. All non-GSC genes (PSTPIP, MEFV, NOD2, IL1RN and NLRP3) that have been associated with HS subtypes are considered as an aggregate category.
For selected missense variants we explored the structural impacts of the substitution and assessed the change in ΔΔG and Δ vibrational entropy energy predictions between the wild-type and mutant structures. An overview of the findings is presented in Supplementary Table S1. Only five of 23 (21.73%) missense variants were predicted to exert conformationally deleterious effects as they impact critical structural residues (NCSTN p. Gly61Val, p. Gln216Pro, p. Glu296Gly, p. Gly576Val and APH p. His170Arg). Four of these variants have VUS/VUS leaning pathogenic classifications while the fifth (NCSTN p. Glu296Gly) is classified as benign based on allele frequency cut-offs. However, most missense variants reported in the literature demonstrated destabilizing effects with changes in conformational flexibility. These findings suggest that missense variants in HS-associated loci may contribute to disease by modulating protein activity in a variety of ways.
Discussion
This review provides a comprehensive summary of individual studies that investigate the genomic etiology of HS in its various forms; published variants to date are collated and appraised using the conservative ACMG-AMP criteria, genotype-phenotype associations assessed, and the structural impact of missense variants evaluated computationally. Our findings expound the locus and allelic heterogeneity underlying this inflammatory disorder and demonstrate a lack of robust correlation with clinical phenotypes. Herein we outline the physiological relevance of the HS-associated loci.
Despite the high heritability estimates of HS (77–80%) only a minority of HS patients demonstrate a strong monogenic etiology in the context of familial or syndromic HS (5%) (Kjaersgaard Andersen et al., 2021). Nevertheless, common forms of the disease demonstrate familial segregation. A family history of HS was documented in 41% in a cohort of 271 pediatric HS patients (Liy-Wong et al., 2021). The high heritability estimates reported by van Straalen et al suggest that sporadic forms of HS have a strong genetic element that contributes to their causality, despite the absence of fully penetrant variants causing multigenerational disease (Straalen et al., 2020). To date, the exact nature of the genetic variants driving common forms of HS remains unelucidated.
Based on Existing Knowledge on the Genomics of Hidradenitis Suppurativa, the Disease can Be Categorized as
1. Sporadic HS: HS with no identified genetic variation to date
2. Familial HS: HS with a strong family history and established underlying monogenic etiology
3. Syndromic HS: HS in the setting of constellation of other clinical manifestation (PASH, PAPASH, SAPHO)
4. HS+: HS in the setting of Dowling-Degos Disease (HS-DDD) or Familial Mediterranean Fever (HS-FMF).
Familial Hidradenitis Suppurativa: Variation in γ-Secretase Complex Protein-Coding Genes; Knocked down a Notch
Genetic variants in the GSC account for the majority of identified variants in fHS and sHS cases, but for only a minority of cases of sporadic HS. Cohort studies showed a low prevalence of GSC variants in sporadic forms of HS. The largest multicenter study in cases of predominantly Caucasian ethnicity identified pathogenic GSC variation in only 12 out of 188 (6.4%) patients with HS, of which 51% had fHS (Duchatelet et al., 2020). Smaller scale cohort studies in the United Kingdom revealed no GSC pathogenic variants in HS patients irrespective of family history of the disease (Ingram et al., 2013).
The human GSC is a multimeric, intramembrane-cleaving proteases composed of four subunit domains namely: Nicastrin (NCSTN), Presenilin Enhancer 2 (PEN2), Presenilin 1 (PSEN1) or PSEN2 and Anterior Pharynx Defective (APH) 1A or B. This gives rise to at least six different GSCs, which assemble fully in the endoplasmic reticulum and are transported to the cell membrane (Capell et al., 2005). The GSCs are dynamic and can exist in three conformational states; extended, intermediate and compact (Elad et al., 2015). Cryo-electron-microscopy single-particle analysis has elucidated the three-dimensional structure of human GCS, which was shown to have a horseshoe-shaped transmembrane domain (spanning 19 transmembrane segments) and a large extracellular domain (Lu et al., 2014).
The most widely reported variants in fHS lie in the nicastrin-coding gene NCSTN (OMIM #142690). Nicastrin is the largest subunit of the GSC (accounting for two-thirds of the molecular mass of the entire complex), and is postulated to be the substrate-recruiting protein of the GSC [specifically at the DYIGS and peptidase-like (DAP) region in the ectodomain (ECD)] (Shah et al., 2005). Nicastrin is essential for the assembly (particularly of the transmembrane domain) (Shah et al., 2005), maturation and stability of the GSC (Elad et al., 2015). The protein has a bilobed ECD and a single transmembrane domain (TMD) at its C-terminus (Xie et al., 2014). The interface between the two lobes is maintained by extensive van der Waals contacts, amongst which those formed between Phe287 (of the large loop) nestled within a pocket of hydrophobic amino acids Phe103, Leu171, Phe176 and Ile180 (of the small loop), is highly conserved (Xie et al., 2014). A loop extending from the core of the small lobe of the nicastrin ECD forms a lid which covers the putative substrate binding site within the large lobe, amongst which the residue Trp164 is vital (Xie et al., 2014). This amino acid, as well as Pro-141, Trp-164, Asn-165 and Gly-168 have been shown to be highly conserved in the NCST “lid” domain, but not essential for regulating GSC activity, including Notch (Zhang X et al., 2016). Fluorescence imaging microscopy of intact cells detected conformational change of the nicastrin ECD, which is brought closer to the membrane core upon binding an inhibitor (Elad et al., 2015). Residues Tyr337 and Glu333 (Shah et al., 2005) are both located within the substrate biding pocket ECD (Xie et al., 2014). The importance of Glu333 in proteolysis has been confirmed by mutagenesis studies, demonstrating abolished GSC cleavage, activity and maturation after substituting the residue (Shah et al., 2005; Dries et al., 2009), thus dispelling assertions that Glu333 is only involved in GSC maturation (Chávez-Gutiérrez et al., 2008). Nicastrin also forms complexes with PSEN1 and PSEN2, establishing a “secretasome” which allows for intramembranous proteolysis of the transmembrane proteins, including Notch (Yu et al., 2000).
The Notch signaling pathway is a highly conserved pathway involved in cell-cell communication. It regulates cellular differentiation and proliferation in continually renewing adult tissues such as skin. In these tissues, the notch receptor is activated by various ligands and cleaved by the GSC, releasing its intracellular domain which translocates to the nucleus to regulate gene expression (Mumm and Kopan, 2000). Because of the important roles played by Notch in epidermal and follicular homeostasis as well as inflammation, Notch dysregulation has been touted to underpin the molecular basis of HS in patients with pathogenic variants in GSC-protein coding genes (Okuyama et al., 2008; Melnik and Plewig, 2013). Interaction of the Notch receptor with its ligand (delta or jagged) results in two subsequent proteolytic cleavages of the receptor, the first of which is catalyzed by ADAM-family metalloproteinases and the second by the GSC. The product of the second cleavage, Notch intracellular domain (NICD), subsequently translocates to the nucleus where it acts as a transcriptional regulator for various genes (Borggrefe and Oswald, 2009) after complexing with CBF1-Suppressor of Hairless-LAG1 (CSL) and the co-activator mastermind (Wilson and Kovall, 2006).
In human models, downregulation of Notch signaling pathway has been shown to perturb keratinocyte differentiation and result in uncontrolled proliferation, disorganization of the suprabasal layers of the epidermis as well as dermal invasion (Thélu et al., 2002). These findings were also replicated in murine models wherein alteration in notch signaling resulted in altered sebaceous gland differentiation and terminal differentiation of the epidermis (Pan et al., 2004; Blanpain et al., 2006; Wang et al., 2008). Loss of ADAMS10 (responsible for notch receptor cleavage) (Groot and Vooijs, 2012) in murine models has been shown to result in impaired epidermal differentiation resulting in various pathologies including epidermal hyperproliferation and cyst formation as a result of altered notch signaling (Weber et al., 2011). Studies have also demonstrated that ADAMS10 is downregulated in HS (Frew and Navrazhina, 2020). Dysregulation of ADAM10-Notch signaling axis has been shown to impair the epithelial barrier and favor cutaneous dysbiosis (favoring Corynebacterium spp) (Sakamoto et al., 2021). Potentially, the altered microbiome promotes chronic inflammation by triggering the innate lymphoid cell population in an IL-17R dependent manner (Sakamoto et al., 2021). These findings may partly underpin the dysregulated cutaneous microbiome that accompanies HS (Mintoff et al., 2021a). Murine models have also demonstrated that disruption of notch nuclear target RBP-J results in cyst formation and epidermal hyperkeratinization (Yamamoto et al., 2003). A study scrutinizing publicly available genomic data revealed significant downregulation of Notch 1–4, and suggests ADAM17 as a key mediator in the pathogenesis of HS (Frew and Navrazhina, 2020).
Evidence for the role of Notch in HS can also be drawn from HS + disease such as HS-DDD. DDD is an autosomal dominant genodermatosis characterized by flexural and reticulated pigmentation. The condition is attributed to heterozygous variants in KRT5 (DDD1, OMIM #179850) at 12q13 and POFUT1 (DDD2, OMIM #615327) at 20q11. (Crovato et al., 1983; Stephan et al., 2021). Dubbed as “clinical collision” diseases, HS-DDD provides avenues for understanding pathophysiology and phenotypes (McGrath, 2018). Both POGLUT1 and POFUT1, causative genes in DDD, are established regulators of Notch pathway activity through their respective protein products namely protein-O glucosyltransferase 1 and protein O-fucosyltransferase 1 (Li et al., 2013; Basmanav et al., 2014). Congruently, patients with HS-DDD having underling POGLUT1 pathogenic variants demonstrate abnormal expression of genes encoding the critical element of the Notch pathway (Pavlovsky et al., 2018). Further molecular evidence is derived from two patients with HS-DDD, where reduced expression of NOTCH1 and NCSTN mRNA was demonstrated in lesional skin when compared to non-lesional skin, (Penha et al., 2020). HS-DDD with underlying POFUT1 pathogenic variants has been suggested to share similar Notch disturbance; however robust serological and in-vitro studies are lacking (González-Villanueva et al., 2018). Indirect evidence supporting the role of Notch downregulation in HS is provided by a case report in which a patient treated with a notch signaling inhibitor AL101 (BMS-906024) developed HS (Wiggins and Chon, 2020).
