- Department of Gynecology, Lianyungang Maternal and Child Health Hospital, Lianyungang, China
Objective: To explore the functions of mRNAs and lncRNAs in the occurrence of uterine leiomyomas (ULs) and further clarify the pathogenesis of UL by detecting the differential expression of mRNAs and lncRNAs in 10 cases of UL tissues and surrounding normal myometrial tissues by high-throughput RNA sequencing.
Methods: The tissue samples of 10 patients who underwent hysterectomy for UL in Lianyungang Maternal and Child Health Hospital from January 2016 to December 2021 were collected. The differentially expressed mRNAs (DEmRNAs) and lncRNAs (DElncRNAs) were identified and further analyzed by Gene Ontology (GO) and Kyoto Encyclopedia of Genes and Genomes (KEGG) pathway enrichment analysis. The protein–protein interaction network (PPI) was constructed in Cytoscape software. Functional annotation of the nearby target cis-DEmRNAs of DElncRNAs was performed with the Database for Annotation, Visualization, and Integrated Discovery (DAVID) (https://david.ncifcrf.gov/). Meanwhile, the co-expression network of DElncRNA–DEmRNA was constructed in Cytoscape software.
Results: A total of 553 DElncRNAs (283 upregulated DElncRNAs and 270 downregulated DElncRNAs) and 3,293 DEmRNAs (1,632 upregulated DEmRNAs and 1,661 downregulated DEmRNAs) were obtained. GO pathway enrichment analysis revealed that several important pathways were significantly enriched in UL such as blood vessel development, regulation of ion transport, and external encapsulating structure organization. In addition, cytokine–cytokine receptor interaction, neuroactive ligand–receptor interaction, and complement and coagulation cascades were significantly enriched in KEGG pathway enrichment analysis. A total of 409 DElncRNAs–nearby-targeted DEmRNA pairs were detected, which included 118 DElncRNAs and 136 DEmRNAs. Finally, we found that the top two DElncRNAs with the most nearby DEmRNAs were BISPR and AC012531.1.
Conclusion: These results suggested that 3,293 DEmRNAs and 553 DElncRNAs were differentially expressed in UL tissue and normal myometrium tissue, which might be candidate-identified therapeutic and prognostic targets for UL and be considered as offering several possible mechanisms and pathogenesis of UL in the future.
Introduction
Uterine leiomyoma (UL), as one of the most common benign tumors in women, arises from the non-neoplastic proliferation of smooth muscle cells within the myometrium (Segars and Al-Hendy, 2017; Yuan et al., 2021). UL occurs in more than 70% of women before menopause, with an incidence of up to 20%–25% in women of reproductive age (Lv et al., 2008; Giuliani et al., 2020). Accumulating clinical evidence has demonstrated that UL has caused a lot of health problems, including uterine bleeding, dysmenorrhoea, repetitive pregnancy loss, menorrhagia, infertility, pelvic pressure, and pain (El et al., 2021; Mathew et al., 2021). Therefore, it is urgent to find new etiological and pathological features of UL to improve the diagnostic efficiency of UL (Yuan et al., 2021). To date, little is known about the pathogenesis of UL, leading to the lack of a valid medical treatment (Falahati et al., 2021). However, there is considerable evidence that estrogen plays a major role in the proliferation of these benign tumors (Ulin et al., 2020). While a strong correlation between ovarian hormone levels or age and the development of UL was found, recent studies of UL have identified novel genes or pathways suggesting the differential expression of mRNAs and lncRNA of this disease progression in order to improve the early detection of tumor and early intervention and treatment of UL (Nothnick, 2016; Falahati et al., 2021).
With the development of high-throughput sequencing, novel bioinformatics approaches, and corresponding experimental validation, a broad spectrum of mRNAs and lncRNAs have been suggested to play an important role in various biological processes (Klapproth et al., 2021; Lin et al., 2022). Long non-coding RNAs (lncRNAs) are a type of long non-coding RNA molecules, more than 200 nucleotides in length, which limited their protein-encoding potential (Zhao et al., 2021). Functionally, accumulating evidences have implicated that lncRNAs are involved in various physiological processes, such as human genome imprinting, chromatin remodeling, X chromosome silencing, epigenetic regulation, and cell cycle and differentiation, and that the abnormal expression and dysfunction of lncRNAs play an functional role in the occurrence and development of a variety of diseases (Wu et al., 2021; Jain et al., 2022). Mechanistically, more and more studies have shown that lncRNAs could participate in gene expression by recruiting the chromosome remodeling complex into a gene promoter (Liu et al., 2021; Ping et al., 2021; Najafi et al., 2022). Also, several lncRNAs fulfill their roles by serving as sponges to arrest miRNA function (Ping et al., 2021). In addition, they also directly interact with some important proteins to augment or attenuate their function (Liu et al., 2021). In addition, the abnormal expression or functional changes in lncRNA expression have become important biological regulators for understanding the molecular mechanisms of these diseases and identifying effective diagnostic biomarkers and therapeutic targets (Hu et al., 2021; Zhao et al., 2021). However, little information available in literature studies about the association between lncRNAs and the progression of UL has yet been elucidated.
