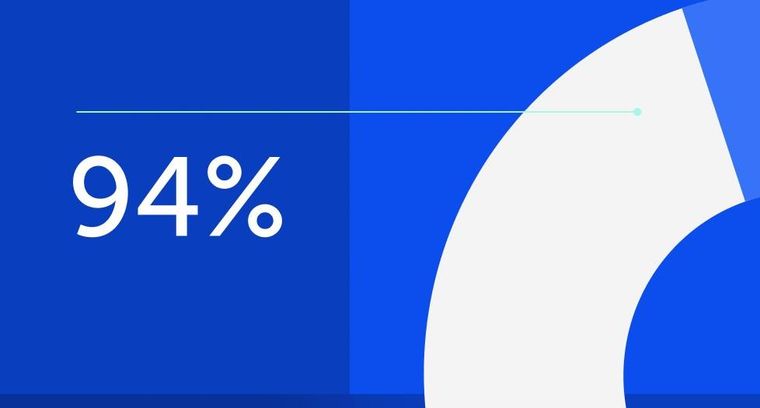
94% of researchers rate our articles as excellent or good
Learn more about the work of our research integrity team to safeguard the quality of each article we publish.
Find out more
BRIEF RESEARCH REPORT article
Front. Genet., 28 April 2022
Sec. Human and Medical Genomics
Volume 13 - 2022 | https://doi.org/10.3389/fgene.2022.813934
In 2014, 157 years after the Sepoy Mutiny of 1857, several unidentified human skeletons were discovered in an abandoned well at Ajnala, Punjab. The most prevailing hypothesis suggested them as Indian soldiers who mutinied during the Indian uprising of 1857. However, there is an intense debate on their geographic affinity. Therefore, to pinpoint their area of origin, we have successfully isolated DNA from cementum-rich material of 50 good-quality random teeth samples and analyzed mtDNA haplogroups. In addition to that, we analyzed 85 individuals for oxygen isotopes (δ18O values). The mtDNA haplogroup distribution and clustering pattern rejected the local ancestry and indicated their genetic link with the populations living east of Punjab. In addition, the oxygen isotope analysis (δ18O values) from archaeological skeletal remains corroborated the molecular data and suggested the closest possible geographical affinity of these skeletal remains toward the eastern part of India, largely covering the Gangetic plain region. The data generated from this study are expected to expand our understanding of the ancestry and population affinity of martyr soldiers.
Mitochondrial DNA (mtDNA) has provided forensic scientists a valuable tool for determining the genetic origin of damaged, degraded, or very limited biological samples. Due to the low DNA recovery from ancient remains, mtDNA is always the best choice to target as sequences from multi-copy loci have more chance to be detected in ancient remains than the single-copy sequences due to their presence in larger numbers per cell (Merheb et al., 2019). Furthermore, mtDNA is widely used to trace matrilineal ancestry as it passes from the mother to the offspring.
The nuclear DNA is either unavailable or highly degraded and insufficient in most forensic situations where only severely putrefied tissues, degraded bones, or teeth are available for identification purposes. The mtDNA analysis is the only option left with forensic anthropologists to attempt identification of such challenged human remains retrieved from forensic or bioarchaeological scenarios (Budowle et al., 2003; Rai et al., 2014; Chaubey et al., 2017; Harney et al., 2019). Each cell contains several hundreds and thousands of copies of mtDNA compared to only two copies of autosomal DNA, so the chances of recovery of mtDNA from badly damaged human osseous remains of forensic interest are increased manyfold (Holland et al., 1993; Lutz et al., 1996). Recent advances in ancient DNA (aDNA) analyses, facilitated by improved sequencing technologies, have helped the experts glean information about past human populations’ identity, genetic history, migration patterns, and admixtures (Haber et al., 2016; Shinde et al., 2019).
The survival and recovery of DNA from human hard tissues like bones and teeth has become an essential scientific tool in forensic anthropological investigations (Adler et al., 2011). Little is known about the quality and quantity of mtDNA extracted from the preserved teeth or bones, so there is a pressing need within forensic anthropology to conduct molecular studies on the human skeletal remains preserved at different stages in diverse conditions. Teeth and bones are morphologically and biochemically different (Dobberstein et al., 2008). No potential differences have been reported in the ancient DNA content within different dental tissues or regions (Shiroma et al., 2004; Alakoc and Aka, 2009). It is a small size and mineralized status of the tooth, which imparts toughness and reduced porosity to the dental enamel and cementum to ensure the safer protection of dental DNA from various environmental challenges and contaminations (Rudbeck et al., 2005; Sampietro et al., 2006; Adler et al., 2011). Tooth DNA is generally of higher quality and less prone to decomposition or decay than bone DNA (Ricaut et al., 2005; Gilbert et al., 2005). Molecular analysis of teeth samples has proven their reliability and suitability for molecular identification of unknown human remains recovered from disaster sites, mass graves, or crime scenes (Rohland and Hofreiter, 2007).