Conflicting findings have also been reported. Nicastrin, Notch 1–3, PIK3R3 and AKT3 levels were found to be significantly higher in lesional skin of 60 HS patients when compared to healthy controls. In lesional skin, these proteins were significantly higher in patients with mild (Hurley stage 1) disease compared to those with moderate and severe disease (Hurley stage II and III), despite excluding smoking and obesity as confounding factors (Hessam et al., 2021). Nicastrin overexpression (particularly dermal) has been associated with hypertrophic scarring as well as with inhibition of Notch signaling resulting in the suppressed production of fibrotic factors such as collagen 1 and 3 and TGF-β1 (Chen et al., 2021). Functional studies have shown that various HS-inducing nicastrin missense variants are active and sustain Notch signaling, and therefore do not fully support the concept of notch as being the singular pathophysiological processes involved in NCSTN-associated HS (Zhang and Sisodia, 2015). Indirect evidence for upregulation of notch can be drawn from other inflammatory pathologies. In patients with psoriasis, the proinflammatory cytokine serum amyloid A (SAA), known to be highly elevated in sera of HS patients (Witte-Händel et al., 2019), has been shown to upregulate Notch1 activity (Rooney et al., 2014).
PSEN has also been implicated in Notch signaling. The homologs PSEN1/PSEN2 are the catalytic subunit of the GSC. PSEN1/GSC is widely distributed in the cell (including its plasma membrane) whilst PSEN2/GSC is mostly restricted to late endosomes and lysosomes (Sannerud et al., 2016). Auto-compensatory mechanisms maintain PSEN levels in equilibrium (Stanga et al., 2018). In animal models, only zebrafish affected by the familial acne inversa-like indel mutation psen1. ptrp233fs (equivalent to human PSEN1 codon P242) had shown a significant alteration in Notch signaling (as opposed to early onset familial Alzheimer disease mutants). Upregulation of genes involved in inflammation was also observed in these mutants (Barthelson et al., 2021). The apparent upregulation of Notch signaling may possibly be accounted for by transcriptional adaptation, the process by which fragments of mutated mRNA translocate to the nucleus leading to transcriptional modulation of “adapting genes” (Sztal and Stainier, 2020). HS patients with underlying PSEN variants are designated OMIM # 613737.
Variants of Presenilin enhancer 2 (PSENEN), which codes for presenilin enhancer 2 (PEN2) have also been described in both sporadic HS as well as HS + DDD. The role of PEN2 in GSC function and Notch signaling was found to be conserved across species (Francis et al., 2002). PEN2 is the last unit to be incorporated within the GSC, with a PEN2 “retention mechanism” ensuring that only fully assembled GSCs are released from the endoplasmic reticulum to the plasma membrane (Capell et al., 2005; Christoph et al., 2007). The incomplete penetrance of PSENEN pathogenic variants has been described in three families by Riis et al (Theut Riis et al., 2020). Potentially, this can be attributed to the fact that PEN2 is not part of the GSC proteolytic domain. Additionally, it is possible that monoallelic pathogenic variants may not cause disease in the absence of other risk factors. In silico studies further support the concept that heterozygous pathogenic variants in NCSTN and PSENEN are not sufficient to cause disease (Nomura et al., 2014; Theut Riis et al., 2020).
APH-1 is the least well-characterized locus in the setting of HS, with a single APH-1B variant (p.His170Arg) having been associated with HS (Theut Riis et al., 2020). The authors postulate that this variant is unlikely to be causative of HS, citing studies which demonstrate much higher APH-1A expression in skin and fibroblast models in which APH-1A exclusively is involved in Notch signaling (Theut Riis et al., 2020). APH1 is a 7-transmembrane helix protein expressed as two homologous isoforms in humans, encoded by two genes (APH1a on chromosome 1; APH1b on chromosome 15). Both APH-1A and APH-1B adopt a water channel topology and transport water across the plasma membrane (Aguayo-Ortiz and Dominguez, 2019). The conserved His170 residue together with His196 play a major role in water transportation across the lipid bilayer (Aguayo-Ortiz and Dominguez, 2019; Dehury and Kepp, 2021).
The significance of GSC variants and the disruption in Notch signaling pathways and its targets in HS, is still evolving. Further functional and multiomic studies are required to determine the definitive role of the Notch signaling pathway in skin disease, including HS (Brandão et al., 2021). A working model of HS pathophysiology suggests that underlying genetic variants lead to lower protein expression which becomes functionally relevant under cellular stress mediated by friction (obesity), temperature and dysbiosis (Pink et al., 2016).
Syndromic Hidradenitis Suppurativa
In a subset of patients, HS develops as part of a constellation of other inflammatory, conditions (sHS). The classical HS syndromes in which genetic variation has been described include PASH (pyoderma gangrenosum, acne and suppurative hidradenitis), PAPASH (pyogenic arthritis + PASH) and SAPHO (synovitis, acne, pustulosis, hyperostosis and osteitis) syndromes (Garcovich et al., 2021a). A PSTPIP1 pathogenic variant was recently identified in a patient with proctitis, pyoderma gangrenosum, HS and fever (dubbed “PPHSF” syndrome) (Hieta et al., 2021). Supplementary Table S2 summarizes the genetic variants associated with sHS.
Braun-Falco et al., had documented the first two families with PASH. No pathogenic variants in PSTPIP1 were detected; however afflicted patients had hemi-allelic increase of the CCTG microsatellite motif (Braun-Falco et al., 2012). Other cases of PASH without PSTPIP1 pathogenic variants have also been reported (Gracia-Cazaña et al., 2015; Niv et al., 2017; Lamiaux et al., 2018). Similarly, no pathogenic variants were described in other forms of sHS namely, PsAPASH (Psoriatic arthritis and PASH) syndrome (Saraceno et al., 2015), PsAPSASH (Psoriasis, arthritis, pyoderma gangrenosum, synovitis, acne, suppurative hidradenitis) (Nikolakis et al., 2021) and PASS (pyoderma gangrenosum, acne, hidradenitis suppurativa and ankylosing spondylitis) (Bruzzese, 2012; Leuenberger et al., 2016) syndromes. Follicular occlusion syndromes such as the follicular occlusion triad [HS, acne conglobata and dissecting cellulitis of the scalp (Perifolliculitis capitis abscedens et suffodiens)] (Chicarilli, 1987) and the follicular tetrad (Vasanth and Chandrashekar, 2014) (follicular triad and pilonidal sinus) are described but likewise, they also lack an identified genetic driver.
PSTPIP1 is the locus most frequently implicated in sHS that features in PASH, PAPASH and PPHSF syndromes. Interestingly, PSTPIP1 variants have not been described in isolated forms of HS. PSTPIP1 codes for Proline-serine-threonine phosphatase-interacting protein 1. It has been demonstrated that PSTPIP1 regulates the transition of macrophage’s podosomes to filopodia-like protrusions and modulates their invasive migration (Starnes et al., 2014). Cellular studies have also demonstrated that pyrin modulates the intracellular distribution of PSTPIP1, and co-localizes at the leading edge of cells mitigating cell migration (Shoham et al., 2003; Waite et al., 2009; Akkaya-Ulum et al., 2015). In fact, PTSPIP1 mutant T-cells were shown to have altered f-actin polymerization (Janssen et al., 2018).
MEFV variants have been shown to be more frequent in patients with HS than healthy controls (Vural et al., 2019). A patient of Turkish origin suffering from comorbid PASH and familial Mediterranean fever (FMF) has been described. Targeted analysis in the MEFV gene identified the two heterozygous pathogenic variants (p.M680I and p. V726A); assessment of the rest of his genome was therefore lacking (Vural et al., 2017). Interestingly, a patient with PAPASH was heterozygous for a microsatellite elongation in the PSTPIP1 promotor region, and homozygous for the pathogenic MEFV p. Met694Val variant. However, he was asymptomatic for FMF. The authors suggest that this could possibly be the result of aberrant mutant Pyrin-PTSPIP1 interaction (Vural et al., 2019). The cause-effect relationship underlying the coexistence of a systemic proinflammatory susceptibility typical of FMF with inflammation of the PSU remains to be established.
Cytokine IL-1β may be the common denominator linking variants described in sHS or HS in combination with pyoderma gangrenosum (Galimberti et al., 2016; Witte-Händel et al., 2019). The protein products encoded by PSTPIP1 and MEFV interact in a multimeric protein complex that regulates assembly and activation of the inflammasome by promoting ASC oligomerization and Caspase-1 activation (Yu et al., 2007). The inflammasome regulates innate immunity and epithelial barrier defenses. On the other hand, gain of function mutations in NLRP3 result in increased IL-1 [a defining feature of Cryopyrin-associated periodic syndrome (CAPS)] (Kuemmerle-Deschner et al., 2017). Conflicting results have been observed with regards to IL-1β levels in patients with sHS. No statistically significant differences in serum Il-1β levels were described between patients with PASH syndrome and healthy controls (Marzano et al., 2014). Conversely, PASS has been characterized as an IL-1-driven autoinflammatory disease which responds to treatment with the Il-1 Receptor antagonist anakinra (Leuenberger et al., 2016). Anakinra has been proven to be ineffective in the treatment of a young female with PASH syndrome (Staub et al., 2015).
HS has also been documented to manifest in the setting of specific chromosomal disorders, where it does not constitute a classical diagnostic feature. It has been speculated that diminished Notch receptor processing and signaling could account for HS is the setting of Trisomy 21; however a definitive functional correlation remains elusive (Gasparic et al., 2017). A case report also describes the occurrence of unilaterally distributed HS, possibly due to constitutional mosaicism in a patient with trisomy 1q (Skroza et al., 2019).
Hidradenitis Suppurativa +
HS has been independently described in the setting of two other heritable conditions namely, HS-DDD and Familial Mediterranean Fever (HS-FMF).
A distinctive subtype of Dowling-Degos with HS (HS-DDD) is defined by heterozygous variants in PSENEN (OMIM # 613736) on 19q13. Patients having underlying PSENEN variants but suffering from DDD exclusively have been described (Ralser et al., 2017; Ren and Zeng, 2020). Interestingly, only obese family members harboring the pathogenic PSENEN c.62-1G > C splice variant manifested HS + DDD, whilst their lean, non-smoking relative who also harbored the same mutation manifested DDD exclusively (Ralser et al., 2017). Non-smoking, lean patients from another family having PSENEN 84_85insT variant manifested DDD without HS (Ralser et al., 2017). On the other hand, the c.216delC PSENEN variant was described in non-smoking, lean patients from two separate families manifesting DDD exclusively but also in an unrelated patient with DDD-HS, whose smoking history and weight were not documented (Ralser et al., 2017; Ren and Zeng, 2020; Theut Riis et al., 2020). This suggests that in the context of HS, PSENEN pathogenic variants exhibit incomplete penetrance and variable expressivity, and possibly only manifest disease in the setting of specific triggers such as obesity. A pathogenic NCSTN nonsense variant p. Arg583Ter (c.1747C > T) has also been described in a lean, non-smoking patient with HS-DDD. The variant segregated with the DDD phenotype, but not HS, in the proband’s daughter (Garcovich et al., 2020; Garcovich et al., 2021b). However, the pathogenicity of this variant and its relevance to HS-DDD has been disputed, mainly because the significance of co-existing KRT5 variants was downplayed and deemed benign (Hermasch et al., 2020).
