- 1Department of Internal Medicine, The Second Affiliated Hospital of Kunming Medical University, Kunming, China
- 2Department of Internal Medicine, Yunnan Fuwai Cardiovascular Hospital, Kunming, China
- 3Medical School, Kunming Medical University, Kunming, China
- 4Department of Internal Medicine, The Affiliated Hospital of Yunnan University, Kunming, China
Background: Osteoporosis (OP) is a serious and common bone metabolic disease with bone mass loss and bone microarchitectural deterioration. The OSTEOWONDER capsule is clinically used to treat OP. However, the potential regulatory mechanism of the OSTEOWONDER capsule in treatment of OP remains largely unknown.
Methods: The bioactive compounds of herbs and their targets were identified using the Traditional Chinese Medicine Systems Pharmacology Database and Analysis Platform (TCMSP) database. The speculative targets of OP were screened out based on GeneCards, DisGeNET, and Online Mendelian Inheritance in Man (OMIM) databases. The gene modules and hub genes of OP were identified using a weighted gene co-expression network analysis (WGCNA). Then, an herb-compound-target network was constructed based on the above analyses. The biological function of targets was subsequently investigated, and a protein–protein interaction (PPI) network was constructed to identify hub targets of OP. Finally, molecular docking was performed to explore the interaction between compounds and targets.
Results: A total of 148 compounds of eight herbs and the corresponding 273 targets were identified based on the TCMSP database. A total of 4,929 targets of OP were obtained based on GeneCards, DisGeNET, and OMIM databases. In addition, six gene modules and 4,235 hub genes of OP were screened out based on WGCNA. Generally, an herb-compound-target network, including eight herbs, 84 compounds, and 58 targets, was constructed to investigate the therapeutic mechanism of the OSTEOWONDER capsule for OP. The biofunction analysis indicated 58 targets mainly associated with the bone metabolism, stimulation response, and immune response. EGFR, HIF1A, MAPK8, IL6, and PPARG were identified as the hub therapeutic targets in OP. Moreover, the interaction between EGFR, HIF1A, MAPK8, IL6, PPARG, and the corresponding compounds (quercetin and nobiletin) was analyzed using molecular docking.
Conclusion: Our finding discovered the possible therapeutic mechanisms of the OSTEOWONDER capsule and supplied the potential therapeutic targets for OP.
Introduction
Osteoporosis (OP) is a systemic skeletal disease that is defined by a low bone mineral density (BMD) and T score, which is a standard deviation (SD) that represents the measured value of BMD varying from average or mean BMD of a healthy young adult (Johnston and Dagar, 2020). OP characterizes by a low bone mass and microarchitectural deterioration in the bone tissue and eventually induces bone fragility and fracture (Ensrud and Crandall, 2017). OP is a serious health problem that affects 200 million people worldwide and threatens 60.2 million older adults in China (Cotts and Cifu, 2018; Zeng et al., 2019). Unfortunately, OP not only has high incidence in the aging population but also majorly affects postmenopausal women, with approximately 10% of the world population and 30% of postmenopausal women suffering from OP (Alibasic et al., 2013; Zhu and Prince, 2015; Brown, 2017). The emergence of OP involves a number of factors, including genetic and environmental factors (Bijelic et al., 2016) as well as lifestyle, which has been recently demonstrated as an important factor contributing to OP in postmenopausal women (Zhu and Prince, 2015). In the past decades, several strategies have been used for prevention and treatment of OP, including anti-osteoporotic drugs (hormonal therapy, bisphosphonates, denosumab), anabolic agents (teriparatide), dual-action drugs (romosozumab), and strontium ranelate (Gatti and Fassio, 2019). However, although many therapeutic strategies have been used in clinics, many high-risk individuals remain without adequate treatment. The restricted and continued long treatment times usually cause treatment failure (Compston et al., 2019). Therefore, the challenges for the future are improving comprehensive nursing for OP patients and exploring more safe, simple, and effective medicines for OP treatment.
In recent years, traditional Chinese medicine (TCM) has been widely used for intractable disease treatment and has emerged with advantages of safety and effectiveness (Wang et al., 2018; Liu et al., 2020). For example, the combination of TCM with hepatic targeted drug delivery systems has been used for liver disease treatment (Ma et al., 2019). In addition, the TCM formula Lily Bulb and Rehmannia Decoction has been used for depression treatment (Chi et al., 2019). TCM formulas also show a good efficiency in treatment of Parkinson’s disease (Han et al., 2017), diabetes mellitus (Wang et al., 2020a), and idiopathic pulmonary fibrosis (Zhang et al., 2021). Moreover, TCM has been used as adjunctive therapy for improving the clinical outcome for cancer patients (Liao et al., 2017; Xiang et al., 2019; Zhai et al., 2019; Wang et al., 2020b). Some Chinese herbs and TCM formulas, such as Rehmanniae Radix (Liu et al., 2017a), Bushen-Jianpi-Huoxue decoction (Zhang et al., 2017), QiangGuYin (Shi et al., 2017), and Xianlinggubao capsules (Bao et al., 2020), have exhibited efficiency against OP.
The OSTEOWONDER capsule, also known as Heng-Gu-Gu-Shang-Yu-He-Ji and osteoking, is a TCM that originates from the Yunnan Province of China (Hu et al., 2005; Zhao et al., 2006) and has been approved by the Chinese State Food and Drug Administration in 2002 (Ling et al., 2021). The OSTEOWONDER capsule is comprised of Chenpi (Citrus Reticulata Reticulatae), Huangqi (Hedysarum Multijugum Maxim.), Renshen (Panax Ginseng C. A. Mey.), Honghua (Carthamus tinctorius L.), Sanqi (Radix Notoginseng), Duzhong (Eucommiae Cortex), Yangjinhua (Daturae Flos), Zuandifeng (Schizophragma integrifolium), and Biejia (Trionycis carapace). Previous studies have demonstrated that osteoking exerts anti-OP effects in OP rat models (Sun et al., 2020a; Sun et al., 2020b). In addition, it has been found that osteoking anti-OP may be through reduction of reactive oxygen species (Qin et al., 2019) or regulating rat bone marrow mesenchymal stem cell osteogenic and adipogenic differentiation (Yu et al., 2019). Nevertheless, the anti-osteoporotic pharmacological mechanism of osteoking remains unclear.
In the present study, a network pharmacology and bioinformatics analysis based on Gene Expression Omnibus (GEO) was utilized to explore the potential mechanism of the OSTEOWONDER capsule in the treatment of OP. Our finding provided the theoretical basis and evidence for OP treatment by the OSTEOWONDER capsule.
Materials and Methods
Screening the Bioactive Compounds and the Corresponding Targets From the OSTEOWONDER Capsule
The active compounds of the OSTEOWONDER capsule were derived from the Traditional Chinese Medicine Systems Pharmacology Database and Analysis Platform (TCMSP, https://tcmspw.com/index.php), which is a public and special traditional Chinese medicine system pharmacology database and analysis platform (Ru et al., 2014). The potential bioactive compounds of eight Chinese herbs, Chenpi, Huangqi, Renshen, Honghua, Sanqi, Duzhong, Yangjinhua, and Zuandifeng (Guang, 2009), were identified according to the oral bioavailability (OB) value ≥30% and drug-likeness (DL) ≥ 0.18. OB refers to the speed and extent of drug entering the circulatory system of the body. In addition, the OB value is represented as the percentage of the oral dose of drug entering the circulatory system. DL is a term that has been used to rationalize how physicochemical properties influence the molecular behavior in vivo. In addition, the corresponding targets of the bioactive compounds of the OSTEOWONDER capsule were identified based on the TCMSP database.
Identification of the Targets of Osteoporosis
The name of osteoporosis was queried in GeneCards (https://www.genecards.org/) (Stelzer et al., 2016), the DisGeNET database (https://www.disgenet.org/home/) (Piñero et al., 2017; Piñero et al., 2020), and the Online Mendelian Inheritance in Man (OMIM) database (https://omim.org/), and the potential targets of OP were obtained.
Data Processing and Weighted Gene Co-expression Network Analysis
Gene expression data and the corresponding clinical information of the GSE56815 dataset was downloaded from the Gene Expression Omnibus (GEO, https://www.ncbi.nlm.nih.gov/gds), which includes 40 OP and non-OP female blood monocyte samples. The platform of the GSE56815 dataset is the [HG-U133A] Affymetrix Human Genome U133A Array.
WGCNA is an efficient method to identify the clusters of highly correlated genes and to summarize such clusters using the module eigengene or an intramodular hub gene, so this is widely used to identify candidate biomarkers or therapeutic targets and analyze the connection between modules and the specific traits and phenotypes (Liu et al., 2017b; Cai et al., 2020). A WGCNA R package was used to perform the weighted correlation network construction and analysis (Langfelder and Horvath, 2008). First, all samples were clustered according to the gene co-expression similarity and the outliers were removed. Second, the power function “pickSoftThreshold” was used to screen the power parameter using a gradient method, which ranges from 1 to 20. An optimal soft threshold of 6 was selected as it met the degree of independence of 0.85 with the minimum power value. Finally, the adjacency matrix was transformed into the topological overlap matrix (TOM). Genes were assigned into different gene modules according to the TOM-related dissimilarity measure and the soft-thresholding setting. In addition, the numbers of gene modules were obtained according to the dissimilarity and the criterion of dynamic tree cutting with the minimal module size as 30 genes.