The physical methods of tooth powdering have potential influences on the quantity and quality of DNA extracted from it. Out of two commonly used powdering techniques (pulverizing or drilling), direct drilling of the tooth with a dental burr or Dremel bit is the most preferred technique, instead of the destructive technique of pulverizing and grinding the whole sample (Kolman and Tuross, 2000), as former technique retains the morphological features of the tooth for further forensic analyses (Brkic et al., 2000; O'Rourke et al., 2000). Although the speed and heat generated during drilling may degrade or denature the DNA structure (Lindahl, 1993), it has not been reported in human genetic forensic studies (Alakoc and Aka, 2009). The tooth type with the largest pulp chamber (i.e., molar) is the best source of DNA (De Leo et al., 2000). The amount and integrity of recovered DNA are considered a more critical issue when dealing with forensic archaeological teeth samples (Krings et al., 1997). Most ancient DNA studies (archaeological and forensic) to date have not utilized the most suitable skeletal material, namely, cementum-rich material at the tip of the tooth root, and have instead focused on dentine. Therefore, here we extracted DNA from cementum-rich material of 50 good-quality random sepoy teeth samples and analyzed mtDNA markers.
The discovery of human remains and artifacts from the past is akin to chancing upon a goldmine. It presents an opportunity to understand the past with some degree of certainty and clarity. The human remains bring new knowledge concerning the biological, cultural, historical, and geographical realities of man in the bygone era. An obscure textbook authored by a civil servant of the British East India Company and Deputy Commissioner of Amritsar in 1857 (Cooper, 1858; Crispin Bates, 2017) mentioned a mass burial site in an abandoned well lying underneath a religious structure at Ajnala (Amritsar, India) (Figure 1). It documented the capture, imprisonment, and eventual killings of 282 Indian soldiers of the 26th Native Bengal Infantry regiment of the British Indian army. In May 1857, the Indian soldiers revolted against the colonial ordeal for the enforced use of beef- and pork-greased cartridges which escalated to a larger scale and further spread to various cantonments. The written accounts mention that the 26th Native Bengal Infantry battalion stationed at the Mian-Meer cantonment had soldiers only from the Indian states of Bengal, Bihar, Uttar Pradesh (eastern), and some northeastern states. After killing some British officers, the mutineer soldiers fled from the cantonment, although 282 were captured near Ajnala, Punjab, and were killed, and their bodies were dumped in the nearby disused well. Sociopolitical sensitivity of the incident and the emergent sanitary concerns were cited as the most immediate reasons for their disposal burial in the said well at Ajnala (Cooper, 1858; East India Papers 1859; Bates and Carter, 2017). Unfortunately, the book reference did not receive the attention it deserved from the state authorities. However, in February 2014, some local amateur archaeologists and curiosity seekers took it on their own to unearth the said, reported remains. They did not employ any scientific excavation technique for their exhumation from the well sediments, and in the process, the brittle skeletal remains were severely damaged, fragmented, and commingled.
FIGURE 1. (A) Map of India showing study site Ajnala (red circle) from which the sepoy’s skeletal remains were excavated. (B) Abandoned deep well where a large number of skeletons were found. (C) Skulls and long bones collected from the abandoned well. (D) Mandibles with attached tooth samples.
Thus, the non-scientific excavation led to the retrieval of a large number of human remains along with some contextual items of identity such as coins, medals, bracelets, and rings. Two major hypotheses surround the origin of these skeletal materials: one hypothesis believes the remains belonged to the rebel soldiers as indicated in Cooper’s book (The Crisis in Punjab: From 10th of May until Fall of Delhi), East India Papers (1859), and Punjab Gazetteers. Some historians have ascribed the remains to the unfortunate mass killings perpetrated during the Indo-Pak partition conflicts of 1947. However, this claim stands dismissed by the engravings of images of Queen Victoria and the year of make on each coin and medal retrieved from the Ajnala skeletal assemblage as none of them has markings beyond 1856. Such claims were also refuted by the radiocarbon dates measured from some teeth collagen samples. Forensic anthropological analyses of these remains were undertaken to test the aforesaid theories regarding their origin. The commingled nature of Ajnala skeletal remains, the haphazard and diverse positioning of the skeletons in soil sediments, their taphonomic degradations, and non-scientific excavation have complicated their identification endeavors. Non-expert-mediated exhumation by the amateur excavators has further compromised the integrity of the already fragile and brittle remains. The government authorities’ delayed intervention led to the loss of forensic evidence of utmost national importance. Only teeth, jaw fragments, vertebrae, phalanges, skulls, femurs, clavicles, and hand and foot bones were retrieved among the skeletal debris; no pelvis, complete femur, sternum, etc. could be recovered from the remains (Sehrawat and Kaur, 2016; Sehrawat and Sankhyan, 2021). Thus, most analyses are based on the teeth preserved in a better state. Nonetheless, the first author of this article, to whom the remains were handed over, has cited the number of people as 246 based on dental counts, which is very close to the figure of 282 claimed in the written accounts (Sehrawat and Kaur, 2016). Through various forensic anthropological techniques, attempts have been made to examine the biological profile of the remains, bringing to light their identity parameters like age, sex, geographical origin, and dental health status. The analysis of the dental wears, caries, and hypoplasia has enabled the researchers to conclude their dental hygiene status, occupation, and dietary habits.
The preliminary analyses have supported the hypothesis that the remains belong to the mid-19th century as they are all adult men with good dental hygiene, indicating their military affiliations. There is historical and cultural evidence to support the geographic and temporal affiliations of Ajnala skeletal remains, which need to be scrutinized genetically/scientifically. Therefore, the present molecular and isotopic analyses were conducted to determine the local or non-local status of the recovered human remains, and whether the remains belonged to the reported geographical regions/states mentioned in the written records or not?