In the present study, it is aimed to explore the functions of mRNAs and lncRNAs in the occurrence of uterine leiomyomas (ULs) and further clarify the pathogenesis of UL by detecting the differentially expressed lncRNAs (DElncRNAs) and mRNAs (DEmRNAs) in 10 cases of UL tissues and surrounding normal myometrial tissues by high-throughput RNA sequencing. The results found that a total of 553 DElncRNAs (283 upregulated DElncRNAs and 270 downregulated DElncRNAs) and 3,293 DEmRNAs (1,632 upregulated DEmRNAs and 1,661 downregulated DEmRNAs) were obtained. This study could represent a novel proposal to increase the understanding of the pathogenesis and molecular targeted therapy of UL.
Materials and methods
Ethical compliance
The experiment complied with the Ethics Committee of Lianyungang Maternal and Child Health Hospital (LYG-ME202004), and written informed consent was obtained from each participant before performing surgical procedures.
Patients and samples
A total of 10 UL pairs with tumor samples and surrounding normal myometrial tissue samples were obtained and chosen for high-throughput RNA sequencing at random from UL patients who underwent hysterectomy in Lianyungang Maternal and Child Health Hospital from January 2016 to December 2021. All of these patients with UL were free of treatment before surgery. Pathological diagnostics for UL were independently diagnosed by three experienced pathologists. Fresh tissue samples were immediately frozen in liquid nitrogen after resection from UL patients to protect the protein or RNA from degradation.
RNA extraction and RNA sequencing
According to the manufacturer’s protocol, the total RNA was isolated and extracted from frozen UL tissues and surrounding normal myometrial tissues using the TRIzol reagent (Invitrogen, Carlsbad, CA, United States). One microgram of total RNA was reverse transcribed to high-quality cDNA using a PrimeScript RT Master Mix (Vazyme Biotech, Nanjing, China). With the Agilent 2100 Bioanalyzer, the concentration, integrity, and RNA integrity number (RIN) values of RNA were assessed. RNA sequencing was performed based on the Illumina HiSeq platform (Illumina, Inc., San Diego, CA, United States) in 151-bp sequencing mode. The RNA sequencing was performed with paired ends and 10 G depth. With Base Calling version 0.11.4 (http://www.bioinformatics.babraham.ac.uk/projects/fastqc/), the FASTQ sequence data were acquired from the RNA sequencing data. The Read QC tool in FastQC version 0.11.4 (http://www.bioinformatics.babraham.ac.uk/projects/ fastqc/) was used for the quality control of FASTQ data with Q > 30. Trimming of raw data was performed with cutadapt version 1.16 (http://cutadapt.readthedocs.io). Reads with low quality were removed to obtain the clean reads.
Identification of DEmRNAs and DElncRNAs
In order to align the clean reads with the human reference genome, Ensembl GRCh38.p7 and HISAT2 version 2.1.0 were applied. The expression levels of mRNAs and lncRNAs were normalized and outputted with StringTie version 1.3.3b (http://ccb.jhu.edu/software/stringtie/). Fragments per kilobase of exon per million fragments mapped (FPKM) of lncRNAs and mRNAs were calculated with StringTie. With edgeR version 3.24 (http://www.bioconductor.org/packages/release/bioc/html/edgeR.html), both DEmRNAs and DElncRNAs were obtained with |log2FC| > 1 and a p value < 0.05. By using the R package “pheatmap,” hierarchical clustering analysis of DElncRNAs and DEmRNAs was conducted.
Functional annotation of DEmRNAs
Gene Ontology (GO) and Kyoto Encyclopedia of Genes and Genomes (KEGG) pathway enrichment analyses were performed with the Database for Annotation, Visualization, and Integrated Discovery (DAVID) (https://david.ncifcrf.gov/). Statistical significance was assumed when p < 0.05.
Construction of protein–protein interaction networks
The top 100 upregulated DEmRNAs and 100 downregulated DEmRNAs were submitted to the STRING (https://www.string-db.org/) database. Then, PPI networks were visualized by Cytoscape software (version 3.5.0, http://www.cytoscape. org).
Cis-nearby-targeted DEmRNAs of the DElncRNAs
DEmRNAs transcribed within a 100-kb window upstream or downstream of DElncRNAs were searched, which were defined as cis-nearby-targeted DEmRNAs of DElncRNAs, to obtain the targeted DEmRNAs of DElncRNAs with cis-regulatory effects. The networks were visualized by Cytoscape software. Functional annotation of the cis-nearby-targeted DEmRNAs of the DElncRNAs was conducted with DAVID.