Identification of Key Modules Correlating to Clinical Traits
After the modules were identified, the correlation between module eigengenes (ME) and clinical traits was investigated to identify the interest modules, which were significantly associated with clinical traits.
The modules significantly correlated with the clinical traits were identified as the OP-related modules in this study. Gene significance (GS) represents the correlation between gene expression and each trait, and module membership (MM) represents the correlation between gene expression and each module eigengene. Therefore, the correlation of module-clinical traits was confirmed by correlation between GS and MM. A correlation of module-clinical traits more than 0.3 served as the threshold to identify the interest modules in this study.
Construction of the Herb–Compound–Target Network
The common genes were obtained by overlapping the targets of active compounds, targets of OP, and hub genes from key modules. Subsequently, a drug–bioactive compound–target network was constructed according to the eight herbs, 84 bioactive compounds, and 58 common genes using Cytoscape software version 3.7.2.
GO and KEGG Pathway Enrichment Analysis
GO annotation and KEGG pathway enrichment analysis were performed using the clusterProfiler R package with adjusted-P value <0.05. GO annotation includes biological process (BP), molecular functions (MF), and cellular components (CC) terms.
Protein–Protein Interaction Network Construction
In order to identify the interaction between the common genes, a PPI network was constructed using STRING (https://string-db.org/) with the Confidence of 0.4 and then visualized using Cytoscape version 3.7.2. Moreover, the potential hub targets and key sub networks were identified using “cytohbba” in Cytoscape according the Clustering Coefficient algorithm (Peng et al., 2021).
Validation of the Expression of the Hub Targets
We explored the expression levels of five hub targets in different tissues based on Human Protein Atlas (HPA, https://www.proteinatlas.org/). In addition, we also investigated the correlation between the expression levels of five hub targets and clinical traits of OP using the Kruskal–Wallis test based on the GSE56815 dataset.
Molecular Docking
Molecular docking is an important method which is used for drug design and discovery based on the interaction between the drug molecule and receptor. Molecular docking can be used to identify novel compounds and predict the ligand–target interaction at a molecular level or delineate structure–activity relationships (Pinzi and Rastelli, 2019). The bases of the molecular interaction and stable combination between molecules are spatial and energy matchings. Calculation of geometric matching was performed by grid computing and fragment growth, and energy calculation was performed by simulated annealing and genetic algorithms. AutoDock Vina is a common open-source and free software tool which is widely used for molecular docking. AutoDock Vina supports the AutoDock scoring function, simultaneous docking of multiple ligands, and a batch mode for docking a large number of ligands (Nguyen et al., 2020; Eberhardt et al., 2021). Here, we first obtained the protein structure of five hub proteins from the PDB database, then removed the existing water molecules and small molecules, and subsequently hydrogenated and calculated charges using AutoDock Tools. Second, we downloaded the structures of bioactive compounds and then checked the charge balance and rotatable bonds of small molecules. Third, the range of butt boxes was confirmed according to the receptor active center. Fourth, the semi-flexible docking of receptors and ligands was performed using AutoDock Vina by accepting the default settings, and the docking structures with the lowest binding free energy were output. The absolute values of negative values indicate the greater energy values. Finally, the docking structures were visualized and beautified using PyMol software (Yuan et al., 2016).
Results
Screening of Active Compounds and Potential Targets of the OSTEOWONDER Capsule
Considering the therapeutic effects of the OSTEOWONDER capsule on OP, we employed the system network pharmacology and WGCNA to explore the potential molecular mechanism of the OSTEOWONDER capsule in OP. The workflow of this study is shown in Figure 1. First of all, according to TCMSP database retrieval, a total of 917 compounds corresponding to seven herbs were obtained from the TCMSP database (Table 1). Thereafter, 148 bioactive compounds were screened based on the parameter of OB ≥ 30% and DL ≥ 0.18 (Table 1, Supplementary Table S1). In addition, we also predicated the targets of these compounds based on TCMSP, and we obtained a total of 1,153 targets of compounds (Table 2). Of these targets, 273 independent targets were obtained by removing duplication (Supplementary Tables S2, S3).
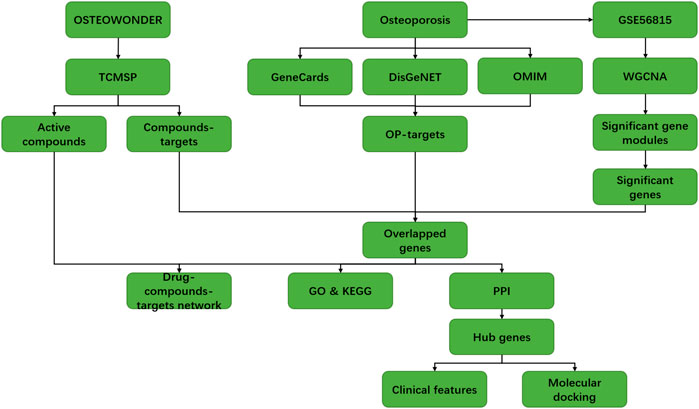
FIGURE 1. Flow diagram of the present study to investigate the potential mechanism of the OSTEOWONDER capsule in the OP treatment.
Identification of the Potential Targets of Osteoporosis
Next, we screened OP-related genes based on the online databases. Unsurprisingly, a total of 4,613 targets of OP were identified from the GeneCards database (Supplementary Table S4), 1,098 targets of OP were identified from the DisGeNET database (Supplementary Table S5), and 197 targets of OP were screened from the OMIM database (Supplementary Table S6). A total of 4,929 targets of OP were obtained based on GeneCards, DisGeNET, and OMIM databases (Supplementary Table S7).
Construction of WGCNA
In order to identify the comprehensive regulatory networks for OP, we employed a WGCNA to determine a network correlated to OP. In this study, a total of 13,230 genes in 80 samples were used to construct the co-expression network. Moreover, the clustering results of samples are shown as Figures 2A,B; these results indicated the high quality of clustering, and therefore, no outliers were removed in these samples. A soft-threshold power of 6 was used to obtain the approximate scale-free topology fit index (signed R2) > 0.85 and the lowest power (Figure 2C). As a result, 13,230 genes were clustered into a total of 23 modules with the minimal module size as 30 genes using the average linkage hierarchical clustering algorithm (Figure 2D, Supplementary Table S8).
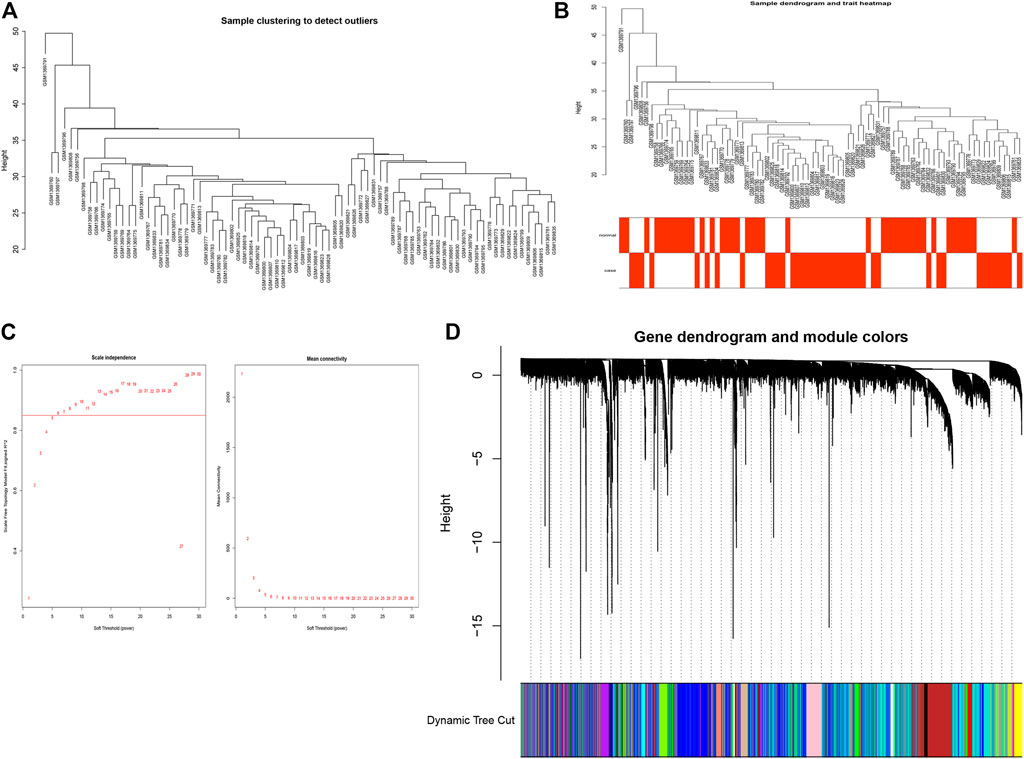
FIGURE 2. Construction of WGCNA. (A) Clustering dendrogram of samples (B) Clustering dendrogram of samples with trait heatmap. (C) Analysis of the scale-free index for various soft-threshold powers (β). Left panel, X-axis represents a function of soft-threshold power, and Y-axis represents the scale-free fit index. Right panel, X-axis represents a function of soft-threshold power, and Y-axis represents the mean connectivity (degree). (D) Clustering dendrogram of genes based on the measurement of dissimilarity (1-TOM).
