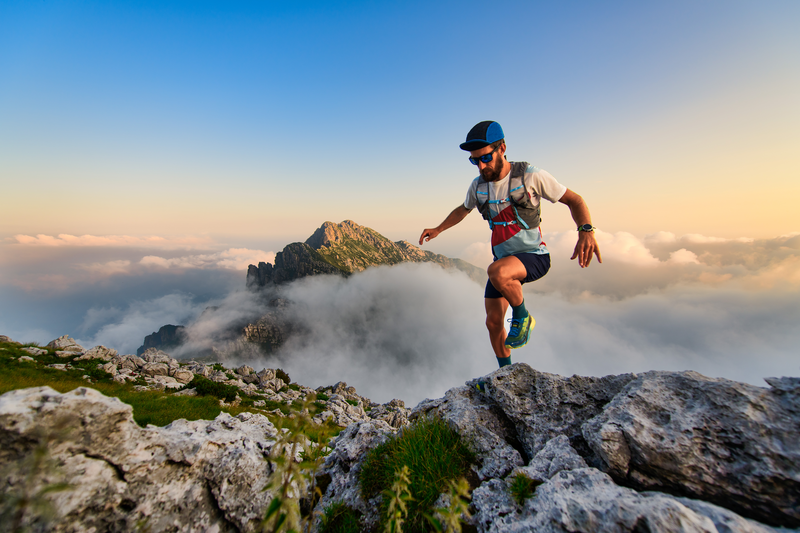
95% of researchers rate our articles as excellent or good
Learn more about the work of our research integrity team to safeguard the quality of each article we publish.
Find out more
ORIGINAL RESEARCH article
Front. Genet. , 07 December 2022
Sec. Genetics of Common and Rare Diseases
Volume 13 - 2022 | https://doi.org/10.3389/fgene.2022.1057293
Hearing loss is one of the most common sensory disorders in humans. This study proposes a stepwise strategy of deafness gene detection using multiplex PCR combined with high-throughput sequencing, Sanger sequencing, multiplex ligation-dependent probe amplification (MLPA), and whole-exome sequencing (WES) to explore its application in molecular diagnosis of hearing loss families. A total of 152 families with hearing loss were included in this study, the highest overall diagnosis rate was 73% (111/152). The diagnosis rate of multiplex PCR combined with high-throughput sequencing was 52.6% (80/152). One families was diagnosed by Sanger sequencing of GJB2 exon 1. Two families were diagnosed by MLPA analysis of the STRC gene. The diagnosis rate with additional contribution from WES was 18.4% (28/152). We identified 21 novel variants from 15 deafness genes by WES. Combining WES and deep clinical phenotyping, we diagnosed 11 patients with syndromic hearing loss (SHL). This study demonstrated improved diagnostic yield in a cohort of hearing loss families and confirmed the advantages of a stepwise strategy in the molecular diagnosis of hearing loss.
Hearing loss is the most common sensory disorder in humans, with an estimated prevalence of 1–3 in every 1,000 newborns worldwide (Morton and Nance, 2006). According to the World Report on Hearing by the World Health Organization in 2021, more than 5% of the world’s population (about 466 million people) suffer from hearing loss (https://www.who.int/news-room/fact-sheets/detail/deafness-and-hearing-loss). In China, around 30, 000 babies with congenital hearing impairment are born every year (Dai et al., 2006). It is estimated that genetic factors account for more than 60% of cases of hearing loss (Marazita et al., 1993; Morton and Nance, 2006). The inheritance patterns of deafness include autosomal recessive (80%), autosomal dominant (15%–20%), sex chromosome-linked (1%), and mitochondrial inheritance (1%) (Dai and Yuan, 2017). Hereditary hearing loss is a highly genetically heterogeneous disorder (Brownstein et al., 2012) that can be divided into syndromic hearing loss and non-syndromic hearing loss (NSHL), among which NSHL is the predominant type accounting for ∼70% of cases (Morton and Nance, 2006). To date, more than 400 syndromes related to hearing loss have been found (Alford et al., 2014), and over 110 NSHL genes have been identified (https://hereditaryhearingloss.org/).
Molecular epidemiological studies in China have identified several common deafness genes, such as GJB2, SLC26A4, and MT-RNR1, which account for 30%–50% of congenital hearing loss cases (Yuan et al., 2009; Du et al., 2014). In China, the ratio of patients carrying monoallelic variants in the coding exons of GJB2 is 6.1% (Dai et al., 2009). However, few studies have examined the non-coding exon 1 of GJB2 in Chinese patients with hearing loss. In 2010, Yuan et al. (2010) showed that testing for the NM_004004.6 (GJB2): c.-23 + 1G>A variant explained deafness in 1.89% (4/212) of Chinese patients with GJB2 monoallelic variants. In 2020, Yu et al. (2020) screened 1, 852 Chinese patients with deafness by Sanger sequencing in the GJB2 coding exon and flanking regions as well as the non-coding exon 1 and its flanking splice sites. The results showed that 475 patients had biallelic variants, one of whom carried a c.-23 + 1G>A (1/475, 0.2%) variant. Therefore, Sanger sequencing of GJB2 exon 1 should be included in routine testing of patients with GJB2 monoallelic pathogenic variants.
Recently, the STRC gene has been suggested to be an important molecular cause of mild-to-moderate deafness (Shearer et al., 2014; Plevova et al., 2017; Yokota et al., 2019). In 2019, Yokota et al. (2019) investigated the frequency of STRC deletions in the Japanese population. This study showed that the prevalence of STRC homozygous deletions was 1.7% (17/1,025) in the overall population with hearing loss, and 4.3% (17/398) among patients with mild-to-moderate hearing loss. In 2020, Kim et al. (2020) showed that approximately two-thirds (52/83, 62.7%) of mild-to-moderate sensorineural hearing loss (SNHL) have a clear Mendelian genetic etiology, with STRC-related deafness (29/83, 34.9%) being the most prevalent. These studies highlight the STRC gene as a major cause of mild-to-moderate hearing loss.