HS can occur in combination with other inherited autoinflammatory syndromes. Two patients with co-morbid HS - mevalonate kinase deficiency (Benhadou et al., 2021), an autosomal recessive inborn error of metabolism which leads to chronic inflammation, have been described. Various studies have also described the co-existance of an HS phenotype in patients bearing pathogenic variation at the MEFV locus (Abbara et al., 2017; Vural et al., 2017; Jfri et al., 2019; Vural et al., 2019). This gene encodes pyrin, a protein which modulates the activity of the GSC. These studies suggest that HS and FMF are autoinflammatory disorders that may possibly share converging pathophysiologic processes. Despite the systemic proinflammatory state in these conditions, proof of causal associations remains lacking.
Genotype-Phenotype Correlations
Patients with HS exhibit extensive phenotypic heterogeneity making genotype-phenotype correlations difficult to establish (Kent, 2009). The task is further complicated by poor interrater reliability of HS phenotypes (van Straalen et al., 2018), differences in severity scoring (Zouboulis et al., 2019) as well as evidence of pleiotropism (Gratton et al., 2020). Notwithstanding, some general patterns are emerging, and encouraging results are emerging even from relatively low-powered studies (Marzano et al., 2022). HS patients having underlying NCSTN variants appear to have a follicular-type HS, in which comedones, papules and folliculitis predominate. Patients with this subtype of HS are likelier to manifest lesions in the nape and back and have co-morbid pilonidal sinus disease (PND) (Xu et al., 2016), a common co-morbidity and a possible intergluteal localization of disease in HS patients (Benhadou et al., 2019). Similarly, phenotyping of a large cohort of HS patients showed that cases exhibiting a follicular (LC2) type phenotype, typified by epidermal cysts, PND and comedones were more likely to have a family history of HS when compared to the patients with axillary-mammary (LC1) and gluteal (LC3) phenotypes (Canoui-Poitrine et al., 2013). An inverse correlation between LC1 HS phenotypes and NCSTN variants has been described (Frew et al., 2019). Patients with an endotype defined by GSC pathogenic variants and higher levels of serum IL-10 are more likely to be non-obese males with predominantly nodular lesions on the trunk and posterior sites and have a history of PND (referred to as “Cluster 1” HS patients). On the other hand, patients with high serum IL-1, IL-17, IL-16 and CRP are more likely to be obese and have later-onset disease, with tunnels and abscesses predominating (Cluster 2) (González-Manso et al., 2020). With regards to patients with sHS, both promoter and PSTPIP1 variants were found to be significantly associated with syndromic forms of disease (Frew et al., 2019).
In a broader sense, HS patients having an affected first-degree relative develop more severe disease at a younger age than their parents. The gender of the affected parents also influences the resulting phenotype in the offspring, with patients having an affected mother more frequently reporting axillary involvement, and patients with affected fathers being significantly more likely to involve the buttocks and the genitals. The mean number of affected body sites was found to be significantly higher in patients whose father has HS then those with a maternal history of the condition (Plenary Lectures, 2019).
Patients who are obese and smokers and without any underlying genetic variants are more likely to present with a “wet” phenotype characterized by draining abscesses in intertriginous regions. Conversely, lean non-smokers with a known family history are more likely to develop a “dry” phenotype characterized by follicular lesions in atypical regions such as the nuchal area and antecubital fossae (Vossen et al., 2020a; Mintoff et al., 2021b), or with syndromic HS.
Hidradenitis Suppurativa Genomics—Caveats and Challenges
The interpretation of genetic findings in HS presents several limitations. When compared to other common complex traits, there is a deficiency of large-scale genomic studies on ethnically diverse cohorts. The relative contribution of common vs. rare polymorphisms remains unascertained. Applying high-throughput sequencing studies to kindreds with early-onset familial or atypical HS phenotypes is a valid approach. However, variant prioritization and pathogenicity scoring can be complicated by pitfalls such as the overreliance on in-silico predictors and the use of inappropriate allele frequency cut-offs. Additionally, limited conclusions about the role of monoallelic variants causing recessive disorders can be drawn. It must be acknowledged that the assessment of allele frequencies in aggregate datasets unselected for disease, such as GnomAD, is a valuable approach. However, the presence of rare variants causative of late-onset disease in genomic databases can confound variant classification (Lek et al., 2016). Attributing causality to variants remains a considerable challenge, particularly for missense substitutions that are not structurally deleterious. Functional evaluation using in-vitro or in-vivo models are required to support pathogenicity and robustly define gene-disease associations for disputed loci. This is reinforced by conflicting interpretations of pathogenicity attributed to some variants in clinical databases. Furthermore, studies based on exome capture and sequencing may fail to identify deep intronic variants that modulate splicing or pathogenic structural variation. In the broader context of HS genomic architecture, it is essential to consider that studies sequencing cases with multigenerational early-onset disease are likely to skew towards the identification of high-penetrance variants. These represent the ‘low-hanging fruit’ of genomic discovery, at the expense of variants that lack adequate penetrance to drive familial segregation of disease. Plausibly, such intermediate penetrance variants predispose to later onset or milder disease.
This review is intrinsically limited by study selection criteria, and it is possible that some studies may not have been included (language other than English, Italian and Spanish, articles missing key data and conference proceedings). In addition, key phenotypic data, such as obesity and smoking status, as well as familial segregation of identified variants was not reported by some investigators.
Conclusion
The extent to which HS pathogenesis and risk are driven by the shared overlap between comorbid clinical risk factors, such as obesity and smoking, and one’s genetic predisposition remains unknown. The degree to which different variants contribute to the two main pathophysiological processes at the PSU namely hyperkeratosis and inflammation (Nomura, 2020) also remains unelucidated. The weak genotype-phenotype associations observed in HS are similar to other complex diseases. Potentially, this can be attributed to diagnostic delays, phenotypic heterogeneity and pleiotropic genetic effects acting against background modifiers such as changes in the composition of the skin microbiome.
Notwithstanding, the limited number of studies investigating HS by whole exome sequencing, and to a lesser extent by whole genome sequencing, show promising results and highlight the need for patients at the extreme ends of the HS phenotypic spectrum to be identified and prioritized for rare variant screening. To this end, phenotypic evaluation and categorization criteria need to be standardized to facilitate their use and interpretation in clinical care settings (Daxhelet et al., 2020; Frew et al., 2021).
The incompletely understood genomic risk factors of HS warrants further study, possibly by alternative approaches such as genome-wide association studies (GWAS). GWAS should elucidate the contribution of common genomic variants to HS and potentially identify new loci associated with this trait. Such endeavors require large-scale multicenter, collaborative genomic efforts (Daxhelet et al., 2021; Jabbour et al., 2021) which will allow for endotyping (González-Manso et al., 2020), deep phenotyping and, ultimately precision medicine (Robinson, 2012; Delude, 2015) for HS patients. Genetic variables also have the propensity to act as diagnostic and predictive HS biomarkers (Der Sarkissian et al., 2022). The evidence outlined in this review suggests that considering HS as a single disease may be misleading. Conversely, considering a precision-medicine approach tailored to every individual may be unrealistic in health care systems burdened by limited access to genetic testing and escalating health care costs. Important lessons can be derived from studies of other complex traits. Several investigators have shown that precision phenotyping of polygenic disease based on disease mechanisms is superior to traditional clinical classifications as it better identifies patients at risk of complications and can guide therapeutic choices (Ahlqvist et al., 2018). Whether or not dissecting the phenotypic heterogeneity of HS improves clinical outcomes remains to be assessed.
Author Contributions
All authors listed have made a substantial, direct, and intellectual contribution to the work and approved it for publication.
Conflict of Interest
The authors declare that the research was conducted in the absence of any commercial or financial relationships that could be construed as a potential conflict of interest.
Publisher’s Note
All claims expressed in this article are solely those of the authors and do not necessarily represent those of their affiliated organizations, or those of the publisher, the editors, and the reviewers. Any product that may be evaluated in this article, or claim that may be made by its manufacturer, is not guaranteed or endorsed by the publisher.