As the anthropological and odontological examinations provided some conflicting clues about the identity of the remains in question (Sehrawat JS 2016), 50 randomly selected molar teeth were subjected to mtDNA analyses and 85 samples for stable C and O isotope analyses. More than 165-year deposition of human remains in well sediments would influence the potential molecular profiling of the Ajnala skeletal remains. The data generated from this study are expected to expand our understanding of martyr soldiers’ ancestry and population affinity.
All mitochondrial DNA-related work was performed following strict aDNA standards in the clean laboratory of Birbal Sahni Institute of Palaeosciences, Lucknow, India. The outer surface of each tooth was cleaned manually with bleach to remove possible contaminants during excavation and split into two pieces, crown and root, using a sterile Dremel drill. The dentine and pulp were drilled out of the root using a fine drill bit, and the fine white powder was collected in sterile 2-ml Eppendorf tubes. aDNA extraction was done using an extraction buffer having 10 M urea, 0.5 M EDTA, and 10 µl of proteinase K (20 mg/ml), followed by incubation at 37°C for 24 h. After spinning down each tube at 4,000 rpm for 5 min, the pellet containing the cellular debris was discarded, and the supernatant containing the DNA was transferred to a 4-ml Amicon filter (Sigma-Aldrich®). It was performed to concentrate the samples with DNA since the volume was >1 ml. We brought the volume down to 250 ul by spinning the samples through the filters for 2–5 min. The supernatant was transferred to a 2-ml Eppendorf tube containing 5X PB binding buffer, that is, 5X 250 = 1.25 ml (Qiagen®). The PB–sample mix (for each tube separately) was transferred to MinElute spin columns (Qiagen®) and spun at 7,000 rpm for 1 min, and the eluate was discarded. A measure of 710 µl of PE wash buffer (Qiagen®) was added to the filters and spun at 10,000 rpm for 1 min. After discarding the supernatant, ethanol was used to wash off the salts, and each tube was spun empty at 14,000 rpm for a minute to remove any trace ethanol, followed by discarding the collection tube. The filters were placed in fresh 1.5-ml Eppendorf tubes, and 45 µl of EB elution buffer (Qiagen®) was added to the center of each filter. This was followed by incubation at 37°C for 15 min. After spinning the tube at 14,000 rpm for 1 min, an additional 30 μl EB buffer was added to the same filter (to recover any leftover bound DNA molecules), incubated for another 10 min at 37°C, and spun as before. The filter was finally discarded. This was followed for each tube.
The mtDNA genotyping experiment was performed on Sequenom’s MassARRAY platform, a powerful and flexible method for assaying up to a few thousand markers and up to thousands of individuals. It is based on distinguishing allele-specific primer extension products by mass spectrometry (MALDI-TOF). Most stages of the experimental protocol reflect adaptations of established PCR protocols to multiplexing, which allows the simultaneous amplification and detection of multiple markers per reaction.
In total, 115 mtDNA-specific diagnostic variations were carefully chosen to assign each sample to a specific haplogroup. We used a globally published mtDNA database (van Oven and Kayser, 2009) to select the diagnostic variations across human mtDNA. All the 115 polymorphic variations were amplified in four different pools, and genotyping was done on Sequenom iPLEX assay via the MassARRAY 28 system (SEQUENOM, San Diego, CA). This technology requires minimal input DNA 29 (pictogram scale) and is compatible with degraded, small-sized amplicons. Using this system, we designed four panels of amplification and extension primers, targeting 115 diagnostic mtDNA sites (23, 36, 31, and 25 sites, respectively). We performed multiplex PCR and genotyping in accordance with the manufacturer’s instructions. We report the genotyping results in Supplementary Data for the 50 samples from which we successfully extracted ancient DNA. We assigned mitochondrial haplogroups manually based on the combination of mutations (Supplementary Table S1).
All the haplogroups identified among the analyzed samples were pooled, and these data were considered a single population. Principal component analysis (PCA) was performed using POPSTR, which is provided by H. Harpending (Ahn et al., 2018). The state-wise frequency of haplogroup was obtained from the published sources (Metspalu et al., 2004; Chaubey et al., 2007; Chaubey et al., 2008; Thangaraj et al., 2008; Chaubey et al., 2011; Tamang et al., 2018).
The dentine powder of the selected tooth samples was collected in vials using a Dremel bit, and the powdered samples were kept in airtight centrifuge tubes to prevent any contamination. The organic and inorganic fractions were separated using standardized techniques. Approximately ∼ 500 μg samples were put in 12-ml vials and kept at 72°C in a Gas Bench tray and then flushed with ultrapure helium gas (≥99.9995%) to remove all atmospheric gasses from the vials. Further 50–70 μl phosphoric acid (≥99%) was added to the vials. All the samples were kept at 72°C for 45 min to equilibrate and produce CO2 gas. The produced CO2 was introduced into the IRMS through the GC column to analyze their isotopic ratio, and the isotopic ratios of carbon (δ13C) and oxygen (δ18O) were obtained using the SSH correction (Santrock et al., 1985). The stable isotopic composition of tooth samples were measured at the Stable Isotope Facility at the University of California, Davis, West Sacramento (United States) using a gas source continuous flow isotope ratio mass spectrometer (CF-IRMS) through Gas Bench.
