- 1State Key Laboratory of Plant Cell and Chromosome Engineering, Institute of Genetics and Developmental Biology, The Innovative Academy of Seed Design, Chinese Academy of Sciences, Beijing, China
- 2College of Life Science and Technology, Inner Mongolia Normal University, Hohhot, China
- 3University of Chinese Academy of Sciences, Beijing, China
To accelerate the exploitation and use of marginal soils and develop salt-tolerant forage germplasm suitable for the coastal regions of China, seven lines of decaploid tall wheatgrass [Thinopyrum ponticum (Podp.) Barkworth and D. R. Dewey, 2n = 10x = 70] were transplanted under low (.3%) and high (.5%) salt conditions for a comprehensive analysis at the adult-plant stage. Differences were observed among these materials, especially in terms of grass yield, agronomic characteristics, and physiological and biochemical indices. Line C2 grew best with the highest shoot total fresh and dry weights under all conditions except for the milk-ripe stage in Dongying in 2019. The total membership value of C2 also reflected its excellent performance after transplanting. As superior germplasm, its relatively high antioxidant enzyme activities and chlorophyll a/b ratio suggested C2 may maintain normal metabolic and physiological functions under saline conditions. Furthermore, decaploid tall wheatgrass as a forage grass species has a high nutritive value beneficial for animal husbandry. Accordingly, line C2 may be used as excellent germplasm to develop salt-tolerant cultivars in the Circum-Bohai sea.
Introduction
Soil salinization is a major environmental stress factor that inhibits normal plant growth while also leading to soil degradation and significant deterioration of the global ecosystem (Bazihizina et al., 2012; Ding et al., 2021). More than 800 million hectares (6.5%) of the land area worldwide contain saline-alkali soil (FAO, 2017; Zhang et al., 2018). In China, the related figure is 100 million, of which over 80% are undeveloped (Xie et al., 2021). Salinized soils, which are mainly distributed in the northwestern, northern, northeastern, and coastal regions of China, result from seawater impregnation, volcanic movement, salt bioaccumulation, uplift of saline groundwater, and agricultural irrigation (Wang et al., 2020). The most effective and environmentally friendly methods for controlling soil salinization involve the absorption, transformation, or transfer of salt from the soil via the metabolic and growth activities of plants and microorganisms. Cultivating salt-tolerant crops on salinized land may lead to increased transpiration, decreased groundwater levels, and inhibited soil salinization (Qadir and Oster, 2004). For the past decade, studies on many forage species, such as Leymus chinensis (Wang et al., 1994), Achnatherum splendens (Xu et al., 2008), and Hordeum jubatum L. (Chen et al., 2021), revealed that these species have adapted to salinized soil, suggesting they are useful for controlling and improving salinized soils.
Decaploid tall wheatgrass [Thinopyrum ponticum (Podp.) Barkworth and D. R. Dewey, 2n = 10x = 70, syn. Agropyron elongatum (Host) P. Beauv., Elytrigia pontica (Podp.) Holub, and Lophopyrum ponticum (Podp.) Á Löve] is a perennial forage grass species and an essential wild relative to improving wheat (Shannon, 1978; Sharma et al., 1989). Previous study indicated that it had stronger salt tolerance than most monocotyledonous species, such as rice, wheat, and barley, and some dicotyledonous species, such as alfalfa (Munns and Tester, 2008). Colmer et al. (2006) determined that several Th. ponticum accessions could survive a treatment with 750 mM NaCl and some could grow reasonably well at an electrical conductivity of 13.9 dS·m−1. Additionally, the enhanced salt tolerance of Lophopyrum elongatum, which is an ancestor of Th. ponticum, appears to be mediated by genes on chromosomes 2Ee, 3Ee, 4Ee, and 7Ee (Dvořák et al., 1988). Furthermore, chromosome 3Ee was linked to a 50% decrease in Na+ accumulation in the flag leaf (Omielan et al., 1991). Researchers subsequently used the ph1b mutant to induce the homoeologous recombination between chromosome 3Ee and wheat chromosomes 3A and 3D. An examination of the resulting recombinant lines revealed that the lines with the smallest alien segments exhibited the sodium exclusion trait (Mullan et al., 2009). Moreover, chromosome 5Eb of Th. bessarabicum, which is another ancestor of Th. ponticum, includes at least one major dominant gene for salt tolerance (Forster et al., 1988; King et al., 1997). Thus, Th. ponticum chromosomal segments likely carry genes that can be used to increase the salt tolerance of wheat. For example, the dominant salt tolerance gene block in the genome of the wheat-Th. ponticum translocation line S148 was detected by a salinity test involving the backcross progenies of S148 and Chinese Spring (Yuan and Tomita, 2015). The saline-tolerant cultivar Shanrong No. 3, which was produced from a somatic hybridization between bread wheat and tall wheatgrass, is a valuable genetic resource for characterizing the mechanisms underlying salt tolerance. Its excellent growth recovery, ionic homeostasis, and ability to excrete toxic products were demonstrated by two-dimensional gel electrophoresis and mass spectrometry analyses (Peng et al., 2009).
The nearly 200,000 ha of saline soil in the Yellow River Delta, which is a representative coastal saline zone in China, are mainly the result of the excessive accumulation of Na+ and Cl− ions (Jia et al., 2013). This region has not been well exploited and used, which has seriously hindered the regional economy and ecological development. The salt content of the saline soil widely distributed in the Yellow River Delta can exceed 3% but usually ranges from .6 to 1.0%. Moreover, extreme weather conditions in this area, such as drought in the spring and excessive rainfall in the summer, have been common in recent years. In 2020, Prof. Zhensheng Li proposed a new initiative involving the development of a coastal grass belt, in which salt-tolerant forage grass species are cultivated on 667,000 ha of saline and alkaline soils around the Bohai Sea (Li et al., 2022). Because Th. ponticum can grow in saline soil and withstand drought and waterlogging, it may be a suitable forage species for the coastal saline zone in China (Rogers and Bailey, 1963; Shannon, 1978; Weimberg and Shannon, 1988; Jenkins et al., 2010; Guo et al., 2015; Borrajo et al., 2018; Ruf and Emmerling, 2018). Furthermore, the developed root system of tall wheatgrass can accumulate soil organic matter and improve soil fertility, which will positively contribute to regional agricultural and ecological development (Pimentel et al., 1987). To achieve this goal, Th. ponticum lines suitable for local conditions must be identified.
In this study, we selected the sexually reproducing plants of seven Th. ponticum clone lines as materials for an investigation of their agronomic traits, quality-related characteristics, and physiological and biochemical indices under salt stress conditions. The objective of this study was to identify salt-tolerant Th. ponticum germplasm with excellent agronomic characteristics that may be useful for breeding new Th. ponticum varieties, ideal for the saline soil in the coastal regions of China.
Materials and Methods
Plant Materials
In 2008, Zhensheng Li’s group selected seven phenotypically distinct tall wheatgrass individuals. Their clone lines (designated as C1–C7) were vegetatively propagated by cutting tillers with living roots. The seeds of these clone lines were collected for this study. The clone lines and the seeds were preserved in the laboratory of Zhensheng Li at the Institute of Genetics and Developmental Biology, The Innovative Academy of Seed Design, Chinese Academy of Sciences.
