- 1Department of Forensic Medicine, Tongji Medical College of Huazhong University of Science and Technology, Wuhan, China
- 2Division of Thoracic Surgery, Union Hospital, Tongji Medical College of Huazhong University of Science and Technology, Wuhan, China
- 3Division of Cardiology, Departments of Internal Medicine and Genetic Diagnosis Center, Tongji Hospital, Tongji Medical College, Huazhong University of Science and Technology, Wuhan, China
- 4Division of Thoracic Surgery, Tongji Hospital, Tongji Medical College of Huazhong University of Science and Technology, Wuhan, China
Aortic dissection (AD) is a cardiovascular disease characterized by high mortality and poor prognosis. Although FBN1 is associated with syndromic AD, its association with non-syndromic AD remains unclear. In this study, DNA samples from 90 Chinese individuals with non-syndromic AD (60 Stanford A, 30 Stanford B types) were analyzed to determine the relationship between diverse genotypes of the FBN1 gene and non-syndromic AD. Eleven pathogenic/likely pathogenic variants (1 novel) were identified in 12.2% of patients with non-syndromic AD. Patients with positive variants suffered from AD at a younger age than those in the negative variant group. Among the six positive missense mutations associated with cysteine residue hosts, four (66.7%) were Stanford A AD, whereas two (33.3%) were Stanford B AD. Three (100%) positive splicing/truncation variant hosts were Stanford A AD. The splicing/truncation variants and missense variants involving cysteine residues in the FBN1 gene increased the risk of Stanford A AD. Ten common SNPs that increased susceptibility to AD were identified. In particular, five SNPs were detected significantly in Stanford A AD, whereas another four SNPs were significantly detected in Stanford B AD. These significant variants can function as biomarkers for the identification of patients at risk for AD. Our findings have the potential to broaden the database of positive mutations and common SNPs of FBN1 in non-syndromic AD among the Chinese population.
Introduction
Aortic dissection (AD) is usually asymptomatic until the aorta ruptures and has a high risk of mortality and poor prognosis (Li et al., 2017). Presenting with overlapping clinical manifestations, AD can occur in individuals with genetic syndromic diseases, such as Marfan syndrome (MFS, OMIM 154700) or Ehlers-Danlos syndrome (EDS, OMIM 130050), causing vascular dysfunction (Regalado et al., 2016). However, a vast majority of AD cases (approximately 80%) usually occur in non-syndromic forms, such as familial thoracic AD (fTAAD, OMIM 607086) and sporadic AD (Guo et al., 2011; Hoffjan, 2012; Goldfinger et al., 2014). Over the last 2 decades, numerous genes (e.g., FBN1, ACTA2, TGFBR1/2, and SMAD3) have been identified as predisposing humans to syndromic AD and fTAAD (Hoffjan, 2012; Zhang and Wang, 2016; Pinard et al., 2019).
FBN1 is a classic gene associated with MFS and encodes fibrillin-1 as a structural macromolecule that forms extracellular matrix (ECM) microfibrils (Sakai et al., 2016). Fibrillin microfibrils play many critical functional roles and serve as scaffolds for elastin deposition in the ECM (Fahrenbach et al., 1966). They are essential components for maintaining the structural integrity of both the aortic wall and the suspensory ligament of the eye lens and for regulating members of the transforming growth factor beta (TGFβ) family to sequester them to induce the formation and repair of organs (Sakai et al., 2016). Owing to these multiple functions, FBN1 variants have been associated with pleiotropic clinical phenotypes. Moreover, some studies have suggested that variants in different FBN1 regions are related to different phenotypes. However, the spectrum of known FBN1 variants remains incomplete, and the association between FBN1 and non-syndromic AD is unclear.
Based on the widely used Stanford system, dissections involving the ascending aorta are classified as Stanford A AD, and those involving only the descending aorta are classified as Stanford B AD (Gawinecka et al., 2017). Stanford A AD is a high-risk subtype and requires surgical intervention, with ∼50% of the patients succumbing to death before hospital admission, high mortality and morbidity in survivors after hospitalization, and emergency surgical repair (Howard et al., 2013). In addition, in our daily forensic practice, the majority of sudden death cases related to AD primarily include Stanford A AD. As some of the genes that increase the risk for AD have been identified, it is important to further investigate whether diverse genotypes of these genes may be linked to different types of AD (especially Stanford A AD), which would be useful in personalized preventive surgery. Therefore, samples from 90 Chinese Han individuals with non-syndromic AD were sequenced, not only to identify and report the frequency of FBN1 pathogenic/likely pathogenic variants in AD, but also to determine the correlation between common single nucleotide polymorphisms (SNPs) and AD. Furthermore, we explored possible genotypic differences between the two AD types. We also investigated the presence of a hotspot mutation region, according to the distribution characteristics of the common SNPs and pathogenic/likely pathogenic variants identified along the gene.