We randomly selected 50 sepoy samples collected from Ajnala, Punjab, and considered them a single population. We classified them into their respective haplogroup based on their mutation combination (Supplementary Table S1). In the sepoy group, at least thirteen haplogroups have been observed. Majority of the samples fell in the macrohaplogroup M (0.7). Moreover, with the current methodology used, we could classify a large number of samples at the sub-haplogroup level (Supplementary Table S1, S2). The west Eurasian-specific haplogroups observed were HV0e (0.06), U3 (0.02), and U7 (0.02). Among the haplogroups observed, the most frequent haplogroup was M39 (0.24), followed by R32 (0.14). These haplogroups are autochthonous to South Asia (Sun et al., 2006; Chaubey et al., 2008; Chandrasekar et al., 2009). Haplogroup M39 is widespread in South Asia; however, R32 is sporadic with a substantial presence in Gangetic plain populations (Supplementary Table S2).
It is well documented that the west Eurasian genetic component follows a clinal pattern in South Asia (Metspalu et al., 2004; Chaubey et al., 2007). Therefore, to pinpoint the genetic relatedness of the sepoy samples from Ajnala, Punjab, we first classified the observed haplogroups to their regional affiliations (Figure 2A). Populations native to Punjab and adjoining regions carried significantly higher West Eurasian maternal ancestry than the populations of Gangetic plain (two-tailed p-value < 0.005) (Figure 2A). The regional haplogroup spectrum of sepoy samples was more akin to the Gangetic plain populations, rather than local Punjabi populations. These results suggest their closer genetic affinity with the Gangetic plain populations, rather than the local Punjabi populations.
FIGURE 2. (A) Pie chart showing the proportion of the East Eurasian, West Eurasian, and South Asian genetic ancestries among the studied group (Ajnala, India), with respect to their adjacent geographical regions. South Asian haplogroups: M2-M6, M18, M25, M30-M67, N5, R5-R8, R30-R32, and U2a,b; west Eurasian haplogroups: HV, H, J, K, R0, R1, R2, U1-U5, U7, U8, U9, W, and X; east Eurasian haplogroups: A-G, M7-M12, R22, and N9. (B) Principal component analysis (PCA) on maternal haplogroups of South Asian populations, showing the affinity of sepoy samples with South Asian populations. Pak, Pakistan; Pun, Punjab India; Aj, Ajnala (sepoy samples); Raj, Rajasthan; Guj, Gujarat; Mah, Maharashtra; Up, Uttar Pradesh; Bih, Bihar; Jhk, Jharkhand; WB, West Bengal; Mp, Madhya Pradesh; Cg, Chhattisgarh; Odi, Odisha; Ap, Andhra Pradesh; Ker, Kerala.
To better understand the genetic affiliation of our studied group, we have implemented principal component analysis (PCA) comparing its mtDNA profile with a dataset of haplogroup frequencies from Pakistani and Indian populations (Supplementary Table S2). We hypothesized that the placement of the sepoy samples in the PC plot would be closer to the states of their closest genetic similarity. We also observed a similar result in our PCA, where Indian populations were mainly distributed into two geographical clines. The sepoy group showed a genetic affinity with the Bihar, Uttar Pradesh, West Bengal, and Jharkhand state populations (Figure 2B). These observations placed their likely homeland near the north and east Indian geographic regions, mainly populated at the Gangetic plain.
Isotopic studies have been widely used to examine diet and provenance in archaeological studies. The mean δ18O values for molar teeth samples analyzed in the present study are 24.5 ± 0.6‰ and 24.2 ± 1‰ (VSMOW), with values ranging from 23.3 to 25.8‰ (n = 42) and 21.4 to 26.0‰ (n = 46), respectively. Both the sites are characterized by the δ18O values larger than the range of ∼2‰. The δ13C values in the maxillary sites range from −12.5 to −4.6‰ and −13 to −1.7‰.
The δ18O value in meteoric water varies regionally according to temperature and other climatic parameters, such as distance from the coastline, altitude, and latitude (Dansgaard, 1964; Rozanski et al., 2013). The δ18O values in the body are subject to several steps of metabolic fractionation. The fractionation mechanisms are relatively well known, allowing the calculation of approximate drinking water (δ18Ow) values from the δ18Oc of biogenic carbonate using conversion equations (Ehleringer et al., 2010; Posey, 2011). Although there are complexities in the calculation of meteoric water isotope composition in the past, the oxygen isotope composition of human remains has allowed for the identification of palaeomobility patterns. Oxygen isotope is usually used as a proxy for meteoric drinking water in mammals due to the direct correlation between δ18O of local meteoric drinking water, body water, and δ18O structural carbonate with subtle variations in fractionation according to species and climate variables (D’Angela and Longinelli, 1990; Daniel Bryant and Froelich, 1995; Daux et al., 2008; Kohn, 1996; Levinson et al., 1987; Longinelli, 1984; Luz and Kolodny, 1985; Luz et al., 1984). For this study, we used the equations of (Posey, 2011) (δ18Oc = 0.77*δ18Odw + 28.1‰) to calculate the δ18O values of drinking water. Using relationships between oxygen isotopes ratios of tooth carbonate (δ18Oc) and local precipitation (δ18Ow), precipitation δ18Ow values for the samples were calculated, which ranged from −3.0 to −6.2‰ to −2.7 to −8.8‰, respectively. Overall, the majority of δ18O values in the studied tooth samples fit “non-local people” to the excavation site and point to locations in the Gangetic plain (Uttar Pradesh) and coastal areas (like Orissa) (Figure 3). However, the lower δ18O values in the few samples suggest a small percentage of the local population (Figure 3).
FIGURE 3. Map showing the spatial distribution of oxygen isotope ratio of Ganga and Indus River system (circles) and oxygen isotope ratios in groundwater (triangles) (Gupta et al., 2005; Karim, 2002).