Supplementary Material
The Supplementary Material for this article can be found online at: https://www.frontiersin.org/articles/10.3389/fgene.2022.861241/full#supplementary-material
References
Abbara, S., Georgin-Lavialle, S., Stankovic Stojanovic, K., Bachmeyer, C., Senet, P., Buob, D., et al. (2017). Association of Hidradenitis Suppurativa and Familial Mediterranean Fever: A Case Series of 6 Patients. Jt. Bone Spine. 84 (2), 159–162. doi:10.1016/j.jbspin.2016.02.021
Aguayo-Ortiz, R., and Dominguez, L. (2019). APH-1A Component of γ-Secretase Forms an Internal Water and Ion-Containing Cavity. ACS Chem. Neurosci. 10 (6), 2931–2938. doi:10.1021/acschemneuro.9b00150
Ahlqvist, E., Storm, P., Käräjämäki, A., Martinell, M., Dorkhan, M., Carlsson, A., et al. (2018). Novel Subgroups of Adult-Onset Diabetes and Their Association with Outcomes: a Data-Driven Cluster Analysis of Six Variables. Lancet Diabetes Endocrinol. 6 (5), 361–369. doi:10.1016/s2213-8587(18)30051-2
Akkaya-Ulum, Y. Z., Balci-Peynircioglu, B., Purali, N., and Yilmaz, E. (2015). Pyrin-PSTPIP1 Colocalises at the Leading Edge during Cell Migration. Cell Biol Int. 39 (12), 1384–1394. doi:10.1002/cbin.10514
Barthelson, K., Dong, Y., Newman, M., and Lardelli, M. (2021). PRESENILIN 1 Mutations Causing Early-Onset Familial Alzheimer's Disease or Familial Acne Inversa Differ in Their Effects on Genes Facilitating Energy Metabolism and Signal Transduction. J. Alzheimers Dis. 82 (1), 327–347. doi:10.3233/JAD-210128
Basmanav, F. B., Oprisoreanu, A.-M., Pasternack, S. M., Thiele, H., Fritz, G., Wenzel, J., et al. (2014). Mutations in POGLUT1, Encoding Protein O-Glucosyltransferase 1, Cause Autosomal-Dominant Dowling-Degos Disease. Am. J. Hum. Genet. 94 (1), 135–143. doi:10.1016/j.ajhg.2013.12.003
Benhadou, F., Vlaeminck-Guillem, V., Duquesne, A., Mintoff, D., and Guillem, P. (2021). Association hidradénite suppurée et déficit en mévalonate kinase : deux cas. Ann. Dermatol. Vénéréologie - FMC. 1 (8Suppl. 1), 1A276–7. doi:10.1016/j.fander.2021.09.282
Benhadou, F., Van der Zee, H. H., Pascual, J. C., Rigopoulos, D., Katoulis, A., Liakou, A. I., et al. (2019). Pilonidal Sinus Disease: an Intergluteal Localization of Hidradenitis Suppurativa/acne Inversa: a Cross‐sectional Study Among 2465 Patients. Br. J. Dermatol. 181 (6), 1198–1206. doi:10.1111/bjd.17927
Blanpain, C., Lowry, W. E., Pasolli, H. A., and Fuchs, E. (2006). Canonical Notch Signaling Functions as a Commitment Switch in the Epidermal Lineage. Genes Dev. 20 (21), 3022–3035. doi:10.1101/gad.1477606
Borggrefe, T., and Oswald, F. (2009). The Notch Signaling Pathway: Transcriptional Regulation at Notch Target Genes. Cell. Mol. Life Sci. 66 (10), 1631–1646. doi:10.1007/s00018-009-8668-7
Brandão, L. A. C., Tricarico, P. M., Gratton, R., Agrelli, A., Zupin, L., Abou-Saleh, H., et al. (2021). Multiomics Integration in Skin Diseases with Alterations in Notch Signaling Pathway: PlatOMICs Phase 1 Deployment. Int. J. Mol. Sci. 22 (4), 1523. doi:10.3390/ijms22041523
Braun-Falco, M., Kovnerystyy, O., Lohse, P., and Ruzicka, T. (2012). Pyoderma Gangrenosum, Acne, and Suppurative Hidradenitis (PASH)?a New Autoinflammatory Syndrome Distinct from PAPA Syndrome. J. Am. Acad. Dermatol. 66 (3), 409–415. doi:10.1016/j.jaad.2010.12.025
Bruzzese, V. (2012). Pyoderma Gangrenosum, Acne Conglobata, Suppurative Hidradenitis, and Axial Spondyloarthritis. J. Clin. Rheumatol. 18 (8), 413–415. doi:10.1097/rhu.0b013e318278b84c
Calderón-Castrat, X., Bancalari-Díaz, D., Román-Curto, C., Romo-Melgar, A., Amorós-Cerdán, D., Alcaraz-Mas, L. A., et al. (2016). PSTPIP1 Gene Mutation in a Pyoderma Gangrenosum, Acne and Suppurative Hidradenitis (PASH) Syndrome. Br. J. Dermatol. 175 (1), 194–198. doi:10.1111/bjd.14383
Canoui-Poitrine, F., Le Thuaut, A., Revuz, J. E., Viallette, C., Gabison, G., Poli, F., et al. (2013). Identification of Three Hidradenitis Suppurativa Phenotypes: Latent Class Analysis of a Cross-Sectional Study. J. Invest. Dermatol. 133 (6), 1506–1511. doi:10.1038/jid.2012.472
Capell, A., Beher, D., Prokop, S., Steiner, H., Kaether, C., Shearman, M. S., et al. (2005). γ-Secretase Complex Assembly within the Early Secretory Pathway. J. Biol. Chem. 280 (8), 6471–6478. doi:10.1074/jbc.m409106200
Chávez-Gutiérrez, L., Tolia, A., Maes, E., Li, T., Wong, P. C., and de Strooper, B. (2008). Glu(332) in the Nicastrin Ectodomain Is Essential for Gamma-Secretase Complex Maturation but Not for its Activity. J. Biol. Chem. 283 (29), 20096–20105. doi:10.1074/jbc.M803040200
Chen, L., Zhang, X., Yu, Z., Song, Y., Wang, T., Yang, K., et al. (2021). Inhibition of Notch Intracellular Domain Suppresses Cell Activation and Fibrotic Factors Production in Hypertrophic Scar Fibroblasts versus Normal Skin Fibroblasts. Ann. Plast. Surg. 86 (4), 400–405. doi:10.1097/sap.0000000000002540
Chicarilli, Z. N. (1987). Follicular Occlusion Triad: Hidradenitis Suppurativa, Acne Conglobata, and Dissecting Cellulitis of the Scalp. Ann. Plast. Surg. 18 (3), 230–237. doi:10.1097/00000637-198703000-00009
Christoph, K., Johanna, S., and Matthias, F. (2007). Endoplasmic Reticulum Retention of the γ-secretase Complex Component Pen2 by Rer1. EMBO Rep. 8, 743–748. doi:10.1038/sj.embor.7401027
Crovato, F., Nazzari, G., and Rebora, A. (1983). Dowling-Degos Disease (Reticulate Pigmented Anomaly of the Flexures) Is an Autosomal Dominant Condition. Br. J. Dermatol. 108 (4), 473–476. doi:10.1111/j.1365-2133.1983.tb04602.x
Danby, F. W., Jemec, G. B. E., Marsch, W. C., and von Laffert, M. (2013). Preliminary Findings Suggest Hidradenitis Suppurativa May Be Due to Defective Follicular Support. Br. J. Dermatol. 168 (5), 1034–1039. doi:10.1111/bjd.12233
Daxhelet, M., Daoud, M., Suppa, M., Benhadou, F., Njimi, H., Tzellos, T., et al. (2021). European Registry for Hidradenitis Suppurativa: State of Play. J. Eur. Acad. Dermatol. Venereol. 35 (4), e274–6. doi:10.1111/jdv.17023
Daxhelet, M., Suppa, M., White, J., Benhadou, F., Thorlacius, L. R., Jemec, G. B. E., et al. (2020). Proposed Definitions of Typical Lesions in Hidradenitis Suppurativa. Dermatology. 236 (5), 431–438. doi:10.1159/000507348
Dehury, B., and Kepp, K. P. (2021). Membrane Dynamics of γ‐secretase with the Anterior Pharynx‐defective 1B Subunit. J. Cell Biochem 122 (1), 69–85. doi:10.1002/jcb.29832
Delude, C. M. (2015). Deep Phenotyping: The Details of Disease. Nature 527 (7576), S14–S15. doi:10.1038/527s14a
Der Sarkissian, S., Hessam, S., Kirby, J. S., Lowes, M. A., Mintoff, D., Naik, H. B., et al. (2022). Identification of Biomarkers and Critical Evaluation of Biomarker Validation in Hidradenitis Suppurativa. JAMA Dermatol. doi:10.1001/jamadermatol.2021.4926
Dries, D. R., Shah, S., Han, Y.-H., Yu, C., Yu, S., Shearman, M. S., et al. (2009). Glu-333 of Nicastrin Directly Participates in γ-Secretase Activity. J. Biol. Chem. 284 (43), 29714–29724. doi:10.1074/jbc.m109.038737
Duchatelet, S., Miskinyte, S., Delage, M., Ungeheuer, M.-N., Lam, T., Benhadou, F., et al. (2020). Low Prevalence of GSC Gene Mutations in a Large Cohort of Predominantly Caucasian Patients with Hidradenitis Suppurativa. J. Invest. Dermatol. 140 (10), 2085–2088. doi:10.1016/j.jid.2019.10.025
Duchatelet, S., Miskinyte, S., Join‐Lambert, O., Ungeheuer, M. N., Francès, C., Nassif, A., et al. (2015). First Nicastrin Mutation in PASH (Pyoderma Gangrenosum, Acne and Suppurative Hidradenitis) Syndrome. Br. J. Dermatol. 173 (2), 610–612. doi:10.1111/bjd.13668
Elad, N., De Strooper, B., Lismont, S., Hagen, W., Veugelen, S., Arimon, M., et al. (2015). The Dynamic Conformational Landscape of Gamma-Secretase. J. Cell Sci. 128 (3), 589–598. doi:10.1242/jcs.164384
Faraji Zonooz, M., Sabbagh-Kermani, F., Fattahi, Z., Fadaee, M., Akbari, M. R., Amiri, R., et al. (2016). Whole Genome Linkage Analysis Followed by Whole Exome Sequencing Identifies Nicastrin (NCSTN) as a Causative Gene in a Multiplex Family with γ-Secretase Spectrum of Autoinflammatory Skin Phenotypes. J. Invest. Dermatol. 136 (6), 1283–1286. doi:10.1016/j.jid.2016.02.801
Fitzsimmons, J. S., Fitzsimmons, E. M., and Gilbert, G. (1984). Familial Hidradenitis Suppurativa: Evidence in Favour of Single Gene Transmission. J. Med. Genet. 21 (4), 281–285. doi:10.1136/jmg.21.4.281
Fitzsimmons, J. S., and Guilbert, P. R. (1985). A Family Study of Hidradenitis Suppurativa. J. Med. Genet. 22 (5), 367–373. doi:10.1136/jmg.22.5.367