Materials and Methods
Study Subjects
Ninety individuals with non-syndromic AD were recruited from the Han Chinese population with non-syndromic AD. The diagnosis criteria of AD were confirmed using the patient medical history, computed tomography (CT) or magnetic resonance imaging (MRI), or pathological evidence of AD from postmortem autopsy. Patients with syndromic AD (such as MFS, EDS, Loeys-Dietz syndrome), aneurysm only, or traumatic AD, as well as those not of Han Chinese ethnicity were excluded. Peripheral blood was obtained from 79 in-hospital patients diagnosed with AD at several hospitals in Wuhan, China, between January 2016 and December 2020. Heart arterial blood was also collected from 11 patients who died from AD based on a precise diagnosis at the Department of Forensic Medicine, Tongji Medical College, Huazhong University of Science and Technology, Wuhan, China, from January 2016 to December 2020. Data were also collected from the medical and anatomical records. The control group consisted of 568 unrelated healthy (with no AD) Han Chinese ethnicity individuals from the Novo-Zhonghua project.
All sample collection procedures met the ethical guidelines of Tongji Medical College, Huazhong University of Science and Technology (approval number [2017] IEC (S059)). Consent forms were signed by the patients or legal relatives of the deceased.
Determination of Genotypes
Peripheral or heart blood samples extracted from each subject were stored in ethylenediaminetetraacetic acid (EDTA) tubes. Genomic DNA was extracted using a TIANamp Blood DNA Kit (TIANGEN, DP318). The FBN1 sequencing data of 90 individuals were obtained by next-generation sequencing on the Illumina HiSeq 4,000 platform. The coverage obtained was 50% on average, at a depth of 100×.
The obtained sequencing data were compared with the human reference genome (GRCh37/hg19) to sort out variants from the raw sequences, and the qualified variants were further annotated using ANNOVAR (Wang et al., 2010). Variants with a minor allele frequency (MAF) < 0.01 in all three databases (1000Genomes, ExAC, and ESP6500) were defined as rare variants. Variants were annotated and classified into five categories (pathogenic, likely pathogenic, uncertain significance, likely benign, and benign) according to the American College of Medical Genetics (ACMG) Guidelines Revisions (Richards et al., 2015). Both pathogenic and likely pathogenic variants were defined as positive variants. Variants with an MAF >0.05, in the 1,000 Genomes Project, ExAC, and ESP databases, were defined as common SNPs and were selected for analysis of associations within different phenotypes of AD.
Statistical Analysis
Continuous variables are presented as mean ± standard deviation, and all comparisons of mean values were performed using unpaired t-test or analysis of variance (ANOVA). Categorical data are presented as percentages or rates and were compared using the chi-square test or two-tailed Fisher’s exact test. Odds ratios (ORs) and associated 95% confidence intervals (CIs) were used to evaluate the association of each identified FBN1 common SNPs with AD susceptibility. A significant P-value corrected by the Bonferroni correction was used owing to multiple comparisons of the FBN1 common SNPs studied. Hardy–Weinberg equilibrium was evaluated using PowerStats v1.2 software to examine whether or not the samples showed group representation. Other statistical analyses were performed using SPSS version 25.0. All tests were two-sided and assessed at a significance level of p < 0.05.
Results
Clinical Characteristics
The study group consisted of 90 individuals diagnosed with non-syndromic AD. The demographic and clinical features of the study participants were summarized in Table 1. The mean age for the onset of AD was 48.76 ± 10.20 years, ranging from 21 to 72 years. Of these individuals, 68.9% (62/90) were males and 31.1% (28/90) were females; 60 patients (66.7%) had dissection involving the ascending aorta (Stanford A AD) and 30 (33.3%) had dissection involving only the descending aorta (Stanford B AD). In addition, 51 individuals (56.7%) presented with hypertension, 23 (25.6%) had a history of smoking, 14 (15.6%) had coronary atherosclerotic heart disease, and 8 (8.9%) presented with coronary artery dissection. The specific clinical and demographic information of the patients is presented in Supplementary Table S1.
General Information of Variants
A total of 125 variants of FBN1 were identified from the 90 individuals with AD, including 99 variants in introns, 22 variants in exons, two splicing variants, and two variants in the 5’ UTR area. The distribution of genotypes was consistent with Hardy–Weinberg equilibrium (p > 0.12) in the 90 AD patients included in the study.
There were 44 rare variants (MAF <0.01). Among these, five pathogenic and six likely pathogenic variants (for a total of 11 positive variants) were identified. All 11 positive variants were heterozygous and absent in the healthy control group. Detailed genetic information of these 11 positive variants was provided in Table 2. Among these, 10 positive variants were reported to be associated with AD in the ClinVar database or HMGD, and one was novel (not previously reported), suggesting that similar pathogenic mechanisms are shared among different types of AD. The genetic diagnostic yield of FBN1 positive variants was 12.2% (11/90) in the experimental group, 11.7% (7/60) in Stanford A AD, and 13.3% (4/30) in Stanford B AD, with no significant difference, according to type.