The δ13C value of the carbonate associated with mammalian bioapatite is related to diet, is preserved on archaeological and geological time scales, and is widely used for reconstructing dietary preferences and availability of different food resources to mammals (Ambrose and Norr, 1993; Luz et al., 1990; Passey and Cerling, 2002; Passey et al., 2005a; Passey et al., 2005b; Stevens et al., 2006). It has been shown that the bioapatite carbonate is enriched in 13C by several per mil (9–15‰) relative to diet (Tieszen and Fagre, 1993; Cerling and Harris, 1999). Such enrichment in 13C is likely the result of fractionation during the precipitation of bioapatite minerals from dissolved inorganic carbon within the body. Therefore, the δ13C values of human tooth enamel reflect the diet, which varies with intake of relative proportion of C3 vs. C4 plant and intake of meat in the regional context.
The δ13C values of tooth samples range from −12.5 to −4.6‰ and −13 to −1.7‰, respectively, which, in turn, reveal dominant C3 to mixed C3–C4 diet patterns. Very high δ13C in two samples (HF-1 and F4) suggests a chief C4 diet. In the Indian context, the diet is rich in a mixture of C3 (legumes) and C4 (millets). The δ13C results from archaeo enamel samples from these two sites revealed that the dietary pattern matched with the modern human population from India with an admixture of C3 and C4.
Thus, in the present study, we could analyze DNA from human skeletal remains and stable isotope data to reconstruct the geographical origin of human skeletal remains excavated from an abandoned well for the first time. Analysis of two independent techniques (genetics and stable isotope) adds significant study novelty. Consequently, stable isotope and mtDNA analyses are consistent with the historical evidence, stating that the 26th Native Bengal Infantry battalion comprised Bengal, Odisha, Bihar, and eastern parts of Uttar Pradesh. Thus, the current research can uncover the hidden aspects of the struggle of the unknown martyrs against the colonial yoke. If reviewed in light of the findings of this study, the historical data and literature will further corroborate the incidence of this massacre and add another chapter in the annals of Indian history dedicated to the unsung heroes of India’s first freedom struggle. Our results expect to lay the groundwork and make a rich contribution to similar forensic investigations in the future for exploring the biological profile of remains even if recovered in mangled and damaged shape.
The datasets presented in this study can be found in online repositories. The names of the repository/repositories and accession number(s) can be found in the article/Supplementary Material.
Ethical clearance has been obtained from Institutional Ethics Committee of Panjab University, Chandigarh (India) vide letter no. PUEIC/2018/99/A/09/01 dated: 28.01.2018 for carrying out research on the material used in present study.
NR, JS, and KT directed the work; SP, SK, RR, MS, and DS did the laboratory work; SA, NR, JS, GC, and SK analyzed the data; and NR, GC and JS wrote the manuscript with the help of KT and SA.
The financial supports provided to JS by SERB-DST, New Delhi, in the form of “Core Research Grant” (Animal Sciences) (vide Grant No SERB/F/4790/2018-2019), helped in conceptualization, experimentation, and designing of the present study.
The authors declare that the research was conducted in the absence of any commercial or financial relationships that could be construed as a potential conflict of interest.
All claims expressed in this article are solely those of the authors and do not necessarily represent those of their affiliated organizations, or those of the publisher, the editors, and the reviewers. Any product that may be evaluated in this article, or claim that may be made by its manufacturer, is not guaranteed or endorsed by the publisher.
The corresponding author (NR) is highly thankful to Birbal Sahni Institute of Paleosciences, Lucknow (India), for providing facilities for experimental work with the material presented in this article. The first author (JS) is highly indebted to Punjab govt. for entrusting him toward biological profiling of unknown human remains excavated from an abandoned well at Ajnala (India). GC is supported by the ICMR India (grant number-2021-6389) and University Faculty IOE grant (6031). KT was supported by the J.C. Bose Fellowship (GAP0542) from SERB, DST, Government of India.