Identification of the Hub Modules and Genes for OP via WGCNA
After construction of the WGCNA, we further explored the interaction among these modules. As shown in Figure 3A, the eigengene dendrogram and the eigengene adjacency heatmap indicated that 23 modules could be divided into several subgroups, suggesting the differences of correlation between different modules. Subsequently, we detected the correlation between modules and clinical traits according to the module-trait association analysis. Focusing on the case trait (i.e., OP), a total of six modules, including the blue module (|cor| > 0.3 and p < 0.05), darkturquoise module (|cor| > 0.39 and p < 0.05), grey60 module (|cor| > 0.34 and p < 0.05), lightgreen module (|cor| > 0.38 and p < 0.05), red module (|cor| > 0.39 and p < 0.05), and salmon module (|cor| >0.47 and p < 0.05), were identified to have the highest association with OP (Figure 3B). Moreover, a total of 3,410, 41, 94, 66, 524, and 118 genes were obtained from blue, darkturquoise, grey60, lightgreen, red, and salmon modules, respectively (Supplementary Table S9). Therefore, a total of 4,235 hub genes were identified based on WGCNA.
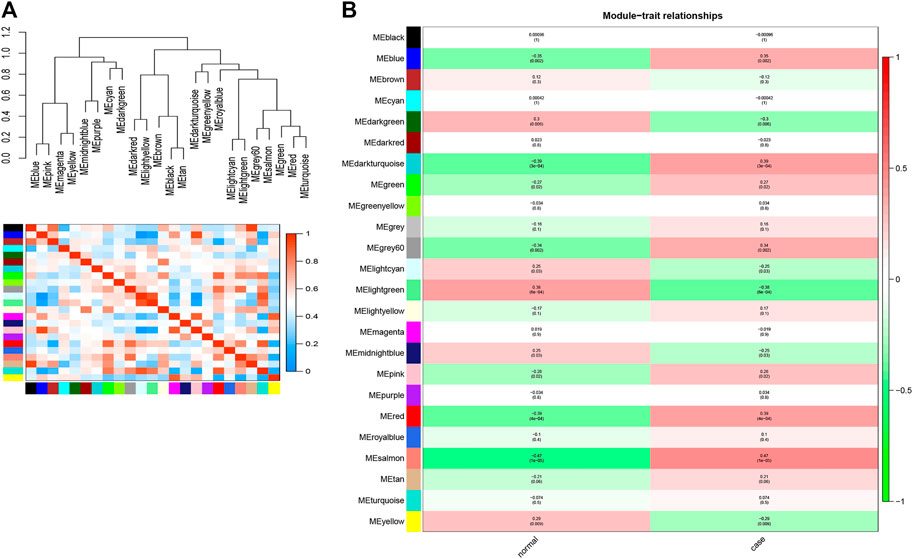
FIGURE 3. Identification of the hub modules and genes for OP via WGCNA. (A) Eigengene dendrogram and the heatmap of eigengene adjacency. (B) Heatmap of the correlation between module eigengenes and clinical traits of OP.
Construction of the Herb–Compound–Target Network
Generally, 58 potential targets of OP were identified by overlapping the 273 targets of compounds, 4,929 targets of OP, and 4235 OP-related hub genes (Figure 4A; Supplementary Table S10). To further investigate the effectiveness of the OSTEOWONDER capsule to prevent OP, which depends on the synergistic effects of compounds and their targets, an herb–compound–target network was constructed in this study based on eight herb ingredients, 84 bioactive compounds, and 58 common targets (Figure 4B, Supplementary Table S11). The network included 150 nodes (eight herb ingredients, 84 bioactive compounds, and 58 common targets) and 422 edges.
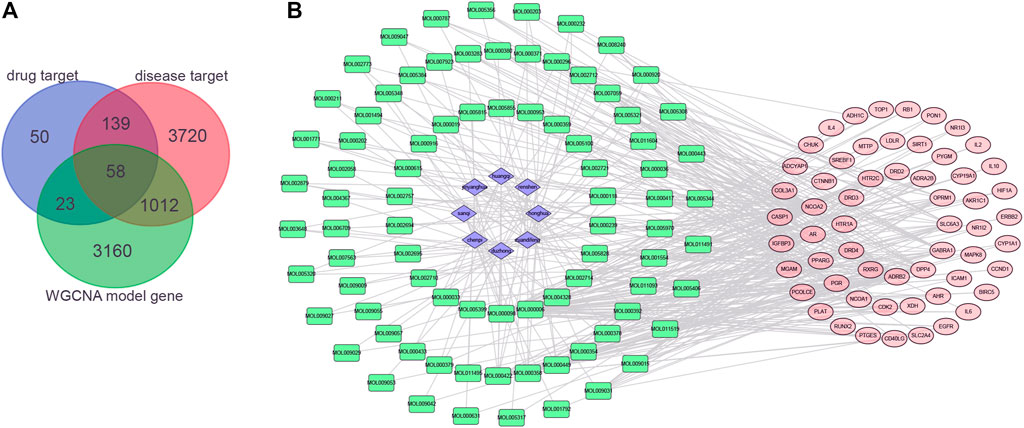
FIGURE 4. Construction of the herb–compound–target network. (A) Venn plot indicates the common targets among drug targets from the TCMSP database; targets of OP from GeneCards, DisGeNET, and OMIM databases; and hub genes from WGCNA. (B) Herb–compound–targets network. Blue rhombus represents herbs, green rectangle represents compounds, and red ellipse represents targets of OP.
Biofunction Analysis of Targets
To further investigate the potential mechanism of the OSTEOWONDER capsule for OP, GO and KEGG enrichment analyses were conducted based on 58 targets. We found these genes enriched in 778 BP terms, 34 CC terms, and 48 MF terms (Supplementary Table S12). The top 10 BP terms are shown in Figure 5A, including response to the steroid hormone, organic hydroxy compound transport, response to antibiotics, the rhythmic process, cellular response to steroid hormone stimuli, regulation of peptide secretion, cellular response to the drug, response to xenobiotic stimuli, response to alcohol, and response to nutrient levels. In addition, the top 10 CC terms included the transcription regulator complex, RNA polymerase Ⅱ transcription regulator complex, the apical part of the cell, dopaminergic synapse, the integral component of the postsynaptic membrane, the intrinsic component of the postsynaptic membrane, the membrane raft, the membrane microdomain, the membrane region, and the integral component of the synaptic membrane (Figure 5A). Moreover, the top 10 MF terms concentrated on nuclear receptor activity, ligand-activated transcription factor activity, catecholamine binding, G-protein-coupled amine receptor activity, steroid hormone receptor activity, dopamine binding, neurotransmitter receptor activity, hormone receptor binding, adrenergic receptor activity, and nuclear hormone receptor binding (Figure 5A). Additionally, these genes were enriched in 73 KEGG pathways (Supplementary Table S13), and the top 10 pathways were screened according to adjusted-P value <0.05, including prostate cancer, chemical carcinogenesis-receptor activation, the Foxo signaling pathway, Th17 cell differentiation, lipid and atherosclerosis, alcoholic liver disease, pancreatic cancer, the intestinal immune network for IgA production, Kaposi sarcoma-associated herpesvirus infection, and breast cancer pathways (Figure 5B). These results suggested that the OSTEOWONDER capsule for OP treatment might regulate the bone metabolism, stimulation response, and immune response.
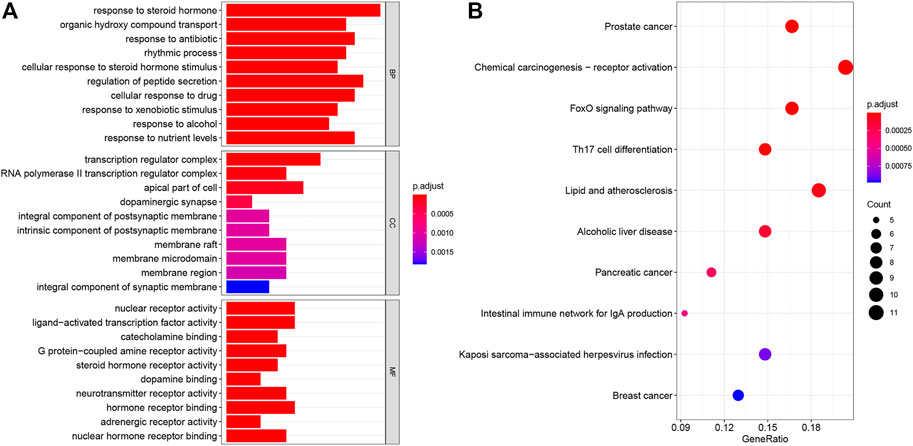
FIGURE 5. Biofunction analysis of targets. (A) Bar plot indicates the GO enrichment analysis of the common targets, including top 10 BP, top 10 CC, and top 10 MP terms. (B) Bubble plot indicates the top 10 pathways by KEGG pathway enrichment analysis of the common targets.