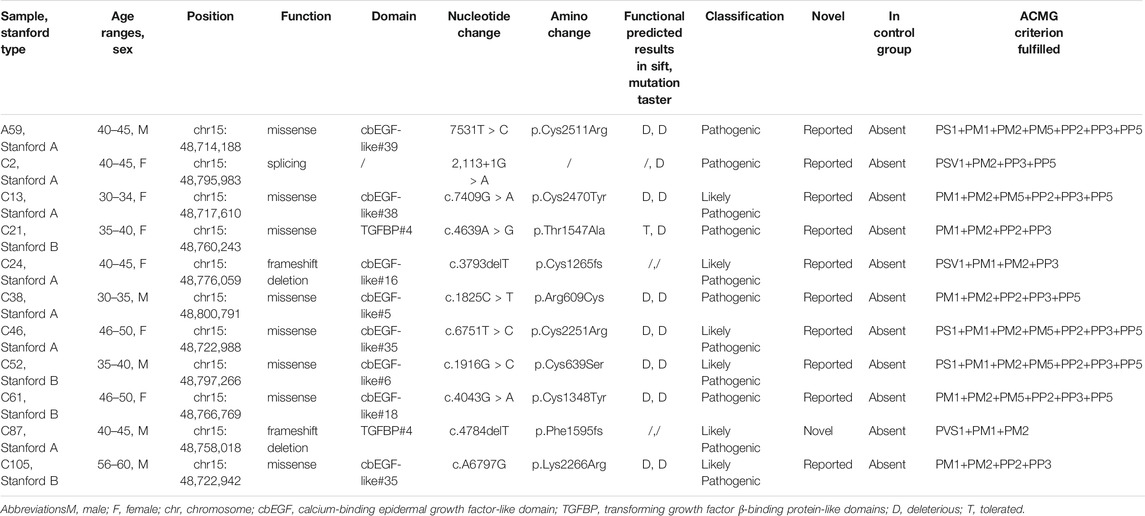
TABLE 2. Detailed genetic and clinical information of AD patients harboring positive variants in FBN1.
Details of the 11 Pathogenic/Likely Pathogenic Variants
All the 11 positive variants were unique to a single patient; that is, the same pathogenic or likely pathogenic variants were never linked to more than one individual in our study. Of these, eight (72.7%) were missense variants, two were frameshift deletions, and one was a splicing variant. Six of the eight missense variants (75%) involved cysteine residues: five unique missense variants involved substitution of a cysteine, and one missense variant created a new cysteine (p.Cys2511Arg in A59, p.Cys2470Try in C13, p.Arg609Cys in C38, p.Cys2251Arg in C46, p.Cys639Ser in C52, and p.Cys1348Try in C61). Specifically, in Stanford A AD, there were four missense variants, two frameshifts, and one splicing variant, whereas in Stanford B AD, there were four missense variants. Interestingly, four missense variants in Stanford A AD were all linked to cysteine residues, whereas only 2/4 missense variants in Stanford B AD were related to cysteine residues.
Variants in the 5′ region (exons 1–10), 3′ region (exons 59–65), and middle region (exons 24–32) of the FBN1 gene are related to specific manifestations (Sakai et al., 2016). In the AD group, only two positive variants were detected in the 3′ region, one in the middle region, and there was no 5′ region-positive variant. The above three positive variants were found in Stanford A AD.
The calcium-binding epidermal growth factor-like (cbEGF-like) domains are critical functional areas in fibrillin structures (http://www.umd.be/FBN1/). Among the 11 positive variants of the FBN1 gene, eight (seven missense variants and one frameshift deletion) were detected in this module, that is, the positive variants in the cbEGF domains were clearly dominant in our study group (Figure 1). The remaining three positive variants were located in the TGFβ-binding-protein-like (TGFBP) domains, homologous with TGFβ-1 binding protein, which were mainly interspersed among the cbEGF domains. Specifically, 8 AD individuals showed positive variants in the cbEGF domains, with 5/8 (62.5%) of these individuals diagnosed with Stanford A AD. Moreover, three patients had TGFBP domain-positive variants, two of which (66.7%) were diagnosed with Stanford A AD.
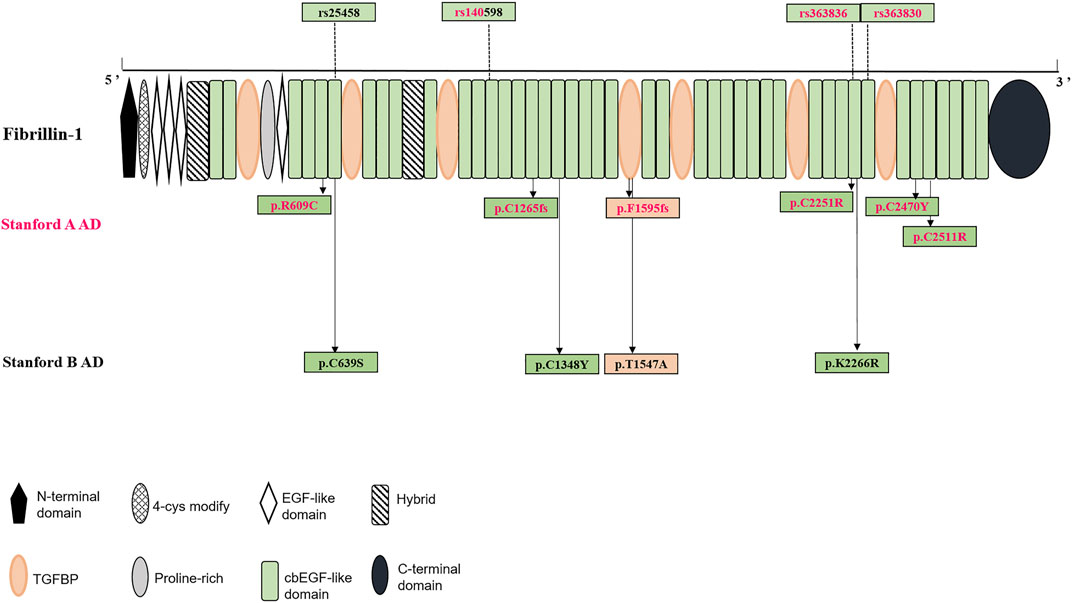
FIGURE 1. Structural domain of fibrillin-1 (encoded by FBN1 gene) and distribution of both positive variants and significant SNPs in the protein were shown.
