- Department of Cardiology, Zhongshan Hospital, Fudan University, Shanghai, China
Background: Coronary chronic total occlusion (CTO) disease is common and its specific characteristic is collateral formation. The Integrated analysis of angiogenesis related lncRNA-miRNA-mRNA network remains unclear and might provide target for future studies.
Methods: A total of five coronary artery disease (control group) and five CTO (CTO group) patients were selected for deep RNA and miRNA sequencing. The expression profiles of lncRNAs, mRNAs circRNA and miRNAs were obtained. Gene Ontology (GO) and Kyoto Encyclopedia of Genes and Genomes (KEGG) pathway enrichment analyses were then performed. The expression of a 14q32 miRNA gene cluster, including miRNA-494, miRNA-495 and miRNA-329, were selected to be determined in another larger patient cohort. Analysis of the lncRNA-miRNA495-mRNA network was constructed to find potential targets for future studies.
Results: A total of 871 lncRNAs, 1,080 mRNAs, 138 circRNAs and 56 miRNAs were determined as differentially expressed (DE) in CTO patients compared with control patients. GO and KEGG analyses revealed that the top terms included MAPK signaling pathway, HIF-1 signaling pathway, EGFR tyrosine kinase inhibitor resistance, embryonic organ development, wound healing, MAPK signaling pathway and JAK-STAT signaling pathway, which are related to angiogenesis. The expression of miRNA-494, miRNA-495 and miRNA-329 were all significantly down-regulated in CTO patients and they were confirmed to be down-regulated in another cohort of 68 patients. Then we divided the CTO patients into two groups according to CC grade (poor CC group, CC = 0 or one; good CC group, CC = 2). MiRNA-494, miRNA-495 and miRNA-329 were found to be down-regulated in good CC group compared with poor CC group. Analysis of the lncRNA-miRNA495-mRNA network showed 3 DE lncRNA sponges (NONHSAG008675, NONHSAG020957 and NONHSAG010989), 4 DE lncRNA targets (NONHSAT079547.2, NONHSAT081776.2, NONHSAT148555.1 and NONHSAT150928.1) and 2 DE mRNA targets (RAD54L2 and ZC3H4) of miRNA495.
Conclusion: This study revealed that the lncRNA-miRNA-mRNA network might play a critical role in angiogenesis in CTO patients.
Introduction
Cardiovascular disease is still the leading cause of death worldwide. The prevalence of chronic total occlusions (CTO) among patients with known coronary artery disease (CAD) can be as high as 30–50% (Srinivas et al., 2002; Christofferson et al., 2005). CTO disease is defined as a complete vessel occlusion of native coronary artery and the estimated occlusion duration is more than 3 months. If the relief of angina symptoms and/or an ischemia reduction in the CTO territory can be expected, current guidelines recommended that revascularization can be considered (Windecker et al., 20142014). Thus, new methods or biomarkers screening potential candidates for revascularization are of interest of both clinical and basic science researchers.
One of the specific characteristics of CTO is collateral formation, which can be seen in a majority of these patients (Werner, 2014). Although well-developed collaterals are not enough to provide sufficient blood supply (Sachdeva et al., 2014), they have been demonstrated to be related with improved ventricular function (Werner et al., 2005; Choi et al., 2013). According to the collateral size and filling, collateral vessels can be classified by collateral connection (CC) grade (Meisel et al., 2013). CC grade was defined as follows: CC 0, no continuous connection between donor and recipient artery; CC 1, continuous, threadlike connection (diameter ≤0.3 mm); CC 2, continuous, small, side-branch-like size of the collateral throughout its course (diameter ≥0.4 mm). The major two processes of collateral growth are arteriogenesis and angiogenesis (Zimarino et al., 2014). The underlying mechanisms were complex, including shear stress and molecular and cellular response to hypoxia.
MicroRNAs (miRNAs) are small non-coding RNA molecules and are well investigated in last decades. They have been demonstrated to play an important role in angiogenesis. The roles of miRNAs have been deeply studied in stable CAD (Economou et al., 2015) and myocardial infarction (Chistiakov et al., 2016). However, expression profile and underline mechanisms of miRNAs in CTO patients have not been thoroughly investigated. Recently, long noncoding RNAs (lncRNAs) (Zhao et al., 2020) and circular RNAs (circRNAs) (Liu et al., 2020) have been suggested to be associated with angiogenesis. A complicated network of lncRNAs, circRNAs and miRNAs has been proposed to regulate mRNA function. Therefore, it is necessary to investigate lncRNA-miRNA-mRNA regulatory networks to comprehensively understand their roles in angiogenesis in CTO patients.
In this study, we determined miRNA, circRNAs, mRNAs and lncRNAs expression profiles in CTO patients with deep RNA sequencing (RNA-seq). Gene Ontology (GO) and Kyoto Encyclopedia of Genes and Genomes (KEGG) analyses were performed to explore the potential regulatory functions of differentially expressed (DE) profiles. A network analysis between lncRNA-miRNA-mRNA was also conducted. Then we focused on a 14q32 miRNA gene cluster, including miRNA-494, miRNA-495 and miRNA-329, which were confirmed to be down-regulated in another larger sample of CTO patients.