Study Sites and Field Experiments
The seeds of each clone line were sown in seedling trays containing a mixture of field soil and nutrient soil (3:1). The resulting seedlings with fully expanded second leaves were transplanted on 21 March 2018 to fields at the Haixing Experimental Station of Chinese Academy of Sciences (117.6°E, 38.2°N; .5% soil salt content) and on 9 April 2018 to fields at the Dongying Molecular Design Breeding Experimental Station of Chinese Academy of Sciences (118.9°E, 37.7°N; .3% soil salt content), with three plants per hole. The holes in each row were separated by 30 cm, and the rows were separated by 30 cm. Three replicates of each line were set, and each replicate was grown in a 3.0 m × 1.2 m plot. The transplanted seedlings were irrigated with freshwater to optimize the survival rate. There was no additional artificial irrigation during the cropping season. Plants were cultivated using conventional field management practices. Three uniform plants were selected from each plot, and the plant height (PH, cm), tiller number (TN), spike number (SN), spikelet number per spike (SNPS), leaf fresh weight (LFW, g), stem fresh weight (SFW, g), shoot total fresh weight (STFW, g), and shoot total dry weight (STDW, g) of the Th. ponticum plants were analyzed at the flowering and milk-ripe stages in Haixing in 2018 (18HF and 18HM) and Dongying in 2018 (18DF and 18DM) and 2019 (19DF and 19DM). Due to the heavy rain in June 2019, the accuracy of STFW at the flowering stage might be affected. Thus, only STDW was measured in 19DF.
Analysis of Nutrient Contents
Fresh leaves of three plants were collected from each plot at the flowering stage in Haixing in 2018 and then heated at 105°C for 30 min, dried to a constant weight at 65°C, and ground to a powder and mixed in equal proportions for an analysis of the nutrient content. The dry matter (DM) content was determined after drying samples according to a published method by Zhang (2007). The crude protein (CP) content was determined using the Folin–Ciocalteu reagent (Yang, 1999). The neutral detergent fiber (NDF) content and the acid detergent fiber (ADF) content were measured on the basis of filter bag technology using the ANKOM A2000i automatic and semi-automatic fiber meters, respectively, according to Van Soest et al. (1991) with some modifications. The water-soluble carbohydrate (WSC) content was determined using an anthrone colorimetric technique (Zhang, 2007). The ether extract (EE) content and the ash content were measured as previously described (ISO 6492:1999, IDT and Mlejnkova et al., 2016, respectively), with some modifications. The tannin content (TC) was determined according to the vanillin hydrochloric acid method. The forage relative feeding value (RFV) and total digestible nutrient (TDN) content, as well as the relative forage quality (RFQ), were calculated on the basis of the NDF and ADF data using the following equations (Zhang et al., 2004; Schacht et al., 2010; Xiong et al., 2018):
Determination of Physiological and Biochemical Indices
For each replicate, a .05 g fresh sample of three plants was collected at the milk-ripe stage in Dongying in 2019 and used to measure the superoxide dismutase (SOD) activity (absorbance at 560 nm) as described by García-Triana et al. (2010). The catalase (CAT) activity (absorbance at 240 nm) was determined using a method developed by Sima et al. (2011). Chlorophyll a (Chl a) and chlorophyll b (Chl b) were extracted using 80% acetone, and their contents were determined by measuring the absorbances of the extracts at 645 and 663 nm as described by Arnon (1949). All absorbances were measured using the SpectraMax190 microplate reader (Molecular Devices, America). The proline (Pro) content was determined using ninhydrin (Bates et al., 1973).
Data Analysis
The membership function value was used for the total membership value (TMV) analysis. The specific formula is as follows:
Results
Biomass Indices
To evaluate the growth of seven Th. ponticum lines under coastal saline conditions, we analyzed the biomass indices, including STFW and STDW, of each line at the flowering and milk-ripe stages at the Haixing and Dongying experimental stations in 2018 and the Dongying experimental station in 2019. The results for the seven examined lines in six environments are provided in Figure 1. The yields of all Th. ponticum lines were generally higher in Haixing than in Dongying during the same growth period in 2018, possibly because the seedlings were transplanted about 20 days earlier in Haixing than in Dongying. In 2018, for most lines, the yields were greater at the milk-ripe stage than at the flowering stage at both experimental stations. A comparison of the data collected during the same developmental period in 2018 and 2019 revealed that most Th. ponticum lines produced more biomass in 2019.
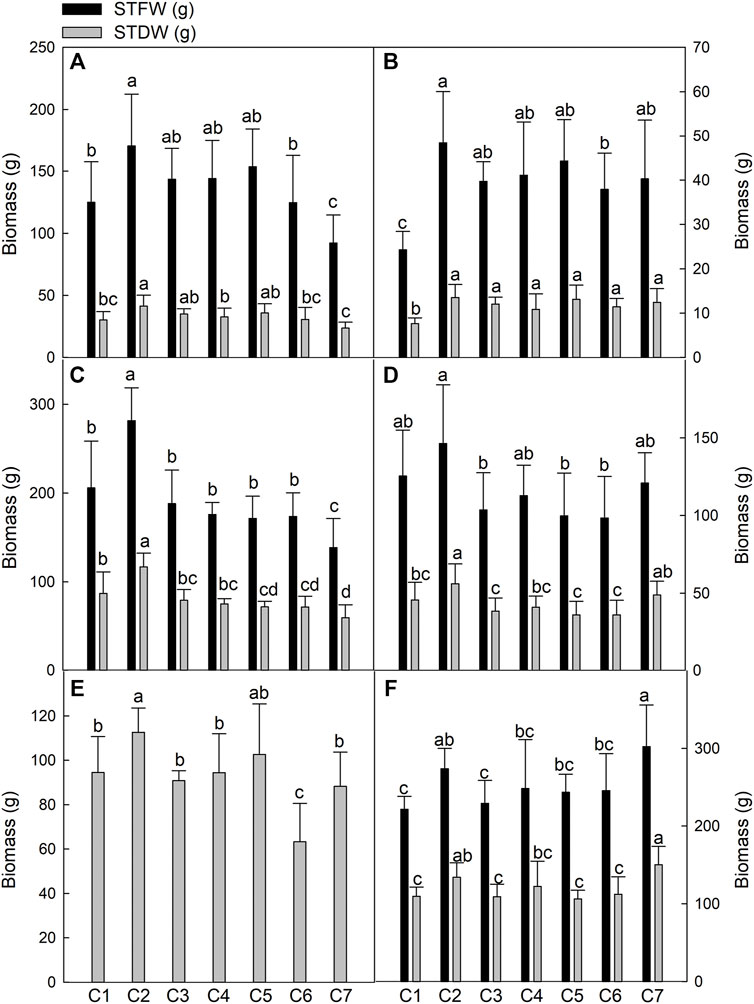
FIGURE 1. Comparison of biomass of seven Th. ponticum lines at the flowering stage at Haixing station in 2018 (A), at the flowering stage at Dongying station in 2018 (B), at the milk-ripe stage at Haixing station in 2018 (C), at the milk-ripe stage at Dongying station in 2018 (D), at the flowering stage at Dongying station in 2019 (E), and at the milk-ripe stage at Dongying station in 2019 (F). STFW, shoot total fresh weight; STDW, shoot total dry weight. Different letters indicate a significant difference between seven Th. ponticum lines at p < .05 level.
During the milk-ripe stage in 2018, STFW was 138.62–281.64 g in Haixing and 98.43–146.40 g in Dongying, whereas STDW was 59.66–116.90 g in Haixing and 36.03–56.13 g in Dongying. In 2019, STDW was 63.26–112.63 g at the flowering stage and 106.33–150.37 g at the milk-ripe stage. Thus, there were distinct biomass differences among the samples. More specifically, STFW and STDW were highest for line C2 under all conditions, except for 19DM. Although the grass yield of line C7 was the highest in 19DM, there were no significant differences between C2 and C7 in terms of STFW and STDW. During the first growing season, the biomass of C2 was significantly higher (p < .05) than that of the other lines in 18HM. After one growing season, C2 could still grow vigorously, while C7 flourished similarly at the milk-ripe stage in Dongying in 2019. Compared with the corresponding growth in Dongying, C7 in Haixing had a lower STFW and STDW during both stages in 2018, implying C7 may be suitable for growth in a relatively low salinity soil with high regeneration ability. In summary, the biomass of C2 was prominent among these seven lines under salt stress.