Association of Common SNPs With AD
Associations of common SNPs with AD susceptibility were evaluated using the chi-square test, which provided ORs, 95% CIs, and level of significance (P). The MAFs of 10 SNPs were significantly higher in the AD group than in the healthy control subjects, which may appear to be significantly associated with an increased risk of AD. Detailed information on MAFs and OR values of these 10 significant SNPs is shown in Table 3. Among these, 4 SNPs (rs140598, rs363830, rs25458, and rs363836) were in the exon, while the rest 6 SNPs (rs11853943, rs140605, rs142758852, rs363838, rs55840194, and rs57512865) were in the intron. Notably, these four exonic SNPs were all located in the cbEGF domains (Figure 1), which further confirmed the importance of the cbEGF domains of the FBN1 and AD groups.
All 10 significant SNPs showed different distributions between Stanford A AD and Stanford B AD. The detailed information is presented in Table 4. Clearly, MAFs of five SNPs (rs363830, rs363836, rs142758852, rs363838, and rs55840194) were significantly higher in Stanford A AD than control group, but not in Stanford B AD. That is, these five SNPs may increase the susceptibility to Stanford A AD. Simultaneously, another four SNPs (rs25458, rs11853943, rs140605, and rs57512865) were more relevant to Stanford B AD. The remaining 1 SNP (rs140598) was significant in both Stanford A AD and Stanford B AD.
Among the four exonic SNPs, two were significantly associated with higher MAFs in Stanford A AD, 1 was significant in Stanford B AD, and 1 was significant with higher MAFs in both Stanford A AD and Stanford B AD (Table 4).
Correlation Between Genotype and Phenotype
The mean onset age for AD in individuals with FBN1 positive-variant (42.55 ± 6.95 years) was much lower than that of the non-FBN1 positive-variant group (49.63 ± 10.31 years, p = 0.03, Figure 2), whereas the mean onset age in the significant SNPs group showed no differences from that of other groups. The fact that AD patients with FBN1 positive-variant tended to suffer from AD at an earlier age supports the pathogenicity of these FBN1 variants. In addition, compared to the FBN1 negative-variant group, the missense variant-positive group showed a smaller mean onset age (42.00 vs. 49.63 years, P<0.05), while the mean onset age in the truncating and splicing group (44.00 years) was not significantly different from that of the FBN1 negative-variant group. Further, the mean onset ages in the cbEGF (42.88 years) and TGFBP domain (41.00 years) group showed no differences with those in the FBN1 negative-variant group, and there were no differences in those between the two domain groups.
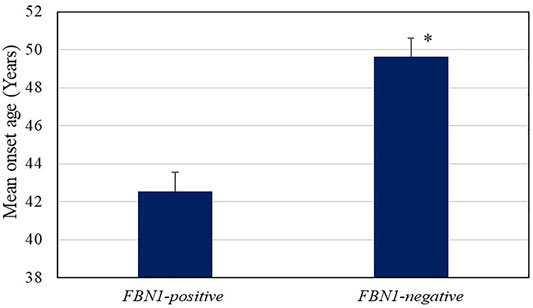
FIGURE 2. Age of onset of AD in the FBN1 positive variant (42.55 ± 6.95 years) and negative groups (49.63 ± 10.31 years, p = 0.03). The error bars indicate standard error.
In contrast to the male predominance in the FBN1 negative-variant group (72.2%, 57/79), females were predominant in the FBN1 positive-variant group (54.6%, 6/11), with no statistical differences. Moreover, the FBN1 positive-variant group showed a tendency for a less frequent history of hypertension (45.5 vs. 58.2%) and smoking (9.1 vs. 27.8%). The precise genetic information for Stanford A and Stanford B AD is provided in Table 5.
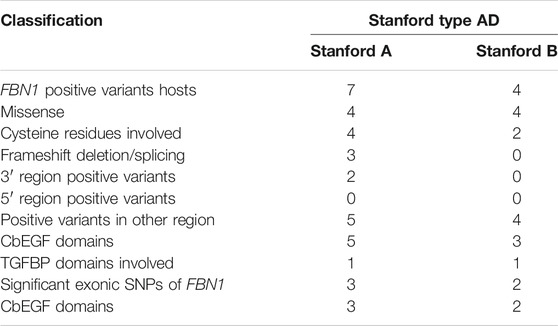
TABLE 5. Specific positive variants and SNPs information of FBN1 between Stanford A and Stanford B cases.