Methods
Patients
A total of five CAD (control group) and five CTO (CTO group) patients were selected for deep RNA and miRNA sequencing. They were all male, aged from 45 to 65 years old, with no left ventricular dysfunction/myocardial infarction history/tumor history, normal liver and renal function. All of the 10 samples were analyzed for lncRNA, mRNA and circRNA sequencing. For miRNA sequencing, five CAD and four CTO samples (the other one was abandoned because of RNA degradation) were analyzed. We selected another cohort of 68 patients to verify expression of miRNAs, including 22 CAD and 46 CTO patients. The CAD patients had a 50–90% stenosis of at least one main vessel. The exclusive criteria were: symptomatic peripheral arterial disease, recent ST-segment elevation myocardial infarction, decompensated heart failure, any concomitant inflammation or infectious diseases, neoplastic diseases, and severe liver and kidney dysfunction. We collected all the patients’ venous blood sample upon hospitalization (within 24 h). The blood sample was centrifuged at 1,500 g for 10 min to precipitate blood cells and plasma was then frozen at −80°C until use.
This study was approved by the medical ethics committee of Zhongshan Hospital. Informed consent was obtained from all patients. All procedures performed in studies involving human participants were in accordance with the ethical standards of the institutional and/or national research committee and with the Helsinki declaration and its later amendments.
RNA Extraction
RNAs were isolated from the patients’ plasma using TRIzol reagent (Life Technologies). RNA concentrations were measured with a NanoDrop ND-2000 instrument (Thermo Fisher Scientific) and miRNA concentrations were measured with a NanoDrop ND-1000 instru-ment (Thermo Fisher Scientific). Then the high-tthroughput sequencing was performed by WuXi NextCODE company (Shanghai, China) (Cui et al., 2020; Li et al., 2020).
MiRNA Sequencing
NEB Next Multiplex Small RNA Library Prep Set for Illumina (New England Biolabs) was performed to construct miRNA sequencing libraries. The quality of the libraries was evaluated by a Bioanalyser 2100 system (Agilent Technologies). A HiSeq 4000 sequencing system (Illumina) was used for sequencing for 50 cycles according to the manufacturer’s instructions. Cutadapt software (v1.9.3) was used to trim the adaptor sequences from the sequencing data. Trimmed readswere aligned tohuman reference genome (hg19) using Bowtie. Mapped reads were used to identify known miRNAs applying miRBasev21. MiRDeep2 weas used to predict novel miRNAs.
RNA Sequencing
TruSeq Stranded Total RNA Library Prep Kit (Illumina) was used to construct RNA sequencing libraries. The quality was evaluated by a Bioanalyser 2100 system (Agilent Technologies). Then, single-stranded DNA molecules were clustered and sequenced for 150 cycles on an Illumina HiSeq 2500 sequencing system (Illumina). Paired-end reads were acquired from the Illumina HiSeq sequencer, and the quality control was performed with Q30. Cutadapt software (v1.9.3) was used for removal of 3’ adaptor-trimming and low-quality reads. Alignment of the high-quality clean reads to the human reference genome (hg19) was performed by hisat2 (v2.0.4). The transcriptome was assembled using Cufflinks. Cuffcompare was used to identify known lncRNAs in NONCODE V5.0 and novel lncRNAs. Acfs was used to identify circRNAs. Then we used circBase to separate known circRNAs and novel circRNAs.
Differentially Expressed Analysis
EdgeR was used to identify differential expressed miNRA, mRNAs, lncRNAs and circRNAs (Benjamini Hochber method corrected p-value <0.05, Fold change >2) for the compare between control and CTO group.
Functional Enrichment Analysis
We used “ClusterProfiler” package in R software to perform functional enrichment analysis, and GO biological processes and KEGG pathways at the significant level (q-value < 0.01) were employed.
Construction of the lncRNA-miRNA-mRNA Network
The lncRNA-miRNA interactions were predicted by NPinter v4.0 and LncBase v3.0. We merged interactions with strong and weak evidence. We required each lncRNA-miRNA interactions shared with miRNA more than two times. And the lncRNA-miRNA interactions should be both significantly up/down-regulated expressed with adjusted p-value < 0.01. The targets of differentially expressed miRNA were predicted by miRTarBase. Also, mRNA-miRNA pairs should be both significantly expressed with adjusted p-value < 0.01. The network was plotted by Cytoscape.
Reverse Transcription-PCR
Total RNAs were extracted from plasma with TRIzol reagent according to manufacturer’s protocol (Invitrogen; Thermo Fisher Scientific, Inc.). MicroRNA qRT-PCR syb kit (Taqman) was used for generating cDNA from microRNA and qPCR detection. We used ABI 7500 real-time PCR system to perform real-time PCR. The qPCR conditions were as follows: 95°C for 15 min, 30 cycles of 95°C for 5 s, and 60°C for 30 s. The relative expression of the miRNAs was normalized to that of U6 by using the 2-ΔΔCq cycle threshold method. We used Student t test to determine statistical significance between the groups. All statistical analyses were performed by SPSS 17.0 (SSPS Inc., Chicago, IL, United States). A p < 0.05 was considered significant.