Agronomic Traits
Traits related to growth performance, including PH, TN, SNPS, SN, LFW, and SFW, were evaluated for each Th. ponticum line in Haixing (Figure 2) and Dongying (Figure 3) in 2018. At the Haixing experimental station, the values of all traits, except for LFW, generally increased as plants matured. The differences among the seven lines were greater in 18HM than in 18HF. For example, there were no significant differences in PH among the seven lines in 18HF. However, in 18HM, the lines were divided into three groups according to their PH, and C7 was significantly shorter (p < .05) than C1, C2, C3, and C4. Additionally, PH was the highest for C4, with an average of 144.92 cm, 15.15 cm greater than the PH of C7 (Figure 2A). The SNPS of C2 (19.40) and C3 (19.00) were significantly higher (p < .05) than that of C6 (16.56) in 18HM. Line C2 had the highest TN and SN at both stages, and the differences with the corresponding values for the other lines were significant (p < .05) in 18HM. In contrast, C7 had the lowest TN in 18HM (Figure 2B) and the lowest SN in 18HF and 18HM (Figure 2D). In Haixing, LFW was a little lower at the milk-ripe stage than at the flowering stage for all lines. At both stages, C2 and C7 had the highest and lowest LFW, respectively. Additionally, C2 had a significantly higher LFW (p < .05) than the other lines in 18HM. Similarly, C2 had the highest SFW among the seven lines in both 18HF and 18HM; the differences in SFW between C2 and the other lines were significant (p < .05) in 18HM. Considered together, C2 exhibited excellent growth during the first growing season under high salt conditions. At 132 days after transplanting, the three individual plants in each hole of C2 were 142 cm tall, with 84 tillers, 42 spikes, and 19 spikelets, and produced 52.30 g fresh leaves and 229.34 g fresh stems. Moreover, PH, TN, SN, LFW, and SFW were lowest for C7 among the seven examined lines; the differences in these traits between C7 and C2 were significant (p < .05). From the flowering stage to the milk-ripe stage, line C2 decreased the minimum LFW and increased the maximum SN, which resulted in its maintaining the fastest growth rate after entering the reproductive growth stage when compared with the other lines.
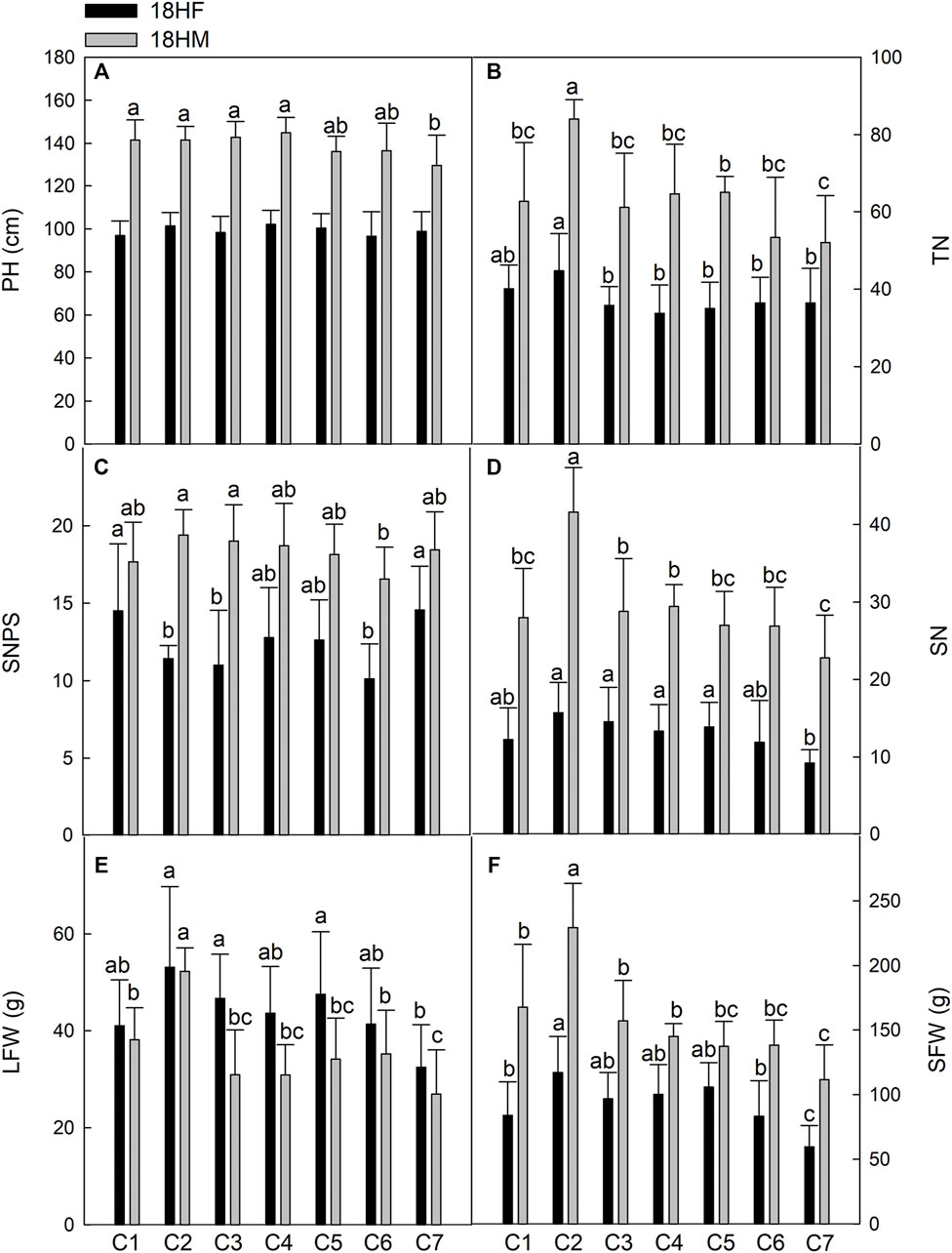
FIGURE 2. Comparison of main agronomic traits of seven Th. ponticum lines at Haixing station at different growing stages. (A) Plant height (PH). (B) Tiller number (TN). (C) Spikelet number per spike (SNPS). (D) Spike number (SN). (E) Leaf fresh weight (LFW). (F) Stem fresh weight (SFW). Different letters indicate a significant difference between seven Th. ponticum lines at p < .05 level.
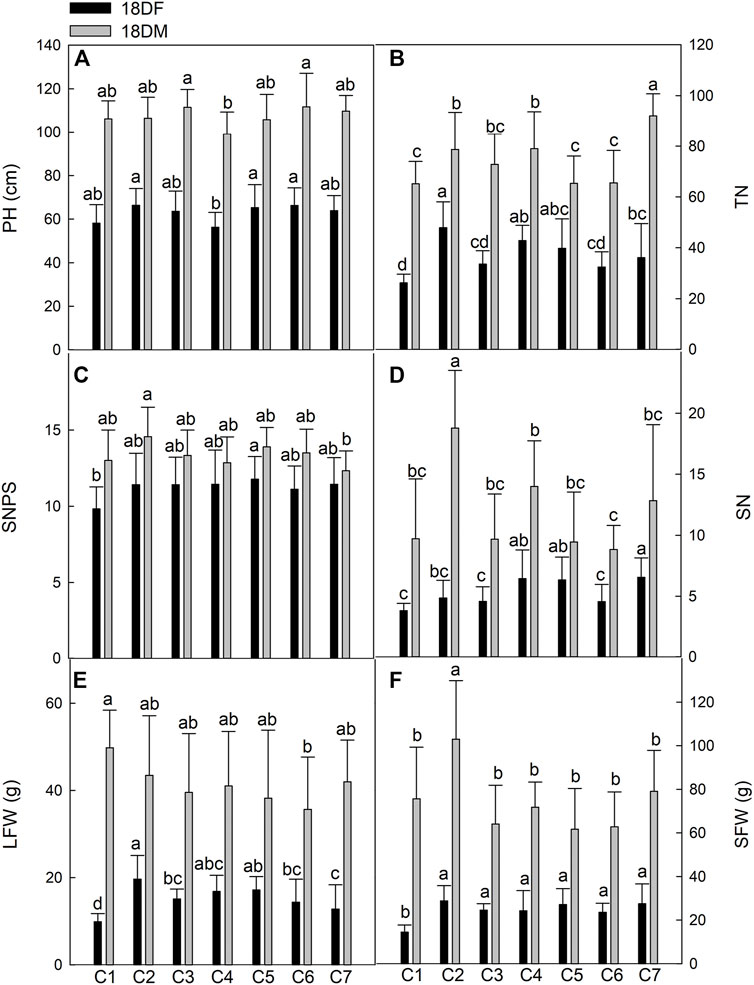
FIGURE 3. Comparison of main agricultural traits of seven Th. ponticum lines at Dongying station during different growth periods. (A) Plant height (PH). (B) Tiller number (TN). (C) Spikelet number per spike (SNPS). (D) Spike number (SN). (E) Leaf fresh weight (LFW). (F) Stem fresh weight (SFW). Different letters indicate a significant difference between seven Th. ponticum lines at p < .05 level.