Due to the high genetic heterogeneity of hearing loss, it is necessary to develop a comprehensive and appropriate diagnostic strategy for patients. Whole-exome sequencing (WES) has been used as a single-step test in patients with hearing loss (Bademci et al., 2016; Zazo Seco et al., 2017; Sheppard et al., 2018; Sang et al., 2019). However, analyzing and interpreting WES data are usually laborious and time-consuming. A number of stepwise diagnostic approaches have been proposed (Baux et al., 2017; Guan et al., 2018; Li et al., 2019; Budde et al., 2020; Wang et al., 2021). Guan et al. (2018) used Sanger sequencing combined with targeted deletion analyses of GJB2 and STRC and two mitochondrial genes, followed by WES analysis of deafness-related genes. This study revealed 11 diagnoses (33%) and 8 possible diagnoses (24.2%) in 33 NSHL patients. Wang et al. (2021) provided a two-tier strategy consisting of multiplex PCR plus next-generation sequencing (NGS) applied to detect GJB2, SLC26A4, and MT-RNR1, followed by WES analysis. A diagnosis was made in 64% (59/92) of the patients in this study, with 44 of these cases diagnosed by multiplex PCR combined with high-throughput sequencing. None of these studies examined exon 1 of the GJB2 gene, which underestimated the diagnosis rate of the GJB2 gene. The SLC26A4 gene is the second leading cause of deafness in China. As Guan et al. (2018) did not include the SLC26A4 gene in tier one, this strategy is not suitable for Chinese populations. Wang et al. (2021) did not evaluate the contribution of STRC CNVs to mild-to-moderate SNHL.
Here, we propose a stepwise strategy including the detection of common deafness genes with multiplex PCR plus high-throughput sequencing, Sanger sequencing of GJB2 non-coding exon 1, MLPA of the STRC gene, and WES (Figure 1). Our stepwise strategy is comprehensive and cost-effective in analyzing the contributions of different genetic factors to deafness. We achieved a diagnosis rate of 73% in 152 hearing loss families. With multiplex PCR plus high-throughput sequencing, we diagnosed 80 (52.6%) families. Sanger sequencing of GJB2 exon 1 identified c.-23 + 1G>A variant in one patient. We detected two patients carrying homozygous deletion variants in the STRC gene by MLPA. Among the remaining undiagnosed patients, we obtained 17 diagnoses and 11 probable diagnoses by WES. The result can provide accurate and comprehensive genetic counseling for deafness families, and provide a strong foundation for prevention and control of birth defects of deafness in the family. In summary, this study improved the diagnostic yield in a cohort of hearing loss families, and confirmed the advantages of a stepwise strategy for molecular diagnosis of hearing loss.
Our cohort consisted of 152 hearing loss families from a special education school in Zhengzhou, Henan Province, China. The level of hearing loss was classified into four tiers in terms of pure tone average (averaged over 0.5, 1.0, 2.0, and 4.0 kHz): mild (21–40 dB nHL), moderate (41–70 dB nHL), severe (71–90 dB nHL), and profound (>91 dB nHL). This study complied with the World Medical Association Declaration of Helsinki and was approved by the Medical Ethics Committee of the Second Affiliated Hospital of Zhengzhou University (approval number: 2018008). Each participant provided signed informed consent (for probands under 18 years old, written informed consent was provided by their guardians) before participate in the present study.
All family members of the cohort were first tested for pathogenic variants in GJB2, SLC26A4, and MT-RNR1 genes (Figure 1). Sanger sequencing of GJB2 exon 1 was then carried out in undiagnosed families carrying GJB2 monoallelic variants. In addition, the STRC gene was tested in probands with mild-to-moderate hearing loss by MLPA. Finally, undiagnosed probands were referred for WES. Due to the variable expressivity and penetrance of c.109G>A in GJB2 (Shen et al., 2019), probands diagnosed with this variant (homozygous or compound heterozygous with other GJB2 variants) were also referred for WES to explore other potential molecular etiologies.
Genomic DNA was extracted from 2 ml of peripheral blood using a DNA extraction kit (GenMagBio, Amherst, NY, United States). NanoDrop One (Thermo Fisher Scientific, Waltham, MA, United States) was used to measure DNA concentration and purity. A multiplex PCR kit was designed to cover the entire coding region of GJB2, SLC26A4, and MT-RNR1, as an updated version of the kit developed in a previous study (Tian et al., 2021). Multiplex PCR assay does not include detection of the GBJ2 exon 1. The resulting libraries were sequenced on an Illumina MiniSeq sequencer (Illumina, San Diego, CA, United States) in 150-bp paired-end mode. Bioinformatics analysis was performed in the bcbio-nextgen framework (https://github.com/bcbio/bcbio-nextgen). Reads trimming, genome aligning, variant annotation, filtering, and interpretation were carried out as described previously (Tian et al., 2021).
GJB2 exon 1, its c.-23 + 1G>A variant and variants in the promoter region were amplified with the primers (Supplementary Table S1) in four families with a monoallelic variant in the coding region of GJB2.
Among probands undiagnosed using multiplex PCR combined with high-throughput sequencing, we screened all patients with mild-to-moderate hearing loss for MLPA. An MLPA probemix kit (Catalogue numbers: P461-A1-050R, MRC-Holland, Amsterdam, the Netherlands) was used to detect CNVs of STRC, as suggested by the manufacturer. Amplification products were run on an Applied Biosystems™ SeqStudio™ Genetic Analyzer (Thermo Fisher Scientific), and the results were analyzed using GeneMarker 1.91 software (SoftGenetics, State College, PA, United States).
WES was applied to identify the potential genetic causes for probands undiagnosed in the steps described above. Standard WES-based genetic testing, including sample preparation and quantification, library construction, hybrid sequence capture, sequencing, and data analyses, was performed as described previously (Pan et al., 2020; Zhang et al., 2021). Copy number analysis was performed from NGS data using DECoN (Fowler et al., 2016) with the alignment BAM files from the same enrichment panel and sequencing run.
We performed PCR amplification and Sanger sequencing to confirm candidate variants detected by WES and conduct co-segregation analyses in family members. The specific primers (Supplementary Table S1) were designed using NCBI Primer-BLAST and synthesized by Sunya Biotech Co., Ltd. (Zhengzhou, China). Sequencing was performed using a SeqStudio Genetic Analyzer (Applied Biosystems, Foster City, CA, United States) after PCR product purification, and the results were visualized with Chromas software.