Results
Characteristics of Patients
The patients’ baseline characteristics were shown in Table 1. Briefly, they were all male, aged from 45 to 65 years old, with no renal dysfunction and heart failure. The CAD patients were all presented with left anterior descending (LAD) stenosis of 70–90%. As for CTO vessel in CTO group, all the occluded vessels are LADs. All of the five CTO patients for RNA sequencing had good collaterals with CC grade of 2.
Overview of lncRNA, mRNA, circRNA and miRNA Expression in CTO Patients
The expression heatmaps of lncRNA, mRNA, circRNA and miRNA were shown in Figure 1. Compared with control samples, the CTO samples had 871 significantly and DE lncRNA transcripts, of which 83 were up-regulated and 788 were down-regulated. For mRNA, there were 1,080 significantly and DE mRNA transcripts, of which 166 transcripts were up-regulated and 914 were down-regulated. For circRNA, there were 138 significantly and DE circRNA transcripts and all of them were down-regulated. Finally, we detected 56 DE miRNAs between the two groups, of which 24 were up-regulated and 32 were down-regulated. The top significantly up-regulated and down-regulated lncRNA, mRNA, circRNA and miRNA were summarized in Tables 2–5.
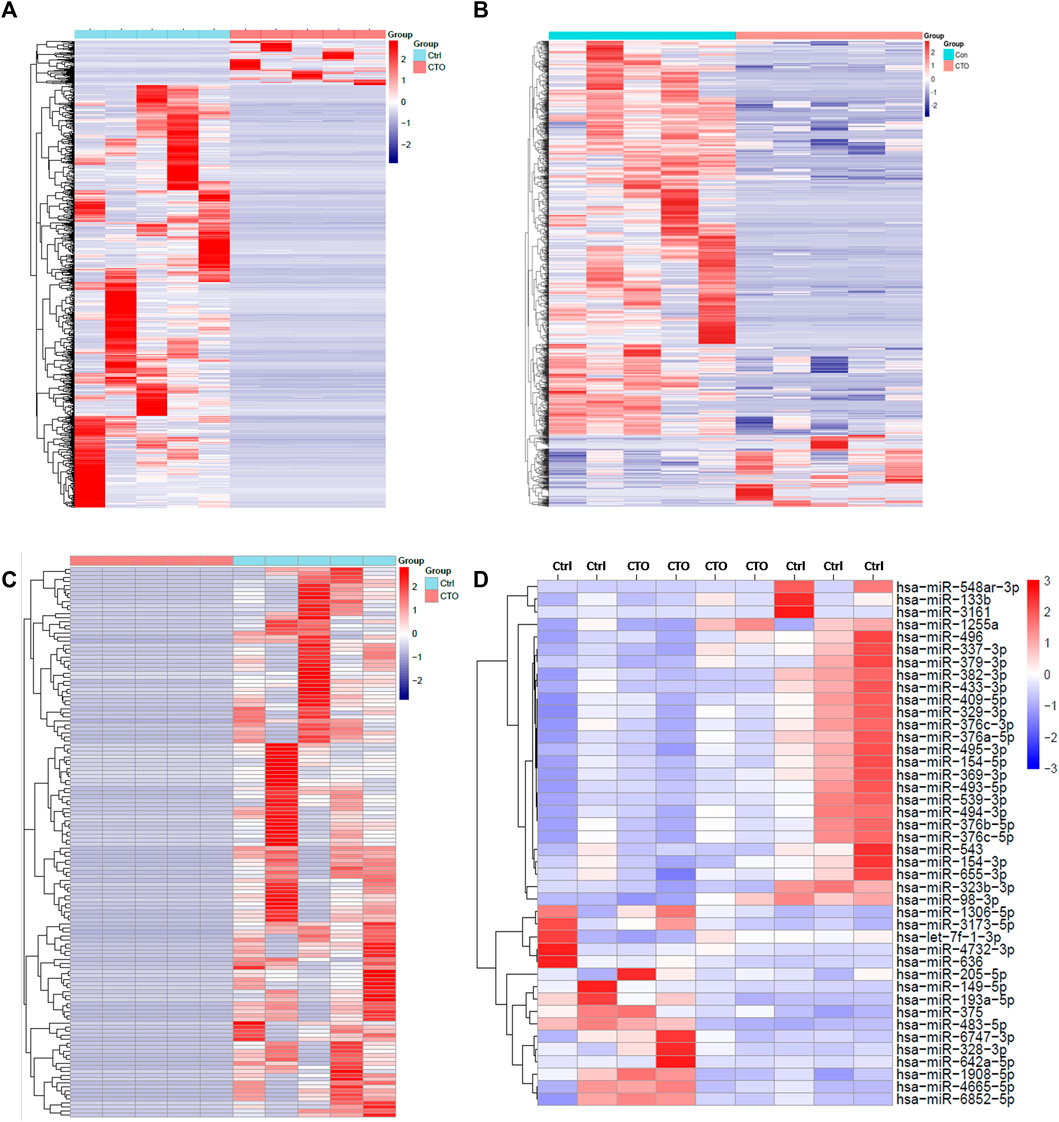
FIGURE 1. Expression profile of lncRNAs, mRNAs, circRNAs and miRNAs. Hierarchical clustering of DE lncRNAs (A), mRNAs (B), circRNA (C) and miRNAs (D) were showed in heatmaps.