DElncRNA–DEmRNA co-expression networks
To further examine the potential roles of DElncRNAs and DEmRNAs in UL, the DElncRNA–DEmRNA co-expression networks were constructed. DElncRNA–DEmRNA pairs with an absolute value of PCC > 0.95 and p < 0.01 were defined as co-expressed DElncRNA–DEmRNA pairs. By using Cytoscape, the co-expressed DElncRNA–DEmRNA networks were visualized. Functional annotation of the DEmRNAs co-expressed with DElncRNAs was performed with DAVID. A value of p < 0.05 was set as the cut-off for significance.
Results
DElncRNA and DEmRNA expression profiling in uterine leiomyomas
A total of 553 DElncRNAs (283 upregulated DElncRNAs and 270 downregulated DElncRNAs) and 3,293 DEmRNAs (1,632 upregulated and 1,661 downregulated DEmRNAs) were found in UL tissue samples. Furthermore, the top 10 upregulated and downregulated DElncRNAs and DEmRNAs are shown in Table 1 and Table 2, respectively. Hierarchical clustering analysis of the top 100 upregulated and downregulated DElncRNAs and DEmRNAs between UL samples and surrounding normal myometrial tissue samples is presented in Figure 1A and Figure 1B, respectively. The volcano and scatter plots displayed the variation in DElncRNA and DEmRNA expression between UL groups and surrounding normal myometrial tissue groups (Figure 1C and Figure 1D).
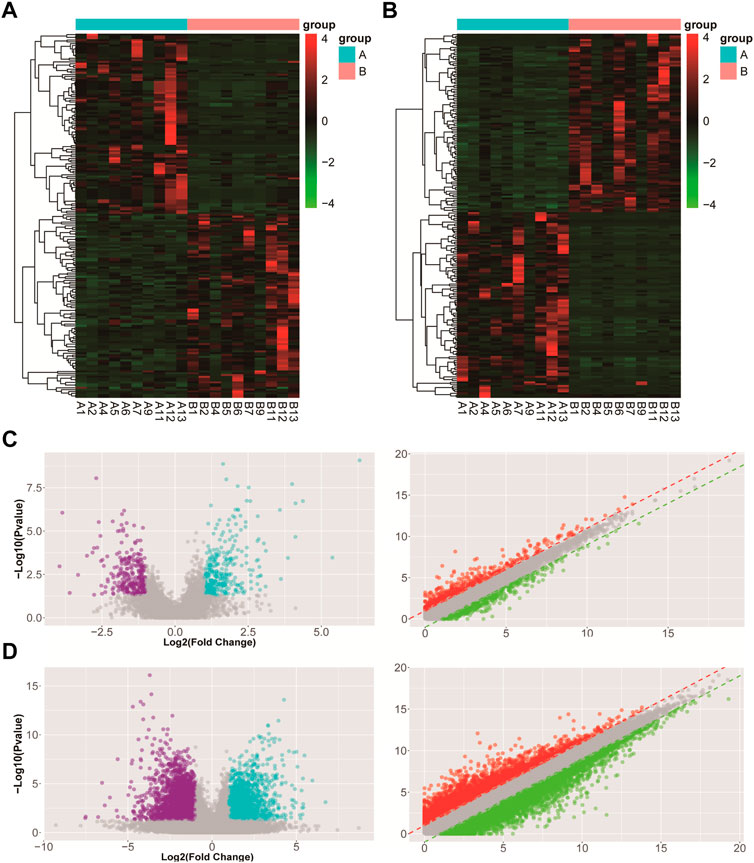
FIGURE 1. DElncRNA and DEmRNA expression patterns in uterine leiomyoma tissues are related to those in surrounding normal myometrial tissues. (A) Hierarchical cluster analysis of all differentially expressed DElncRNAs. (B) Hierarchical cluster analysis of all differentially expressed DEmRNAs; each column represents a sample, and each row represents a lncRNA and mRNA. The color scale indicates relative expression: upregulation (red) and downregulation (blue). A represents the uterine leiomyoma tissue group, and B represents the surrounding normal myometrial tissue group. (C) Volcano and scatter plots demonstrate differential expression of DElncRNAs between two different conditions. (D) Volcano and scatter plots demonstrate differential expression of DEmRNAs between two different conditions; red points indicate upregulated expression, while green points indicate downregulated expression. The values plotted on the X and Y axes are the averaged normalized signal values of each group (log2 scaled). FC ≥ 2 and p ≤ 0.05 were regarded as the differentially expressed DElncRNAs and DEmRNAs.