Fitzsimmons, J. S., Guilbert, P. R., and Fitzsimmons, E. M. (1985). Evidence of Genetic Factors in Hidradenitis Suppurativa. Br. J. Dermatol. 113 (1), 1–8. doi:10.1111/j.1365-2133.1985.tb02037.x
Francis, R., McGrath, G., Zhang, J., Ruddy, D. A., Sym, M., Apfeld, J., et al. (2002). aph-1 and Pen-2 Are Required for Notch Pathway Signaling, γ-Secretase Cleavage of βAPP, and Presenilin Protein Accumulation. Developmental Cell 3 (1), 85–97. doi:10.1016/s1534-5807(02)00189-2
Frew, J. W., Hawkes, J. E., Sullivan‐Whalen, M., Gilleaudeau, P., and Krueger, J. G. (2019). Inter‐rater Reliability of Phenotypes and Exploratory Genotype-Phenotype Analysis in Inherited Hidradenitis Suppurativa. Br. J. Dermatol. 181 (3), 566–571. doi:10.1111/bjd.17695
Frew, J. W., Lowes, M. A., Goldfarb, N., Butt, M., Piguet, V., O’Brien, E., et al. (2021). Global Harmonization of Morphological Definitions in Hidradenitis Suppurativa for a Proposed Glossary. JAMA Dermatol. 157 (4), 449–455. doi:10.1001/jamadermatol.2020.5467
Frew, J. W., and Navrazhina, K. (2020). No Evidence that Impaired Notch Signalling Differentiates Hidradenitis Suppurativa from Other Inflammatory Skin Diseases. Br. J. Dermatol. 182 (4), 1042–1043. doi:10.1111/bjd.18593
Galimberti, R. L., Vacas, A. S., Bollea Garlatti, M. L., and Torre, A. C. (2016). The Role of Interleukin-1β in Pyoderma Gangrenosum. JAAD Case Rep. 2 (5), 366–368. doi:10.1016/j.jdcr.2016.07.007
Gao, M., Wang, P.-G., Cui, Y., Yang, S., Zhang, Y.-H., Lin, D., et al. (2006). Inversa Acne (Hidradenitis Suppurativa): a Case Report and Identification of the Locus at Chromosome 1p21.1-1q25.3. J. Invest. Dermatol. 126 (6), 1302–1306. doi:10.1038/sj.jid.5700272
Garcovich, S., Genovese, G., Moltrasio, C., Malvaso, D., Marzano, A. V., and Papash, Ps. A. P. A. S. H. (2021). PASH, PAPASH, PsAPASH, and PASS: The Autoinflammatory Syndromes of Hidradenitis Suppurativa. Clin. Dermatol. 39 (2), 240–247. doi:10.1016/j.clindermatol.2020.10.016
Garcovich, S., Tricarico, P. M., Meddour, C. N., Giovanardi, G., Peris, K., Crovella, S., et al. (2021). Comorbid Acne Inversa and Dowling-Degos Disease Due to a Single NCSTN Mutation: Is There Enough Evidence? Reply from the Authors. Br. J. Dermatol. 184 (2), 375–376. doi:10.1111/bjd.19543
Garcovich, S., Tricarico, P. M., Nait‐Meddour, C., Giovanardi, G., Peris, K., Crovella, S., et al. (2020). Novel Nicastrin Mutation in Hidradenitis Suppurativa-Dowling-Degos Disease Clinical Phenotype: More Than Just Clinical Overlap? Br. J. Dermatol. 183 (4), 758–759. doi:10.1111/bjd.19121
Gasparic, J., Theut Riis, P., and Jemec, G. B. (2017). Recognizing Syndromic Hidradenitis Suppurativa: a Review of the Literature. J. Eur. Acad. Dermatol. Venereol. 31 (11), 1809–1816. doi:10.1111/jdv.14464
González-Manso, A., Agut-Busquet, E., Romaní, J., Vilarrasa, E., Bittencourt, F., Mensa, A., et al. (2020). Hidradenitis Suppurativa: Proposal of Classification in Two Endotypes with Two-step Cluster Analysis. Dermatology. 237 (3), 365–377. doi:10.1159/000511045
González-Villanueva, I., Gutiérrez, M., Hispán, P., Betlloch, I., and Pascual, J. C. (2018). Novel POFUT1 Mutation Associated with Hidradenitis Suppurativa-Dowling-Degos Disease Firm up a Role for Notch Signalling in the Pathogenesis of This Disorder. Br. J. Dermatol. 178 (4), 984–986. doi:10.1111/bjd.16264
Gracia-Cazaña, T., Frias, M., Roselló, R., Vera-Álvarez, J., and Gilaberte, Y. (2015). PASH Syndrome Associated with Osteopoikilosis. Int. J. Dermatol. 54 (9), e369–371. doi:10.1111/ijd.12827
Gratton, R., Tricarico, P. M., Moltrasio, C., Lima Estevão de Oliveira, A. S., Brandão, L., Marzano, A. V., et al. (2020). Pleiotropic Role of Notch Signaling in Human Skin Diseases. Int. J. Mol. Sci. 21 (12), E4214. doi:10.3390/ijms21124214
Groot, A. J., and Vooijs, M. A. (2012). The Role of Adams in Notch Signaling. Adv. Exp. Med. Biol. 727, 15–36. doi:10.1007/978-1-4614-0899-4_2
He, Y., Xu, H., Li, C., Zhang, X., Zhou, P., Xiao, X., et al. (2019). Nicastrin/miR-30a-3p/RAB31 Axis Regulates Keratinocyte Differentiation by Impairing EGFR Signaling in Familial Acne Inversa. J. Invest. Dermatol. 139 (1), 124–134. doi:10.1016/j.jid.2018.07.020
Hermasch, M. A., Schön, M. P., and Frank, J. (2020). Comorbid Acne Inversa and Dowling-Degos Disease Due to a Single NCSTN Mutation - Is There Enough Evidence? Br. J. Dermatol. 184 (2), 374. doi:10.1111/bjd.19539
Hessam, S., Gambichler, T., Skrygan, M., Scholl, L., Sand, M., Meyer, T., et al. (2021). Increased Expression Profile of NCSTN, Notch and PI3K/AKT3 in Hidradenitis Suppurativa. J. Eur. Acad. Dermatol. Venereol. 35, 203. doi:10.1111/jdv.16962
Hieta, N., Nuutinen, H., Roivas, J., Salminen, K., Kujari, H., Talve, L., et al. (2021). Severe Ulcerative Proctitis, Pyoderma Gangrenosum, Hidradenitis Suppurativa and Fever in a Patient with a Rare Variant of the PSTPIP1 Gene. Clin. Exp. Dermatol. 46 (7), 1387–1390. doi:10.1111/ced.14754
Ingram, J. R., Wood, M., John, B., Butler, R., and Anstey, A. V. (2013). Absence of Pathogenic γ-secretase Mutations in a South Wales Cohort of Familial and Sporadic Hidradenitis Suppurativa (Acne Inversa). Br. J. Dermatol. 168 (4), 874–876. doi:10.1111/bjd.12048
Ittisoponpisan, S., Islam, S. A., Khanna, T., Alhuzimi, E., David, A., and Sternberg, M. J. E. (2019). Can Predicted Protein 3D Structures Provide Reliable Insights into whether Missense Variants Are Disease Associated? J. Mol. Biol. 431 (11), 2197–2212. doi:10.1016/j.jmb.2019.04.009
Jabbour, A. J., van Straalen, K. R., Colvin, A., Prens, E. P., and Petukhova, L. (2021). Clinical Translation of Hidradenitis Suppurativa Genetic Studies Requires Global Collaboration. Br. J. Dermatol. 186 (1), 183–185. doi:10.1111/bjd.20749
Janssen, W. J. M., Grobarova, V., Leleux, J., Jongeneel, L., van Gijn, M., van Montfrans, J. M., et al. (2018). Proline-serine-threonine Phosphatase Interacting Protein 1 (PSTPIP1) Controls Immune Synapse Stability in Human T Cells. J. Allergy Clin. Immunol. 142 (6), 1947–1955. doi:10.1016/j.jaci.2018.01.030
Jfri, A., Litvinov, I. V., Netchiporouk, E., and O'Brien, E. (2020). Novel Variants of MEFV and NOD2 Genes in Familial Hidradenitis Suppurativa: A Case Report. SAGE Open Med. Case Rep. 8, 2050313X20953113. doi:10.1177/2050313X20953113
Jfri, A. H., O’Brien, E. A., Litvinov, I. V., Alavi, A., and Netchiporouk, E. (2019). Hidradenitis Suppurativa: Comprehensive Review of Predisposing Genetic Mutations and Changes. J. Cutan. Med. Surg. 23 (5), 519–527. doi:10.1177/1203475419852049
Jfri, A., Nassim, D., O’Brien, E., Gulliver, W., Nikolakis, G., and Zouboulis, C. C. (2021). Prevalence of Hidradenitis Suppurativa. JAMA Dermatol. 157 (8), 924–931. doi:10.1001/jamadermatol.2021.1677
Jiao, T., Dong, H., Jin, L., Wang, S., and Wang, J. (2013). A Novel Nicastrin Mutation in a Large Chinese Family with Hidradenitis Suppurativa. Br. J. Dermatol. 168 (5), 1141–1143. doi:10.1111/bjd.12135
Kan, T., Takahagi, S., Shindo, H., Tanaka, A., Kawai, M., and Hide, M. (2018). A Unique Clinical Phenotype of a Patient Bearing a Newly Identified Deletion Mutation in the PSENEN Gene along with the Pathogenic Serum Desmoglein-1 Antibody. Clin. Exp. Dermatol. 43 (3), 329–332. doi:10.1111/ced.13326
Kent, J. W. (2009). Analysis of Multiple Phenotypes. Genet. Epidemiol. 33 (S1), S33–S39. doi:10.1002/gepi.20470
Kjaersgaard Andersen, R., Clemmensen, S. B., Larsen, L. A., Hjelmborg, J. V. B., Ødum, N., Jemec, G. B. E., et al. (2021). Evidence of Gene-Gene Interaction in Hidradenitis Suppurativa: a Nationwide Registry Study of Danish Twins. Br. J. Dermatol. 186 (1), 78–85. doi:10.1111/bjd.20654
Kopanos, C., Tsiolkas, V., Kouris, A., Chapple, C. E., Albarca Aguilera, M., Meyer, R., et al. (2019). VarSome: the Human Genomic Variant Search Engine. Bioinforma Oxf Engl. 35 (11), 1978–1980. doi:10.1093/bioinformatics/bty897
Kotzerke, M., Mitri, F., Marbach, F., Enk, A., and Haenssle, H. (2021). A Case of PAPASH Syndrome in a Young Man Carrying a Novel Heterozygote Missense Variant in PSTPIP1. J. Eur. Acad. Dermatol. Venereol. 35 (7), e439–40. doi:10.1111/jdv.17171
Kuemmerle-Deschner, J. B., Verma, D., Endres, T., Broderick, L., de Jesus, A. A., Hofer, F., et al. (2017). Brief Report: Clinical and Molecular Phenotypes of Low-Penetrance Variants of NLRP3 : Diagnostic and Therapeutic Challenges. Arthritis Rheumatol. 69 (11), 2233–2240. doi:10.1002/art.40208