Functional Annotation: Gene Ontology and Kyoto Encyclopedia of Genes and Genomes
GO and KEGG analyses were conducted on the differential expressed lncRNA, mRNA, circRNA and miRNA. For lncRNA, the top 20 GO-BP terms and top 30 KEGG terms were shown in Supplementary Figures S1, S2. For mRNA, the top 10 GO-BP terms was shown in Supplementary Figure S3 and no enriched KEGG pathways were found. For circRNA, the top 20 GO-BP terms and top 30 KEGG terms were shown in Supplementary Figures S4, S5. For miRNA, the top GO-BP terms and top KEGG terms were shown in Supplementary Figures S6, S7. We noticed that the top terms included AMPK signaling pathway, HIF-1 signaling pathway, EGFR tyrosine kinase inhibitor resistance, embryonic organ development, wound healing, MAPK signaling pathway and JAK-STAT signaling pathway, which are related to angiogenesis.
Construction of the lncRNA-miRNA-mRNA Network
RNA transcripts can effectively interact with one another based on the ceRNA hypothesis. LncRNAs can absorb miRNAs by binding to miRNAs and subsequently exhibiting a miRNA sponge function. Prediction results from bioinformatic analyses indicated that 32 lncRNAs were targeted by 13 miRNAs; nine lncRNAs potentially acted as decoys for five miRNAs. Based on the lncRNA-miRNA and miRNA-mRNA interaction pairs, a lncRNA-miRNA-mRNA network was constructed (Supplementary Figures S8). The network consisted of 46 lncRNA-miRNA relationship pairs and 199 miRNA-mRNA relationship pairs. The top GO-BP and KEGG terms of lncRNA-miRNA-mRNA network analysis were shown in Figure 2. Some angiogenesis related pathways were also observed, such as MAPK and HIF-1 signaling pathway.
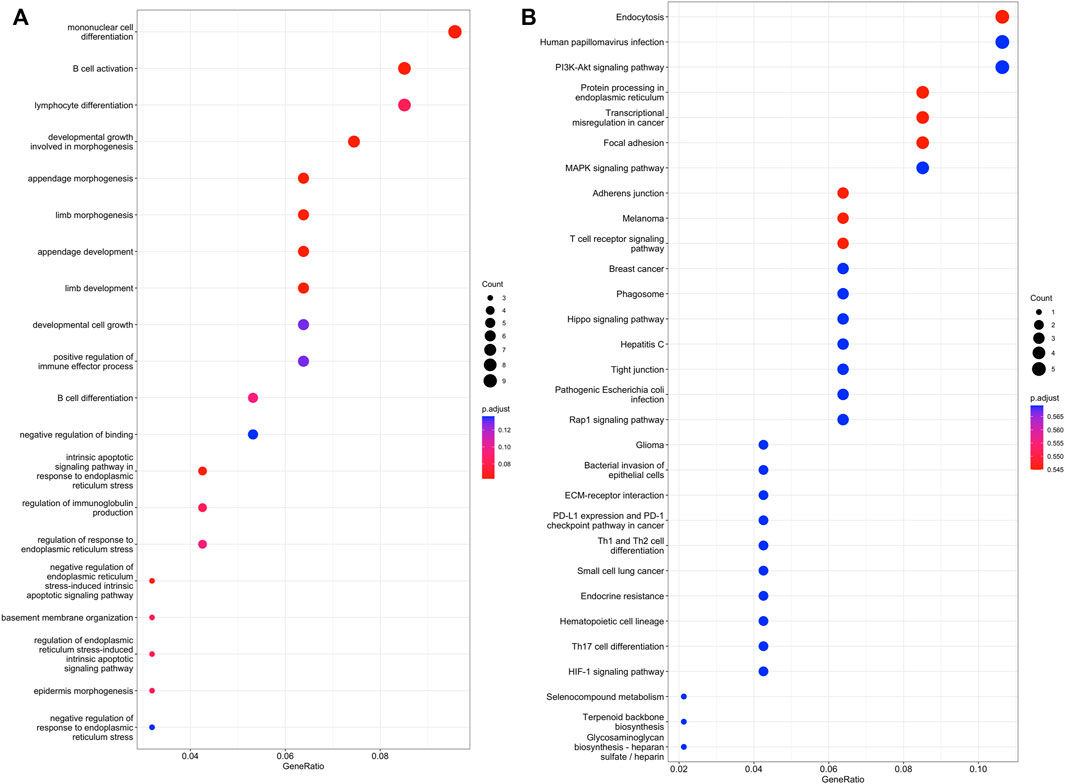
FIGURE 2. GO and KEGG pathway analysis of DE lncRNA-miRNAs and miRNA-mRNA networks. (A), the top 20 GO terms. (B), the top 30 KEGG terms.