The Supplementary Material for this article can be found online at: https://www.frontiersin.org/articles/10.3389/fgene.2022.813934/full#supplementary-material
Adler, P. B., Seabloom, E. W., Borer, E. T., Hillebrand, H., Hautier, Y., Hector, A., et al. (2011). Productivity Is a Poor Predictor of Plant Species Richness. Science 333 (6050), 1750–1753. doi:10.1126/science.1204498
Ahn, J., Conkright, B., Boca, S. M., and Madhavan, S. (2018). POPSTR: Inference of Admixed Population Structure Based on Single-Nucleotide Polymorphisms and Copy Number Variations. J. Comput. Biol. 25 (4), 417–429. doi:10.1089/cmb.2017.0127
Alakoç, Y. D., and Aka, P. S. (2009). "Orthograde Entrance Technique" to Recover DNA from Ancient Teeth Preserving the Physical Structure. Forensic Sci. Int. 188 (1-3), 96–98. doi:10.1016/j.forsciint.2009.03.020
Ambrose, S. H., and Norr, L. (1993). “Experimental Evidence for the Relationship of the Carbon Isotope Ratios of Whole Diet and Dietary Protein to Those of Bone Collagen and Carbonate,” in Prehistoric Human Bone: Archaeology at the Molecular Level. Editors J. B. Lambert, and G. Grupe (Springer Berlin Heidelberg), 1–37. doi:10.1007/978-3-662-02894-0_1
Brkic, H., Strinovic, D., Kubat, M., and Petrovecki, V. (2000). Odontological Identification of Human Remains from Mass Graves in Croatia. Int. J. Leg. Med 114 (1), 19–22. doi:10.1007/s004149900130
Budowle, B., Schutzer, S. E., Einseln, A., Kelley, L. C., Walsh, A. C., Smith, J. A. L., et al. (2003). Building Microbial Forensics as a Response to Bioterrorism. Science 301 (5641), 1852–1853. doi:10.1126/science.1090083
Cerling, T. E., and Harris, J. M. (1999). Carbon Isotope Fractionation between Diet and Bioapatite in Ungulate Mammals and Implications for Ecological and Paleoecological Studies. Oecologia 120 (3), 347–363. doi:10.1007/s004420050868
Chaubey, G., Ayub, Q., Rai, N., Prakash, S., Mushrif-Tripathy, V., Mezzavilla, M., et al. (2017). "Like Sugar in Milk": Reconstructing the Genetic History of the Parsi Population. Genome Biol. 18 (1), 110. doi:10.1186/s13059-017-1244-9
Chaubey, G., Karmin, M., Metspalu, E., Metspalu, M., Selvi-Rani, D., Singh, V. K., et al. (2008). Phylogeography of mtDNA Haplogroup R7 in the Indian peninsula. BMC Evol. Biol. 8, 227. doi:10.1186/1471-2148-8-227
Chaubey, G., Metspalu, M., Choi, Y., Magi, R., Romero, I. G., Soares, P., et al. (2011). Population Genetic Structure in Indian Austroasiatic Speakers: the Role of Landscape Barriers and Sex-specific Admixture. Mol. Biol. Evol. 28 (2), 1013–1024. doi:10.1093/molbev/msq288
Chaubey, G., Metspalu, M., Kivisild, T., and Villems, R. (2007). Peopling of South Asia: Investigating the Caste-Tribe Continuum in India. Bioessays 29 (1), 91–100. doi:10.1002/bies.20525
Cooper, F. H. (1858). The Crisis in the Punjab : From the 10th of May until the Fall of Delhi. SmithElder and Co.
Crispin Bates, M. C. (2017). “Mutiny at the Margins: New Perspectives on the Indian Uprising of 1857,” in Documents of the Indian Uprising (SAGE Publications Pvt Ltd), 7. doi:10.4135/9789353287726
D'Angela, D., and Longinelli, A. (1990). Oxygen Isotopes in Living Mammal's Bone Phosphate: Further Results. Chem. Geology. Isotope Geosci. section 86 (1), 75–82. doi:10.1016/0168-9622(90)90007-y
Daniel Bryant, J., and Froelich, P. N. (1995). A Model of Oxygen Isotope Fractionation in Body Water of Large Mammals. Geochimica et Cosmochimica Acta 59 (21), 4523–4537. doi:10.1016/0016-7037(95)00250-4
Dansgaard, W. (1964). Stable Isotopes in Precipitation. Tellus 16 (4), 436–468. doi:10.3402/tellusa.v16i4.8993
Daux, V., Lécuyer, C., Héran, M.-A., Amiot, R., Simon, L., Fourel, F., et al. (2008). Oxygen Isotope Fractionation between Human Phosphate and Water Revisited. J. Hum. Evol. 55 (6), 1138–1147. doi:10.1016/j.jhevol.2008.06.006
De Leo, D., Turrina, S., and Marigo, M. (2000). Effects of Individual Dental Factors on Genomic DNA Analysis. Am. J. Forensic Med. Pathol. 21 (4), 411–415. doi:10.1097/00000433-200012000-00023
Dobberstein, R. C., Huppertz, J., von Wurmb-Schwark, N., and Ritz-Timme, S. (2008). Degradation of Biomolecules in Artificially and Naturally Aged Teeth: Implications for Age Estimation Based on Aspartic Acid Racemization and DNA Analysis. Forensic Sci. Int. 179 (2-3), 181–191. doi:10.1016/j.forsciint.2008.05.017
Ehleringer, J. R., Thompson, A. H., Podlesak, D. W., Bowen, G. J., Chesson, L. A., Cerling, T. E., et al. (2010). A Framework for the Incorporation of Isotopes and Isoscapes in Geospatial Forensic Investigations. Isoscapes, 357–387. doi:10.1007/978-90-481-3354-3_17