Lamiaux, M., Dabouz, F., Wantz, M., Lebas, D., Lasek, A., Courivaud, D., et al. (2018). Successful Combined Antibiotic Therapy with Oral Clindamycin and Oral Rifampicin for Pyoderma Gangrenosum in Patient with PASH Syndrome. JAAD Case Rep. 4 (1), 17–21. doi:10.1016/j.jdcr.2017.05.005
Lek, M., Karczewski, K. J., Minikel, E. V., Samocha, K. E., Banks, E., Fennell, T., et al. (2016). Analysis of Protein-Coding Genetic Variation in 60,706 Humans. Nature 536 (7616), 285–291. doi:10.1038/nature19057
Leuenberger, M., Berner, J., Di Lucca, J., Fischer, L., Kaparos, N., Conrad, C., et al. (2016). PASS Syndrome: An IL-1-Driven Autoinflammatory Disease. Dermatology 232 (2), 254–258. doi:10.1159/000443648
Li, C., Xu, H., and Wang, B. (2018). Is SAPHO Syndrome Linked to PASH Syndrome and Hidradenitis Suppurativa by Nicastrin Mutation? A Case Report. J. Rheumatol. 45 (11), 1605–1607. doi:10.3899/jrheum.171007
Li, C.-R., Jiang, M.-J., Shen, D.-B., Xu, H.-X., Wang, H.-S., Yao, X., et al. (2011). Two Novel Mutations of the Nicastrin Gene in Chinese Patients with Acne Inversa. Br. J. Dermatol. 165 (2), 415–418. doi:10.1111/j.1365-2133.2011.10372.x
Li, C., Li, W., Xu, H., Zhang, X., Su, B., Zhang, W., et al. (2017). PSENEN Mutation Carriers with Co-manifestation of Acne Inversa (AI) and Dowling-Degos Disease (DDD): Is AI or DDD the Subphenotype? J. Invest. Dermatol. 137 (10), 2234–2236. doi:10.1016/j.jid.2017.05.021
Li, M., Cheng, R., Liang, J., Yan, H., Zhang, H., Yang, L., et al. (2013). Mutations in POFUT1, Encoding Protein O-Fucosyltransferase 1, Cause Generalized Dowling-Degos Disease. Am. J. Hum. Genet. 92 (6), 895–903. doi:10.1016/j.ajhg.2013.04.022
Lin, Y.-Y., Lin, L.-H., Kang, J., Li, C.-R., and Xiao, X.-M. (2021). Hidradenitis Suppurativa Associated with a Novel NCSTN Mutation and Concomitant Klippel-Trenaunay Syndrome. Eur. J. Dermatol. 31 (2), 246–248. doi:10.1684/ejd.2021.4005
Liu, M., Davis, J. W., Idler, K. B., Mostafa, N. M., Okun, M. M., and Waring, J. F. (2016). Genetic Analysis of NCSTN for Potential Association with Hidradenitis Suppurativa in Familial and Nonfamilial Patients. Br. J. Dermatol. 175 (2), 414–416. doi:10.1111/bjd.14482
Liu, Y., Gao, M., Lv, Y.-m., Yang, X., Ren, Y.-q., Jiang, T., et al. (2011). Confirmation by Exome Sequencing of the Pathogenic Role of NCSTN Mutations in Acne Inversa (Hidradenitis Suppurativa). J. Invest. Dermatol. 131 (7), 1570–1572. doi:10.1038/jid.2011.62
Liy-Wong, C., Kim, M., Kirkorian, A. Y., Eichenfield, L. F., Diaz, L. Z., Horev, A., et al. (2021). Hidradenitis Suppurativa in the Pediatric Population. JAMA Dermatol. 157 (4), 385–391. doi:10.1001/jamadermatol.2020.5435
Lu, P., Bai, X.-c., Ma, D., Xie, T., Yan, C., Sun, L., et al. (2014). Three-dimensional Structure of Human γ-secretase. Nature 512 (7513), 166–170. doi:10.1038/nature13567
Ma, S., Yu, Y., Yu, G., and Zhang, F. (2014). Identification of One Novel Mutation of the NCSTN Gene in One Chinese Acne Inversa Family. Dermatologica Sinica 32 (2), 126–128. doi:10.1016/j.dsi.2013.06.003
Marzano, A. V., Ceccherini, I., Gattorno, M., Fanoni, D., Caroli, F., Rusmini, M., et al. (2014). Association of Pyoderma Gangrenosum, Acne, and Suppurative Hidradenitis (PASH) Shares Genetic and Cytokine Profiles with Other Autoinflammatory Diseases. Medicine (Baltimore) 93 (27), e187. doi:10.1097/md.0000000000000187
Marzano, A. V., Genovese, G., Moltrasio, C., Tricarico, P. M., Gratton, R., Piaserico, S., et al. (2022). Whole-Exome Sequencing in 10 Unrelated Patients with Syndromic Hidradenitis Suppurativa: A Preliminary Step for a Genotype-Phenotype Correlation. Dermatology., 1–10. doi:10.1159/000521263
Marzano, A. V., Trevisan, V., Gattorno, M., Ceccherini, I., De Simone, C., and Crosti, C. (2013). Pyogenic Arthritis, Pyoderma Gangrenosum, Acne, and Hidradenitis Suppurativa (PAPASH): a New Autoinflammatory Syndrome Associated with a Novel Mutation of the PSTPIP1 Gene. JAMA Dermatol. 149 (6), 762–764. doi:10.1001/jamadermatol.2013.2907
McGrath, J. A. (2018). Concurrent Hidradenitis Suppurativa and Dowling-Degos Disease Taken Down a 'Notch'. Br. J. Dermatol. 178 (2), 328. doi:10.1111/bjd.16068
Melnik, B. C., and Plewig, G. (2013). Impaired Notch-MKP-1 Signalling in Hidradenitis Suppurativa: an Approach to Pathogenesis by Evidence from Translational Biology. Exp. Dermatol. 22 (3), 172–177. doi:10.1111/exd.12098
Mintoff, D., Borg, I., and Pace, N. P. (2021a). The Clinical Relevance of the Microbiome in Hidradenitis Suppurativa: A Systematic Review. Vaccines 9 (10), 1076. doi:10.3390/vaccines9101076
Mintoff, D., Pace, N. P., Bauer, P., and Borg, I. (2021b). A novel c.671_682del NCSTN variant in a family with Hidradenitis Suppurativa: a pilot study. Clin. Exp. Dermatol. 46 (7), 1306–1308. doi:10.1111/ced.14677
Mintoff, D., Pace, N. P., Bauer, P., and Borg, I. (2021c). A novel c.671_682del NCSTN variant in a family with Hidradenitis Suppurativa: a pilot study. Clin. Exp. Dermatol. 46, 1306. doi:10.1111/ced.14677
Miskinyte, S., Nassif, A., Merabtene, F., Ungeheuer, M.-N., Join-Lambert, O., Jais, J.-P., et al. (2012). Nicastrin Mutations in French Families with Hidradenitis Suppurativa. J. Invest. Dermatol. 132 (6), 1728–1730. doi:10.1038/jid.2012.23
Mumm, J. S., and Kopan, R. (2000). Notch Signaling: From the outside in. Developmental Biol. 228 (2), 151–165. doi:10.1006/dbio.2000.9960
Nikolakis, G., Kreibich, K., Vaiopoulos, A., Kaleta, K., Talas, J., Becker, M., et al. (2021). Case Report: PsAPSASH Syndrome: an Alternative Phenotype of Syndromic Hidradenitis Suppurativa Treated with the IL-17A Inhibitor Secukinumab. F1000Res 10, 381. doi:10.12688/f1000research.52100.1
Nishimori, N., Hayama, K., Kimura, K., Fujita, H., Fujiwara, K., and Terui, T. (2020). A Novel NCSTN Gene Mutation in a Japanese Family with Hidradenitis Suppurativa. Acta Derm Venereol. 100 (17), adv00283. doi:10.2340/00015555-3632
Niv, D., Ramirez, J. A., and Fivenson, D. P. (2017). Pyoderma Gangrenosum, Acne, and Hidradenitis Suppurativa (PASH) Syndrome with Recurrent Vasculitis. JAAD Case Rep. 3 (1), 70–73. doi:10.1016/j.jdcr.2016.11.006
Nomura, T. (2020). Hidradenitis Suppurativa as a Potential Subtype of Autoinflammatory Keratinization Disease. Front. Immunol. 11, 847. doi:10.3389/fimmu.2020.00847
Nomura, Y., Nomura, T., Sakai, K., Sasaki, K., Ohguchi, Y., Mizuno, O., et al. (2013). A Novel Splice Site Mutation inNCSTNunderlies a Japanese Family with Hidradenitis Suppurativa. Br. J. Dermatol. 168 (1), 206–209. doi:10.1111/j.1365-2133.2012.11174.x
Nomura, Y., Nomura, T., Suzuki, S., Takeda, M., Mizuno, O., Ohguchi, Y., et al. (2014). A Novel NCSTN Mutation Alone May Be Insufficient for the Development of Familial Hidradenitis Suppurativa. J. Dermatol. Sci. 74 (2), 180–182. doi:10.1016/j.jdermsci.2014.01.013
Okuyama, R., Tagami, H., and Aiba, S. (2008). Notch Signaling: its Role in Epidermal Homeostasis and in the Pathogenesis of Skin Diseases. J. Dermatol. Sci. 49 (3), 187–194. doi:10.1016/j.jdermsci.2007.05.017
Pan, Y., Lin, M.-H., Tian, X., Cheng, H.-T., Gridley, T., Shen, J., et al. (2004). γ-Secretase Functions through Notch Signaling to Maintain Skin Appendages but Is Not Required for Their Patterning or Initial Morphogenesis. Developmental Cell 7 (5), 731–743. doi:10.1016/j.devcel.2004.09.014
Pavlovsky, M., Sarig, O., Eskin-Schwartz, M., Malchin, N., Bochner, R., Mohamad, J., et al. (2018). A Phenotype Combining Hidradenitis Suppurativa with Dowling-Degos Disease Caused by a Founder Mutation in PSENEN. Br. J. Dermatol. 178 (2), e160. doi:10.1111/bjd.16285
Penha, R., Cortez de Almeida, R. F., Mariz, J., Lisboa, L., Barbosa, L., and da Silva, R. (2020). The Deregulation of NOTCH Pathway, Inflammatory Cytokines, and Keratinization Genes in Two Dowling-Degos Disease Patients with Hidradenitis Suppurativa. Am. J. Med. Genet. 182 (11), 2662–2665. doi:10.1002/ajmg.a.61800
Peter, D. C. V., Smith, F. J. D., Wilson, N. J., and Danda, S. (2021). PSENEN Mutation in Coexistent Hidradenitis Suppurativa and Dowling-Degos Disease. Indian Dermatol. Online J. 12 (1), 147–149. doi:10.4103/idoj.IDOJ_218_20
Pink, A. E., Dafou, D., Desai, N., Holmes, O., Hobbs, C., Smith, C. H., et al. (2016). Hidradenitis Suppurativa: Haploinsufficiency of Gamma-Secretase Components Does Not Affect Gamma-Secretase Enzyme Activityin Vitro. Br. J. Dermatol. 175 (3), 632–635. doi:10.1111/bjd.14621