Variant interpretation was performed according to the guidelines of the American College of Medical Genetics and Genomics (ACMG) (Richards et al., 2015) and the ClinGen Hearing Loss Expert Group’s recommendations (Oza et al., 2018). According to the GenCC database (https://search.thegencc.org/), the candidate gene was included in the results only when there was a definitive, strong, or moderate relationship between the gene and deafness. Patients were categorized as “diagnosed” if they were homozygous or compound heterozygous for a pathogenic/likely pathogenic variant(s) in a recessively inherited gene or heterozygous for a pathogenic/likely pathogenic variant in a dominantly inherited gene. In addition, patients with a pathogenic/likely pathogenic variant plus a rare variant of uncertain significance (VUS) in a recessively inherited gene, or two rare VUS in the CDH23 or MYO15A gene, were considered as “probably diagnosed.” When there were two VUS in the candidate pathogenic gene of the patient, and the clinical phenotype caused by the gene variant was consistent with the clinical phenotype of the patient, it was also considered as “probably diagnosed.” Patients with one pathogenic/likely pathogenic variant in a recessively inherited gene were considered as “undiagnosed.”
We recruited 152 hearing loss families from a special education school in Zhengzhou City, Henan Province, China. The probands included 100 (65.8%) males and 52 (34.2%) females; 131 (86.2%) patients had prelingual hearing loss and 21 (13.8%) patients were postlingual; 139 (91.5%) patients had severe-to-profound deafness, 9 (5.9%) patients had mild-to-moderate hearing loss, and 4 (2.6%) patients had no records; 128 (84.2%) patients showed stable hearing loss, 21 (13.8%) patients were progressive, and 3 (2%) patients had no records. A few patients were suspected to have SHL (2%) before genetic testing, and the majority (98%) were found to have NSHL (Table 1).
All family members in 152 families were tested for mutations in the common deafness genes by multiplex PCR plus high-throughput sequencing. The average sequencing depth of target regions was 500×, with 100% of target regions having coverage greater than 100×. The diagnosis rate of multiplex PCR combined with high-throughput sequencing was 52.6% (80/152), of which GJB2 accounted for 29.6% (45/152), SLC26A4 accounted for 21.7% (33/152), and MT-RNR1 accounted for 1.3% (2/152) (Figures 2A,B). The genotypes of the 80 patients who tested positive are listed in Table 2. The variant c.235del was the most prevalent in the GJB2 gene, including 19 patients who were homozygous and 22 patients who were compound heterozygous. The variant c.919-2A>G was the most prevalent in the SLC26A4 gene, including 10 patients who were homozygous and 16 patients who were compound heterozygous. The patients whose pathogenic gene was MT-RNR1 carried a homoplasmic m.1555A>G variant. One patient carried SLC26A4 c.1124A>G and c.1409G>A variants both of which came from the mother, and there was no enlarged vestibular aqueduct (EVA) in clinical diagnosis. In addition, four patients have a single heterozygous variant of GJB2 (Supplementary Table S2). Therefore, Sanger sequencing of GJB2 exon 1 was carried in these four families. We detected one patient carrying the c.-23 + 1G>A variant, forming a compound heterozygous variant with c.235del (Table 3). The remaining three patients were further examined by WES.
FIGURE 2. Molecular diagnostic results of 152 families classified according to genes (A) and detection methods (B).
TABLE 2. Genotypes of 80 patients detected by multiplex PCR sequencing. Hom, homozygous; Het, heterozygous; Homo, homoplasmy; AR, autosomal recessive; Mi, mitochondrial; P, pathogenic; LP likely pathogenic.
Of the 71 undiagnosed patients, four had mild-to-moderate NSHL, and were examined by MLPA. We detected two patients carrying homozygous deletion variants in exons 19 and 23–25 of the STRC gene (Table 4), and the homozygous form may be the primary cause of hearing loss. The female patient was diagnosed with homozygous continuous gene deletion of STRC and CATSPER2. However, if the proband were male, he may have suffered from deafness-infertility syndrome (MIM611102) (Avidan et al., 2003; Zhang et al., 2007).
Sixty-nine undiagnosed patients were referred for WES, resulting in 17 diagnoses and 11 probable diagnoses (Supplementary Table S2). The diagnosis rate with additional contribution from WES was 18.4% (28/152). In the 28 cases, a total of 46 different variants were detected in 15 known deafness genes, of which 21 (21/46, 45.7%) were novel from TRIOBP, MPZL2, MYO15A, PCDH15, LOXHD1, POU3F4, CDH23, COL11A2, and HARS2 genes. All detected variants fulfilled the requirement for phenotype–genotype co-segregation. The genes with the largest proportion of patients detected were CHD23 (5/28, 17.9%) and MYO15A (6/28, 21.4%). One patient 3312236) with mild-to-moderate NSHL was negative on the STRC MLPA test, and the pathogenic gene was identified as MPZL2 by WES. This was the first reported case of mild-to-moderate hearing loss caused by MPZL2 variants in China.
In addition, due to the variable expressivity and incomplete penetrance of GJB2 c.109G>A (Shen et al., 2019), two compound heterozygous patients for GJB2 c.109G>A identified by multiplex PCR were also referred for WES to exclude other potential molecular etiologies. No other causally associated hearing loss variants were identified in these two patients by these analyses. Therefore, we included these two patients in the group diagnosed by multiplex PCR combined with high-throughput sequencing. The molecular diagnosis results of 152 hearing loss families are shown in Figure 2. The results of Sanger validation of families are shown in Supplementary Figure S1.
Combining WES and deep clinical phenotyping, we diagnosed 11 patients with SHL. Four had Waardenburg syndrome (WS)-II type A and type E, caused by MITF and SOX10 gene variants, respectively. Before genetic testing, patients 3312301 and 3312311 were primarily diagnosed with WS with congenital bilateral profound SNHL and blue iris. In contrast, patients 3312176 and 3312249 were diagnosed with NSHL. After genetic testing, we detected a de novo variant in the SOX10 gene in patient 3312301 and a de novo variant of the MITF gene in patient 3312311. Their clinical phenotypes were consistent with the genotypes and could be identified as WS-II. We found that MITF was a candidate pathogenic gene for patients 3312176 and 3312249. Therefore, we revisited the two families for further clinical phenotypic examination. It is worth noting that patient 3312176 had profound bilateral deafness and freckles caused by NM_198159.3 (MITF): c.1212G>A, but there was no other clinical phenotype associated with WS. The variant came from the father, and the proband’s sister also had this variant, but only had freckles and had normal hearing. A similar situation occurred in patient 3312249 (MITF c.1021C>T). The proband had clinical phenotypes of deafness and freckles but no other classic phenotype of WS. The variant came from the mother, who has normal hearing and only a few freckles. Therefore, we confirmed that the patients in these two families had WS-II.