Discussion
Positive Variant Rate of FBN1
FBN1 was initially reported to be associated with MFS (Comeglio et al., 2007; Faivre et al., 2007). However, further research has revealed that many other clinical phenotypes without syndromic disease are associated with FBN1. Lesauskaite et al. (Lesauskaite et al., 2015) suggested that five FBN1 polymorphisms increased susceptibility to AD. Using exome sequencing, Regalado et al. (Regalado et al., 2016) found that the frequency of FBN1 mutations was 2.7% (5/183) in patients with familial thoracic aortic aneurysms and dissection. In addition, Tan et al. (Tan et al., 2017) detected a total of 26 FBN1 positive variant cases among 687 sporadic AD cases, with a detection rate of 3.9%. In our study, 11 FBN1 positive-variants (1 of which were novel) were identified, with a genetic diagnostic yield of 12.2% (11/90). Collectively, these data emphasize the pathogenicity of FBN1 in individuals with AD, and the clinical differences between FBN1 positive-variant carriers and non-FBN1 positive-variant (FBN1 negative-variant) carriers were also confirmed. Moreover, the FBN1 positive-variant group show a lower frequency of history of hypertension and smoking than FBN1 negative-variant group, which are well-known risk factors for AD.
Notably, the positive variant rate of FBN1 was high in our cohort. This wide range may be attributed to differences in the ethnicity of the patients in different studies. Although the population in our study was the same as that in the study by Tan et al. (Tan et al., 2017), there was a difference in the diagnostic yield of FBN1. One possible reason is that the proportion of AD type differed between our study group and that of Tan et al. (Tan et al., 2017). Our study group mainly comprised patients with the more severe type of AD, Stanford A (66.7%), whereas only 23.9% of the cohort of the previous study had Stanford A AD. However, further investigations are needed to confirm the factors contributing to the differences in these rates.
Different Types in Positive Variant of FBN1 Types
Different types of FBN1 variants have been reported in various studies (Robinson et al., 2006). However, missense variants have been reported to account for approximately 2/3 of MFS cases (Sakai et al., 2016), commonly involving the substitution or creation of new cysteine residues (Arbustini et al., 2005; Rommel et al., 2005). Positive variants involving cysteine residues may disturb right intra-domain disulfide bond formation, ultimately resulting in the alteration of the fibrillin-1 structure in the medial layer of the aorta (Tan et al., 2017). Among the 11 FBN1 positive variants, the large proportion of cysteine residues in our identified variants as well as the younger mean onset age of the group corroborates this point. In our study, positive missense variants (that is, missense variants of 11 FBN1 positive variants) accounted for a larger proportion (8/11, 72.7%). Moreover, in line with previous studies, missense variants involving cysteine accounted for a large proportion of these variants. It is worth noting that four missense variants in Stanford A AD were all associated with cysteine residues, while only 2/4 missense variants in Stanford B AD that were related to cysteine residues, suggesting the need to pay more attention to positive missense variants involved in cysteine residues in Stanford A AD.
Meanwhile, some studies (Wang et al., 2003; Yu et al., 2006; Jin et al., 2007; Li et al., 2008; Zhao et al., 2009; Wang et al., 2013) found that positive truncating or splicing variants were significantly increased over positive missense variants in Chinese patients with cardiovascular defects. In addition, some studies reported that MFS patients with aortic events had a higher frequency of FBN1 truncating (frameshift and nonsense) or splicing variants than those with no aortic events (Baudhuin et al., 2015), but this was not observed in our AD group. However, all three AD cases presented with splicing or truncation variants, which were all Stanford AD. In summary, among the six missense variants involved in cysteine residue hosts, four (66.7%) were cases of Stanford A AD, whereas 2 (3.3% (2/6) were Stanford B AD. Among the three splice or truncation variant hosts, 100% were Stanford A AD. Therefore, missense variants involved in cysteine residues and splicing/truncation variant hosts are more likely to suffer from a severe type of AD (Stanford A AD). Certain variant types and their affected clinical features in the Chinese population require further investigation and more data accumulation.
Significant SNPs in Stanford A and Stanford B AD
Many studies have focused on the positive variants between the FBN1 gene and AD. Nevertheless, the association between FBN1 polymorphisms and AD warrants further investigation. For instance, Lesauskaite et al. (Lesauskaite et al., 2015) indicated that two SNPs of FBN1 (rs1036477, rs2118181) were prone to increase the risk of ascending aortic aneurysm. In this study, a total of 10 FBN1 SNPs were detected to be significant in the AD group: having five SNPs may increase susceptibility to Stanford A AD, and having 4 SNPs were linked to the risk of Stanford B AD.
Specific Regions and Domains of the Identified Positive Variants
Specific regions of FBN1 are associated with concrete phenotypes. Positive variants in the 5 region (exons 1–10) and 3′ region (exons 59–65) are more likely to have milder cardiovascular features (Robinson et al., 2002; Comeglio et al., 2007). Two positive variants in the 3 region were identified: c.G7409A leading to Cys2470Try in exon 60 from a 34-year-old female patient with Stanford A AD and c.T7531C leading to Cys2511Arg in exon 61 from a 40-year-old male patient with Stanford A AD. In addition, positive variants identified in the middle region of FBN1 (exons 24–32), containing consecutive cbEGF-like domains, were linked to “neonatal MFS” and severe forms of MFS (Faivre et al., 2007; Faivre et al., 2009). One positive frameshift deletion variant was detected, c.3793delT, leading to Cys1265fs in exon 31, from a 45-year-old female patient with Stanford A AD. A few variants were concentrated in the above regions, and the remaining seven positive variants were evenly distributed in other FBN1 areas without a hotspot or specific region. This information indicates that all the regions of FBN1 are critical contributors to non-syndromic AD in our study.