Construction of the PPI Network and Screening Hub Targets
In order to clarify the potential mechanisms of 58 targets of the OSTEOWONDER capsule for OP treatment, we constructed a PPI network based on interactions among 58 targets. As shown in Figure 6A, Supplementary Table S14, a PPI network included 56 nodes and 310 edges were constructed according to the confidence of 0.4. We further identified the subnetwork and hub targets from the PPI network using the cytohubba function. As shown in Figure 6B, Supplementary Table S15, a subnetwork was identified, including five nodes and 10 edges. Moreover, EGFR, HIF1A, MAPK8, IL6, and PPARG were identified as the hub targets in the OSTEOWONDER capsule for OP treatment.
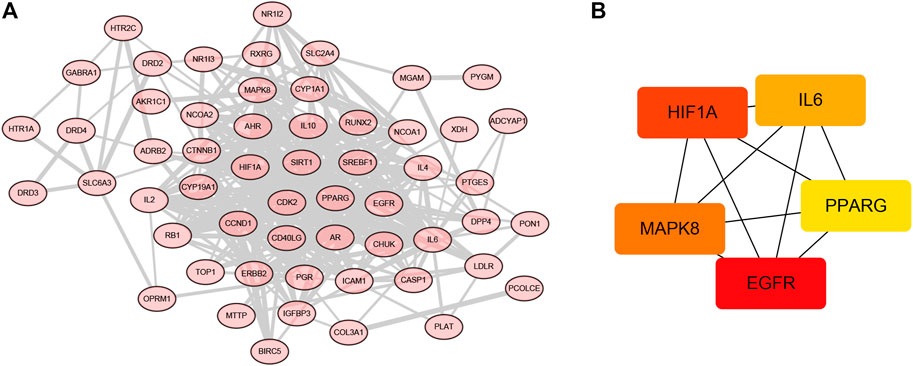
FIGURE 6. Construction of the PPI network and screening hub targets. (A) PPI network of 58 common targets. (B) Subnetwork of the PPI network.
Exploration of the Expression of Hub Targets
In order to analyze the functions of hub targets in OP, we first detected the expression of five hub targets in the HPA database. The results indicated that IL6, HIF1A, and MAPK8 expressed in the bone marrow (Figures 7A–E). We also detected the association between these gene expression and clinical traits, including bone mineral density (BMD) and female pre-/postmenopause, as shown in Figures 7F,G and Supplementary Table S16, EGFR and MAPK8 associated with low BMD, HIF1A, and IL6 were associated with postmenopausal patients.
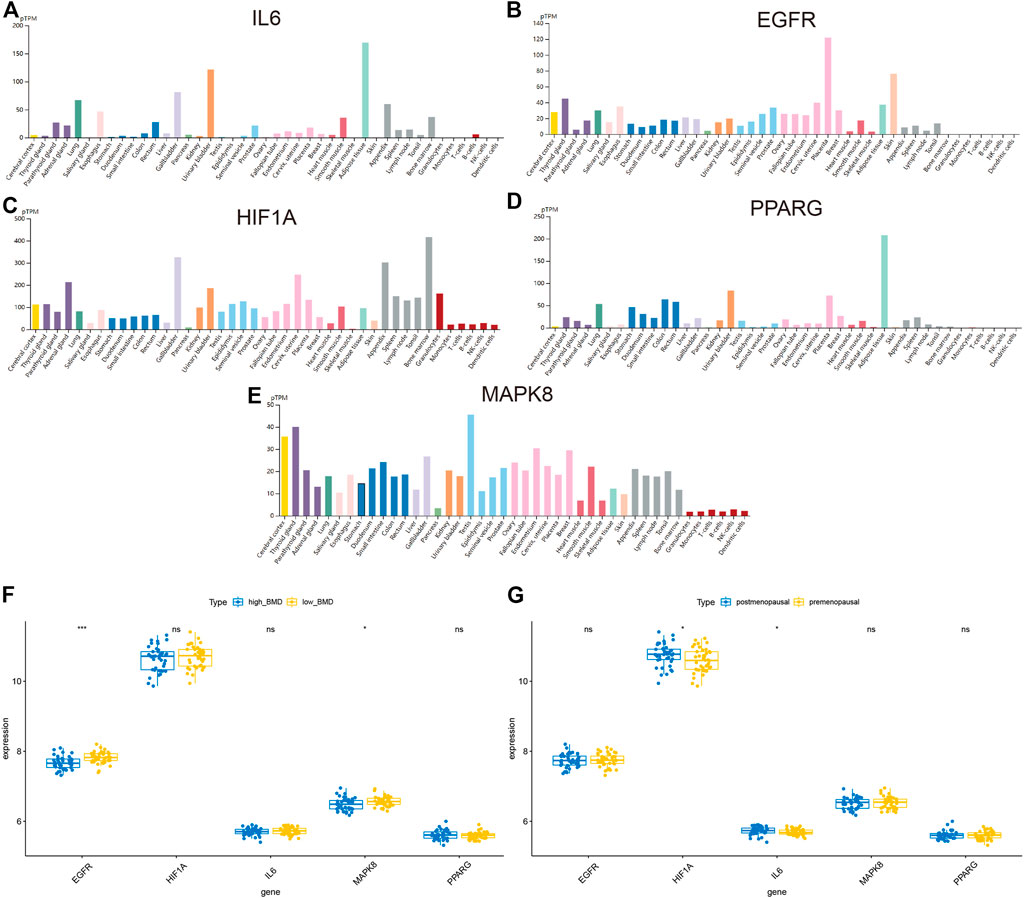
FIGURE 7. Exploration of the expression of hub targets. (A–E) Histograms of IL6, EGFR, HIF1A, PPARG, and MAPK8 in different tissues based on the HPA database. (F) Histograms showing the correlation between BMD and IL6, EGFR, HIF1A, PPARG, and MAPK8. (G) Histograms showing the correlation between pre-/postmenopausal and IL6, EGFR, HIF1A, PPARG, and MAPK8.
Bioactive Compound–Target Docking
We further performed compound–target docking to determine the interaction of the targets and their corresponding compounds. According to the herb ingredient–compound–target network, we found five hub targets mainly interacting with quercetin and nobiletin (Table 3). Therefore, we analyzed the interaction relationship between these targets and compounds. Before performing molecular docking, the structures of quercetin and nobiletin were downloaded from PubChem (Figures 8A,B), and the tertiary structures of EGFR, HIF1A, MAPK8, IL6, and PPARG proteins were obtained from the PDB database (Figure 8C). Then, molecular docking was performed using AutoDock Vina and visualized using PyMol software. We found that quercetin targeted with ALA-743, ASP-855, and THR-854 residues of EGFR through hydrogen bonding (Figure 8D), and EGFR strongly targeted with quercetin (docking score = −9.1 kcal/mol). HIF1A interacted with quercetin by hydrogen bonding connecting quercetin and THR-241, ASP-238, THR-456, and GLU-453 residues (Figure 7E), and docking score = −7.7 kcal/mol. In addition, IL6 strongly interacted with quercetin through hydrogen bonding connecting quercetin and LEU-19, ARG-24, DG-5, DG-29, and DUZ-30 based on docking score = −10.8 kcal/mol (Figure 7F). MAPK8 also strongly interacted with nobiletin (docking score = −7.5 kcal/mol), and LYS-55, LEU-110, and MET-111 targeted with nobiletin through hydrogen bonding (Figure 7G). Moreover, nobiletin targeted with PPARG through hydrogen bonding connecting ILE-326, ARG-288, and nobiletin based on docking score = −8.1 kcal/mol (Figure 7H).