Before genetic testing, patients 3312205 and 3312291 were primarily diagnosed with NSHL, and patient 3312343 was included as “syndromic” because of her small stature and progeroid appearance with hearing loss. After a genetic diagnosis, we found two de novo variants of PTPN11 in patients 3312205 and 3312291, and one de novo variant of the POLD1 gene in patient 3312343, which led to diagnoses of Noonan syndrome (MIM163950) and MDPL syndrome (mandibular hypoplasia, deafness, progeroid features, and lipodystrophy syndrome, MIM615381), respectively. The case of patient 3312343 was described in detail in another article. For patient 3312205, we conducted an in-depth clinical examination and the results revealed congenital heart disease and atrial septal defect, which could be identified as Noonan syndrome. We also informed the parents of patient 3312291 of the genetic testing results and suggested that relevant clinical examinations should be carried out as soon as possible to determine the etiology of the proband and prevent more serious disease consequences.
In addition, we also found that the pathogenic gene of an early childhood patient, 3312198, was USH2A. Although the patient was primarily diagnosed with NSHL before genetic testing, the results showed that he had Usher syndrome (type 2A) (MIM276901). We found two frameshift variants of the PCDH15 gene in an early childhood patient, 3312279. Before genetic testing, the patient was primarily diagnosed with NSHL. However, the results showed that she was likely to have Usher syndrome. We recommended that the guardian accompany the patient regularly to check her motor development and visual acuity, and follow the doctor’s treatment advice. Patient 3312229 was identified as a compound heterozygote composed of splicing and missense variants of the CDH23 gene. Although the patient 3312229 was more likely to have Usher syndrome, we cannot exclude the possibility of non-syndromic hearing loss. The patient was only in early childhood, and there was no syndrome-related phenotype, such as retinitis pigmentosa, which may occur at a relatively late age.
We detected two missense variants of HARS2 in an early childhood boy, patient 3312255, who was primarily diagnosed with NSHL before genetic testing. Variants in this gene lead to a diagnosis of Perrault syndrome 2 (MIM614926). Females with this disease will have clinical phenotypes, such as ovarian dysgenesis and infertility, while affected males have normal pubertal development and fertility.
Therefore, WES combined with deep phenotyping improved diagnostic yield, and comprehensive and complete phenotypic information is indispensable for genetic analysis of hearing loss.
This study proposed a stepwise strategy of deafness genes with multiplex PCR combined with high-throughput sequencing, Sanger sequencing, MLPA, and WES to explore molecular diagnoses of 152 Chinese hearing loss families, achieving a 73% (111/152) diagnostic yield. Our study had a higher diagnosis rate than the stepwise detection strategies reported in previous studies (48%–64%) (Baux et al., 2017; Wang et al., 2021).
Our study used multiplex PCR plus NGS to detect mutations in the GJB2, SLC26A4, and MT-RNR1 genes with a diagnosis rate of 52.6%, providing rapid molecular diagnosis and saving the cost of WES. This is because there are hotspot genes and variants in the Chinese hearing loss population, such as GJB2 c.235del, SLC26A4 c.919-2A>G, and MT-RNR1 m.1555A>G. Compared with the diagnostic strategy of analyzing only the GJB2 gene in the first step (Baux et al., 2017; Guan et al., 2018), our multiplex PCR detection strategy is more effective and economical for the Chinese hearing loss population. In addition, for Chinese patients with hearing loss, most detection strategies reported to date may underestimate the diagnosis rate of the GJB2 gene because they did not examine mutations in exon 1 (Sakuma et al., 2016; Guan et al., 2018; Wang et al., 2021). The GJB2 c.-23 + 1G>A variant accounts for 0.2%–1.89% of hearing loss patients in China (Yuan et al., 2010; Yu et al., 2020).
It is reported that MT-RNR1 m.1555A>G variant may lead to low or non-penetrance hearing loss (Li et al., 2004). In the absence of aminoglycosides, the m.1555A>G variant produces a clinical phenotype that ranges from severe congenital deafness, to moderate progressive hearing loss of later onset, to completely normal hearing (Prezant et al., 1993; Estivill et al., 1998). If there are patients with deafness who have m.1555A>G variant and no history of aminoglycosides exposure, we suggest that WES should be used to explore other potential molecular etiologies.
If there are mild-to-moderate hearing loss patients in the cohort, then it would also be necessary to include MLPA detection of the STRC gene in the stepwise strategy. Although there have been few studies on hearing loss caused by the STRC gene in China, Yokota et al. (2019) and Kim et al. (2020) emphasized that the STRC gene is the main cause of mild-to-moderate hearing loss in the East Asian population. However, Wang et al. (2021) did not analyze STRC CNVs in their detection strategy. Our study also confirmed the above inference as two of the four patients with mild-to-moderate hearing loss in the cohort were diagnosed by MLPA testing.
Combining WES and deep clinical phenotyping, we revealed a number of cases of SHL. WS has a high degree of genetic heterogeneity and highly variable phenotype expressivity (Newton, 1990; Read and Newton, 1997; Song et al., 2016). We identified two families with WS caused by MITF gene variants, and their members had different degrees of phenotype expressivity. PCDH15 and CDH23 are the pathogenic genes for Usher syndrome. It was reported that missense variants of the PCDH15 gene led to NSHL, while other variants (frameshift, nonsense, splicing, and large fragment deletion) caused Usher syndrome (type 1F) (MIM602083) (Ahmed et al., 2008). Li et al. (2020) reported a family with Usher syndrome type 1 caused by two frameshift variants in the PCDH15 gene. This suggested that patient 3312279 was likely to have Usher syndrome. Similarly, it was reported that nonsense variants, splicing variants, and frameshift variants in the CDH23 gene led to Usher syndrome (type 1D) (MIM601067), while missense variants led to DFNB12 (Bork et al., 2001; Astuto et al., 2002). We identified a splicing variant and a missense variant of the CDH23 gene in patient 3312229. Our previous study confirmed that compound heterozygosity of CDH23 splicing and missense variants led to Usher syndrome (Chen et al., 2020). Therefore, the patient was more likely to have Usher syndrome. These cases highlight the advantages of WES in diagnosing hearing loss-related syndromes. This allows the early recognition of the syndrome, especially Usher syndrome, in patients and suggests the effect of cochlear implantation and reduces the risk of disease recurrence in families.