Positive Variants in cbEGF Domains and TGFBP Domains
Multiple functional domains constitute the fibrillin protein, including 47 epidermal growth factor-like (EGF-like) domains, 43 of which are cbEGF-like domains, seven are TGBP domains, two are hybrid modules, one is a proline-rich domain, one is a unique amino-terminal, and one is a terminal region (Wang et al., 2013) (Figure 1). Therefore, it would be useful to determine whether variants in different domains are associated with specific phenotypes.
In our study group, common SNPs showed a clear distribution along the fibrillin protein domains. Four exon SNPs were located in the cbEGF domains, 3/4 were significant in Stanford A AD, and 2/4 were significant in Stanford B AD. Among the 11 positive variants, eight were located in the cbEGF domains, and two were located in the TGFBP domains. Specifically, among the seven Stanford A AD positive variants, five were located in the cbEGF domain and one was in the TGFBP domain. Similarly, four positive variants of Stanford B AD, three were in the cbEGF domain and one in the TGFBP domain.
In addition, the structure of a four–domain fibrillin fragment (EGF2-EGF3-Hyb1-cbEGF1) and C-terminal LTBP1 fragment demonstrated a bipartite interaction, which presumably facilitated a force-induced/traction-based TGF-β activation mechanism (https://www.ncbi.nlm.nih.gov/Structure/pdb/5MS9). Therefore, variants in this region may be related to AD, and should be considered. While there were no notable positive variants in this region, we will focus on it in future studies.
There were certain limitations to our study. First, only variants of FBN1 in patients with AD were analyzed. Second, the number of patients with AD enrolled in our study was not as large as that in other studies. Therefore, larger-scale testing should be carried out in the future to verify our findings and further confirm the distribution of the differences between Stanford A and Stanford B AD.
This comprehensive systematic study used FBN1 sequencing information to assess the role of both common SNPs and positive variants (pathogenic/likely pathogenic variants) of AD risk in the Han Chinese population. Eleven positive variants (1 novel) were detected in 12.2% (11/90) of AD individuals. FBN1 positive variants also led to an earlier onset of AD. Positive variants were spread along FBN1 without concentrated or hotspot regions. However, it is noteworthy that both missense variants involved in cysteine residues and splicing/truncation variant hosts were more likely to suffer from a severe type of AD (Stanford A AD). Thus, the critical role of the variant type of FBN1 was emphasized in this study, indicating that individuals with missense variants involved in cysteine residues and splicing/truncation variants may have a higher risk of developing Stanford A AD. In addition, 10 SNPs increased susceptibility to AD, which might be considered biomarkers for identifying individuals at risk for AD. In particular, five SNPs were detected significantly in Stanford A AD, while four SNPs were identified to be linked to Stanford B AD. Our study thus broadens the molecular pathology and database of common SNPs of FBN1 associated with non-syndromic AD among the Chinese population.
Among the 7 positive FBN1 variants in Stanford A AD, 5 were found in cbEGF domains, 1 was in TGEFB domains, and the rest 1 was splicing. Among the 4 positive FBN1 variants in Stanford B AD, 3 were found in cbEGF domains while 1 was in TGEFB domains. Four significant exonic SNPs were identified in AD, of which 2/4 (rs363836, rs363830) were link to Stanford A AD, 1/4 (rs25458) was prone to Stanford B AD, and the remaining 1 (rs140598) was associated in both Stanford A AD and Stanford B AD. These 4 SNPs were all located in the cbEGF domains, as well.
Data Availability Statement
The data that support the findings of this study have been deposited into CNGB Sequence Archive (CNSA) of China National GeneBank DataBase (CNGBdb), https://db.cngb.org/cnsa/, with accession number CNP0002539.
Ethics Statement
The studies involving human participants were reviewed and approved by This study was approved by the ethical committee of Tongji Medical College, Huazhong University of Science and Technology [approval number [2017] IEC (S059)]. The patients/participants provided their written informed consent to participate in this study.
Author Contributions
MP and LL: conceptualization, formal analysis, investigation, methodology, visualization, and writing – original draft. ZL: sample collection, data analysis and writing – original draft. SC: sample collection and writing – original draft. ZL: methodology, formal analysis, and writing – original draft. YW, HH, and LL: data analysis and writing – original draft. QL and HW: conceptualization, data curation, supervision, writing – original draft and review and editing.
Conflict of Interest
The authors declare that the research was conducted in the absence of any commercial or financial relationships that could be construed as a potential conflict of interest.
Publisher’s Note
All claims expressed in this article are solely those of the authors and do not necessarily represent those of their affiliated organizations, or those of the publisher, the editors and the reviewers. Any product that may be evaluated in this article, orclaim that may be made by its manufacturer, is not guaranteed or endorsed by the publisher.