In Dongying, the values for all of the measured indices increased as Th. ponticum plants matured. Line C2 had the highest PH (66.30 cm) at the flowering stage, whereas line C6 had the highest PH (111.68 cm) at the milk-ripe stage. The C4 plants were the shortest at the flowering and milk-ripe stages (56.27 and 99.11 cm, respectively). The TN of C2 (47.84) was significantly higher (p < .05) than that of C1, C3, C6, and C7 in 18DF, whereas the TN of C7 (92.00) was significantly higher (p < .05) than that of any other line in 18DM. With the exception of C1, all lines had an SNPS greater than 11 in 18DF, with no significant differences between lines. SNPS (11.78) of C5 was the highest in 18DF, whereas SNPS (14.56) of C2 was the highest in 18DM, significantly higher (p < .05) than that of C7 (12.33). However, SN was significantly higher (p < .05) for C7 (6.56) than for C1, C2, C3, and C6 at the flowering stage, whereas SN was significantly higher (p < .05) for C2 (18.78) than for any other line at the milk-ripe stage. LFW of C2 (19.63 g) was significantly higher (p < .05) than that of C1, C3, C6, and C7 in 18DF, whereas LFW of C1 (49.77 g) was the highest in 18DM, although there were no significant differences with the other lines, except for C6. Line C2 consistently had the highest SFW at both examined stages, with an average of 28.76 and 102.97 g at the flowering and milk-ripe stages, respectively. There were significant differences (p < .05) in SFW between C2 and the other lines at 18DM. In summary, PH, TN, LFW, and SFW of C2 were the highest at the flowering stage, whereas PH of C6; TN of C7; LFW of C1; and SN, SNPS, and SFW of C2 were the highest at the milk-ripe stage. Of the tested lines, C2 consistently grew the fastest. Line C1 initially grew slowly and then grew quickly, whereas C6 exhibited the opposite growth trend.
The total membership values were calculated based on the agronomic performance of the seven Th. ponticum lines in the four environments of 2018. There were substantial differences in the analyzed traits among the seven lines, which resulted in a wide range of TMV (Table 1). In Haixing, based on the size of TMV, the rank order (highest to lowest) of the membership functions was C2, C5, C4, C3, C1, C6, and C7 in 18HF, whereas it was C2, C1, C3, C4, C5, C6, and C7 in 18HM. In Dongying, the rank order (highest to lowest) of TMV was C2, C5, C7, C4, C3, C6, and C1 at the flowering stage, whereas it was C2, C7, C1, C4, C3, C6, and C5 at the milk-ripe stage. Regardless of the condition, C2 grew better than the other lines at the adult-plant stage. Its scores (.89 in 18HF and .97 in 18HM) were far higher than those of the second-best line (.64 in 18HF and .46 in 18HM) under high salt stress. Line C7 ranked third (.72 in 18DF) and second (.53 in 18DM) in Dongying but exhibited the poorest growth in Haixing, suggesting it is appropriate for soil with a salt content less than .3% (i.e., it is sensitive to high salinity).
Nutrient Indices
The results of the analysis of eight nutrient indices in Th. ponticum lines are provided in Table 2. There were no significant differences among the lines for the following six indices: DM (39.17%–42.85%FW), CP (13.15%–14.45%DM), NDF (53.21%–56.30%DM), ADF (28.18%–30.24%DM), EE (3.42%–3.86%DM), and WSC (1.88%–2.39%DM). However, there were significant variations in TC and the ash content. Specifically, TC was significantly higher (p < .05) in C2 (2.29 mg/g FW) than in any other line. The ash content was the highest in C5 (9.75%DM) and then C1 (9.69%DM), whereas it was the lowest in C6 (8.46%DM).
The NDF and ADF data were used to calculate the RFV, TDN, and RFQ of the Th. ponticum lines to assess the forage quality and value. There were no significant differences in the RFV (108–118), TDN (59–62), and RFQ (103–113). In short, nutrient indices expressed no significant differences among the lines except for TC and ash.
Physiological and Biochemical Indices
To compare the physiological and biochemical indices of the different lines exposed to saline conditions, osmotic regulatory compounds and protective enzymes were analyzed in the leaves at the milk-ripe stage. The leaf Pro content was .54–.69 μg/ml (Figure 4), with no significant differences among the Th. ponticum lines. There were also no significant differences in the Chl a/b ratio. However, the Chl a/b ratio was the highest for C6 (2.62) and then C2 (2.59), whereas it was the lowest for C5 and C7 (2.26). The CAT activity of C1, C2, C6, and C7 were higher levels in these materials, and there was no significant difference between these lines. The SOD activity was the highest in C7 and C2, with no significant difference. In general, Th. ponticum can maintain normal physiological metabolism under salt stress by increasing its main osmotic regulation substances, especially line C2.
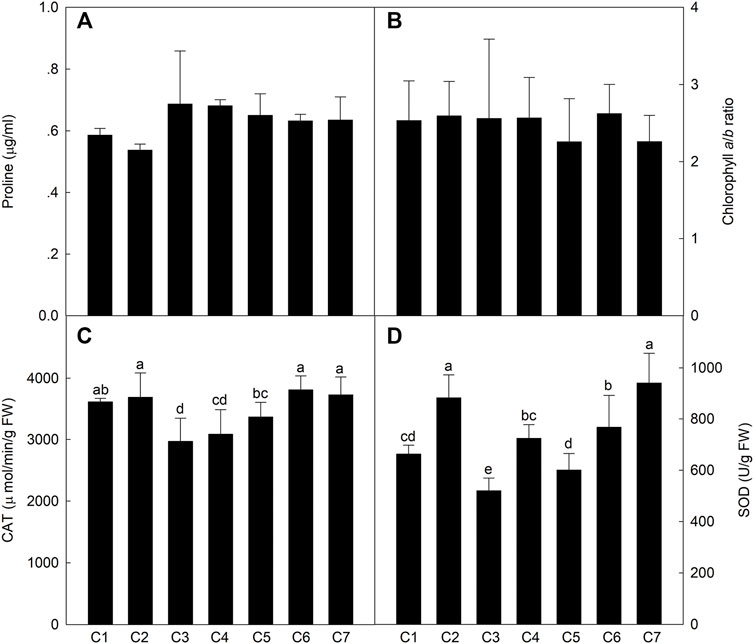
FIGURE 4. Comparison of the content of proline (A), chlorophyll a/b ratio (B), activities of CAT (C), and SOD (D) in the seven Th. ponticum lines. Different letters indicate a significant difference between seven Th. ponticum lines at p < .05 level.