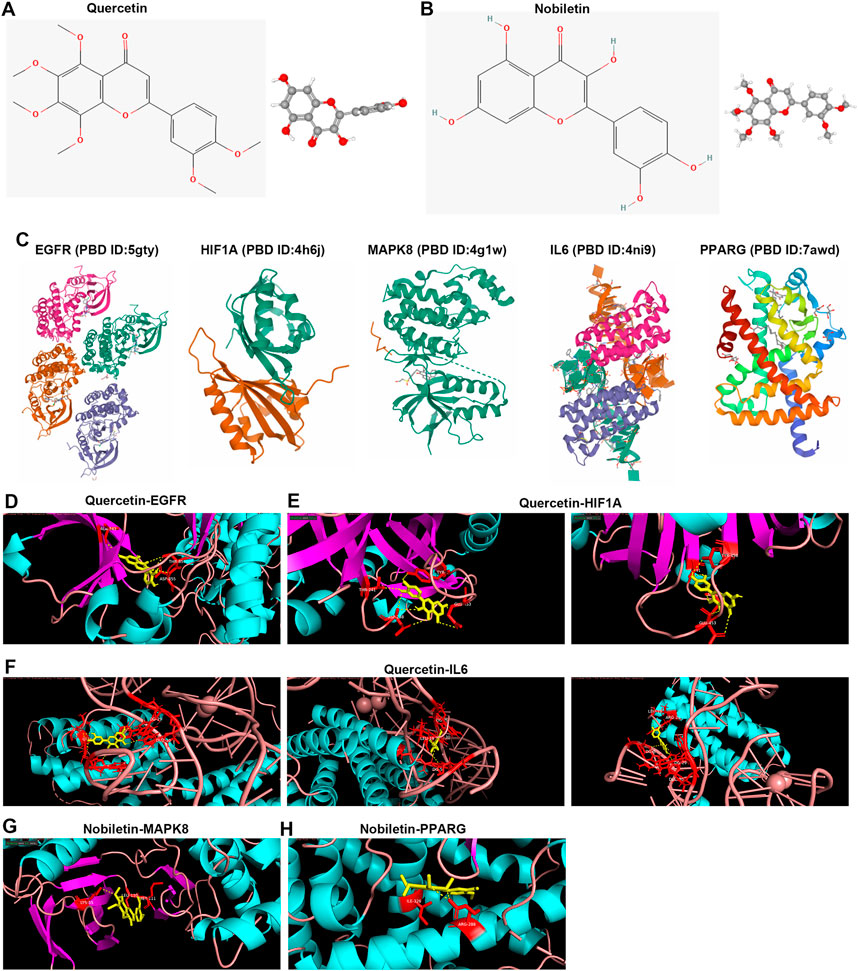
FIGURE 8. Bioactive compound–target docking. (A,B) Chemical structures of quercetin and nobiletin. (C) Tertiary structure of proteins, including EGFR, HIF1A, MAPK8, IL6, and PPARG. (D,H) Molecular docking of quercetin–EGFR, quercetin–HIFIA, quercetin–IL6, nobiletin–MAPK8, and nobiletin–PPARG. The red sticks represent amino acid residues, and yellow dotted lines represent hydrogen bonds.
Discussion
Although TCM treatment in China had a long history of thousands of years (Li and Weng, 2017), due to the lack of modern scientific and clinical evidence, and the absence of explicit molecular mechanisms and targets, TCM has been regarded as a complementary or alternative form of medicine in western countries, and this obstructs the worldwide application of TCM (Li et al., 2016; Wang et al., 2018). Artemisinin derives from the herbal Artemisia annua, which is a potent anti-malarial that was discovered by Tu Youyou. The discovery shocked the world and highlights the potential value of herbal medicine (Tu, 2016). In recent years, more and more Chinese herbals have been widely used for most disease treatments based on modern science and computer technology (Huang et al., 2019; Yang et al., 2021a; Niu et al., 2021). Network pharmacology is a powerful method that is increasingly applied for TCM research, including compounds of herb identification, targets of compound investigation, network construction, network analysis, and network verification (Luo et al., 2020).
The OSTEOWONDER capsule is a Yi ethnic medicine widely used for OP treatment (Jianming, 2020; Zhengying et al., 2021). However, the molecular mechanism of the OSTEOWONDER capsule in OP treatment remains unclear. The OSTEOWONDER capsule is comprised of Chenpi (Citrus Reticulata Reticulatae), Huangqi (Hedysarum Multijugum Maxim.), Renshen (Panax Ginseng C. A. Mey.), Honghua (Carthamus tinctorius L.), Sanqi (Radix Notoginseng), Duzhong (Eucommiae Cortex), Yangjinhua (Daturae Flos), Zuandifeng (Schizophragma integrifolium), and Biejia (Trionycis carapace). Here, we focused on the functions and regulatory mechanism of eight herbs. In this study, a total of 148 bioactive compounds and the corresponding 273 targets were identified based on TCMSP, and 4,929 targets of OP were obtained from GeneCards, DisGeNET, and OMIM databases. Moreover, six modules and 4,235 hub targets associated with OP were identified based on WGCNA. Then, 58 intersected genes were obtained by overlapping the above genes. A drug–compound–target network was constructed, which includes eight herbs, 84 bioactive compounds, and 58 targets.
The GO and KEGG pathway enrichment analyses indicated that the OSTEOWONDER capsule for OP treatment might regulate the bone metabolism, stimulation response, and immune response. For example, the biological process enrichment analysis indicated that these targets mainly associated to response to the steroid hormone, organic hydroxy compound transport, response to antibiotics, the rhythmic process, cellular response to steroid hormone stimulus, regulation of peptide secretion, cellular response to the drug, response to xenobiotic stimulus, response to alcohol, and response to nutrient levels. Steroid-induced OP is the most common form of secondary OP (Adami and Saag, 2019), and a previous study has indicated Rehmanniae radix anti-OP by intervening steroid hormone biosynthesis (Xia et al., 2019). Here, we found that the OSTEOWONDER capsule exerted anti-OP effects and was involved in response to the steroid hormone or steroid hormone stimuli. Besides, the nutrients and dietary patterns were significantly associated with OP (Muñoz-Garach et al., 2020), and OSTEOWONDER capsule anti-OP also regulated the biological process of response to nutrient levels. Besides, the molecular function was involved in nuclear receptor activity, ligand-activated transcription factor activity, catecholamine binding, G-protein-coupled amine receptor activity, steroid hormone receptor activity, dopamine binding, neurotransmitter receptor activity, hormone receptor binding, adrenergic receptor activity, and nuclear hormone receptor binding. In addition, KEGG pathway enrichment analysis revealed that these genes were mainly enriched in the Foxo signaling pathway and Th17 cell differentiation. The Foxo signaling pathway acts as an important role in regulating bone cell functions and bone health; for example, Foxo promotes normal skeletal development in chondrocytes but decreases bone resorption and formation in osteoclasts (Kim et al., 2018; Ma et al., 2020). It is known to us that regulatory T (Treg) cells and T helper 17 (Th17) cells play crucial roles in maintaining bone homeostasis, and Th17 cells promote osteoclast differentiation, which contribute to OP (Zhu et al., 2020). Maintaining the balance between Treg and Th17 cells may provide a novel promising strategy for treatment of OP (Yang et al., 2021b; Sapra et al., 2021).
In addition, we investigated the interaction relationship between these targets and identified hub targets by constructing the PPI network, and EGFR, HIF1A, MAPK8, IL6, and PPARG were identified as the hub targets in the network with the highest degree. Molecular docking was performed to mimic the connection between EGFR, HIF1A, MAPK8, IL6, and PPARG and two compounds (quercetin and nobiletin). Previous studies have demonstrated that EGFR, HIF1A, MAPK8, IL6, and PPARG exert important roles in OP progression; for example, downregulated EGFR promotes bone formation on the endosteal surface of the cortical bone (Liu et al., 2019). In addition, MAPK8 is involved in the remarkable bone loss (Li et al., 2018). However, HIF1A facilitates osteocyte-mediated osteoclastogenesis in vitro (Zhu et al., 2019). IL-6 exerts dual effects on the differentiation of osteoblasts and osteoclasts (Wang and He, 2020). Moreover, PPARG is essential for sclerostin production, which has been approved as a target for treating OP (Baroi et al., 2021). Here, we identified these genes as the key targets for OP treatment by the OSTEOWONDER capsule. Two compounds, quercetin and nobiletin, were also found to mainly target these genes.
Quercetin is one of the members of the naturally occurring flavonoid family, and is well known for its anti-oxidant, anti-inflammatory, and anti-tumor properties (Eid and Haddad, 2017; Tang et al., 2020). Surprisingly, quercetin exerts anti-OP by promoting osteogenic differentiation, anti-oxidant response, and anti-inflammatory properties (Wang et al., 2017; Yuan et al., 2018; Huang et al., 2020; Oh et al., 2020; Wang et al., 2021). Here, we found that quercetin has been used as an agent for OP by reducing EGFR, HIF1A, and IL6 expression. EGFR, HIF1A, and IL6 have also been demonstrated as the therapeutic targets of OP (Fayed et al., 2019; Luo et al., 2021; Xiong et al., 2021). Nobiletin, a polymethoxylated flavone, exhibits anti-oxidative, anti-inflammatory, and anti-cancer properties (Murakami et al., 2000; Hagenlocher et al., 2019; Feng et al., 2020). It has been found that nobiletin exerts the anti-OP effects in mice (Matsumoto et al., 2018; Wang et al., 2019). In the present study, we found that nobiletin exerted the anti-OP effects by targeting MAPK8 and PPARG.
Conclusion
In summary, a network pharmacology approach was applied to identify bioactive compounds of the OSTEOWONDER capsule and the corresponding targets. In addition, we also systemically screened out the therapeutic targets of the OSTEOWONDER capsule on OP based on online databases and WGCNA. Our evidence indicated the potential mechanism by which the OSTEOWONDER capsule ameliorates multiple pathological features of OP with multiple targets and biological pathways. Our finding provided evidence supporting the clinical application of the OSTEOWONDER capsule in OP treatment.