Supplementary Material
The Supplementary Material for this article can be found online at: https://www.frontiersin.org/articles/10.3389/fgene.2022.778806/full#supplementary-material
References
Arbustini, E., Grasso, M., Ansaldi, S., Malattia, C., Pilotto, A., Porcu, E., et al. (2005). Identification of Sixty-Two Novel and Twelve Known FBN1 Mutations in Eighty-One Unrelated Probands with Marfan Syndrome and Other Fibrillinopathies. Hum. Mutat. 26, 494. doi:10.1002/humu.9377
Baudhuin, L. M., Kotzer, K. E., and Lagerstedt, S. A. (2015). Increased Frequency of FBN1 Truncating and Splicing Variants in Marfan Syndrome Patients with Aortic Events. Genet. Med. 17, 177–187. doi:10.1038/gim.2014.91
Comeglio, P., Johnson, P., Arno, G., Brice, G., Evans, A., Aragon-Martin, J., et al. (2007). The Importance of Mutation Detection in Marfan Syndrome and Marfan-Related Disorders: Report of 193FBN1 Mutations. Hum. Mutat. 28, 928. doi:10.1002/humu.9505
Fahrenbach, W. H., Sandberg, L. B., and Cleary, E. G. (1966). Ultrastructural Studies on Early Elastogenesis. Anat. Rec. 155, 563–575. doi:10.1002/ar.1091550409
Faivre, L., Collod-Béroud, G., Loeys, B. L., Child, A., Binquet, C., Gautier, E., et al. (2007). Effect of Mutation Type and Location on Clinical Outcome in 1,013 Probands with Marfan Syndrome or Related Phenotypes and FBN1 Mutations: An International Study. Am. J. Hum. Genet. 81, 454–466. doi:10.1086/520125
Faivre, L., Masurel-Paulet, A., Collod-Béroud, G., Callewaert, B. L., Child, A. H., Stheneur, C., et al. (2009). Clinical and Molecular Study of 320 Children with Marfan Syndrome and Related Type I Fibrillinopathies in a Series of 1009 Probands with Pathogenic FBN1 Mutations. Pediatrics 123, 391–398. doi:10.1542/peds.2008-0703
Gawinecka, J., Schönrath, F., and von Eckardstein, A. (2017). Acute Aortic Dissection: Pathogenesis, Risk Factors and Diagnosis. Swiss Med. Wkly 147, w14489. doi:10.4414/smw.2017.14489
Goldfinger, J. Z., Halperin, J. L., Marin, M. L., Stewart, A. S., Eagle, K. A., and Fuster, V. (2014). Thoracic Aortic Aneurysm and Dissection. J. Am. Coll. Cardiol. 64, 1725–1739. doi:10.1016/j.jacc.2014.08.025
Guo, D.-C., Regalado, E. S., Minn, C., Tran-Fadulu, V., Coney, J., Cao, J., et al. (2011). Familial Thoracic Aortic Aneurysms and Dissections: Identification of a Novel Locus for Stable Aneurysms with a Low Risk for Progression to Aortic Dissection. Circ. Cardiovasc. Genet. 4, 36–42. doi:10.1161/circgenetics.110.958066
Hoffjan, S. (2012). Genetic Dissection of Marfan Syndrome and Related Connective Tissue Disorders: An Update 2012. Mol. Syndromol 3, 47–58. doi:10.1159/000339441
Howard, D. P. J., Banerjee, A., Fairhead, J. F., Perkins, J., Silver, L. E., Rothwell, P. M., et al. (2013). Population-based Study of Incidence and Outcome of Acute Aortic Dissection and Premorbid Risk Factor Control:10-Year Results from the Oxford Vascular Study. Circulation 127, 2031–2037. doi:10.1161/circulationaha.112.000483
Jin, C., Yao, K., Jiang, J., Tang, X., Shentu, X., and Wu, R. (2007). Novel FBN1 Mutations Associated with Predominant Ectopia Lentis and Marfanoid Habitus in Chinese Patients. Mol. Vis. 13, 1280–1284.