MiRNA 494, 495 and 329 Expressions in Control and CTO Patients
After analyzing the differentially expressed miRNAs, we noticed that miRNA-494, miRNA-495 and miRNA-329 belong to the 14q32 miRNA gene cluster, which were all significantly down-regulated in CTO patients. The expression of these three miRNAs were then determined in another cohort of 68 male patients, including 22 CAD patients and 46 CTO patients. We confirmed that miRNA-494, miRNA-495 and miRNA-329 were indeed down-regulated in CTO patients (Figure 3A). Then we divided the CTO patients into two groups according to CC grade (poor CC group, CC = 0 or one; good CC group, CC = 2). MiRNA-494, miRNA-495 and miRNA-329 were found to be down-regulated in good CC group compared with poor CC group (Figure 3B). These data indicated that the expression of miRNA-494, miRNA-495 and miRNA-329 were associated with angiogenesis in CTO patients.
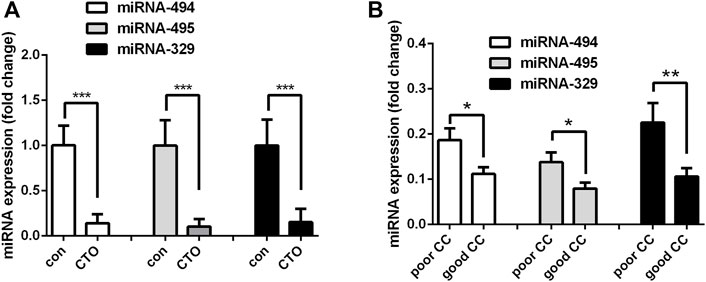
FIGURE 3. Expression of miR-494, miR-495 and miR-329 in another patient cohort. (A), Expressions of these miRNAs in patients with coronary artery disease (control group, N = 22) and chronic total occlusions (CTO group, N = 46). (B), Patients in CTO group were divided into two subgroups according to CC grade. Expression levels of miRNA-329, miRNA-494 and miRNA-495 in poor CC group (N = 17, CC = 0 or 1) and good CC group (N = 27, CC = 2). *p < 0.05, **p < 0.01, ***p < 0.001.
Analysis of the lncRNA-miRNA495-mRNA Network
Previous studies found miRNA-329 and miRNA-494 suppresses angiogenesis by targeting CD146 (Wang et al., 2013) and BMPER (Esser et al., 2017), respectively. Whether and how miRNA-495 inhibits angiogenesis of endothelial cells remains unclear. Then we focused on miRNA-495 and constructed the analysis of lncRNA-miRNA495-mRNA network. As shown in Figure 4, the results revealed that miRNA-495 targeted 3 DE lncRNA sponges (NONHSAG008675, NONHSAG020957 and NONHSAG010989), 4 DE lncRNA targets (NONHSAT079547.2, NONHSAT081776.2, NONHSAT148555.1 and NONHSAT150928.1) and 2 DE mRNA targets (RAD54L2 and ZC3H4). These data indicated that miRNA-495, together with the targeted lncRNAs and mRNAs, might play a regulatory role in angiogenesis. Further experiments investigating the underlying mechanisms are of potential value.
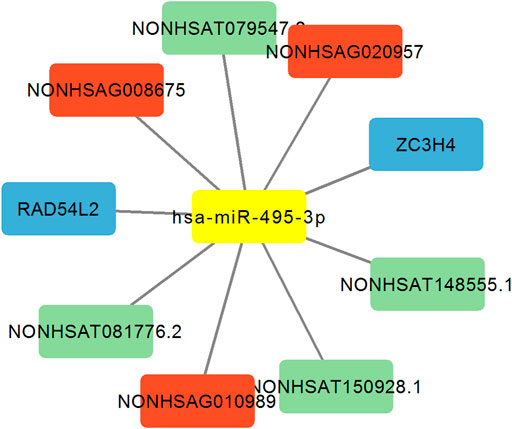
FIGURE 4. Analysis of the lncRNA-miRNA495-mRNA network. Red indicates lncRNA sponge of miR-495. Blue indicates mRNA target of miR-495. Green indicates lncRNA target of miR-495.