Pink, A. E., Simpson, M. A., Brice, G. W., Smith, C. H., Desai, N., Mortimer, P. S., et al. (2011). PSENEN and NCSTN Mutations in Familial Hidradenitis Suppurativa (Acne Inversa). J. Invest. Dermatol. 131 (7), 1568–1570. doi:10.1038/jid.2011.42
Pink, A. E., Simpson, M. A., Desai, N., Dafou, D., Hills, A., Mortimer, P., et al. (2012). Mutations in the γ-Secretase Genes NCSTN , PSENEN , and PSEN1 Underlie Rare Forms of Hidradenitis Suppurativa (Acne Inversa). J. Invest. Dermatol. 132 (10), 2459–2461. doi:10.1038/jid.2012.162
Qian, Y-T., Xiao, M., Liu, K., Ma, D-L., and Zhang, X. (2021). Two Novel Mutations of the γ-secretase Genes in Chinese Acne Inversa (Hidradenitis Suppurativa). Exp. Dermatol.. doi:10.1111/exd.14496
Ralser, D. J., Basmanav, F. B. Ü., Tafazzoli, A., Wititsuwannakul, J., Delker, S., Danda, S., et al. (2017). Mutations in γ-secretase Subunit-Encoding PSENEN Underlie Dowling-Degos Disease Associated with Acne Inversa. J. Clin. Invest. 127 (4), 1485–1490. doi:10.1172/jci90667
Ratnamala, U., Jhala, D., Jain, N. K., Saiyed, N. M., Raveendrababu, M., Rao, M. V., et al. (2016). Expanding the Spectrum Ofγ-Secretase Gene Mutation-Associated Phenotypes: Two Novel Mutations Segregating with Familial Hidradenitis Suppurativa (Acne Inversa) and Acne Conglobata. Exp. Dermatol. 25 (4), 314–316. doi:10.1111/exd.12911
Ren, J., and Zeng, L. Y. (2020). Scrotal Dowling-Degos Disease Caused by a Novel Frameshift Variant in Gamma-Secretase Subunit Presenile Enhancer Gene. Australas. J. Dermatol. 61 (4), e399–402. doi:10.1111/ajd.13316
Richards, S., Aziz, N., Bale, S., Bick, D., Das, S., Gastier-Foster, J., et al. (2015). Standards and Guidelines for the Interpretation of Sequence Variants: a Joint Consensus Recommendation of the American College of Medical Genetics and Genomics and the Association for Molecular Pathology. Genet. Med. 17 (5), 405–424. doi:10.1038/gim.2015.30
Robinson, P. N. (2012). Deep Phenotyping for Precision Medicine. Hum. Mutat. 33 (5), 777–780. doi:10.1002/humu.22080
Rodrigues, C. H., Pires, D. E., and Ascher, D. B. (2018). DynaMut: Predicting the Impact of Mutations on Protein Conformation, Flexibility and Stability. Nucleic Acids Res. 46 (W1), W350–W355. doi:10.1093/nar/gky300
Rooney, P., Connolly, M., Gao, W., McCormick, J., Biniecka, M., Sullivan, O., et al. (2014). Notch-1 Mediates Endothelial Cell Activation and Invasion in Psoriasis. Exp. Dermatol. 23 (2), 113–118. doi:10.1111/exd.12306
Saito, N., Minami-Hori, M., Nagahata, H., Nozaki, H., Iinuma, S., Igawa, S., et al. (2018). Novel PSTPIP1 Gene Mutation in Pyoderma Gangrenosum, Acne and Suppurative Hidradenitis Syndrome. J. Dermatol. 45 (8), e213–e214. doi:10.1111/1346-8138.14259
Sakamoto, K., Jin, S.-P., Goel, S., Jo, J.-H., Voisin, B., Kim, D., et al. (2021). Disruption of the Endopeptidase ADAM10-Notch Signaling axis Leads to Skin Dysbiosis and Innate Lymphoid Cell-Mediated Hair Follicle Destruction. Immunity 54 (10), 2321–2337. doi:10.1016/j.immuni.2021.09.001
Saleh Al-Ali, F. M., Ratnamala, U., Mehta, T. Y., Naveed, M., Al-Ali, M. T., Al-Khaja, N., et al. (2010). Hidradenitis Suppurativa (Or Acne Inversa) with Autosomal Dominant Inheritance Is Not Linked to Chromosome 1p21.1-1q25.3 Region. Exp. Dermatol. 19 (9), 851–853. doi:10.1111/j.1600-0625.2010.01088.x
Sannerud, R., Esselens, C., Ejsmont, P., Mattera, R., Rochin, L., Tharkeshwar, A. K., et al. (2016). Restricted Location of PSEN2/γ-Secretase Determines Substrate Specificity and Generates an Intracellular Aβ Pool. Cell 166 (1), 193–208. doi:10.1016/j.cell.2016.05.020
Saraceno, R., Babino, G., Chiricozzi, A., Zangrilli, A., and Chimenti, S. (2015). PsAPASH: a New Syndrome Associated with Hidradenitis Suppurativa with Response to Tumor Necrosis Factor Inhibition. J. Am. Acad. Dermatol. 72 (1), e42–e44. doi:10.1016/j.jaad.2014.10.002
Shah, S., Lee, S.-F., Tabuchi, K., Hao, Y.-H., Yu, C., LaPlant, Q., et al. (2005). Nicastrin Functions as a γ-Secretase-Substrate Receptor. Cell 122 (3), 435–447. doi:10.1016/j.cell.2005.05.022
Shi, T.-w., Bai, N., Zhang, J.-a., Lu, F., Chen, X.-b., Kong, X.-d., et al. (2018). Mutations in the γ-secretase Genes PSEN1, PSENEN, and NCSTN in a Family with Acne Inversa. Eur. J. Dermatol. EJD 28 (3), 374–376. doi:10.1684/ejd.2018.3244
Shoham, N. G., Centola, M., Mansfield, E., Hull, K. M., Wood, G., Wise, C. A., et al. (2003). Pyrin Binds the PSTPIP1/CD2BP1 Protein, Defining Familial Mediterranean Fever and PAPA Syndrome as Disorders in the Same Pathway. Proc. Natl. Acad. Sci. U.S.A. 100 (23), 13501–13506. doi:10.1073/pnas.2135380100
Skroza, N., Mambrin, A., Tolino, E., Bernardini, N., Proietti, I., Anzalone, A., et al. (2019). A Case of Hidradenitis Suppurativa Linked to Trisomy 1q. J. Eur. Acad. Dermatol. Venereol. 33 Suppl 6 (Suppl. 6), 32–33. doi:10.1111/jdv.15824
Stanga, S., Vrancx, C., Tasiaux, B., Marinangeli, C., Karlström, H., and Kienlen‐Campard, P. (2018). Specificity of Presenilin‐1‐ and Presenilin‐2‐dependent γ‐secretases towards Substrate Processing. J. Cell Mol Med 22 (2), 823–833. doi:10.1111/jcmm.13364
Starnes, T. W., Bennin, D. A., Bing, X., Eickhoff, J. C., Grahf, D. C., Bellak, J. M., et al. (2014). The F-BAR Protein PSTPIP1 Controls Extracellular Matrix Degradation and Filopodia Formation in Macrophages. Blood 123 (17), 2703–2714. doi:10.1182/blood-2013-07-516948
Staub, J., Pfannschmidt, N., Strohal, R., Braun-Falco, M., Lohse, P., Goerdt, S., et al. (2015). Successful Treatment of PASH Syndrome with Infliximab, Cyclosporine and Dapsone. J. Eur. Acad. Dermatol. Venereol. 29 (11), 2243–2247. doi:10.1111/jdv.12765
Stephan, C., Kurban, M., and Abbas, O. (2021). Dowling‐Degos Disease: a Review. Int. J. Dermatol. 60 (8), 944–950. doi:10.1111/ijd.15385
Straalen, K. R., Prens, E. P., Willemsen, G., Boomsma, D. I., and Zee, H. (2020). Contribution of Genetics to the Susceptibility to Hidradenitis Suppurativa in a Large, Cross-Sectional Dutch Twin Cohort. JAMA Dermatol. 156 (12), 1359–1362. doi:10.1001/jamadermatol.2020.3630 [Internet] [cited 2020 Dec 6]; Available at: https://jamanetwork.com/journals/jamadermatology/fullarticle/2771622
Sztal, T. E., and Stainier, D. Y. R. (2020). Transcriptional Adaptation: a Mechanism Underlying Genetic Robustness. Development 147 (15), dev186452. doi:10.1242/dev.186452
Takeichi, T., Matsumoto, T., Nomura, T., Takeda, M., Niwa, H., Kono, M., et al. (2020). A Novel NCSTN Missense Mutation in the Signal Peptide Domain Causes Hidradenitis Suppurativa, Which Has Features Characteristic of an Autoinflammatory Keratinization Disease. Br. J. Dermatol. 182 (2), 491–493. doi:10.1111/bjd.18445
Thélu, J., Rossio, P., and Favier, B. (2002). Notch Signalling Is Linked to Epidermal Cell Differentiation Level in Basal Cell Carcinoma, Psoriasis and Wound Healing. BMC Dermatol. 2, 7. doi:10.1186/1471-5945-2-7
Theut Riis, P., Loft, I. C., Yazdanyar, S., Kjaersgaard Andersen, R., Pedersen, O. B., Ring, H. C., et al. (2020). Full Exome Sequencing of 11 Families with Hidradenitis Suppurativa. J. Eur. Acad. Dermatol. Venereol. JEADV 35 (5), 1203–1211. doi:10.1111/jdv.17095
van Straalen, K. R., Verhagen, T., Horváth, B., Ardon, C., Vossen, A. R. J. V., Driessen, R., et al. (2018). Poor Interrater Reliability of Hidradenitis Suppurativa Phenotypes. J. Am. Acad. Dermatol. 79 (3), 577–578. doi:10.1016/j.jaad.2018.02.048
Vasanth, V., and Chandrashekar, B. (2014). Follicular Occlusion Tetrad. Indian Dermatol. Online J. 5 (4), 491–493. doi:10.4103/2229-5178.142517
Venselaar, H., te Beek, T. A., Kuipers, R. K., Hekkelman, M. L., and Vriend, G. (2010). Protein Structure Analysis of Mutations Causing Inheritable Diseases. An E-Science Approach with Life Scientist Friendly Interfaces. BMC Bioinformatics 11 (1), 548. doi:10.1186/1471-2105-11-548
von Laffert, M., Stadie, V., Wohlrab, J., and Marsch, W. C. (2011). Hidradenitis Suppurativa/acne Inversa: Bilocated Epithelial Hyperplasia with Very Different Sequelae. Br. J. Dermatol. 164 (2), 367–371. doi:10.1111/j.1365-2133.2010.10034.x
Vossen, A. R. J. V., van Straalen, K. R., Swagemakers, S. M. A., de Klein, J. E. M. M., Stubbs, A. P., Venter, D. J., et al. (2020a). A Novel Nicastrin Mutation in a Three-Generation Dutch Family with Hidradenitis Suppurativa: a Search for Functional Significance. J. Eur. Acad. Dermatol. Venereol. JEADV 34 (10), 2353–2361. doi:10.1111/jdv.16310