Discussion
A Comprehensive Analysis Is Necessary to Assess Forage Salt Tolerance
Plant salt tolerance is a comprehensive trait involving multiple signaling pathways (Strizhov et al., 1997; Gohari et al., 2021). Because of the decrease in water potential and increase in osmotic pressure associated with saline soils, plant growth and development are seriously hindered by a decrease in the absorption of water by the roots, the closing of stomata, an increase in the leaf temperature, the inhibited differentiation of tissues and organs, and delayed cells growth. Salt stress can also severely affect plant physiological processes (Munns and Tester, 2008; Roy et al., 2014). Two new salt-tolerant bread wheat cultivars (“Maycan” and “Yildiz”) have significantly higher Chl a, Chl b, and total chlorophyll contents than salt-sensitive wheat varieties under saline conditions (Aycan et al., 2021). In response to salt stress, plants accumulate small organic compounds, such as Pro, to resist the adverse effects of stress while also synthesizing antioxidant enzymes to scavenge excess reactive oxygen species. As the core enzymes in the antioxidant system, SOD and CAT can minimize plant damage caused by reactive oxygen species by degrading hydrogen peroxide under saline conditions (Sekmen et al., 2007). Gohari et al. (2021) determined that, in grapevine, SOD and CAT activities increased following exposure to salinity stress. Additionally, Pro is an important component under salt treatment of plants, whose function may protect protein turnover normally and upregulate stress-protective proteins in the desert plant Pancratium maritimum L. (Khedr et al., 2003). Kim et al. (2016) performed Pro analyses of 46 switchgrass under salt stress, and the result revealed that salt-sensitive lines increased sharply. However, salt-resistant lines increased slightly in Pro concentration in response to salt treatment. In our study, C2 had the lowest Pro content, stable biomass advantage, and relatively high CAT and SOD activities and Chl a/b ratio, suggesting it may be better able to maintain normal metabolic and physiological activities under salt stress than the other lines.
Because plant salt tolerance varies among growth stages, it is difficult to accurately assess using a single index (An et al., 2021). To the best of our knowledge, herbage salt tolerance is currently evaluated primarily on the basis of the biomass, K+ and Na+ contents, and the relative water content during the germination or seedling stage (Johnson, 1991; Sagers et al., 2017). There has been relatively little research on herbage salt tolerance at the adult-plant stage, especially during the flowering and mike-ripe stages. To evaluate plant salt tolerance, a comprehensive analysis of morphological, physiological, biochemical, and other indices should be conducted. In the current study, the agronomic performance of C2 was generally the best among the Th. ponticum lines transplanted in coastal saline soil (.3% or .5% salinity). In Haixing, TN, SN, LFW, and SFW were the highest for C2 at two sampling stages. In Dongying, SNPS, SN, and SFW were the highest for C2 at the milk-ripe stage. A membership function analysis revealed that C2 exhibited better overall growth and produced more seeds than the other examined lines in both experimental fields. Additionally, STFW and STDW were higher for C2 than for the other six tall wheatgrass materials in six different environments, implying that C2 has a greater production potential under salt stress conditions than the other lines.
Importance of the Nutrient Content for Evaluating Forage Species
The nutrient content directly affects animal feed intake and forage palatability. The nutritional value of herbage is generally evaluated according to CP and the crude fiber (CF) content. Van Soest et al. (1991) divided CF into NDF, ADF, and acid detergent lignin, whose contents can directly affect animal feed intake and digestibility. For example, sheep feed intake decreased as NDF and ADF of perennial ryegrass (Lolium perenne) increased (Aitchison et al., 1986). Moreover, a high NDF in silage corn can decrease beef cattle feed intake (Tjardes et al., 2002). The CP content, which comprises protein and non-protein nitrogenous compounds, represents the essential materials for animal tissues, cells, and metabolism. An examination of the forage yield and quality traits of a collection of 50 tall wheatgrass accessions from the USDA-ARS Western Regional Plant Introduction Station demonstrated that the shoot CP ranged from 6.6 to 11% at the heading stage (Vogel and Moore, 1998). Additionally, dynamic changes were observed in forage grass CP during different growth periods. On the basis of their data for 17 Th. ponticum accessions from Iran, Jafari et al. (2014) revealed the average CP contents were 7.96 and 4.86% for two and one cutting managements, respectively. In the current study, the average NDF and ADF of seven tall wheatgrass lines were 54.89%DM and 29.24%DM, respectively. Furthermore, the CP of each line, which exceeded 13%DM, accounted for a higher percentage of the DM than the CP of Poa annua L. (9.93%) (Qu and Shi, 2017), silage corn (7.68%), and other gramineous forage species (Liu et al., 2018). Thus, Th. ponticum lines are useful as high-quality protein feed.
An increase in NDF and a decrease in CP will lead to an increase in TC. Some studies indicated that the bitter taste associated with a tannin DM greater than 1% would substantially decrease plant palatability. However, when TC is less than 1%, the accumulation of tannin may contribute to an increase in protein conversions and prevent ruminal tympany among herbivores (Barry and McNabb, 1999). In the present study, the TC of C2 (2.29 mg/g FW, equivalent to .45%DM) was within the relative permissible TC range and was significantly higher (p < .05) than the TC of the other lines, implying that C2 is a palatable line beneficial for nutrient metabolism and the prevention of bloating.
Hay quality is mainly assessed via a comprehensive analysis that divides the total digestible nutrients of forage materials on the basis of detergent fiber and DMI. As indices used for evaluating quality, TDN, RFV, and RFQ are positively correlated with forage palatability and digestibility (Rohweder et al., 1978). Xiong et al. (2018) analyzed the nutrient content of Avena sativa L. in different regions in China and determined that the TDN, RFV, and RFQ of A. sativa were, respectively, 52, 93, and 88 in Shanxi province and 51, 86, and 81 in Gansu province. In this study, the average TDN, RFV, and RFQ values of Th. ponticum were 60.41, 112.31, and 107.61, respectively, which were higher than the corresponding values for A. sativa. The WSC and EE values of Th. ponticum were 2.09%DM and 3.56%DM, respectively, representing a relatively large proportion of DM. The EE of this study was greater than the EE reported for some gramineous species, including oat (2.65%), Setaria viridis (L.) Beauv (2.11%), and Sorghum–sudangrass hybrids (1.26%) (Liu et al., 2018). These findings suggest that Th. ponticum growing in the Circum-Bohai sea region is a forage grass species with a high nutritive value, with implications for animal husbandry.
Decaploid Tall Wheatgrass Can Accelerate the Improvement of Coastal Saline Soils
Elytrigia (now named Thinopyrum), which is a genus in the gramineous wheat subfamily, includes the following species: Elytrigia repens, Elytrigia smithii, Elytrigia trichophora, Elytrigia elongata, and Elytrigia intermedia (Geng, 1959). Many studies have confirmed the differential salt tolerance of Elytrigia varieties (Dewey, 1960; Shannon, 1978). Th. ponticum, which is usually referred to as decaploid tall wheatgrass, has been used as a wild relative for the genetic improvement of wheat stress resistance (Li et al., 2008; Wang, 2011).