Haber, M., Mezzavilla, M., Bergström, A., Prado-Martinez, J., Hallast, P., Saif-Ali, R., et al. (2016). Chad Genetic Diversity Reveals an African History Marked by Multiple Holocene Eurasian Migrations. Am. J. Hum. Genet. 99 (6), 1316–1324. doi:10.1016/j.ajhg.2016.10.012
Harney, É., Nayak, A., Patterson, N., Joglekar, P., Mushrif-Tripathy, V., Mallick, S., et al. (2019). Ancient DNA from the Skeletons of Roopkund Lake Reveals Mediterranean Migrants in India. Nat. Commun. 10 (1), 3670. doi:10.1038/s41467-019-11357-9
Holland, M. M., Fisher, D. L., Mitchell, L. G., Rodriquez, W. C., Canik, J. J., Merril, C. R., et al. (1993). Mitochondrial DNA Sequence Analysis of Human Skeletal Remains: Identification of Remains from the Vietnam War. J. Forensic Sci. 38 (3), 542–553. doi:10.1520/jfs13439j
Kohn, M. J. (1996). Predicting Animal δ18O: Accounting for Diet and Physiological Adaptation. Geochimica et Cosmochimica Acta 60 (23), 4811–4829. doi:10.1016/s0016-7037(96)00240-2
Kolman, C. J., and Tuross, N. (2000). Ancient DNA Analysis of Human Populations. Am. J. Phys. Anthropol. 111 (1), 5–23. doi:10.1002/(SICI)1096-8644(200001)111:1<5::AID-AJPA2>3.0.CO;2-3-
Krings, M., Stone, A., Schmitz, R. W., Krainitzki, H., Stoneking, M., and Pääbo, S. (1997). Neandertal DNA Sequences and the Origin of Modern Humans. Cell 90 (1), 19–30. doi:10.1016/s0092-8674(00)80310-4
Levinson, A. A., Luz, B., and Kolodny, Y. (1987). Variations in Oxygen Isotopic Compositions of Human Teeth and Urinary Stones. Appl. Geochem. 2 (4), 367–371. doi:10.1016/0883-2927(87)90021-7
Lindahl, T. (1993). Instability and Decay of the Primary Structure of DNA. Nature 362 (6422), 709–715. doi:10.1038/362709a0
Longinelli, A. (1984). Oxygen Isotopes in Mammal Bone Phosphate: A New Tool for Paleohydrological and Paleoclimatological Research? Geochimica et Cosmochimica Acta 48 (2), 385–390. doi:10.1016/0016-7037(84)90259-x
Lutz, C. S., Murthy, K. G., Schek, N., O'Connor, J. P., Manley, J. L., and Alwine, J. C. (1996). Interaction between the U1 snRNP-A Protein and the 160-kD Subunit of Cleavage-Polyadenylation Specificity Factor Increases Polyadenylation Efficiency In Vitro. Genes Dev. 10 (3), 325–337. doi:10.1101/gad.10.3.325
Luz, B., Cormie, A. B., and Schwarcz, H. P. (1990). Oxygen Isotope Variations in Phosphate of Deer Bones. Geochimica et Cosmochimica Acta 54 (6), 1723–1728. doi:10.1016/0016-7037(90)90403-8
Luz, B., Kolodny, Y., and Horowitz, M. (1984). Fractionation of Oxygen Isotopes between Mammalian Bone-Phosphate and Environmental Drinking Water. Geochimica et Cosmochimica Acta 48 (8), 1689–1693. doi:10.1016/0016-7037(84)90338-7
Luz, B., and Kolodny, Y. (1985). Oxygen Isotope Variations in Phosphate of Biogenic Apatites, IV. Mammal Teeth and Bones. Earth Planet. Sci. Lett. 75 (1), 29–36. doi:10.1016/0012-821x(85)90047-0
Merheb, M., Matar, R., Hodeify, R., Siddiqui, S. S., Vazhappilly, C. G., Marton, J., et al. (2019). Mitochondrial DNA, a Powerful Tool to Decipher Ancient Human Civilization from Domestication to Music, and to Uncover Historical Murder Cases. Cells 8 (5). doi:10.3390/cells8050433
Metspalu, M., Kivisild, T., Metspalu, E., Parik, J., Hudjashov, G., Kaldma, K., et al. (2004). Most of the Extant mtDNA Boundaries in South and Southwest Asia Were Likely Shaped during the Initial Settlement of Eurasia by Anatomically Modern Humans. BMC Genet. 5, 26. doi:10.1186/1471-2156-5-26
O'Rourke, D. H., Hayes, M. G., and Carlyle, S. W. (2000). Ancient DNA Studies in Physical Anthropology. Annu. Rev. Anthropol. 29, 217–242. doi:10.1146/annurev.anthro.29.1.217
Passey, B. H., Cerling, T. E., Schuster, G. T., Robinson, T. F., Roeder, B. L., and Krueger, S. K. (2005b). Inverse Methods for Estimating Primary Input Signals from Time-Averaged Isotope Profiles. Geochimica et Cosmochimica Acta 69 (16), 4101–4116. doi:10.1016/j.gca.2004.12.002
Passey, B. H., and Cerling, T. E. (2002). Tooth Enamel Mineralization in Ungulates: Implications for Recovering a Primary Isotopic Time-Series. Geochimica et Cosmochimica Acta 66, 3225–3234. doi:10.1016/s0016-7037(02)00933-x
Passey, B. H., Robinson, T. F., Ayliffe, L. K., Cerling, T. E., Sponheimer, M., Dearing, M. D., et al. (2005a). Carbon Isotope Fractionation between Diet, Breath CO2, and Bioapatite in Different Mammals. J. Archaeological Sci. 32, 1459–1470. doi:10.1016/j.jas.2005.03.015
Posey, R. (2011). Development and Validation of a Spatial Prediction Model for Forensic Geographical Provenancing of Human Remains.