Vossen, A. R. J. V., van Straalen, K. R., Swagemakers, S. M. A., de Klein, J. E. M. M., Stubbs, A. P., Venter, D. J., et al. (2020b). A Novel Nicastrin Mutation in a Three‐generation Dutch Family with Hidradenitis Suppurativa: a Search for Functional Significance. J. Eur. Acad. Dermatol. Venereol. 34 (10), 2353–2361. doi:10.1111/jdv.16310
Vural, S., Baumgartner, M., Lichtner, P., Eckstein, G., Hariry, H., Chen, W. C., et al. (2021). Investigation of Gamma Secretase Gene Complex Mutations in German Population with Hidradenitis Suppurativa Designate a Complex Polygenic Heritage. J. Eur. Acad. Dermatol. Venereol. 35 (6), 1386–1392. doi:10.1111/jdv.17163
Vural, S., Gündoğdu, M., Gökpınar İli, E., Durmaz, C. D., Vural, A., Steinmüller‐Magin, L., et al. (2019). Association of Pyrin Mutations and Autoinflammation with Complex Phenotype Hidradenitis Suppurativa: a Case-Control Study. Br. J. Dermatol. 180 (6), 1459–1467. doi:10.1111/bjd.17466
Vural, S., Gundogdu, M., Kundakci, N., and Ruzicka, T. (2017). Familial Mediterranean Fever Patients with Hidradenitis Suppurativa. Int. J. Dermatol. 56 (6), 660–663. doi:10.1111/ijd.13503
Waite, A. L., Schaner, P., Richards, N., Balci-Peynircioglu, B., Masters, S. L., Brydges, S. D., et al. (2009). Pyrin Modulates the Intracellular Distribution of PSTPIP1. Plos One 4 (7), e6147. doi:10.1371/journal.pone.0006147
Wang, B., Yang, W., Wen, W., Sun, J., Su, B., Liu, B., et al. (2010). γ-Secretase Gene Mutations in Familial Acne Inversa. Science 330, 1065. doi:10.1126/science.1196284
Wang, X., Pasolli, H. A., Williams, T., and Fuchs, E. (2008). AP-2 Factors Act in Concert with Notch to Orchestrate Terminal Differentiation in Skin Epidermis. J. Cell Biol 183 (1), 37–48. doi:10.1083/jcb.200804030
Weber, S., Niessen, M. T., Prox, J., Lüllmann-Rauch, R., Schmitz, A., Schwanbeck, R., et al. (2011). The Disintegrin/metalloproteinase Adam10 Is Essential for Epidermal Integrity and Notch-Mediated Signaling. Development 138 (3), 495–505. doi:10.1242/dev.055210
Wiggins, C. J., and Chon, S. Y. (2020). Cutaneous Effects of Notch Inhibitor Therapy: A Report of Two Cases. Case Rep. Dermatol. Med. 2020, 8842242. doi:10.1155/2020/8842242
Wilson, J. J., and Kovall, R. A. (2006). Crystal Structure of the CSL-Notch-Mastermind Ternary Complex Bound to DNA. Cell 124 (5), 985–996. doi:10.1016/j.cell.2006.01.035
Witte-Händel, E., Wolk, K., Tsaousi, A., Irmer, M. L., Mößner, R., Shomroni, O., et al. (2019). The IL-1 Pathway Is Hyperactive in Hidradenitis Suppurativa and Contributes to Skin Infiltration and Destruction. J. Invest. Dermatol. 139 (6), 1294–1305. doi:10.1016/j.jid.2018.11.018
Wu, C., Yang, J., Zhang, S., Li, J., Jin, H., and Zhang, X. (2018). A Novel NCSTN Gene Mutation in a Chinese Family with Acne Inversa. Mol. Genet. Genomics 293 (6), 1469–1475. doi:10.1007/s00438-018-1475-9
Wu, J., Ge, H., Fan, Y., Zhen, Q., Tang, L., and Sun, L. (2020). Novel Mutation of the NCSTN Gene Identified in a Chinese Acne Inversa Family. Ann. Dermatol. 32 (3), 237–242. doi:10.5021/ad.2020.32.3.237
Xiao, X.-M., Zhou, P.-J., Zhu, C.-H., Lin, L.-H., Liu, J.-J., and Han, Y. (2020). Coexistence of Acne Inversa with Psoriasis and Dowling-Degos Disease Harboring Impaired PSENEN-Notch Signaling. Chin. Med. J. (Engl). 133 (19), 2383–2385. doi:10.1097/cm9.0000000000001040
Xiao, X., He, Y., Li, C., Zhang, X., Xu, H., and Wang, B. (2016). Nicastrin Mutations in Familial Acne Inversa Impact Keratinocyte Proliferation and Differentiation through the Notch and Phosphoinositide 3-kinase/AKT Signalling Pathways. Br. J. Dermatol. 174 (3), 522–532. doi:10.1111/bjd.14223
Xiao, Y. J., Yang, Y., and Liang, Y. H. (2018). hsa-miR-155 Targeted NCSTN 3'UTR Mutation Promotes the Pathogenesis and Development of Acne Inversa. Int. J. Clin. Exp. Pathol. 11 (4), 1878–1889.
Xie, T., Yan, C., Zhou, R., Zhao, Y., Sun, L., Yang, G., et al. (2014). Crystal Structure of the γ-secretase Component Nicastrin. Proc. Natl. Acad. Sci. U.S.A. 111 (37), 13349–13354. doi:10.1073/pnas.1414837111
Xu, H., Xiao, X., Hui, Y., Zhang, X., He, Y., Li, C., et al. (2016). Phenotype of 53 Chinese Individuals with Nicastrin Gene Mutations in Association with Familial Hidradenitis Suppurativa (Acne Inversa). Br. J. Dermatol. 174 (4), 927–929. doi:10.1111/bjd.14268
Yamamoto, N., Tanigaki, K., Han, H., Hiai, H., and Honjo, T. (2003). Notch/RBP-J Signaling Regulates Epidermis/hair Fate Determination of Hair Follicular Stem Cells. Curr. Biol. 13 (4), 333–338. doi:10.1016/s0960-9822(03)00081-2
Yu, G., Nishimura, M., Arawaka, S., Levitan, D., Zhang, L., Tandon, A., et al. (2000). Nicastrin Modulates Presenilin-Mediated Notch/glp-1 Signal Transduction and βAPP Processing. Nature 407 (6800), 48–54. doi:10.1038/35024009
Yu, J.-W., Fernandes-Alnemri, T., Datta, P., Wu, J., Juliana, C., Solorzano, L., et al. (2007). Pyrin Activates the ASC Pyroptosome in Response to Engagement by Autoinflammatory PSTPIP1 Mutants. Mol. Cell 28 (2), 214–227. doi:10.1016/j.molcel.2007.08.029
Zhang, C., Wang, L., Chen, L., Ren, W., Mei, A., Chen, X., et al. (2013). Two Novel Mutations of the NCSTN Gene in Chinese Familial Acne Inverse. J. Eur. Acad. Dermatol. Venereol. JEADV 27 (12), 1571–1574. doi:10.1111/j.1468-3083.2012.04627.x
Zhang, X., He, Y., Xu, H., and Wang, B. (2020). First PSENEN Mutation in PASH Syndrome. J. Dermatol. 47 (11), 1335–1337. doi:10.1111/1346-8138.15527
Zhang, X., and Sisodia, S. S. (2015). Acne Inversa Caused by Missense Mutations in NCSTN Is Not Fully Compatible with Impairments in Notch Signaling. J. Invest. Dermatol. 135 (2), 618–620. doi:10.1038/jid.2014.399
Zhang, X., Sullivan, E., Scimeca, M., Wu, X., Li, Y.-m., and Sisodia, S. S. (2016). Evidence that the "Lid" Domain of Nicastrin Is Not Essential for Regulating γ-Secretase Activity. J. Biol. Chem. 291 (13), 6748–6753. doi:10.1074/jbc.c115.701649
Zhang, S., Meng, J., Jiang, M., and Zhao, J. (2016). Characterization of a Novel Mutation in the NCSTN Gene in a Large Chinese Family with Acne Inversa. Acta Derm Venerol 96 (3), 408–409. doi:10.2340/00015555-2259
Zhang, Z., Zhang, B., Guo, Y., Chen, J., Zheng, S., Gao, X., et al. (2021). A Novel Mutation of the NCSTN Gene in a Chinese Hidradenitis Suppurativa Family with Familial Comedones as the Main Clinical Manifestation. Int. J. Dermatol. doi:10.1111/ijd.16021
Zhou, P., Liu, J., Xu, T., Guo, Y., Han, Y., He, Y., et al. (2021). Mutations in γ-secretase Subunit-Encoding PSENEN Gene Alone May Not Be Sufficient for the Development of Acne Inversa. J. Dermatol. Sci. 103 (21), 7300143–7300812. doi:10.1016/j.jdermsci.2021.06.007
Zhou, C., Wen, G.-D., Soe, L. M., Xu, H.-J., Du, J., and Zhang, J.-Z. (2016). Novel Mutations in PSENEN Gene in Two Chinese Acne Inversa Families Manifested as Familial Multiple Comedones and Dowling-Degos Disease. Chin Med J (Engl). 129 (23), 2834–2839. doi:10.4103/0366-6999.194648
Zhou, X., Edmonson, M. N., Wilkinson, M. R., Patel, A., Wu, G., Liu, Y., et al. (2016). Exploring Genomic Alteration in Pediatric Cancer Using ProteinPaint. Nat. Genet. 48 (1), 4–6. doi:10.1038/ng.3466
Keywords: hidradenitis suppurativa, genetics, pathophysiology, gamma secretase (γ-secretase), familial
Citation: Pace NP, Mintoff D and Borg I (2022) The Genomic Architecture of Hidradenitis Suppurativa—A Systematic Review. Front. Genet. 13:861241. doi: 10.3389/fgene.2022.861241
Received: 24 January 2022; Accepted: 07 March 2022;
Published: 23 March 2022.
Edited by:
Jared C Roach, Institute for Systems Biology (ISB), United StatesReviewed by:
Chiara Moltrasio, IRCCS Ca ‘Granda Foundation Maggiore Policlinico Hospital, ItalyMarcelo Távora Mira, Pontifical Catholic University of Parana, Brazil
Copyright © 2022 Pace, Mintoff and Borg. This is an open-access article distributed under the terms of the Creative Commons Attribution License (CC BY). The use, distribution or reproduction in other forums is permitted, provided the original author(s) and the copyright owner(s) are credited and that the original publication in this journal is cited, in accordance with accepted academic practice. No use, distribution or reproduction is permitted which does not comply with these terms.
*Correspondence: Nikolai Paul Pace, Tmlrb2xhaS5wLnBhY2VAdW0uZWR1Lm10
†These authors share first authorship