There are only a few reports that describe Th. ponticum as a high-quality pasture crop with strong tolerance to stress conditions. Although 25 Th. ponticum materials were selected and compared as early as in 1960 (Dewey, 1960), relatively few Th. ponticum varieties are available for production (Vogel and Moore, 1998). To date, eight Th. ponticum varieties (Jose, Largo, Alkar, Nebraska98526, Orbit, Platte, NFTW6001, and Plainsmen) have been developed and released in the United States and Canada (Alderson and Sharp, 1994; Trammell et al., 2016; Trammell et al., 2021). Two salt-tolerant varieties (Tyrrell and Dundas) were bred in Australia (Rogers and Bailey, 1963; Smith and Kelman, 2000). In China, Th. ponticum was initially transported from the United States to the Tianshui Experimental Station of Soil and Water Conservation (Gansu province). Professor Zhensheng Li brought it to the Laboratory of Genetics and Plant Breeding of CAS in Beijing in 1954 and then to Northwest Institute of Botany (Yangling, Shaanxi province) in 1956 to be used for wheat distant hybridizations. There are no decaploid tall wheatgrass varieties that have been certified in China, which has seriously restricted their use. The Yellow River Delta has many undeveloped land resources that are suitable for the cultivation of salt-tolerant herbage. Because of its excellent tolerance to salt, waterlogging, and drought stresses, Th. ponticum is an ideal forage species for improving saline coastal soils and developing animal husbandry. Line C2, which was identified as a superior germplasm in the present study, may be used to develop improved cultivars with elite traits resulting from the cross pollination between C2 individuals or between C2 and other appropriate lines with high regeneration ability and accelerate the application of its useful genes in improving wheat genetic variability by chromosome engineering. Furthermore, these materials would achieve the target of planting 667,000 ha salt-tolerant forage grass in saline and alkaline soils in the Circum-Bohai sea region.
Conclusion
On the basis of its high biomass and excellent agronomic performances revealed in this study, C2 is better suited for saline environments than the other examined tall wheatgrass lines. Thus, it may be relevant for optimizing the use of marginal soils and developing improved Th. ponticum cultivars that can be planted in the Circum-Bohai sea region of China.
Data Availability Statement
The raw data supporting the conclusion of this article will be made available by the authors without undue reservation.
Author Contributions
ZL and QZ conceived the research. CT and QZ performed the experiments. CT, GY, and QZ drafted the manuscript. AB, Tr, HL, and BL provided substantial help in preparing materials and filed experiments. All authors read and approved the final manuscript.
Funding
This research was supported by the Strategic Priority Research Program of the Chinese Academy of Sciences (no. XDA26040105), the Key Programs of the Chinese Academy of Sciences (KFZD-SW-112), and the STS Project of the Chinese Academy of Sciences (KFJ-STS-ZDTP-053).
Conflict of Interest
The authors declare that the research was conducted in the absence of any commercial or financial relationships that could be construed as a potential conflict of interest.
Publisher’s Note
All claims expressed in this article are solely those of the authors and do not necessarily represent those of their affiliated organizations, or those of the publisher, the editors, and the reviewers. Any product that may be evaluated in this article, or claim that may be made by its manufacturer, is not guaranteed or endorsed by the publisher.
References
Aitchison, E. M., Gill, M., Dhanoa, M. S., and Osbourn, D. F. (1986). The Effect of Digestibility and Forage Species on the Removal of Digesta from the Rumen and the Voluntary Intake of hay by Sheep. Br. J. Nutr. 56, 463–476. doi:10.1079/bjn19860126
Alderson, J., and Sharp, W. C. (1994). Grass Varieties in the United States. Washington, DC: U.S. Gov. Print. Office. USDA-SCS Agric. Handb. 170.
An, Y., Gao, Y., Tong, S., and Liu, B. (2021). Morphological and Physiological Traits Related to the Response and Adaption of Bolboschoenus Planiculmis Seedlings Grown under Salt-Alkaline Stress Conditions. Front. Plant Sci. 12, 567782. doi:10.3389/fpls.2021.567782
Arnon, D. I. (1949). Copper Enzymes in Isolated Chloroplasts. Polyphenoloxidase in Beta Vulgaris. Plant Physiol. 24, 1–15. doi:10.1104/pp.24.1.1
Aycan, M., Baslam, M., Asiloglu, R., Mitsui, T., and Yildiz, M. (2021). Development of New High-Salt Tolerant Bread Wheat (Triticum aestivum L.) Genotypes and Insight into the Tolerance Mechanisms. Plant Physiol. Biochem. 166, 314–327. doi:10.1016/j.plaphy.2021.05.041
Barry, T. N., and McNabb, W. C. (1999). The Implications of Condensed Tannins on the Nutritive Value of Temperate Forages Fed to Ruminants. Br. J. Nutr. 81, 263–272. doi:10.1017/S0007114599000501
Bates, L. S., Waldren, R. P., and Teare, I. D. (1973). Rapid Determination of Free Proline for Water-Stress Studies. Plant Soil 39, 205–207. doi:10.1007/BF00018060
Bazihizina, N., Barrett-Lennard, E. G., and Colmer, T. D. (2012). Plant Growth and Physiology under Heterogeneous Salinity. Plant Soil 354, 1–19. doi:10.1007/s11104-012-1193-8
Borrajo, C. I., Sánchez-Moreiras, A. M., and Reigosa, M. J. (2018). Morpho-physiological Responses of Tall Wheatgrass Populations to Different Levels of Water Stress. PLoS One 13, e0209281. doi:10.1371/journal.pone.0209281
Chen, Z., Jin, Y., Li, X., Wei, X., Li, C., White, J. F., et al. (2021). Characterization of the Complete Chloroplast Genome of Hordeum Jubatum (Poaceae: Pooideae: Triticeae) and Phylogenetic Analysis. Mitochondrial DNA B 6, 2933–2935. doi:10.1080/23802359.2021.1972868
Colmer, T. D., Flowers, T. J., and Munns, R. (2006). Use of Wild Relatives to Improve Salt Tolerance in Wheat. J. Exp. Bot. 57, 1059–1078. doi:10.1093/jxb/erj124
Dewey, D. R. (1960). Salt Tolerance of Twenty‐five Strains of Agropyron 1. Agron.j. 52, 631–635. doi:10.2134/agronj1960.00021962005200110006x
Ding, Z., Liu, Y., Lou, Y., Jiang, M., Li, H., and Lü, X. (2021). How Soil Ion Stress and Type Influence the Flooding Adaptive Strategies of Phragmites Australis and Bolboschoenus Planiculmis in Temperate saline-alkaline Wetlands? Sci. Total Environ. 771, 144654. doi:10.1016/j.scitotenv.2020.144654
Dvorak, J., Edge, M., and Ross, K. (1988). On the Evolution of the Adaptation of Lophopyrum Elongatum to Growth in saline Environments. Proc. Natl. Acad. Sci. 85, 3805–3809. doi:10.1073/pnas.85.11.3805
FAO (2017). FAO Soils Portal. Available at: https://www.fao.org/soils-portal/soil-management/management-of-some-problem-soils/salt-affected-soils/more-information-on-salt-affected-soils/en/, Accessed October 2021.
Forster, B. P., Miller, T. E., and Law, C. N. (1988). Salt Tolerance of Two Wheat - Agropyron Junceum Disomic Addition Lines. Genome 30, 559–564. doi:10.1139/g88-094
García-Triana, A., Zenteno-Savín, T., Peregrino-Uriarte, A. B., and Yepiz-Plascencia, G. (2010). Hypoxia, Reoxygenation and Cytosolic Manganese Superoxide Dismutase (cMnSOD) Silencing in Litopenaeus Vannamei: Effects on cMnSOD Transcripts, Superoxide Dismutase Activity and Superoxide Anion Production Capacity. Dev. Comp. Immunol. 34, 1230–1235. doi:10.1016/j.dci.2010.06.018
Geng, Y. L. (1959). Flora Illustralis Plantarum Primarum Sinicarum, Gramineae. Beijing: Science Press, 342–409.
Gohari, G., Panahirad, S., Sepehri, N., Akbari, A., Zahedi, S. M., Jafari, H., et al. (2021). Enhanced Tolerance to Salinity Stress in grapevine Plants through Application of Carbon Quantum Dots Functionalized by Proline. Environ. Sci. Pollut. Res. 28, 42877–42890. doi:10.1007/s11356-021-13794-w
Guo, Q., Meng, L., Mao, P.-C., and Tian, X.-X. (2015). Salt Tolerance in Two Tall Wheatgrass Species Is Associated with Selective Capacity for K+ over Na+. Acta Physiol. Plant 37, 1708. doi:10.1007/s11738-014-1708-4
Jafari, A. A., Elmi, A., and Bakhtiari, M. (2014). Evaluation of Yield and Quality Traits in 17 Populations of Tall Wheatgrass (Agropyron Elongatum) Grown in Rain Fed Area of Iran, under Two Cutting Management. Rom. Agric. Res. 31, 49–58.