Discussion
The specific characteristic of CTO is collateral circulation, which attracted lots of attention in CTO research. Clinical doctors focused on whether the collateral circulation is sufficient to provide blood supply and can predict clinical prognosis. Basic researchers were interested in how it takes shape and what the impact factors are. In this study, we evaluated the expression profiles of mRANs and non-coding RNAs, including lncRNAs, cirRNAs and miRNAs, in the plasma of CTO patients. We also conducted a network between these RNAs and proposed several potential targets involving into angiogenesis. To our knowledge, this is the first bioinformatics study to comprehensively investigate the RNA expression profile and their network in CTO patients.
lncRNAs are a class of non-coding RNAs that are more than 200 nt in length. In addition to being directly involved in the regulation of gene expression, lncRNA can also function as a competing endogenous RNA, which competes with other RNA transcripts for the same miRNA, resulting in interactions and subsequent regulation. As discussed in a previous review (Zhao et al., 2020), lncRNA-miRNA interaction play a role in angiogenesis through several signaling pathways, including VEGF, Notch, PI3K/AKT, JAK-STAT and ERK (Li et al., 2017; Gao et al., 2018; Wang et al., 2018; Yin et al., 2018; Moradi et al., 2019). In our study, we noticed that a previous reported angiogenesis related lncRNA, TUG1 (ENST0000051907), was differently expressed in CTO patients compared with control patients (not shown in Table). And also, GO and KEGG analyses revealed some angiogenesis related pathways, such as HIF-1 and EGFR tyrosine kinase inhibitor resistance. When focusing on miRNA-495 targeted lncRNAs, we found some new lncRNAs, which are potentially involved into angiogenesis of CTO disease. Future studies focusing on these new lncRNAs are needed.
CircRNAs are a class of novel RNAs that have a special covalent loop structure without a 5′ cap and 3’ tail. The formation mechanism of circRNAs is not well understood. Although circRNAs are less studied compared with lncRNAs or miRNAs, some recent studies have shown that circRNA has a role in the regulation of angiogenesis related diseases, such as atherosclerosis, myocardial hypertrophy and hypertension (Boeckel et al., 2015; Wang et al., 2016; Bao et al., 2018). In our study, the GO and KEGG analyses showed some angiogenesis related pathways, including MAPK and JAK-STAT signaling pathway. Then we used starBase (https://starbase.sysu.edu.cn/) to predict miRNA495-circRNA interactions. Among the top 10 significantly down-regulated circRNAs in Table 4, circRNA.2642 (DNAJC3), circRNA.10222 (PHC3) and circRNA.9677 (MBOAT2) were the targets of miRNA-495. These circRNAs are worthy to be investigated in future studies.
The most deeply studied non-coding RNAs are miRNAs. As discussed in a recent review, miRNAs can be both anti-angiogenic and proangiogenic (Kir et al., 2018). Among the differently expressed miRNAs between control and CTO patients, we focused on a 14q32 miRNA gene cluster, including miRNA-494, miRNA-495 and miRNA-329. In a previous study, Gene-Specific Oligonucleotides (GSO) were used to systemically inhibit these miRNAs in hind limb ischemia models and improved recovery of perfusion was observed (Welten et al., 2014). We found that down-regulation of these three miRNAs were associated with well developed collateral circulation in CTO patients. We further investigated the mechanism of how miRNA-495 regulates angiogenesis in both in vitro and in vivo study (data not shown). The lncRNA-miRNA495-mRNA network is also of our great interest. In this current study, we showed several lncRNAs and mRNAs targets of miRNA495, which are worthy of more investigation in future studies.
Conclusion
This is the first bioinformatics study to comprehensively investigate the RNA expression profile and their network in CTO patients. The results showed that lncRNA-miRNA-mRNA network might play a critical role in angiogenesis in CTO patients.
Data Availability Statement
The datasets presented in this study can be found in online repositories. The names of the repository/repositories and accession number(s) can be found below: https://ncbi.nlm.nih.gov/sra; PRJNA799206.
Ethics Statement
The studies involving human participants were reviewed and approved by the Medical ethics committee of Zhongshan Hospital. The patients/participants provided their written informed consent to participate in this study.
Author Contributions
WG designed and performed experiments, analyzed the data, and wrote the manuscript. JZ performed experiments and analyzed the data. RW performed experiments. JY and JG designed and supervised the study.
Funding
This study was supported by the National Natural Science Foundation of China (No. 81900305 to WG, and No. 81870200 to JY).
Conflict of Interest
The authors declare that the research was conducted in the absence of any commercial or financial relationships that could be construed as a potential conflict of interest.
Publisher’s Note
All claims expressed in this article are solely those of the authors and do not necessarily represent those of their affiliated organizations, or those of the publisher, the editors and the reviewers. Any product that may be evaluated in this article, or claim that may be made by its manufacturer, is not guaranteed or endorsed by the publisher.