Lesauskaite, V., Sepetiene, R., Jariene, G., Patamsyte, V., Zukovas, G., Grabauskyte, I., et al. (2015). FBN1 Polymorphisms in Patients with the Dilatative Pathology of the Ascending Thoracic Aorta. Eur. J. Cardiothorac. Surg. 47, e124–e130. doi:10.1093/ejcts/ezu520
Li, D., Yu, J., Gu, F., Pang, X., Ma, X., Li, R., et al. (2008). The Roles of Two NovelFBN1Gene Mutations in the Genotype-Phenotype Correlations of Marfan Syndrome and Ectopia Lentis Patients with Marfanoid Habitus. Genet. Test. 12, 325–330. doi:10.1089/gte.2008.0002
Li, Z., Zhou, C., Tan, L., Chen, P., Cao, Y., Li, C., et al. (2017). Variants of Genes Encoding Collagens and Matrix Metalloproteinase System Increased the Risk of Aortic Dissection. Sci. China Life Sci. 60, 57–65. doi:10.1007/s11427-016-0333-3
Pinard, A., Jones, G. T., and Milewicz, D. M. (2019). Genetics of Thoracic and Abdominal Aortic Diseases. Circ. Res. 124, 588–606. doi:10.1161/circresaha.118.312436
Regalado, E. S., Guo, D. C., Santos-Cortez, R. L. P., Hostetler, E., Bensend, T. A., Pannu, H., et al. (2016). PathogenicFBN1variants in Familial Thoracic Aortic Aneurysms and Dissections. Clin. Genet. 89, 719–723. doi:10.1111/cge.12702
Richards, S., Aziz, N., Bale, S., Bick, D., Das, S., Gastier-Foster, J., et al. (2015). Standards and Guidelines for the Interpretation of Sequence Variants: a Joint Consensus Recommendation of the American College of Medical Genetics and Genomics and the Association for Molecular Pathology. Genet. Med. 17, 405–424. doi:10.1038/gim.2015.30
Robinson, P. N., Arteaga-Solis, E., Baldock, C., Collod-Béroud, G., Booms, P., De Paepe, A., et al. (2006). The Molecular Genetics of Marfan Syndrome and Related Disorders. J. Med. Genet. 43, 769–787. doi:10.1136/jmg.2005.039669
Robinson, P. N., Booms, P., Katzke, S., Ladewig, M., Neumann, L., Palz, M., et al. (2002). Mutations of FBN1 and Genotype-Phenotype Correlations in Marfan Syndrome and Related Fibrillinopathies. Hum. Mutat. 20, 153–161. doi:10.1002/humu.10113
Rommel, K., Karck, M., Haverich, A., von Kodolitsch, Y., Rybczynski, M., Müller, G., et al. (2005). Identification of 29 Novel and Nine Recurrent Fibrillin-1 (FBN1) Mutations and Genotype-Phenotype Correlations in 76 Patients with Marfan Syndrome. Hum. Mutat. 26, 529–539. doi:10.1002/humu.20239
Sakai, L. Y., Keene, D. R., Renard, M., and De Backer, J. (2016). FBN1: The Disease-Causing Gene for Marfan Syndrome and Other Genetic Disorders. Gene 591, 279–291. doi:10.1016/j.gene.2016.07.033
Tan, L., Li, Z., Zhou, C., Cao, Y., Zhang, L., Li, X., et al. (2017). FBN1 Mutations Largely Contribute to Sporadic Non-syndromic Aortic Dissection. Hum. Mol. Genet. 26, 4814–4822. doi:10.1093/hmg/ddx360
Wang, B., Hu, D., Xia, J., Li, Q., Yang, J., and Lu, G. (2003). FBN1 Mutation in Chinese Patients with Marfan Syndrome and its Gene Diagnosis Using Haplotype Linkage Analysis. Chin. Med. J. (Engl) 116, 1043–1046.
Wang, K., Li, M., and Hakonarson, H. (2010). ANNOVAR: Functional Annotation of Genetic Variants from High-Throughput Sequencing Data. Nucleic Acids Res. 38, e164. doi:10.1093/nar/gkq603
Wang, W.-J., Han, P., Zheng, J., Hu, F.-Y., Zhu, Y., Xie, J.-S., et al. (2013). Exon 47 Skipping of Fibrillin-1 Leads Preferentially to Cardiovascular Defects in Patients with Thoracic Aortic Aneurysms and Dissections. J. Mol. Med. 91, 37–47. doi:10.1007/s00109-012-0931-y
Yu, R., Lai, Z., Zhou, W., Ti, D.-D., and Zhang, X.-N. (2006). Recurrent FBN1 Mutation (R62C) in a Chinese Family with Isolated Ectopia Lentis. Am. J. Ophthalmol. 141, 1136–1138. doi:10.1016/j.ajo.2005.12.044
Zhang, L., and Wang, H.-H. (2016). The Genetics and Pathogenesis of Thoracic Aortic Aneurysm Disorder and Dissections. Clin. Genet. 89, 639–646. doi:10.1111/cge.12713
Keywords: FBN1, cbEGF domains, cysteine residues, SNP, aortic dissection, splicing/truncation variants
Citation: Pan M, Li L, Li Z, Chen S, Li Z, Wang Y, He H, Lin L, Wang H and Liu Q (2022) Rare Variants and Polymorphisms of FBN1 Gene May Increase the Risk of Non-Syndromic Aortic Dissection. Front. Genet. 13:778806. doi: 10.3389/fgene.2022.778806
Received: 17 September 2021; Accepted: 04 January 2022;
Published: 27 January 2022.
Edited by:
Charles C. Hong, University of Maryland, United StatesReviewed by:
Mahyar Heydarpour, Brigham and Women’s Hospital and Harvard Medical School, United StatesAtiyeh Abdallah, Birmingham Women’s NHS Foundation Trust, United Kingdom
Copyright © 2022 Pan, Li, Li, Chen, Li, Wang, He, Lin, Wang and Liu. This is an open-access article distributed under the terms of the Creative Commons Attribution License (CC BY). The use, distribution or reproduction in other forums is permitted, provided the original author(s) and the copyright owner(s) are credited and that the original publication in this journal is cited, in accordance with accepted academic practice. No use, distribution or reproduction is permitted which does not comply with these terms.
*Correspondence: Qian Liu, Y2FpeGVfbGl1MDIyMkB0b20uY29t; Haihao Wang, aGh3YW5nQHRqaC50am11LmVkdS5jbg==
†These authors have contributed equally to this work