Data Availability Statement
The datasets presented in this study can be found in online repositories. The names of the repository/repositories and accession number(s) can be found in the article/Supplementary Material.
Author Contributions
JF and JZ drafted and edited the article. ZQ and HP performed the statistical analysis. SM, YP, and TL conducted data management and bioinformatics analysis. QL and LD supervised the project and carried out project administration, and LD acquired funding. All authors agreed on and approved the final article.
Funding
This study was supported by a grant from the Applied Basic Research Joint Project of Kunming Medical University 2019FE001 (-227).
Conflict of Interest
The authors declare that the research was conducted in the absence of any commercial or financial relationships that could be construed as a potential conflict of interest.
Publisher’s Note
All claims expressed in this article are solely those of the authors and do not necessarily represent those of their affiliated organizations or those of the publisher, the editors, and the reviewers. Any product that may be evaluated in this article or claim that may be made by its manufacturer is not guaranteed or endorsed by the publisher.
Supplementary Material
The Supplementary Material for this article can be found online at: https://www.frontiersin.org/articles/10.3389/fgene.2022.833027/full#supplementary-material
References
Adami, G., and Saag, K. G. (2019). Glucocorticoid-induced Osteoporosis Update. Curr. Opin. Rheumatol. 31 (4), 388–393. doi:10.1097/bor.0000000000000608
Alibasic, E., Ramic, E., Mujanovic, O., Avdibasic, E., Husic, D., and Alic, A. (2013). Assessment of Osteoporosis in Family Medicine Obtained by Ultrasound Densitometry. Acta Inform. Med. 21 (4), 274–276. doi:10.5455/aim.2013.21.274-276
Bao, H., Guo, H., Feng, Z., and Li, X. (2020). Deciphering the Underlying Mechanism of Xianlinggubao Capsule against Osteoporosis by Network Pharmacology. BMC Complement. Med. Ther. 20 (1), 208. doi:10.1186/s12906-020-03007-1
Baroi, S., Czernik, P. J., Chougule, A., Griffin, P. R., and Lecka-Czernik, B. (2021). PPARG in Osteocytes Controls Sclerostin Expression, Bone Mass, Marrow Adiposity and Mediates TZD-Induced Bone Loss. Bone 147, 115913. doi:10.1016/j.bone.2021.115913
Bijelic, R., Balaban, J., and Milicevic, S. (2016). Correlation of the Lipid Profile, BMI and Bone Mineral Density in Postmenopausal Women. Mater. Sociomed 28 (6), 412–415. doi:10.5455/msm.2016.28.412-415
Cai, Y., Ma, F., Qu, L., Liu, B., Xiong, H., Ma, Y., et al. (2020). Weighted Gene Co-expression Network Analysis of Key Biomarkers Associated with Bronchopulmonary Dysplasia. Front. Genet. 11, 539292. doi:10.3389/fgene.2020.539292
Chi, X., Wang, S., Baloch, Z., Zhang, H., Li, X., Zhang, Z., et al. (2019). Research Progress on Classical Traditional Chinese Medicine Formula Lily Bulb and Rehmannia Decoction in the Treatment of Depression. Biomed. Pharmacother. 112, 108616. doi:10.1016/j.biopha.2019.108616
Compston, J. E., McClung, M. R., and Leslie, W. D. (2019). Osteoporosis. The Lancet 393 (10169), 364–376. doi:10.1016/s0140-6736(18)32112-3
Cotts, K. G., and Cifu, A. S. (2018). Treatment of Osteoporosis. Jama 319 (10), 1040–1041. doi:10.1001/jama.2017.21995
Eberhardt, J., Santos-Martins, D., Tillack, A. F., and Forli, S. (2021). AutoDock Vina 1.2.0: New Docking Methods, Expanded Force Field, and Python Bindings. J. Chem. Inf. Model. 61 (8), 3891–3898. doi:10.1021/acs.jcim.1c00203
Eid, H. M., and Haddad, P. S. (2017). The Antidiabetic Potential of Quercetin: Underlying Mechanisms. Curr. Med. Chem. 24 (4), 355–364. doi:10.2174/0929867323666160909153707
Ensrud, K. E., and Crandall, C. J. (2017). Osteoporosis. Ann. Intern. Med. 167 (3), Itc17–itc32. doi:10.7326/aitc201708010
Fayed, H. A., Barakat, B. M., Elshaer, S. S., Abdel-Naim, A. B., and Menze, E. T. (2019). Antiosteoporotic Activities of Isoquercitrin in Ovariectomized Rats: Role of Inhibiting Hypoxia Inducible Factor-1 Alpha. Eur. J. Pharmacol. 865, 172785. doi:10.1016/j.ejphar.2019.172785
Feng, S.-L., Tian, Y., Huo, S., Qu, B., Liu, R.-M., Xu, P., et al. (2020). Nobiletin Potentiates Paclitaxel Anticancer Efficacy in A549/T Xenograft Model: Pharmacokinetic and Pharmacological Study. Phytomedicine 67, 153141. doi:10.1016/j.phymed.2019.153141
Gatti, D., and Fassio, A. (2019). Pharmacological Management of Osteoporosis in Postmenopausal Women: The Current State of the Art. J. Popul. Ther. Clin. Pharmacol. 26 (4), e1–e17. doi:10.15586/jptcp.v26.i4.646
Guang, Z. (2009). Chemical Constituents and Anti Inflammation Effect of the Essential Oil in Schizophragma Integrifolium. Nat. Product. Res. Dev. 21 (1), 129–131.
Hagenlocher, Y., Gommeringer, S., Held, A., Feilhauer, K., Köninger, J., Bischoff, S. C., et al. (2019). Nobiletin Acts Anti-inflammatory on Murine IL-10−/− Colitis and Human Intestinal Fibroblasts. Eur. J. Nutr. 58 (4), 1391–1401. doi:10.1007/s00394-018-1661-x
Han, L., Xie, Y.-h., Wu, R., Chen, C., Zhang, Y., and Wang, X.-p. (2017). Traditional Chinese Medicine for Modern Treatment of Parkinson's Disease. Chin. J. Integr. Med. 23 (8), 635–640. doi:10.1007/s11655-016-2537-7
Hu, M., Zhao, H. B., Wang, B., Liang, H. S., Zhang, C. Q., Zheng, H. Y., et al. (2005). Clinical Observation on Promoting Effect of Henggu Gushang union Agent on post-operational Healing of Gosselin's Fracture. Zhongguo Zhong Xi Yi Jie He Za Zhi 25 (2), 160–161.
Huang, F., Du, J., Liang, Z., Xu, Z., Xu, J., Zhao, Y., et al. (2019). Large-scale Analysis of Small RNAs Derived from Traditional Chinese Herbs in Human Tissues. Sci. China Life Sci. 62 (3), 321–332. doi:10.1007/s11427-018-9323-5
Huang, Y. Y., Wang, Z. H., Deng, L. H., Wang, H., and Zheng, Q. (2020). Oral Administration of Quercetin or its Derivatives Inhibit Bone Loss in Animal Model of Osteoporosis. Oxid Med. Cel Longev 2020, 6080597. doi:10.1155/2020/6080597
Jianming, C. (2020). Clinical Study of the Osteoking in Treating Postmenopausal. Osteoporos. Chin. J. Traditional Med. Traumatol. Orthopedics 28 (08), 46–49.