We identified 11 probable diagnoses in probands by WES, of which five were attributed to variants in the CHD23 gene and three to variants in the MYO15A gene. This is because the main type of variation detected in these two genes is missense variation and the functional effects of variants cannot be determined. Most missense variants can only be interpreted as VUS by following the ACMG guidelines. Experimental validation of the possible pathogenic effects by in vivo or in vitro functional studies is an accurate method of determining the molecular basis of disease caused by missense variants (George Priya Doss et al., 2013). Deep mutational scanning may also be useful (Fowler and Fields, 2014). In addition, practical bioinformatics tools can be used to predict the functional effects of missense variants (Ng and Henikoff, 2006). Many disease-related missense variants have been evaluated by in silico methods, and have helped to reveal protein structure–function relationships and disease genotype–phenotype correlations (Thusberg and Vihinen, 2006; Doss and Sethumadhavan, 2009; Forman et al., 2009).
In this study, up to 111 families with deafness can be diagnosed, but effective treatment is still lacking. Although current treatments, such as hearing aids and cochlear implants, can rehabilitate hearing in some patients, these approaches are limited by their sensitivity and perception of natural sounds in noisy environments (Lesica, 2018; Tan et al., 2019). Hearing loss is mainly caused by the irreversible loss of cochlear hair cells and auditory neurons. Thus, an effective way to treat hearing loss is to regenerate or repair damaged cells in the inner ear. During the past decade, gene therapy and neural stem cell therapy have emerged as promising treatment strategies for hearing loss (He et al., 2021; Nourbakhsh et al., 2021; Qi et al., 2022). One major challenge of gene therapy is effectively delivering genes to target cells, and at present, the delivery vectors contain two types: viral and non-viral. Among viral vectors, the Adeno-associated virus (AAV) vector is suitable for the treatment of hearing loss with a number of AAV serotypes have been developed, such as AAV1(Akil et al., 2012), AAV-ie (Tan et al., 2019; Tao et al., 2022), AAV9-PHP.B (Taiber et al., 2021), etc. In addition to gene therapy, using stem cells to induce differentiation to regenerate or repair damaged cells in the inner ear is regarded as the most feasible treatment (He et al., 2021). There are usually two routes of research: the transplantation of exogenous stem cells and their subsequent differentiation in the damaged tissue and the genetic modification of endogenous cells to promote their transdifferentiation potential (de Felipe et al., 2011; Duran-Alonso, 2020). We predict that, with the application of biomaterials in stem cell therapy (Xia et al., 2022), pathogenesis-based gene therapy and stem cell therapy will be ideal for human hereditary deafness.
Forty-one patients in this study were still undiagnosed, among whom two negative patients had a single heterozygous variant of GJB2, and one patient carried SLC26A4 (c.1124A>G and c.1409G>A) variants with a single maternal haplotype, and there was no EVA in clinical diagnosis. No pathogenic variants were found in the remaining patients. There were two families in the cohort, each with two patients with prelingual deafness suggesting a monogenic trait, and the molecular etiology was not identified by WES. We may conduct further research in the future. The hearing loss in some of the patients may not have had a genetic etiology, e.g., patient 3312294 was suspected to have post-traumatic hearing loss based on the clinical phenotypic survey. In addition, undiagnosed patients may have hearing loss caused by new deafness genes that have not been identified previously or variants that could not be detected by the methods used in this study. In the next step, we will perform further analyses of these patients, such as WGS and third-generation sequencing.
In conclusion, this study improved the diagnostic yield, revealed rich phenotypic differences, and confirmed the advantages of a stepwise strategy in the molecular diagnosis of hearing loss families.
The raw sequencing data supporting this article cannot be placed in public repository due to national legislation/guidelines, specifically the Regulation of the People's Republic of China on the Administration of Human Genetic Resources (http://www.gov.cn/zhengce/content/2019-06/10/content_5398829.htm, http://english.www.gov.cn/policies/latest_releases/2019/06/10/content_281476708945462.htm) and institutional guidelines by the special education school. The exome sequencing data for undiagnosed patients were deposited in the National Supercomputing Center in Zhengzhou. Please email bnNjY0B6enUuZWR1LmNu for detailed application guidance only for academic use.
This study complied with the World Medical Association Declaration of Helsinki and was approved by the Medical Ethics Committee of the Second Affiliated Hospital of Zhengzhou University (approval number: 2018008). Each participant provided signed informed consent (for probands under 18 years old, written informed consent was provided by their guardians) before participate in the present study.
WT, MC, and HX designed the study. BZ, HX, SL, and YT enrolled the cohort and collected the clinical phenotype. BZ, YY, YT, TL, and HW conducted the experiments. HX and ZY conducted the bioinformatic analyses. BZ, HX, SL, and GW analyzed the data and interpreted variation. BZ, HX, WT, and SL wrote the manuscript, all authors have read and approved the final version. BZ and HX contributed equally to this study.
The study is funded by the Collaborative Innovation Project of Zhengzhou (Zhengzhou University) (Grant no. 18XTZX12004) to WT and the Open Research Fund of National Health Commission Key Laboratory of Birth Defects Prevention (Grant no. ZD202102 and ZD202007) to WT and HX.
Sincere thanks to all the subjects for their participation in this study. We thank the Supercomputing Center of Zhengzhou University for providing computational and storage resources.
The authors declare that the research was conducted in the absence of any commercial or financial relationships that could be construed as a potential conflict of interest.
All claims expressed in this article are solely those of the authors and do not necessarily represent those of their affiliated organizations, or those of the publisher, the editors and the reviewers. Any product that may be evaluated in this article, or claim that may be made by its manufacturer, is not guaranteed or endorsed by the publisher.