Functional annotation analysis
GO and KEGG pathway enrichment analyses were performed for the DEmRNAs. GO analysis revealed that mRNAs were downregulated in UL and mainly involved in a number of signaling pathways, including blood vessel development (p = 1.21 E-21), regulation of ion transport (p = 1.94E-16), and external encapsulating structure organization (p = 8.05E-16) (Figure 2A). KEGG pathway analysis revealed that the deregulated mRNAs were primarily enriched in signaling pathways associated with cytokine–cytokine receptor interaction (p = 3.10E-21), neuroactive ligand–receptor interaction (p = 2.67E-21), and complement and coagulation cascades (CAMs) (p = 1.50E-18) (Figure 2B).
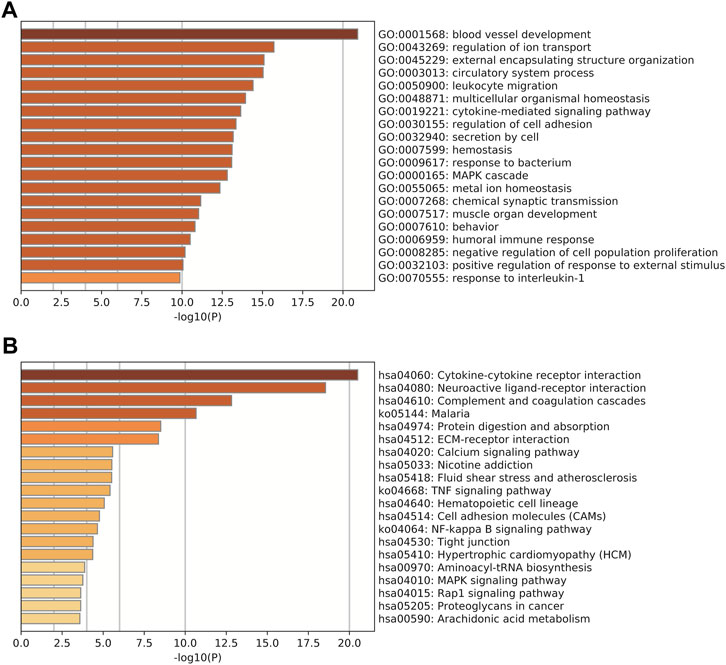
FIGURE 2. Functional annotation analysis for the validated DEmRNA gathering genes. (A) GO analysis. (B) KEGG pathway analysis. The vertical axis shows the annotated functions of the target genes. The horizontal axes show the enrichment score (−log10 transformed p value) and the gene number of each cluster, respectively. Only the top 10 significantly enriched clusters are included.
Protein–protein interaction networks
PPI networks were constructed and included 313 nodes and 347 edges. NR3C1 (degree = 57), BIRC3 (degree = 27), ARRB1 (degree = 24), KRT8 (degree = 20), and APOA1 (degree = 15) were the top five hub proteins in the PPI networks (Figure 3).
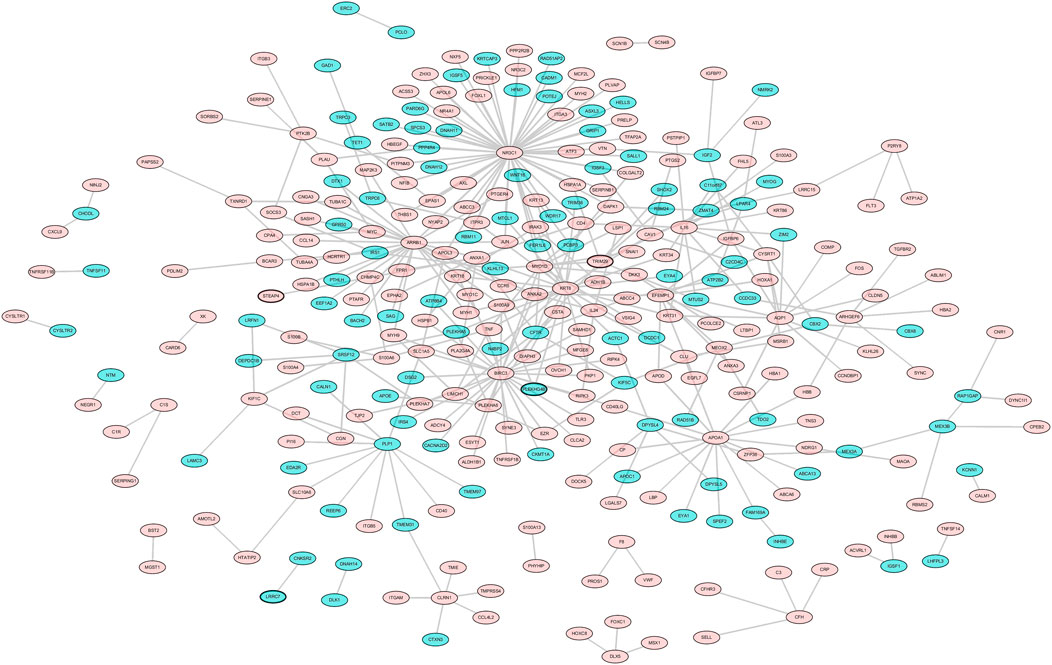
FIGURE 3. Protein–protein interaction (PPI) network analysis. The network comprising the top upregulated (red triangles) and downregulated lncRNAs (green inverted arrows) and their target mRNAs is presented.