Johnston, C. B., and Dagar, M. (2020). Osteoporosis in Older Adults. Med. Clin. North America 104 (5), 873–884. doi:10.1016/j.mcna.2020.06.004
Kim, H.-N., Iyer, S., Ring, R., and Almeida, M. (2018). The Role of FoxOs in Bone Health and Disease. Curr. Top. Dev. Biol. 127, 149–163. doi:10.1016/bs.ctdb.2017.10.004
Langfelder, P., and Horvath, S. (2008). WGCNA: an R Package for Weighted Correlation Network Analysis. BMC Bioinformatics 9, 559. doi:10.1186/1471-2105-9-559
Li, F.-S., and Weng, J.-K. (2017). Demystifying Traditional Herbal Medicine with Modern Approach. Nat. Plants 3, 17109. doi:10.1038/nplants.2017.109
Li, H., Li, D., Ma, Z., Qian, Z., Kang, X., Jin, X., et al. (2018). Defective Autophagy in Osteoblasts Induces Endoplasmic Reticulum Stress and Causes Remarkable Bone Loss. Autophagy 14 (10), 1726–1741. doi:10.1080/15548627.2018.1483807
Li, L., Dou, L., Leung, P. C., Chung, T. K., and Wang, C. C. (2016). Chinese Herbal Medicines for Unexplained Recurrent Miscarriage. Cochrane Database Syst. Rev. 2016 (1), Cd010568. doi:10.1002/14651858.CD010568.pub2
Liao, Y.-H., Li, C.-I., Lin, C.-C., Lin, J.-G., Chiang, J.-H., and Li, T.-C. (2017). Traditional Chinese Medicine as Adjunctive Therapy Improves the Long-Term Survival of Lung Cancer Patients. J. Cancer Res. Clin. Oncol. 143 (12), 2425–2435. doi:10.1007/s00432-017-2491-6
Ling, H., Zeng, Q., Ge, Q., Chen, J., Yuan, W., Xu, R., et al. (2021). Osteoking Decelerates Cartilage Degeneration in DMM-Induced Osteoarthritic Mice Model through TGF-β/smad-dependent Manner. Front. Pharmacol. 12, 678810. doi:10.3389/fphar.2021.678810
Liu, C., Ma, R., Wang, L., Zhu, R., Liu, H., Guo, Y., et al. (2017). Rehmanniae Radix in Osteoporosis: A Review of Traditional Chinese Medicinal Uses, Phytochemistry, Pharmacokinetics and Pharmacology. J. Ethnopharmacology 198, 351–362. doi:10.1016/j.jep.2017.01.021
Liu, G., Xie, Y., Su, J., Qin, H., Wu, H., Li, K., et al. (2019). The Role of EGFR Signaling in Age‐related Osteoporosis in Mouse Cortical Bone. FASEB j. 33 (10), 11137–11147. doi:10.1096/fj.201900436rr
Liu, M., Gao, Y., Yuan, Y., Yang, K., Shi, S., Zhang, J., et al. (2020). Efficacy and Safety of Integrated Traditional Chinese and Western Medicine for Corona Virus Disease 2019 (COVID-19): a Systematic Review and Meta-Analysis. Pharmacol. Res. 158, 104896. doi:10.1016/j.phrs.2020.104896
Liu, X., Hu, A. X., Zhao, J. L., and Chen, F. L. (2017). Identification of Key Gene Modules in Human Osteosarcoma by Co‐Expression Analysis Weighted Gene Co‐Expression Network Analysis (WGCNA). J. Cel. Biochem. 118 (11), 3953–3959. doi:10.1002/jcb.26050
Luo, P., Wang, Y., Zhao, C., Guo, J., Shi, W., Ma, H., et al. (2021). Bazedoxifene Exhibits Anti-inflammation and Anti-atherosclerotic Effects via Inhibition of IL-6/IL-6R/STAT3 Signaling. Eur. J. Pharmacol. 893, 173822. doi:10.1016/j.ejphar.2020.173822
Luo, T.-t., Lu, Y., Yan, S.-k., Xiao, X., Rong, X.-l., and Guo, J. (2020). Network Pharmacology in Research of Chinese Medicine Formula: Methodology, Application and Prospective. Chin. J. Integr. Med. 26 (1), 72–80. doi:10.1007/s11655-019-3064-0
Ma, X., Su, P., Yin, C., Lin, X., Wang, X., Gao, Y., et al. (2020). The Roles of FoxO Transcription Factors in Regulation of Bone Cells Function. Int. J. Mol. Sci. 21 (3). doi:10.3390/ijms21030692
Ma, Z., Zhang, B., Fan, Y., Wang, M., Kebebe, D., Li, J., et al. (2019). Traditional Chinese Medicine Combined with Hepatic Targeted Drug Delivery Systems: A New Strategy for the Treatment of Liver Diseases. Biomed. Pharmacother. 117, 109128. doi:10.1016/j.biopha.2019.109128
Matsumoto, S., Tominari, T., Matsumoto, C., Yoshinouchi, S., Ichimaru, R., Watanabe, K., et al. (2018). Effects of Polymethoxyflavonoids on Bone Loss Induced by Estrogen Deficiency and by LPS-dependent Inflammation in Mice. Pharmaceuticals (Basel) 11 (1). doi:10.3390/ph11010007
Muñoz-Garach, A., García-Fontana, B., and Muñoz-Torres, M. (2020). Nutrients and Dietary Patterns Related to Osteoporosis. Nutrients 12 (7). doi:10.3390/nu12071986
Murakami, A., Nakamura, Y., Torikai, K., Tanaka, T., Koshiba, T., Koshimizu, K., et al. (2000). Inhibitory Effect of Citrus Nobiletin on Phorbol Ester-Induced Skin Inflammation, Oxidative Stress, and Tumor Promotion in Mice. Cancer Res. 60 (18), 5059–5066.
Nguyen, N. T., Nguyen, T. H., Pham, T. N. H., Huy, N. T., Bay, M. V., Pham, M. Q., et al. (2020). Autodock Vina Adopts More Accurate Binding Poses but Autodock4 Forms Better Binding Affinity. J. Chem. Inf. Model. 60 (1), 204–211. doi:10.1021/acs.jcim.9b00778
Niu, W. H., Wu, F., Cao, W. Y., Wu, Z. G., Chao, Y. C., and Liang, C. (2021). Network Pharmacology for the Identification of Phytochemicals in Traditional Chinese Medicine for COVID-19 that May Regulate Interleukin-6. Biosci. Rep. 41 (1). doi:10.1042/BSR20202583
Oh, J. H., Karadeniz, F., Seo, Y., and Kong, C. S. (2020). Effect of Quercetin 3-O-β-D-Galactopyranoside on the Adipogenic and Osteoblastogenic Differentiation of Human Bone Marrow-Derived Mesenchymal Stromal Cells. Int. J. Mol. Sci. 21 (21). doi:10.3390/ijms21218044
Peng, L., Li, B. y., Wang, W., Gao, X. s., Zeng, X., and Luo, D. y. (2021). Identification of Key Genes in Human Urothelial Cells Corresponding to Interstitial Cystitis/bladder Pain Syndrome in a Lipopolysaccharide‐induced Cystitis Model. Neurourol Urodyn 40 (7), 1720–1729. doi:10.1002/nau.24743
Piñero, J., Ramírez-Anguita, J. M., Saüch-Pitarch, J., Ronzano, F., Centeno, E., Sanz, F., et al. (2020). The DisGeNET Knowledge Platform for Disease Genomics: 2019 Update. Nucleic Acids Res. 48 (D1), D845–d855. doi:10.1093/nar/gkz1021
Piñero, J., Bravo, À., Queralt-Rosinach, N., Gutiérrez-Sacristán, A., Deu-Pons, J., Centeno, E., et al. (2017). DisGeNET: a Comprehensive Platform Integrating Information on Human Disease-Associated Genes and Variants. Nucleic Acids Res. 45 (D1), D833–d839. doi:10.1093/nar/gkw943
Pinzi, L., and Rastelli, G. (2019). Molecular Docking: Shifting Paradigms in Drug Discovery. Int. J. Mol. Sci. 20 (18). doi:10.3390/ijms20184331
Qin, D., Zhang, H., Zhang, H., Sun, T., Zhao, H., and Lee, W.-H. (2019). Anti-osteoporosis Effects of Osteoking via Reducing Reactive Oxygen Species. J. Ethnopharmacology 244, 112045. doi:10.1016/j.jep.2019.112045
Ru, J., Li, P., Wang, J., Zhou, W., Li, B., Huang, C., et al. (2014). TCMSP: a Database of Systems Pharmacology for Drug Discovery from Herbal Medicines. J. Cheminform 6, 13. doi:10.1186/1758-2946-6-13
Sapra, L., Dar, H. Y., Bhardwaj, A., Pandey, A., Kumari, S., Azam, Z., et al. (2021). Lactobacillus Rhamnosus Attenuates Bone Loss and Maintains Bone Health by Skewing Treg-Th17 Cell Balance in Ovx Mice. Sci. Rep. 11 (1), 1807. doi:10.1038/s41598-020-80536-2
Shi, Z. Y., Zhang, X. G., Li, C. W., Liu, K., Liang, B. C., and Shi, X. L. (2017). Effect of Traditional Chinese Medicine Product, QiangGuYin, on Bone Mineral Density and Bone Turnover in Chinese Postmenopausal Osteoporosis. Evid. Based Complement. Alternat Med. 2017, 6062707. doi:10.1155/2017/6062707
Stelzer, G., Rosen, N., Plaschkes, I., Zimmerman, S., Twik, M., Fishilevich, S., et al. (2016). The GeneCards Suite: From Gene Data Mining to Disease Genome Sequence Analyses. Curr. Protoc. Bioinformatics 54, 1–33. 1.30.1-1.30.33. doi:10.1002/cpbi.5
Sun, Y., Chen, R., Zhu, D., Shen, Z. Q., Zhao, H. B., and Lee, W. H. (2020). Osteoking Improves OP Rat by Enhancing HSP90-β E-xpression. Int. J. Mol. Med. 45 (5), 1543–1553. doi:10.3892/ijmm.2020.4529
Sun, Y., Wang, X., Chen, R., Zhu, D., Shen, Z., Zhao, H., et al. (2020). Osteoking Downregulates Mgp in an Osteoporotic Fracture Rat Model. J. Tradit Chin. Med. 40 (3), 422–431. doi:10.19852/j.cnki.jtcm.2020.03.010
Tang, S.-M., Deng, X.-T., Zhou, J., Li, Q.-P., Ge, X.-X., and Miao, L. (2020). Pharmacological Basis and New Insights of Quercetin Action in Respect to its Anti-cancer Effects. Biomed. Pharmacother. 121, 109604. doi:10.1016/j.biopha.2019.109604
Tu, Y. (2016). Artemisinin-A Gift from Traditional Chinese Medicine to the World (Nobel Lecture). Angew. Chem. Int. Ed. 55 (35), 10210–10226. doi:10.1002/anie.201601967
Wang, J., Qi, F., Wang, Z., Zhang, Z., Pan, N., Huai, L., et al. (2020). A Review of Traditional Chinese Medicine for Treatment of Glioblastoma. Biosci. Trends 13 (6), 476–487. doi:10.5582/bst.2019.01323
Wang, J., Ma, Q., Li, Y., Li, P., Wang, M., Wang, T., et al. (2020). Research Progress on Traditional Chinese Medicine Syndromes of Diabetes Mellitus. Biomed. Pharmacother. 121, 109565. doi:10.1016/j.biopha.2019.109565
Wang, J., Wong, Y.-K., and Liao, F. (2018). What Has Traditional Chinese Medicine Delivered for Modern Medicine? Expert Rev. Mol. Med. 20, e4. doi:10.1017/erm.2018.3
Wang, N., Wang, L., Yang, J., Wang, Z., and Cheng, L. (2021). Quercetin Promotes Osteogenic Differentiation and Antioxidant Responses of Mouse Bone Mesenchymal Stem Cells through Activation of the AMPK/SIRT1 Signaling Pathway. Phytother Res. 9. doi:10.1002/ptr.7010
Wang, Q. L., Huo, X. C., Wang, J. H., Wang, D. P., Zhu, Q. L., Liu, B., et al. (2017). Rutin Prevents the Ovariectomy-Induced Osteoporosis in Rats. Eur. Rev. Med. Pharmacol. Sci. 21 (8), 1911–1917.