The Supplementary Material for this article can be found online at: https://www.frontiersin.org/articles/10.3389/fgene.2022.1057293/full#supplementary-material
Ahmed, Z. M., Riazuddin, S., Aye, S., Ali, R. A., Venselaar, H., Anwar, S., et al. (2008). Gene structure and mutant alleles of PCDH15: Nonsyndromic deafness DFNB23 and type 1 usher syndrome. Hum. Genet. 124 (3), 215–223. doi:10.1007/s00439-008-0543-3
Akil, O., Seal, R. P., Burke, K., Wang, C. S., Alemi, A., During, M., et al. (2012). Restoration of hearing in the VGLUT3 knockout mouse using virally mediated gene therapy. Neuron 75 (2), 283–293. doi:10.1016/j.neuron.2012.05.019
Alford, R. L., Arnos, K. S., Fox, M., Lin, J. W., Palmer, C. G., Pandya, A., et al. (2014). American College of Medical Genetics and Genomics guideline for the clinical evaluation and etiologic diagnosis of hearing loss. Genet. Med. 16 (4), 347–355. doi:10.1038/gim.2014.2
Astuto, L. M., Bork, J. M., Weston, M. D., Askew, J. W., Fields, R. R., Orten, D. J., et al. (2002). CDH23 mutation and phenotype heterogeneity: A profile of 107 diverse families with usher syndrome and nonsyndromic deafness. Am. J. Hum. Genet. 71 (2), 262–275. doi:10.1086/341558
Avidan, N., Tamary, H., Dgany, O., Cattan, D., Pariente, A., Thulliez, M., et al. (2003). CATSPER2, a human autosomal nonsyndromic male infertility gene. Eur. J. Hum. Genet. 11 (7), 497–502. doi:10.1038/sj.ejhg.5200991
Bademci, G., Foster, J., Mahdieh, N., Bonyadi, M., Duman, D., Cengiz, F. B., et al. (2016). Comprehensive analysis via exome sequencing uncovers genetic etiology in autosomal recessive nonsyndromic deafness in a large multiethnic cohort. Genet. Med. 18 (4), 364–371. doi:10.1038/gim.2015.89
Baux, D., Vache, C., Blanchet, C., Willems, M., Baudoin, C., Moclyn, M., et al. (2017). Combined genetic approaches yield a 48% diagnostic rate in a large cohort of French hearing-impaired patients. Sci. Rep. 7 (1), 16783. doi:10.1038/s41598-017-16846-9
Bork, J. M., Peters, L. M., Riazuddin, S., Bernstein, S. L., Ahmed, Z. M., Ness, S. L., et al. (2001). Usher syndrome 1D and nonsyndromic autosomal recessive deafness DFNB12 are caused by allelic mutations of the novel cadherin-like gene CDH23. Am. J. Hum. Genet. 68 (1), 26–37. doi:10.1086/316954
Brownstein, Z., Bhonker, Y., and Avraham, K. B. (2012). High-throughput sequencing to decipher the genetic heterogeneity of deafness. Genome Biol. 13 (5), 245. doi:10.1186/gb-2012-13-5-245
Budde, B. S., Aly, M. A., Mohamed, M. R., Bress, A., Altmuller, J., Motameny, S., et al. (2020). Comprehensive molecular analysis of 61 Egyptian families with hereditary nonsyndromic hearing loss. Clin. Genet. 98 (1), 32–42. doi:10.1111/cge.13754
Chen, B., Zhang, S., Tian, Y. A., Liu, H. F., Liu, D. H., Xue, X., et al. (2020). Study on syndromic deafness caused by novel pattern of compound heterozygous variants in the CDH23 gene. Zhonghua Er Bi Yan Hou Tou Jing Wai Ke Za Zhi 55 (9), 822–829. doi:10.3760/cma.j.cn115330-20191015-00629
Dai, P., Liu, X., Yu, F., Zhu, Q., Yuan, Y., Yang, S., et al. (2006). Molecular etiology of patients with nonsyndromic hearing loss from deaf-mute schools in 18 provinces of China. Chin. J. Otology 4 (1), 1–5. doi:10.3969/j.issn.1672-2922.2006.01.001
Dai, P., Yu, F., Han, B., Liu, X., Wang, G., Li, Q., et al. (2009). GJB2 mutation spectrum in 2, 063 Chinese patients with nonsyndromic hearing impairment. J. Transl. Med. 7, 26. doi:10.1186/1479-5876-7-26
Dai, P., and Yuan, Y. (2017). Genetic diagnosis and genetic counseling of deafness. Beijing: People's Medical Publishing House.
de Felipe, M. M. C., Redondo, A. F., Garcia-Sancho, J., Schimmang, T., Alonso, M. B. D., and Feijoo Redondo, A. (2011). Cell- and gene-therapy approaches to inner ear repair. Histol. Histopathol. 26 (7), 923–940. doi:10.14670/HH-26.923
Doss, C. G., and Sethumadhavan, R. (2009). Investigation on the role of nsSNPs in HNPCC genes-a bioinformatics approach. J. Biomed. Sci. 16, 42. doi:10.1186/1423-0127-16-42
Du, W., Wang, Q., Zhu, Y., Wang, Y., and Guo, Y. (2014). Associations between GJB2, mitochondrial 12S rRNA, SLC26A4 mutations, and hearing loss among three ethnicities. Biomed. Res. Int. 2014, 746838. doi:10.1155/2014/746838
Duran-Alonso, M. B. (2020). Stem cell-based approaches: Possible route to hearing restoration? World J. Stem Cells 12 (6), 422–437. doi:10.4252/wjsc.v12.i6.422
Estivill, X., Govea, N., Barcelo, E., Badenas, C., Romero, E., Moral, L., et al. (1998). Familial progressive sensorineural deafness is mainly due to the mtDNA A1555G mutation and is enhanced by treatment of aminoglycosides. Am. J. Hum. Genet. 62 (1), 27–35. doi:10.1086/301676
Forman, J. R., Worth, C. L., Bickerton, G. R., Eisen, T. G., and Blundell, T. L. (2009). Structural bioinformatics mutation analysis reveals genotype-phenotype correlations in von Hippel-Lindau disease and suggests molecular mechanisms of tumorigenesis. Proteins 77 (1), 84–96. doi:10.1002/prot.22419
Fowler, A., Mahamdallie, S., Ruark, E., Seal, S., Ramsay, E., Clarke, M., et al. (2016). Accurate clinical detection of exon copy number variants in a targeted NGS panel using DECoN. Wellcome Open Res. 1, 20. doi:10.12688/wellcomeopenres.10069.1
Fowler, D. M., and Fields, S. (2014). Deep mutational scanning: A new style of protein science. Nat. Methods 11 (8), 801–807. doi:10.1038/nmeth.3027