Cis-nearby-targeted DEmRNAs of DElncRNAs
There were 409 DElncRNA–nearby-targeted DEmRNA pairs, including 118 DElncRNAs and 136 DEmRNAs (Figure 4). The top two DElncRNAs with the most nearby DEmRNAs were BISPR and AC012531.1, which contained four DElncRNAs and four nearby DEmRNAs, respectively. Blood vessel development (p = 6.61E-10), ventricular septum development (p = 2.21E-5), and skeletal system development (p = 3.08E-06) were significantly enriched GO terms (Figure 5A). Hypertrophic cardiomyopathy (p = 6.54E-04), the TNF signaling pathway (p = 1.75E-03), and chemical carcinogenesis (p = 6.95E-03) were significantly enriched KEGG pathways (Figure 5B).
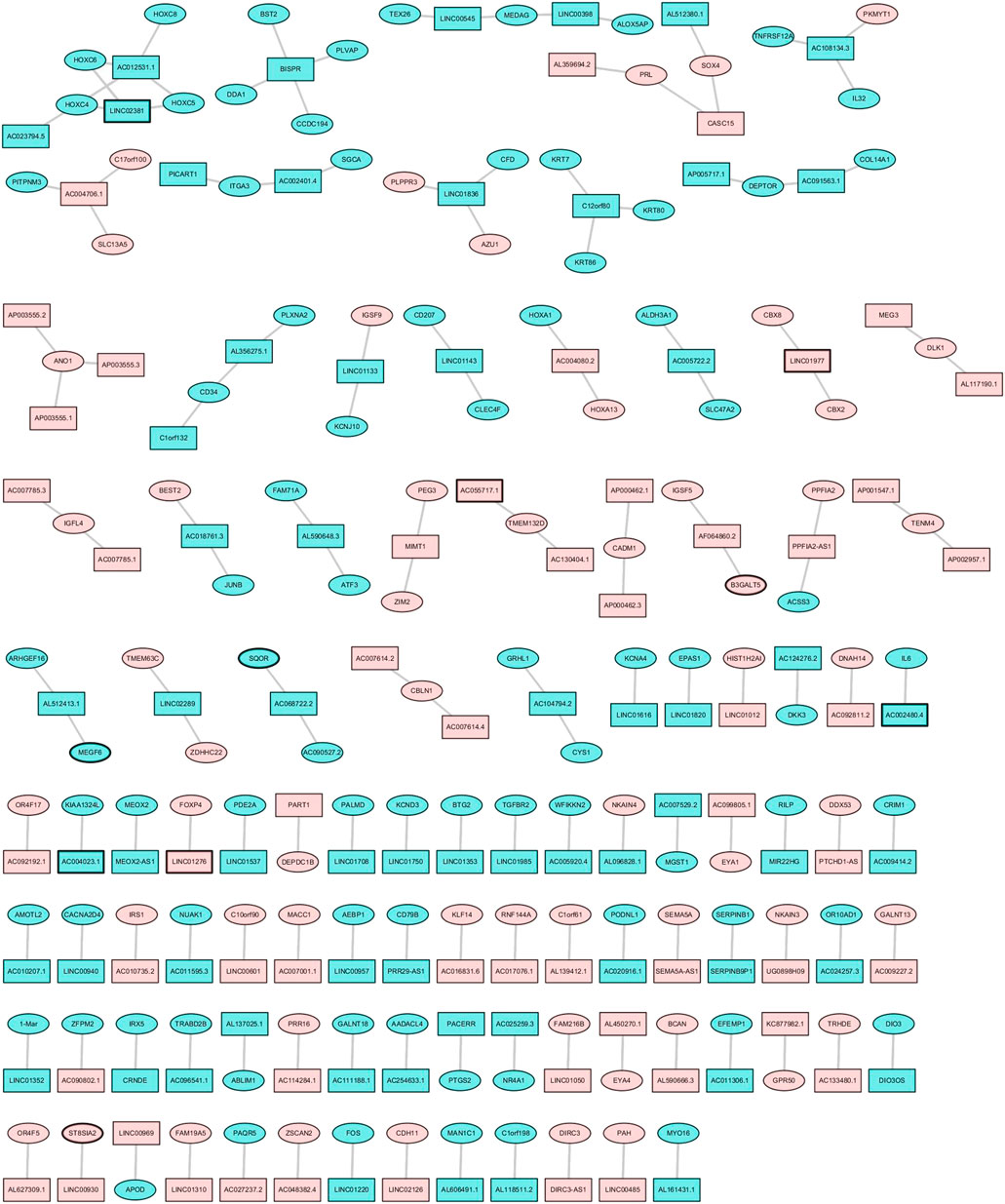
FIGURE 4. Cis-nearby-targeted DEmRNAs of DElncRNAs. There were 409 DElncRNA–nearby-targeted DEmRNA pairs, including 118 DElncRNAs and 136 DEmRNAs.