Rai, N., Taher, N., Singh, M., Chaubey, G., Jha, A. N., Singh, L., et al. (2014). Relic Excavated in Western India Is Probably of Georgian Queen Ketevan. Mitochondrion 14 (1), 1–6. doi:10.1016/j.mito.2013.12.002
Ricaut, F.-X., Keyser-Tracqui, C., Crubézy, E., and Ludes, B. (2005). STR-genotyping from Human Medieval Tooth and Bone Samples. Forensic Sci. Int. 151 (1), 31–35. doi:10.1016/j.forsciint.2004.07.001
Rohland, N., and Hofreiter, M. (2007). Comparison and Optimization of Ancient DNA Extraction. Biotechniques 42 (3), 343–352. doi:10.2144/000112383
Rozanski, K., Araguás-Araguás, L., and Gonfiantini, R. (2013). Isotopic Patterns in Modern Global Precipitation, 1–36. doi:10.1029/GM078p0001
Rudbeck, L., Gilbert, M. T. P., Willerslev, E., Hansen, A. J., Lynnerup, N., Christensen, T., et al. (2005). mtDNA Analysis of Human Remains from an Early Danish Christian Cemetery. Am. J. Phys. Anthropol. 128 (2), 424–429. doi:10.1002/ajpa.20294
Sampietro, D. A., Vattuone, M. A., and Isla, M. I. (2006). Plant Growth Inhibitors Isolated from Sugarcane (Saccharum Officinarum) Straw. J. Plant Physiol. 163 (8), 837–846. doi:10.1016/j.jplph.2005.08.002
Santrock, J., Studley, S. A., and Hayes, J. M. (1985). Isotopic Analyses Based on the Mass Spectra of Carbon Dioxide. Anal. Chem. 57 (7), 1444–1448. doi:10.1021/ac00284a060
Sehrawat, J. S., and Sankhyan, D. (2021). Forensic Anthropology in Investigations of Crimes against Humanity: Global Dimensions and the Mid-19th-Century Ajnala (India) Massacre. Forensic Sci. Rev. 33 (1), 37–65. https://www.ncbi.nlm.nih.gov/pubmed/33518514.
Sehrawat, P. R. K., and Kaur, J. (2016). Human Remains from Ajnala, India, 2014 [Short Fieldwork Reports]. Bioarchaeology of the Near East 10, 9.
Shinde, V., Narasimhan, V. M., Rohland, N., Mallick, S., Mah, M., Lipson, M., et al. (2019). An Ancient Harappan Genome Lacks Ancestry from Steppe Pastoralists or Iranian Farmers. Cell 179 (3), 729–735. e710. doi:10.1016/j.cell.2019.08.048
Shiroma, C. Y., Fielding, C. G., Lewis, J. A., Gleisner, M. R., and Dunn, K. N. (2004). A Minimally Destructive Technique for Sampling Dentin Powder for Mitochondrial DNA Testing. J. Forensic Sci. 49 (4), 791–795. doi:10.1520/jfs2003432
Stevens, R. E., Lister, A. M., and Hedges, R. E. M. (2006). Predicting Diet, Trophic Level and Palaeoecology from Bone Stable Isotope Analysis: A Comparative Study of Five Red Deer Populations. Oecologia 149, 12–21. doi:10.1007/s00442-006-0416-1
Tamang, R., Chaubey, G., Nandan, A., Govindaraj, P., Singh, V. K., Rai, N., et al. (2018). Reconstructing the Demographic History of the Himalayan and Adjoining Populations. Hum. Genet. 137 (2), 129–139. doi:10.1007/s00439-018-1867-2
Thangaraj, K., Chaubey, G., Kivisild, T., Selvi Rani, D., Singh, V. K., Ismail, T., et al. (2008). Maternal Footprints of Southeast Asians in North India. Hum. Hered. 66 (1), 1–9. doi:10.1159/000114160
Thomas, P., Gilbert, M., Rudbeck, L., Willerslev, E., Hansen, A. J., Smith, C., et al. (2005). Biochemical and Physical Correlates of DNA Contamination in Archaeological Human Bones and Teeth Excavated at Matera, Italy. J. Archaeological Sci. 32 (5), 785–793.
Tieszen, L. L., and Fagre, T. (1993). Effect of Diet Quality and Composition on the Isotopic Composition of Respiratory CO2Bone Collagen, Bioapatite, and Soft Tissues, 121–155. doi:10.1007/978-3-662-02894-0_5
Keywords: ancient DNA, stable isotope, forensic, anthropology, mtDNA, human population genetics
Citation: Sehrawat J, Agrawal S, Sankhyan D, Singh M, Kumar S, Prakash S, Rajpal R, Chaubey G, Thangaraj K and Rai N (2022) Pinpointing the Geographic Origin of 165-Year-Old Human Skeletal Remains Found in Punjab, India: Evidence From Mitochondrial DNA and Stable Isotope Analysis. Front. Genet. 13:813934. doi: 10.3389/fgene.2022.813934
Received: 01 December 2021; Accepted: 24 March 2022;
Published: 28 April 2022.
Edited by:
Stephen J Bush, University of Oxford, United KingdomReviewed by:
Maria Golubenko, Tomsk NRMC, RussiaCopyright © 2022 Sehrawat, Agrawal, Sankhyan, Singh, Kumar, Prakash, Rajpal, Chaubey, Thangaraj and Rai. This is an open-access article distributed under the terms of the Creative Commons Attribution License (CC BY). The use, distribution or reproduction in other forums is permitted, provided the original author(s) and the copyright owner(s) are credited and that the original publication in this journal is cited, in accordance with accepted academic practice. No use, distribution or reproduction is permitted which does not comply with these terms.
*Correspondence: Kumarasamy Thangaraj, dGhhbmdzQGNjbWIucmVzLmlu; Niraj Rai, bmlyYWpyYWlAYnNpcC5yZXMuaW4=
†These authors have contributed equally to this work
Disclaimer: All claims expressed in this article are solely those of the authors and do not necessarily represent those of their affiliated organizations, or those of the publisher, the editors and the reviewers. Any product that may be evaluated in this article or claim that may be made by its manufacturer is not guaranteed or endorsed by the publisher.
Research integrity at Frontiers
Learn more about the work of our research integrity team to safeguard the quality of each article we publish.