George Priya Doss, C., Nagasundaram, N., Chakraborty, C., Chen, L., and Zhu, H. (2013). Extrapolating the effect of deleterious nsSNPs in the binding adaptability of flavopiridol with CDK7 protein: A molecular dynamics approach. Hum. Genomics 7, 10. doi:10.1186/1479-7364-7-10
Guan, Q., Balciuniene, J., Cao, K., Fan, Z., Biswas, S., Wilkens, A., et al. (2018). Audiome: A tiered exome sequencing-based comprehensive gene panel for the diagnosis of heterogeneous nonsyndromic sensorineural hearing loss. Genet. Med. 20 (12), 1600–1608. doi:10.1038/gim.2018.48
He, Z. H., Ding, Y. Y., Mu, Y. R., Xu, X. X., Kong, W. J., Chai, R. J., et al. (2021). Stem cell-based therapies in hearing loss. Front. Cell Dev. Biol. 9, 730042. doi:10.3389/fcell.2021.730042
Kim, B. J., Oh, D. Y., Han, J. H., Oh, J., Kim, M. Y., Park, H. R., et al. (2020). Significant Mendelian genetic contribution to pediatric mild-to-moderate hearing loss and its comprehensive diagnostic approach. Genet. Med. 22 (6), 1119–1128. doi:10.1038/s41436-020-0774-9
Lesica, N. A. (2018). Why do hearing aids fail to restore normal auditory perception? Trends Neurosci. 41 (4), 174–185. doi:10.1016/j.tins.2018.01.008
Li, M. F., Luo, L., Yu, Y., and Dongmei, H. (2020). Analysis of PCDH15 gene mutation and prenatal diagnosis in a pedigree affected with Usher syndrome type 1. J. China Med. Univ. 49 (3), 220–223. doi:10.12007/j.issn.0258-4646.2020.03.006
Li, M., Mei, L., He, C., Chen, H., Cai, X., Liu, Y., et al. (2019). Extrusion pump ABCC1 was first linked with nonsyndromic hearing loss in humans by stepwise genetic analysis. Genet. Med. 21 (12), 2744–2754. doi:10.1038/s41436-019-0594-y
Li, R., Xing, G., Yan, M., Cao, X., Liu, X. Z., Bu, X., et al. (2004). Cosegregation of C-insertion at position 961 with the A1555G mutation of the mitochondrial 12S rRNA gene in a large Chinese family with maternally inherited hearing loss. Am. J. Med. Genet. A 124A (2), 113–117. doi:10.1002/ajmg.a.20305
Marazita, M. L., Ploughman, L. M., Rawlings, B., Remington, E., Arnos, K. S., and Nance, W. E. (1993). Genetic epidemiological studies of early-onset deafness in the U.S. school-age population. Am. J. Med. Genet. 46 (5), 486–491. doi:10.1002/ajmg.1320460504
Morton, C. C., and Nance, W. E. (2006). Newborn hearing screening-a silent revolution. N. Engl. J. Med. 354 (20), 2151–2164. doi:10.1056/NEJMra050700
Newton, V. (1990). Hearing loss and waardenburg's syndrome: Implications for genetic counselling. J. Laryngol. Otol. 104 (2), 97–103. doi:10.1017/s002221510011196x
Ng, P. C., and Henikoff, S. (2006). Predicting the effects of amino acid substitutions on protein function. Annu. Rev. Genomics Hum. Genet. 7, 61–80. doi:10.1146/annurev.genom.7.080505.115630
Nourbakhsh, A., Colbert, B. M., Nisenbaum, E., El-Amraoui, A., Dykxhoorn, D. M., Koehler, K. R., et al. (2021). Stem cells and gene therapy in progressive hearing loss: The state of the art. J. Assoc. Res. Otolaryngol. 22 (2), 95–105. doi:10.1007/s10162-020-00781-0
Oza, A. M., DiStefano, M. T., Hemphill, S. E., Cushman, B. J., Grant, A. R., Siegert, R. K., et al. (2018). Expert specification of the ACMG/AMP variant interpretation guidelines for genetic hearing loss. Hum. Mutat. 39 (11), 1593–1613. doi:10.1002/humu.23630
Pan, Z., Xu, H., Tian, Y., Liu, D., Liu, H., Li, R., et al. (2020). Perrault syndrome: Clinical report and retrospective analysis. Mol. Genet. Genomic Med. 8 (10), e1445. doi:10.1002/mgg3.1445
Plevova, P., Paprskarova, M., Tvrda, P., Turska, P., Slavkovsky, R., and Mrazkova, E. (2017). STRC deletion is a frequent cause of slight to moderate congenital hearing impairment in the Czech republic. Otol. Neurotol. 38 (10), e393–e400. doi:10.1097/MAO.0000000000001571
Prezant, T. R., Agapian, J. V., Bohlman, M. C., Bu, X., Oztas, S., Qiu, W. Q., et al. (1993). Mitochondrial ribosomal RNA mutation associated with both antibiotic-induced and non-syndromic deafness. Nat. Genet. 4 (3), 289–294. doi:10.1038/ng0793-289
Qi, J., Fu, X., Zhang, L., Tan, F., Li, N., Sun, Q., et al. (2022). Current AAV-mediated gene therapy in sensorineural hearing loss. Fundam. Res. doi:10.1016/j.fmre.2022.08.015
Read, A. P., and Newton, V. E. (1997). Waardenburg syndrome. J. Med. Genet. 34 (8), 656–665. doi:10.1136/jmg.34.8.656
Richards, S., Aziz, N., Bale, S., Bick, D., Das, S., Gastier-Foster, J., et al. (2015). Standards and guidelines for the interpretation of sequence variants: A joint consensus recommendation of the American College of medical genetics and Genomics and the association for molecular pathology. Genet. Med. 17 (5), 405–424. doi:10.1038/gim.2015.30
Sakuma, N., Moteki, H., Takahashi, M., Nishio, S. Y., Arai, Y., Yamashita, Y., et al. (2016). An effective screening strategy for deafness in combination with a next-generation sequencing platform: A consecutive analysis. J. Hum. Genet. 61 (3), 253–261. doi:10.1038/jhg.2015.143
Sang, S., Ling, J., Liu, X., Mei, L., Cai, X., Li, T., et al. (2019). Proband whole-exome sequencing identified genes responsible for autosomal recessive non-syndromic hearing loss in 33 Chinese nuclear families. Front. Genet. 10, 639. doi:10.3389/fgene.2019.00639
Shearer, A. E., Kolbe, D. L., Azaiez, H., Sloan, C. M., Frees, K. L., Weaver, A. E., et al. (2014). Copy number variants are a common cause of non-syndromic hearing loss. Genome Med. 6 (5), 37. doi:10.1186/gm554
Shen, J., Oza, A. M., Del Castillo, I., Duzkale, H., Matsunaga, T., Pandya, A., et al. (2019). Consensus interpretation of the p.Met34Thr and p.Val37Ile variants in GJB2 by the ClinGen hearing loss Expert panel. Genet. Med. 21 (11), 2442–2452. doi:10.1038/s41436-019-0535-9