Jenkins, S., Barrett-Lennard, E. G., and Rengel, Z. (2010). Impacts of Waterlogging and Salinity on Puccinellia (Puccinellia Ciliata) and Tall Wheatgrass (Thinopyrum Ponticum): Zonation on Saltland with a Shallow Water-Table, Plant Growth, and Na+ and K+ Concentrations in the Leaves. Plant soil 329, 91–104. doi:10.1007/s11104-009-0137-4
Jia, C. L., Sheng, Y. B., Zhang, H. W., Zhao, F. T., Wang, G. L., Bi, Y. B., et al. (2013). Comparisons on Forage Yield and Feeding Value of Sweet Sorghum in saline Soil of Yellow River Delta. Pratacult. Sci. 30, 116–119.
Johnson, R. C. (1991). Salinity Resistance, Water Relations, and Salt Content of Crested and Tall Wheatgrass Accessions. Crop Sci. 31, 730–734. doi:10.2135/cropsci1991.0011183X003100030039x
Khedr, A. H. A., Abbas, M. A., Wahid, A. A., Quick, W. P., and Abogadallah, G. M. (2003). Proline Induces the Expression of Salt-Stress-Responsive Proteins and May Improve the Adaptation of Pancratium Maritimum L. To Salt-Stress. J. Exp. Bot. 54, 2553–2562. doi:10.1093/jxb/erg277
Kim, J., Liu, Y., Zhang, X., Zhao, B., and Childs, K. L. (2016). Analysis of Salt-Induced Physiological and Proline Changes in 46 Switchgrass (Panicum Virgatum) Lines Indicates Multiple Response Modes. Plant Physiol. Biochem. 105, 203–212. doi:10.1016/j.plaphy.2016.04.020
King, I. P., Forster, B. P., Law, C. C., Cant, K. A., Orford, S. E., Gorham, J., et al. (1997). Introgression of Salt-Tolerance Genes from Thinopyrum Bessarabicum into Wheat. New Phytol. 137, 75–81. doi:10.1046/j.1469-8137.1997.00828.x
Li, Z., Li, B., and Tong, Y. (2008). The Contribution of Distant Hybridization with Decaploid Agropyron Elongatum to Wheat Improvement in China. J. Genet. Genomics 35, 451–456. doi:10.1016/S1673-8527(08)60062-4
Li, H. W., Zheng, Q., Li, B., Zhao, M. L., and Li, Z. S. (2022). Progress on Research of Tall Wheatgrass as a Kind of Salt Tolerant Forage Grass. Acta Pratacult. Sin. (in press). doi:10.11686/cyxb2021384
Liu, D. L., Qiu, W. W., and Ma, J. J. (2009). A Comparative Study on Salt Tolerance during Germinating Period of Different Alfalfa Varieties. Pratacult. Sci. 6, 163–169.
Liu, M., Yang, D., Zhang, J., Li, D. M., Wang, J., and Jiang, H. (2018). Determination of GI Value of Sheep Roughage in Bayannur City of Inner Mongolia. Anim. Husb. Feed Sci. 39, 51–54.
Mlejnkova, V., Horky, P., Kominkova, M., Skladanka, J., Hodulikova, L., Adam, V., et al. (2016). Biogenic Amines and Hygienic Quality of lucerne Silage. Open Life Sci. 11, 280–286. doi:10.1515/biol-2016-0037
Mullan, D. J., Mirzaghaderi, G., Walker, E., Colmer, T. D., and Francki, M. G. (2009). Development of Wheat-Lophopyrum Elongatum Recombinant Lines for Enhanced Sodium 'exclusion' during Salinity Stress. Theor. Appl. Genet. 119, 1313–1323. doi:10.1007/s00122-009-1136-9
Munns, R., and Tester, M. (2008). Mechanisms of Salinity Tolerance. Annu. Rev. Plant Biol. 59, 651–681. doi:10.1146/annurev.arplant.59.032607.092911
Omielan, J. A., Epstein, E., and Dvořák, J. (1991). Salt Tolerance and Ionic Relations of Wheat as Affected by Individual Chromosomes of Salt-Tolerant Lophopyrum Elongatum. Genome 34, 961–974. doi:10.1139/g91-149
Peng, Z., Wang, M., Li, F., Lv, H., Li, C., and Xia, G. (2009). A Proteomic Study of the Response to Salinity and Drought Stress in an Introgression Strain of Bread Wheat. Mol. Cell Proteomics 8, 2676–2686. doi:10.1074/mcp.M900052-MCP200
Pimentel, D., Allen, J., Beers, A., Guinand, L., Linder, R., McLaughlin, P., et al. (1987). World Agriculture and Soil Erosion. BioScience 37, 277–283. doi:10.2307/1310591
Qadir, M., and Oster, J. (2004). Crop and Irrigation Management Strategies for saline-sodic Soils and Waters Aimed at Environmentally Sustainable Agriculture. Sci. Total Environ. 323, 1–19. doi:10.1016/j.scitotenv.2003.10.012
Qu, Y. J., and Shi, S. L. (2017). Nutritional Evaluation of New Lines of Poa Pratensis Cv. Longmu. Grassl. Turf. 37, 51–60.
Rogers, A., and Bailey, E. (1963). Salt Tolerance Trials with Forage Plants in South-Western Australia. Aust. J. Exp. Agric. 3, 125–130. doi:10.1071/ea9630125
Rohweder, D. A., Barnes, R. F., and Jorgensen, N. (1978). Proposed hay Grading Standards Based on Laboratory Analyses for Evaluating Quality. J. Anim. Sci. 47, 747–759. doi:10.2527/jas1978.473747x
Roy, S. J., Negrão, S., and Tester, M. (2014). Salt Resistant Crop Plants. Curr. Opin. Biotechnol. 26, 115–124. doi:10.1016/j.copbio.2013.12.004
Ruf, T., and Emmerling, C. (2018). Site-adapted Production of Bioenergy Feedstocks on Poorly Drained Cropland through the Cultivation of Perennial Crops. A Feasibility Study on Biomass Yield and Biochemical Methane Potential. Biomass Bioenergy 119, 429–435. doi:10.1016/j.biombioe.2018.10.007
Sagers, J. K., Waldron, B. L., Creech, J. E., Mott, I. W., and Bugbee, B. (2017). Salinity Tolerance of Three Competing Rangeland Plant Species: Studies in Hydroponic Culture. Ecol. Evol. 7, 10916–10929. doi:10.1002/ece3.3607
Schacht, W. H., Volesky, J. D., Stephenson, M. B., Klopfenstein, T. J., and Adams, D. C. (2010). Plant and Animal Responses to Grazing Systems in the Nebraska Sandhills. Nebr. Beef Cattle Rep. 125, 36–37.