Wang, T., and He, C. (2020). TNF-α and IL-6: The Link between Immune and Bone System. Cdt 21 (3), 213–227. doi:10.2174/1389450120666190821161259
Wang, Y., Xie, J., Ai, Z., and Su, J. (2019). Nobiletin-loaded Micelles Reduce Ovariectomy-Induced Bone Loss by Suppressing Osteoclastogenesis. Ijn 14, 7839–7849. doi:10.2147/ijn.s213724
Xia, T., Dong, X., Jiang, Y., Lin, L., Dong, Z., Shen, Y., et al. (2019). Metabolomics Profiling Reveals Rehmanniae Radix Preparata Extract Protects against Glucocorticoid-Induced Osteoporosis Mainly via Intervening Steroid Hormone Biosynthesis. Molecules 24 (2). doi:10.3390/molecules24020253
Xiang, Y., Guo, Z., Zhu, P., Chen, J., and Huang, Y. (2019). Traditional Chinese Medicine as a Cancer Treatment: Modern Perspectives of Ancient but Advanced Science. Cancer Med. 8 (5), 1958–1975. doi:10.1002/cam4.2108
Xiong, Z., Zheng, C., Chang, Y., Liu, K., Shu, L., and Zhang, C. (2021). Exploring the Pharmacological Mechanism of Duhuo Jisheng Decoction in Treating Osteoporosis Based on Network Pharmacology. Evid. Based Complement. Alternat Med. 2021, 5510290. doi:10.1155/2021/5510290
Yang, X., Zhou, F., Yuan, P., Dou, G., Liu, X., Liu, S., et al. (2021). T Cell-Depleting Nanoparticles Ameliorate Bone Loss by Reducing Activated T Cells and Regulating the Treg/Th17 Balance. Bioactive Mater. 6 (10), 3150–3163. doi:10.1016/j.bioactmat.2021.02.034
Yang, Z., Zhang, Q., Yu, L., Zhu, J., Cao, Y., and Gao, X. (2021). The Signaling Pathways and Targets of Traditional Chinese Medicine and Natural Medicine in Triple-Negative Breast Cancer. J. Ethnopharmacology 264, 113249. doi:10.1016/j.jep.2020.113249
Yu, C., Dai, L., Ma, Z., Zhao, H., Yuan, Y., Zhang, Y., et al. (2019). Effect of Osteoking on the Osteogenic and Adipogenic Differentiation Potential of Rat Bone Marrow Mesenchymal Stem Cells In Vitro. BMC Complement. Altern. Med. 19 (1), 36. doi:10.1186/s12906-019-2435-6
Yuan, S., Chan, H. C. S., Filipek, S., and Vogel, H. (2016). PyMOL and Inkscape Bridge the Data and the Data Visualization. Structure 24 (12), 2041–2042. doi:10.1016/j.str.2016.11.012
Yuan, Z., Min, J., Zhao, Y., Cheng, Q., Wang, K., Lin, S., et al. (2018). Quercetin Rescued TNF-Alpha-Induced Impairments in Bone Marrow-Derived Mesenchymal Stem Cell Osteogenesis and Improved Osteoporosis in Rats. Am. J. Transl Res. 10 (12), 4313–4321.
Zeng, Q., Li, N., Wang, Q., Feng, J., Sun, D., Zhang, Q., et al. (2019). The Prevalence of Osteoporosis in China, a Nationwide, Multicenter DXA Survey. J. Bone Miner Res. 34 (10), 1789–1797. doi:10.1002/jbmr.3757
Zhai, B., Zhang, N., Han, X., Li, Q., Zhang, M., Chen, X., et al. (2019). Molecular Targets of β-elemene, a Herbal Extract Used in Traditional Chinese Medicine, and its Potential Role in Cancer Therapy: A Review. Biomed. Pharmacother. 114, 108812. doi:10.1016/j.biopha.2019.108812
Zhang, Y., Liu, M., Li, H., Chen, Z., Liang, N., Xu, J., et al. (2017). Traditional Chinese Medicine Bushen-Jianpi-Huoxue Decoction Prevents Diabetic Osteoporosis in Rats via Wnt and Nuclear Factor-Kappa B Signaling Pathways. Int. J. Rheum. Dis. 20 (8), 941–948. doi:10.1111/1756-185x.13050
Zhang, Y., Lu, P., Qin, H., Zhang, Y., Sun, X., Song, X., et al. (2021). Traditional Chinese Medicine Combined with Pulmonary Drug Delivery System and Idiopathic Pulmonary Fibrosis: Rationale and Therapeutic Potential. Biomed. Pharmacother. 133, 111072. doi:10.1016/j.biopha.2020.111072
Zhao, H. B., Hu, M., and Liang, H. S. (2006). Experimental Study on Osteoking in Promoting Gene Expression of Core Binding Factor Alpha 1 in Necrotic Femoral Head of Rabbits. Zhongguo Zhong Xi Yi Jie He Za Zhi 26 (11), 1003–1006.
Zhengying, Z., Xiaoning, L., and Liang, S. (2021). Clinical Effect of Henggu Bone Wound Healing Agent in Promoting Postoperative Healing of Lower Tabial Fractures. J. Clin. Rational Drug Use 14 (23), 16–18.
Zhu, J., Tang, Y., Wu, Q., Ji, Y. C., Feng, Z. F., and Kang, F. W. (2019). HIF‐1α Facilitates Osteocyte‐mediated Osteoclastogenesis by Activating JAK2/STAT3 Pathway In Vitro. J. Cel Physiol 234 (11), 21182–21192. doi:10.1002/jcp.28721
Zhu, K., and Prince, R. L. (2015). Lifestyle and Osteoporosis. Curr. Osteoporos. Rep. 13 (1), 52–59. doi:10.1007/s11914-014-0248-6
Keywords: network pharmacology, molecular docking, OSTEOWONDER capsule, osteoporosis, WGCNA
Citation: Fan J, Zhou J, Qu Z, Peng H, Meng S, Peng Y, Liu T, Luo Q and Dai L (2022) Network Pharmacology and Molecular Docking Elucidate the Pharmacological Mechanism of the OSTEOWONDER Capsule for Treating Osteoporosis. Front. Genet. 13:833027. doi: 10.3389/fgene.2022.833027
Received: 13 December 2021; Accepted: 09 February 2022;
Published: 28 February 2022.
Edited by:
Gary S. Stein, University of Vermont, United StatesReviewed by:
Karuppiah Thilakavathy, Universiti Putra Malaysia, MalaysiaRajeev K. Singla, Sichuan University, China
Copyright © 2022 Fan, Zhou, Qu, Peng, Meng, Peng, Liu, Luo and Dai. This is an open-access article distributed under the terms of the Creative Commons Attribution License (CC BY). The use, distribution or reproduction in other forums is permitted, provided the original author(s) and the copyright owner(s) are credited and that the original publication in this journal is cited, in accordance with accepted academic practice. No use, distribution or reproduction is permitted which does not comply with these terms.
*Correspondence: Qiu Luo, THVvcWl1LTIyMEAxNjMuY29t; Lifen Dai, MTc3MjMzNjQ5MkBxcS5jb20=
†These authors have contributed equally to this work and share first authorship