Sheppard, S., Biswas, S., Li, M. H., Jayaraman, V., Slack, I., Romasko, E. J., et al. (2018). Utility and limitations of exome sequencing as a genetic diagnostic tool for children with hearing loss. Genet. Med. 20 (12), 1663–1676. doi:10.1038/s41436-018-0004-x
Song, J., Feng, Y., Acke, F. R., Coucke, P., Vleminckx, K., and Dhooge, I. J. (2016). Hearing loss in Waardenburg syndrome: A systematic review. Clin. Genet. 89 (4), 416–425. doi:10.1111/cge.12631
Taiber, S., Cohen, R., Yizhar-Barnea, O., Sprinzak, D., Holt, J. R., and Avraham, K. B. (2021). Neonatal AAV gene therapy rescues hearing in a mouse model of SYNE4 deafness. EMBO Mol. Med. 13 (2), e13259. doi:10.15252/emmm.202013259
Tan, F., Chu, C., Qi, J., Li, W., You, D., Li, K., et al. (2019). AAV-ie enables safe and efficient gene transfer to inner ear cells. Nat. Commun. 10 (1), 3733. doi:10.1038/s41467-019-11687-8
Tao, Y., Liu, X. Y., Yang, L., Chu, C. F., Tan, F. Z., Yu, Z. H., et al. (2022). AAV-ie-K558R mediated cochlear gene therapy and hair cell regeneration. Signal Transduct. Target. Ther. 7 (1), 109. doi:10.1038/s41392-022-00938-8
Thusberg, J., and Vihinen, M. (2006). Bioinformatic analysis of protein structure-function relationships: Case study of leukocyte elastase (ELA2) missense mutations. Hum. Mutat. 27 (12), 1230–1243. doi:10.1002/humu.20407
Tian, Y., Xu, H., Liu, D., Zhang, J., Yang, Z., Zhang, S., et al. (2021). Increased diagnosis of enlarged vestibular aqueduct by multiplex PCR enrichment and next-generation sequencing of the SLC26A4 gene. Mol. Genet. Genomic Med. 9 (8), e1734. doi:10.1002/mgg3.1734
Wang, J., Xiang, J., Chen, L., Luo, H., Xu, X., Li, N., et al. (2021). Molecular diagnosis of non-syndromic hearing loss patients using a stepwise approach. Sci. Rep. 11 (1), 4036. doi:10.1038/s41598-021-83493-6
Xia, L., Zhao, X. L., Ma, X. Y., Hu, Y. N., Zhang, Y., Li, S. Y., et al. (2022). Controllable growth of spiral ganglion neurons by magnetic colloidal nanochains. Nano Today 44, 101507. doi:10.1016/j.nantod.2022.101507
Yokota, Y., Moteki, H., Nishio, S. Y., Yamaguchi, T., Wakui, K., Kobayashi, Y., et al. (2019). Frequency and clinical features of hearing loss caused by STRC deletions. Sci. Rep. 9 (1), 4408. doi:10.1038/s41598-019-40586-7
Yu, X., Lin, Y., Xu, J., Che, T., Li, L., Yang, T., et al. (2020). Molecular epidemiology of Chinese Han deaf patients with bi-allelic and mono-allelic GJB2 mutations. Orphanet J. Rare Dis. 15 (1), 29. doi:10.1186/s13023-020-1311-2
Yuan, Y., You, Y., Huang, D., Cui, J., Wang, Y., Wang, Q., et al. (2009). Comprehensive molecular etiology analysis of nonsyndromic hearing impairment from typical areas in China. J. Transl. Med. 7, 79. doi:10.1186/1479-5876-7-79
Yuan, Y., Yu, F., Wang, G., Huang, S., Yu, R., Zhang, X., et al. (2010). Prevalence of the GJB2 IVS1+1G >A mutation in Chinese hearing loss patients with monoallelic pathogenic mutation in the coding region of GJB2. J. Transl. Med. 8, 127. doi:10.1186/1479-5876-8-127
Zazo Seco, C., Wesdorp, M., Feenstra, I., Pfundt, R., Hehir-Kwa, J. Y., Lelieveld, S. H., et al. (2017). The diagnostic yield of whole-exome sequencing targeting a gene panel for hearing impairment in The Netherlands. Eur. J. Hum. Genet. 25 (3), 308–314. doi:10.1038/ejhg.2016.182
Zhang, S., Xu, H., Tian, Y., Liu, D., Hou, X., Zeng, B., et al. (2021). High genetic heterogeneity in Chinese patients with Waardenburg syndrome revealed by next-generation sequencing. Front. Genet. 12, 643546. doi:10.3389/fgene.2021.643546
Keywords: hearing loss, deafness, molecular diagnosis, stepwise strategy, GJB2 exon 1, STRC gene
Citation: Zeng B, Xu H, Yu Y, Li S, Tian Y, Li T, Yang Z, Wang H, Wang G, Chang M and Tang W (2022) Increased diagnostic yield in a cohort of hearing loss families using a comprehensive stepwise strategy of molecular testing. Front. Genet. 13:1057293. doi: 10.3389/fgene.2022.1057293
Received: 29 September 2022; Accepted: 14 November 2022;
Published: 07 December 2022.
Edited by:
Corrado Romano, University of Catania, ItalyReviewed by:
Miguel Angel Alcántara-Ortigoza, National Institute of Pediatrics, MexicoCopyright © 2022 Zeng, Xu, Yu, Li, Tian, Li, Yang, Wang, Wang, Chang and Tang. This is an open-access article distributed under the terms of the Creative Commons Attribution License (CC BY). The use, distribution or reproduction in other forums is permitted, provided the original author(s) and the copyright owner(s) are credited and that the original publication in this journal is cited, in accordance with accepted academic practice. No use, distribution or reproduction is permitted which does not comply with these terms.
*Correspondence: Mingxiu Chang, MTM4MzgzNzAwNjNAMTYzLmNvbQ==; Wenxue Tang, dHd4QHp6dS5lZHUuY24=
†These authors have contributed equally to this work and share first authorship
Disclaimer: All claims expressed in this article are solely those of the authors and do not necessarily represent those of their affiliated organizations, or those of the publisher, the editors and the reviewers. Any product that may be evaluated in this article or claim that may be made by its manufacturer is not guaranteed or endorsed by the publisher.
Research integrity at Frontiers
Learn more about the work of our research integrity team to safeguard the quality of each article we publish.