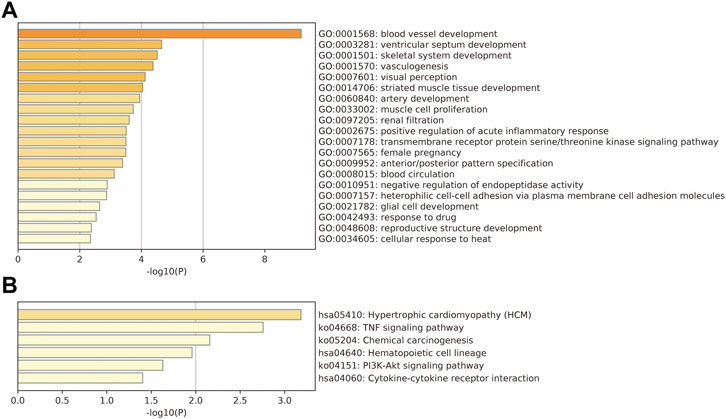
FIGURE 5. Functional annotation analysis for the validated cis-nearby-targeted DEmRNAs of DElncRNA gathering genes. (A) GO analysis. (B) KEGG pathway analysis. The vertical axis shows the annotated functions of the target genes. The horizontal axes show the enrichment score (−log10 transformed p value) and the gene number of each cluster, respectively. Only the top 10 significantly enriched clusters are included.
Discussion
UL, as a common disease in women, occurs in women of reproductive age (Segars and Al-Hendy, 2017; Mathew et al., 2021). Most of the patients with UL do not affect the physical health of women, but there is a need for surgery for UL patients with fertility requirements, which cause female menorrhagia, pelvic pain, and compression symptoms. However, the pathogenesis of UL is not fully understood. Therefore, it is necessary to identify the molecular mechanisms of disease progression that are useful in the diagnosis and treatment of UL (Segars and Al-Hendy, 2017; Ulin et al., 2020; Yuan et al., 2021). Recently, the abnormal expression of lncRNAs and mRNAs has been verified in various diseases, and the growing evidence indicates that lncRNAs and mRNAs are involved in the progression of diseases (Nothnick, 2016; Jain et al., 2022; Lin et al., 2022). LncRNAs are a class of non-coding RNAs with more than 200 nucleotides that are considered to be a transcriptional byproduct and do not encode proteins (Wu et al., 2021). In these years, lncRNAs, which were considered as byproducts of RNA polymerase II, have emerged to play important roles in regulating various physiological and pathological processes (Jain et al., 2022; Lin et al., 2022). In addition, more and more studies have demonstrated that lncRNAs are closely associated with the occurrence and development of tumors, aging, and inflammation, with the rapid development of molecular biology and genetic diagnostic techniques (Klapproth et al., 2021; Zhao et al., 2021). A great number of studies have found the dysregulation of lncRNAs and mRNAs involved in many cell-signaling pathways and participated in the occurrence and development of UL (Nothnick, 2016; Lin et al., 2022). For example, the increased lncRNA XIST in UL could lead to reduced expression of miR-29c and miR-200c by targeting COL1A1, COL3A1, and FN1 (Chuang et al., 2021). In addition, lncRNA AL445665.1–4 was found to be involved in the development of UL through interacting with miR-146b-5p (Yang et al., 2019). At last, lncRNA APTR promoted the proliferation of UL cells through the Wnt pathway by targeting ERα (Zhou et al., 2021). All this indicated that lncRNAs and mRNAs could be used as novel biomarkers for the diagnosis and treatment of UL.
Generally speaking, lncRNAs activate or inhibit gene expression through the recruitment of various remodeling complexes to a gene promoter, ultimately affecting epigenetics (Klapproth et al., 2021; Lin et al., 2022). Accumulating evidence showed that different biological functions of lncRNAs mainly depend on their clearly different subcellular localization (Nothnick, 2016). Cytoplasmic lncRNAs could function as decoys for miRNAs, leading to the regulation of mRNA stability or translation and finally influencing signaling pathways (Chuang et al., 2021; Zhao et al., 2021). In this study, a total of 553 DElncRNAs (283 upregulated DElncRNAs and 270 downregulated DElncRNAs) and 3,293 DEmRNAs (1,632 upregulated and 1,661 downregulated DEmRNAs) were found in UL tissue samples. To further dissect the function of those target genes contained in the DElncRNA and DEmRNA networks, GO analysis revealed that mRNAs were deregulated in UL and were mainly involved in a number of signaling pathways, including blood vessel development, regulation of ion transport, and external encapsulating structure organization. KEGG pathway analysis revealed that the deregulated mRNAs were primarily enriched in signaling pathways associated with cytokine–cytokine receptor interaction, neuroactive ligand–receptor interaction, and complement and coagulation cascades. The main blood supply for uterine fibroids is from the uterine artery and its branches. Blood vessels deliver oxygen and nutrients to every part of the body. The dysfunction of blood vessel formation was responsible for the initiation and progression of diseases including cancer (Naito et al., 2020). Elevated vascular endothelial-derived growth factor (VEGF) was found in UL compared with the uterine myometrial layer, suggesting that blood vessel development may participate in the development and growth of UL (Wei et al., 2006; Tal and Segars, 2014; Naito et al., 2020).