Supplementary Material
The Supplementary Material for this article can be found online at: https://www.frontiersin.org/articles/10.3389/fgene.2022.855549/full#supplementary-material
References
Bao, X., Zheng, S., Mao, S., Gu, T., Liu, S., Sun, J., et al. (2018). A Potential Risk Factor of Essential Hypertension in Case-Control Study: Circular RNA Hsa_circ_0037911. Biochem. Biophysical Res. Commun. 498 (4), 789–794. doi:10.1016/j.bbrc.2018.03.059
Boeckel, J.-N., Jaé, N., Heumüller, A. W., Chen, W., Boon, R. A., Stellos, K., et al. (2015). Identification and Characterization of Hypoxia-Regulated Endothelial Circular RNA. Circ. Res. 117 (10), 884–890. doi:10.1161/circresaha.115.306319
Chistiakov, D. A., Orekhov, A. N., and Bobryshev, Y. V. (2016). Cardiac-specific miRNA in Cardiogenesis, Heart Function, and Cardiac Pathology (With Focus on Myocardial Infarction). J. Mol. Cell Cardiol. 94, 107–121. doi:10.1016/j.yjmcc.2016.03.015
Choi, J.-H., Chang, S.-A., Choi, J.-O., Song, Y. B., Hahn, J.-Y., Choi, S. H., et al. (2013). Frequency of Myocardial Infarction and its Relationship to Angiographic Collateral Flow in Territories Supplied by Chronically Occluded Coronary Arteries. Circulation 127 (6), 703–709. doi:10.1161/circulationaha.112.092353
Christofferson, R. D., Lehmann, K. G., Martin, G. V., Every, N., Caldwell, J. H., and Kapadia, S. R. (2005). Effect of Chronic Total Coronary Occlusion on Treatment Strategy. Am. J. Cardiol. 95 (9), 1088–1091. doi:10.1016/j.amjcard.2004.12.065
Cui, Y., Chen, H., Xi, R., Cui, H., Zhao, Y., Xu, E., et al. (2020). Whole-genome Sequencing of 508 Patients Identifies Key Molecular Features Associated with Poor Prognosis in Esophageal Squamous Cell Carcinoma. Cell Res 30 (10), 902–913. doi:10.1038/s41422-020-0333-6
Economou, E. K., Oikonomou, E., Siasos, G., Papageorgiou, N., Tsalamandris, S., Mourouzis, K., et al. (2015). The Role of microRNAs in Coronary Artery Disease: From Pathophysiology to Diagnosis and Treatment. Atherosclerosis 241 (2), 624–633. doi:10.1016/j.atherosclerosis.2015.06.037
Esser, J. S., Saretzki, E., Pankratz, F., Engert, B., Grundmann, S., Bode, C., et al. (2017). Bone Morphogenetic Protein 4 Regulates microRNAs miR-494 and miR-126-5p in Control of Endothelial Cell Function in Angiogenesis. Thromb. Haemost. 117 (4), 734–749. doi:10.1160/TH16-08-0643
Gao, Y., Yu, H., Liu, Y., Liu, X., Zheng, J., Ma, J., et al. (2018). Long Non-coding RNA HOXA-AS2 Regulates Malignant Glioma Behaviors and Vasculogenic Mimicry Formation via the MiR-373/EGFR Axis. Cell Physiol Biochem 45 (1), 131–147. doi:10.1159/000486253
Kir, D., Schnettler, E., Modi, S., and Ramakrishnan, S. (2018). Regulation of Angiogenesis by microRNAs in Cardiovascular Diseases. Angiogenesis 21 (4), 699–710. doi:10.1007/s10456-018-9632-7
Li, B., Brady, S. W., Ma, X., Shen, S., Zhang, Y., Li, Y., et al. (2020). Therapy-induced Mutations Drive the Genomic Landscape of Relapsed Acute Lymphoblastic Leukemia. Blood 135 (1), 41–55. doi:10.1182/blood.2019002220
Li, L., Wang, M., Mei, Z., Cao, W., Yang, Y., Wang, Y., et al. (2017). lncRNAs HIF1A-AS2 Facilitates the Up-Regulation of HIF-1α by Sponging to miR-153-3p, Whereby Promoting Angiogenesis in HUVECs in Hypoxia. Biomed. Pharmacother. 96, 165–172. doi:10.1016/j.biopha.2017.09.113
Liu, Y., Yang, Y., Wang, Z., Fu, X., Chu, X.-m., Li, Y., et al. (2020). Insights into the Regulatory Role of circRNA in Angiogenesis and Clinical Implications. Atherosclerosis 298, 14–26. doi:10.1016/j.atherosclerosis.2020.02.017
Meisel, S. R., Frimerman, A., Blondheim, D. S., Shotan, A., Asif, A., Shani, J., et al. (2013). Relation of the Systemic Blood Pressure to the Collateral Pressure Distal to an Infarct-Related Coronary Artery Occlusion during Acute Myocardial Infarction. Am. J. Cardiol. 111 (3), 319–323. doi:10.1016/j.amjcard.2012.10.005
Moradi, M. T., Fallahi, H., and Rahimi, Z. (2019). Interaction of Long Noncoding RNA MEG3 with miRNAs: A Reciprocal Regulation. J. Cel Biochem 120 (3), 3339–3352. doi:10.1002/jcb.27604
Sachdeva, R., Agrawal, M., Flynn, S. E., Werner, G. S., and Uretsky, B. F. (2014). The Myocardium Supplied by a Chronic Total Occlusion Is a Persistently Ischemic Zone. Cathet. Cardiovasc. Intervent. 83 (1), 9–16. doi:10.1002/ccd.25001