Sekmen, A. H., Türkan, İ., and Takio, S. (2007). Differential Responses of Antioxidative Enzymes and Lipid Peroxidation to Salt Stress in Salt-Tolerant Plantago Maritima and Salt-Sensitive Plantago media. Physiol. Plant 131, 399–411. doi:10.1111/j.1399-3054.2007.00970.x
Shannon, M. C. (1978). Testing Salt Tolerance Variability Among Tall Wheatgrass Lines 1. Agron.j. 70, 719–722. doi:10.1016/0304-3746(78)90022-710.2134/agronj1978.00021962007000050006x
Sharma, H. C., Ohm, H. W., Lister, R. M., Foster, J. E., and Shukle, R. H. (1989). Response of Wheatgrasses and Wheat × Wheatgrass Hybrids to Barley Yellow dwarf Virus. Theoret. Appl. Genet. 77, 369–374. doi:10.1007/BF00305830
Sima, Y.-H., Yao, J.-M., Hou, Y.-S., Wang, L., and Zhao, L.-C. (2011). Variations of Hydrogen Peroxide and Catalase Expression in Bombyx Eggs during Diapause Initiation and Termination. Arch. Insect Biochem. Physiol. 77, 72–80. doi:10.1002/arch.20422
Smith, K. F., and Kelman, W. M. (2000). Register of Australian Herbage Plant Cultivars Thinopyrum Ponticum (Podp.) (Tall Wheatgrass) Cv. Dundas. Aust. J. Exp. Agric. 40, 119–120. doi:10.1071/EA99156_CU
Strizhov, N., Ábrahám, E., Ökrész, L., Blickling, S., Zilberstein, A., Schell, J., et al. (1997). Differential Expression of Two P5CS Genes Controlling Proline Accumulation during Salt‐stress Requires ABA and Is Regulated by ABA1, ABI1 and AXR2 in Arabidopsis. Plant J. 12, 557–569. doi:10.1111/j.0960-7412.1997.00557.x
Tjardes, K. E., Buskirk, D. D., Allen, M. S., Tempelman, R. J., Bourquin, L. D., and Rust, S. R. (2002). Neutral Detergent Fiber Concentration in Corn Silage Influences Dry Matter Intake, Diet Digestibility, and Performance of Angus and Holstein Steers2. J. Anim. Sci. 80, 841–846. doi:10.2527/2002.803841x
Trammell, M. A., Butler, T. J., Word, K. M., Hopkins, A. A., and Brummer, E. C. (2016). Registration of NFTW6001 Tall Wheatgrass Germplasm. J. Plant Registrations 10, 166–170. doi:10.3198/jpr2015.09.0053crg
Trammell, M. A., Hopkins, A. A., Butler, T. J., and Walker, D. (2021). Registration of 'Plainsmen' Tall Wheatgrass. J. Plant Regist. 15, 415–421. doi:10.1002/plr2.20142
Van Soest, P. J., Robertson, J. B., and Lewis, B. A. (1991). Methods for Dietary Fiber, Neutral Detergent Fiber, and Nonstarch Polysaccharides in Relation to Animal Nutrition. J. Dairy Sci. 74, 3583–3597. doi:10.3168/jds.S0022-0302(91)78551-2
Vogel, K. P., and Moore, K. J. (1998). Forage Yield and Quality of Tall Wheatgrass Accessions in the USDA Germplasm Collection. Crop Sci. 38, 509–512. doi:10.2135/cropsci1998.0011183X003800020039x
Wang, P., Yin, L. J., and Li, J. D. (1994). Studies on the Adaptability and Tolerance of Leymus Chinensis to Salinity in Salinized Grassland in the Songnen Plain. Acta Ecol. Sin. 14, 306–311.
Wang, X. J., Hou, L., Li, G. H., Zhao, C. Z., Zhao, S. Z., and Xia, H. (2020). New Models of High-Efficient Utilization of the Yellow River Delta saline-alkali Land. Shandong Agric. Sci. 52, 128–135.
Wang, R. R.-C. (2011). “Agropyron and Psathyrostachys,” in Wild Crop Relatives: Genomic and Breeding Resources. Editor C. Kole (Berlin, Heidelberg: Springer), 77–108. doi:10.1007/978-3-642-14228-4_2
Weimberg, R., and Shannon, M. C. (1988). Vigor and Salt Tolerance in 3 Lines of Tall Wheatgrass. Physiol. Plant 73, 232–237. doi:10.1111/j.1399-3054.1988.tb00591.x
Xie, H., Li, J., Zhang, Y., Xu, X., Wang, L., and Ouyang, Z. (2021). Evaluation of Coastal Farming under Salinization and Optimized Fertilization Strategies in China. Sci. Total Environ. 797, 149038. doi:10.1016/j.scitotenv.2021.149038
Xiong, Y., Xu, Q. F., Yu, Z., Zhou, Q., Ye, Z. S., Ou, X., et al. (2018). Evaluation of Nutritional and Feeding Value of Oat hay from Different Regions. Pratacult. Sci. 35, 2457–2462.
Xu, H. X., Zhang, X., Wang, S. M., Yan, P., and Dou, J. Z. (2008). Genetic Diversity of Achnatherum Splendens. Agric. Sci. Technol. 36, 6223–6224.
Yang, S. (1999). Feed Analysis and Quality Test Technology. Beijing: Beijing Agricultural University Press.
Yuan, W.-Y., and Tomita, M. (2015). Thinopyrum Ponticum Chromatin-Integrated Wheat Genome Shows Salt-Tolerance at Germination Stage. Ijms 16, 4512–4517. doi:10.3390/ijms16034512
Zhang, J. K., Lu, D. X., Liu, J. X., Bao, S. N., Zou, Q. H., and Liu, Q. H. (2004). The Present Research Situation and Progress of Crude Fodder Quality Evaluation index. Pratacul. Sci. 21, 55–61.
Zhang, H., Xiang, Y., Irving, L. J., Li, Q., and Zhou, D. (2018). Nitrogen Addition Can Improve Seedling Establishment of N-Sensitive Species in Degraded saline Soils. Land Degrad. Dev. 30, 119–127. doi:10.1002/ldr.3195
Zhang, Q., Liu, J., Liu, X., Li, S., Sun, Y., Lu, W., et al. (2020). Optimizing Water and Phosphorus Management to Improve hay Yield and Water‐ and Phosphorus‐use Efficiency in Alfalfa under Drip Irrigation. Food Sci. Nutr. 8, 2406–2418. doi:10.1002/fsn3.1530
Zhang, L. Y. (2007). Feed Analysis and Quality Test Technology. Beijing: China Agricultural University Press.
Glossary
ADF acid detergent fiber
CAT catalase
CF crude fiber
Chl a chlorophyll a
Chl b chlorophyll b
CP crude protein
DDM digestible dry matter
DM dry matter
DMI dry matter intake
EE ether extract
LFW leaf fresh weight
NDF neutral detergent fiber
PH plant height
Pro proline
RFQ relative forage quality
RFV relative feeding value
SFW stem fresh weight
SN spike number
SNPS spikelet number per spike
SOD superoxide dismutase
STDW shoot total dry weight
STFW shoot total fresh weight
TC tannin content
TDN total digestible nutrient
TMV total membership value
TN tiller number
WSC water-soluble carbohydrate
18DF 2018 Dongying, flowering stage
18DM 2018 Dongying, milk-ripe stage
18HF 2018 Haixing, flowering stage
18HM 2018 Haixing, milk-ripe stage
19DF 2019 Dongying, flowering stage
19DM 2019 Dongying, milk-ripe stage
Keywords: Thinopyrum ponticum, salt tolerance, adult-plant stage, agronomic traits, physiological index, nutrient content
Citation: Tong C, Yang G, AoenBolige , Terigen , Li H, Li B, Li Z and Zheng Q (2022) Screening of Salt-Tolerant Thinopyrum ponticum Under Two Coastal Region Salinity Stress Levels. Front. Genet. 13:832013. doi: 10.3389/fgene.2022.832013
Received: 09 December 2021; Accepted: 10 January 2022;
Published: 04 February 2022.
Edited by:
Pengtao Ma, Yantai University, ChinaCopyright © 2022 Tong, Yang, AoenBolige, Terigen, Li, Li, Li and Zheng. This is an open-access article distributed under the terms of the Creative Commons Attribution License (CC BY). The use, distribution or reproduction in other forums is permitted, provided the original author(s) and the copyright owner(s) are credited and that the original publication in this journal is cited, in accordance with accepted academic practice. No use, distribution or reproduction is permitted which does not comply with these terms.
*Correspondence: Qi Zheng, cXpoZW5nQGdlbmV0aWNzLmFjLmNu