In this study, we speculated that some lncRNAs might regulate genes in a cis-regulatory fashion. For example, LncRNA BST2 interferon-stimulated positive regulator (BISPR) may regulate the production of inflammatory mediators and promote tumorigenesis (Pham et al., 2017). Many studies reported that the BISPR was involved in multiple malignant behaviors for tumor development, including renal cell carcinoma and oral cavity cancer (Fang et al., 2014; Pham et al., 2017). Furthermore, BISPR increased the propagation of cancer cells in thyroid papillary carcinoma by inhibiting miR-21-5p (Zhang et al., 2018). In this study, we found that the top two DElncRNAs with the most nearby DEmRNAs were BISPR and AC012531.1, which contained four DElncRNAs and four nearby DEmRNAs, respectively. Blood vessel development, ventricular septum development, and skeletal system development were significantly enriched GO terms. Hypertrophic cardiomyopathy, the TNF signaling pathway, and chemical carcinogenesis were significantly enriched KEGG pathways. Taken together, our findings revealed that lncRNAs might act as ceRNAs to impact blood vessel development and hypertrophic cardiomyopathy during the development of UL.
However, our study has several limitations. First, RNA-seq and mRNA-seq were performed to predict the potential roles of lncRNAs and mRNAs by bioinformatics analysis. Although some experimentally validated gene regulatory networks appeared in our analysis, it is still necessary to validate these results with related experiments. In addition, it remains to be further validated because of the relatively small sample size, contributing to potentially limiting the statistical power to investigate the real association. Hence, a larger sample size is needed to verify our results. In this study, high-throughput RNA sequencing technology was applied to screen lncRNAs specifically expressed in UL tissues, and bioinformatics methods were used to analyze the key pathways enriched by specific lncRNAs in GO terms and KEGG pathways. In the next step, we will still expand the sample size of clinical studies, further verify the differential expression of lncRNAs and their target genes between UL tissues and surrounding normal myometrial tissues, and verify the regulation of lncRNAs on their target genes by in vitro cell experiments in order to discuss the pathogenesis of UL from the perspective of epigenetics and provide a preliminary theory for the prevention and treatment of UL.
Conclusion
In summary, these research results suggest that 3,293 DEmRNAs and 553 DElncRNAs are differentially expressed in UL tissues and surrounding normal myometrial tissues. The DElncRNA–DEmRNA co-expression networks and PPI networks provide new insight into the biological processes and underlying mechanisms of UL. This study might also highlight lncRNAs as candidate-identified therapeutic and prognostic targets for UL and offer several possible mechanisms and pathogenesis of UL in the future.
Data availability statement
The data presented in the study are deposited in the SRA repository, accession number PRJNA12360444.
Ethics statement
The studies involving human participants were reviewed and approved by the experiment complied with the Ethics Committee of Lianyungang Maternal and Child Health Hospital (LYG-ME202004), and written informed consent was obtained from each participant before performing surgical procedures. The patients/participants provided their written informed consent to participate in this study. Written informed consent was obtained from the individual(s) for the publication of any potentially identifiable images or data included in this article.
Author contributions
All the authors clinically managed the patients. MH and YW conducted the literature research. FM, YJ, and XC compiled the manuscript. All the authors read and approved the final manuscript.
Funding
This work was supported by the Jiangsu Provincial Health Commission (LGY2020054).
Conflict of interest
The authors declare that the research was conducted in the absence of any commercial or financial relationships that could be construed as a potential conflict of interest.
Publisher’s note
All claims expressed in this article are solely those of the authors and do not necessarily represent those of their affiliated organizations, or those of the publisher, the editors, and the reviewers. Any product that may be evaluated in this article, or claim that may be made by its manufacturer, is not guaranteed or endorsed by the publisher.