Srinivas, V. S., Brooks, M. M., Detre, K. M., King, S. B., Jacobs, A. K., Johnston, J., et al. (2002). Contemporary Percutaneous Coronary Intervention versus Balloon Angioplasty for Multivessel Coronary Artery Disease: a Comparison of the National Heart, Lung and Blood Institute Dynamic Registry and the Bypass Angioplasty Revascularization Investigation (BARI) Study. Circulation 106 (13), 1627–1633. doi:10.1161/01.cir.0000031570.27023.79
Wang, K., Long, B., Liu, F., Wang, J.-X., Liu, C.-Y., Zhao, B., et al. (2016). A Circular RNA Protects the Heart from Pathological Hypertrophy and Heart Failure by Targeting miR-223. Eur. Heart J. 37 (33), 2602–2611. doi:10.1093/eurheartj/ehv713
Wang, P., Luo, Y., Duan, H., Xing, S., Zhang, J., Lu, D., et al. (2013). MicroRNA 329 Suppresses Angiogenesis by Targeting CD146. Mol. Cel Biol 33 (18), 3689–3699. doi:10.1128/mcb.00343-13
Wang, Z., Wang, R., Wang, K., and Liu, X. (2018). Upregulated Long Noncoding RNA Snhg1 Promotes the Angiogenesis of Brain Microvascular Endothelial Cells after Oxygen-Glucose Deprivation Treatment by Targeting miR-199a. Can. J. Physiol. Pharmacol. 96 (9), 909–915. doi:10.1139/cjpp-2018-0107
Welten, S. M. J., Bastiaansen, A. J. N. M., de Jong, R. C. M., de Vries, M. R., Peters, E. A. B., Boonstra, M. C., et al. (2014). Inhibition of 14q32 MicroRNAs miR-329, miR-487b, miR-494, and miR-495 Increases Neovascularization and Blood Flow Recovery after Ischemia. Circ. Res. 115 (8), 696–708. doi:10.1161/circresaha.114.304747
Werner, G. S., Surber, R., Kuethe, F., Emig, U., Schwarz, G., Bahrmann, P., et al. (2005). Collaterals and the Recovery of Left Ventricular Function after Recanalization of a Chronic Total Coronary Occlusion. Am. Heart J. 149 (1), 129–137. doi:10.1016/j.ahj.2004.04.042
Werner, G. (2014). The Role of Coronary Collaterals in Chronic Total Occlusions. Ccr 10 (1), 57–64. doi:10.2174/1573403x10666140311123814
Windecker, S., Windecker, S., Kolh, P., Alfonso, F., Collet, J. P., Cremer, J., et al. (2014). 2014 ESC/EACTS Guidelines on Myocardial Revascularization: The Task Force on Myocardial Revascularization of the European Society of Cardiology (ESC) and the European Association for Cardio-Thoracic Surgery (EACTS)Developed with the Special Contribution of the European Association of Percutaneous Cardiovascular Interventions (EAPCI). Eur. Heart J. 35 (37), 2541–2619. doi:10.1093/eurheartj/ehu278
Yin, D., Fu, C., and Sun, D. (2018). Silence of lncRNA UCA1 Represses the Growth and Tube Formation of Human Microvascular Endothelial Cells through miR-195. Cel Physiol Biochem 49 (4), 1499–1511. doi:10.1159/000493454
Zhao, Z., Sun, W., Guo, Z., Zhang, J., Yu, H., and Liu, B. (2020). Mechanisms of lncRNA/microRNA Interactions in Angiogenesis. Life Sci. 254, 116900. doi:10.1016/j.lfs.2019.116900
Keywords: coronary artery disease, chronic total occlusion, angiogenesis, lncRNA, mRNA, miRNA
Citation: Gao W, Zhang J, Wu R, Yuan J and Ge J (2022) Integrated Analysis of Angiogenesis Related lncRNA-miRNA-mRNA in Patients With Coronary Chronic Total Occlusion Disease. Front. Genet. 13:855549. doi: 10.3389/fgene.2022.855549
Received: 15 January 2022; Accepted: 06 April 2022;
Published: 25 April 2022.
Edited by:
Gary S. Stein, University of Vermont, United StatesReviewed by:
Mahshid Malakootian, Iran University of Medical Sciences, IranXue-Qiang Wang, Shanghai University of Sport, China
Copyright © 2022 Gao, Zhang, Wu, Yuan and Ge. This is an open-access article distributed under the terms of the Creative Commons Attribution License (CC BY). The use, distribution or reproduction in other forums is permitted, provided the original author(s) and the copyright owner(s) are credited and that the original publication in this journal is cited, in accordance with accepted academic practice. No use, distribution or reproduction is permitted which does not comply with these terms.
*Correspondence: Jie Yuan, yuan.jie@zs-hospital.sh.cn; Junbo Ge, jbge@zs-hospital.sh.cn
†These authors have contributed equally to this work