References
Chuang, T. D., Rehan, A., and Khorram, O. (2021). Functional role of the long noncoding RNA X-inactive specific transcript in leiomyoma pathogenesis. Fertil. Steril. 115, 238–247. doi:10.1016/j.fertnstert.2020.07.024
El, S. M., Saha, S. K., Afrin, S., Islam, M. S., and Borahay, M. A. (2021). Wnt/β-catenin signaling pathway in uterine leiomyoma: Role in tumor biology and targeting opportunities. Mol. Cell. Biochem. 476, 3513–3536. doi:10.1007/s11010-021-04174-6
Falahati, Z., Mohseni-Dargah, M., and Mirfakhraie, R. (2021). Emerging roles of long non-coding RNAs in uterine leiomyoma pathogenesis: A review. Reprod. Sci. 29, 1086–1101. doi:10.1007/s43032-021-00571-w
Fang, K. H., Kao, H. K., Chi, L. M., Liang, Y., Liu, S. C., Hseuh, C., et al. (2014). Overexpression of BST2 is associated with nodal metastasis and poorer prognosis in oral cavity cancer. Laryngoscope 124, E354–E360. doi:10.1002/lary.24700
Giuliani, E., As-Sanie, S., and Marsh, E. E. (2020). Epidemiology and management of uterine fibroids. Int. J. Gynaecol. Obstet. 149, 3–9. doi:10.1002/ijgo.13102
Hu, S. P., Ge, M. X., Gao, L., Jiang, M., and Hu, K. W. (2021). LncRNA HCP5 as a potential therapeutic target and prognostic biomarker for various cancers: A metaanalysis and bioinformatics analysis. Cancer Cell Int. 21, 686. doi:10.1186/s12935-021-02404-x
Jain, N., Gupta, P., Sahoo, S., and Mallick, B. (2022). Non-coding RNAs and their cross-talks impacting reproductive health of women. Wiley Interdiscip. Rev. RNA 13, e1695. doi:10.1002/wrna.1695
Klapproth, C., Sen, R., Stadler, P. F., Findeiss, S., and Fallmann, J. (2021). Common features in lncRNA annotation and classification: A survey. Noncoding. RNA 7, 77. doi:10.3390/ncrna7040077
Lin, Q., Shi, Y., Liu, Z., Mehrpour, M., Hamai, A., and Gong, C. (2022). Non-coding RNAs as new autophagy regulators in cancer progression. Biochim. Biophys. Acta. Mol. Basis Dis. 1868, 166293. doi:10.1016/j.bbadis.2021.166293
Liu, J., Yang, L. Z., and Chen, L. L. (2021). Understanding lncRNA-protein assemblies with imaging and single-molecule approaches. Curr. Opin. Genet. Dev. 72, 128–137. doi:10.1016/j.gde.2021.11.005
Lv, J., Zhu, X., Dong, K., Lin, Y., Hu, Y., and Zhu, C. (2008). Reduced expression of 14-3-3 gamma in uterine leiomyoma as identified by proteomics. Fertil. Steril. 90, 1892–1898. doi:10.1016/j.fertnstert.2007.08.039
Mathew, R. P., Francis, S., Jayaram, V., and Anvarsadath, S. (2021). Uterine leiomyomas revisited with review of literature. Abdom. Radiol. 46, 4908–4926. doi:10.1007/s00261-021-03126-4
Naito, H., Iba, T., and Takakura, N. (2020). Mechanisms of new blood-vessel formation and proliferative heterogeneity of endothelial cells. Int. Immunol. 32, 295–305. doi:10.1093/intimm/dxaa008
Najafi, S., Tan, S. C., Raee, P., Rahmati, Y., Asemani, Y., Lee, E., et al. (2022). Gene regulation by antisense transcription: A focus on neurological and cancer diseases. Biomed. Pharmacother. 145, 112265. doi:10.1016/j.biopha.2021.112265
Nothnick, W. B. (2016). Non-coding RNAs in uterine development, function and disease. Adv. Exp. Med. Biol. 886, 171–189. doi:10.1007/978-94-017-7417-8_9
Pham, Q. T., Oue, N., Yamamoto, Y., Shigematsu, Y., Sekino, Y., Sakamoto, N., et al. (2017). The expression of BTS-2 enhances cell growth and invasiveness in renal cell carcinoma. Anticancer Res. 37, 2853–2860. doi:10.21873/anticanres.11637
Ping, J., Li, L., Dong, Y., Wu, X., Huang, X., Sun, B., et al. (2021). The role of long Non-Coding RNAs and circular RNAs in bone regeneration: Modulating MiRNAs function. J. Tissue Eng. Regen. Med. 16, 227–243. doi:10.1002/term.3277
Segars, J. H., and Al-Hendy, A. (2017). Uterine leiomyoma: New perspectives on an old disease. Semin. Reprod. Med. 35, 471–472. doi:10.1055/s-0037-1606569
Tal, R., and Segars, J. H. (2014). The role of angiogenic factors in fibroid pathogenesis: Potential implications for future therapy. Hum. Reprod. Update 20, 194–216. doi:10.1093/humupd/dmt042
Ulin, M., Ali, M., Chaudhry, Z. T., Al-Hendy, A., and Yang, Q. (2020). Uterine fibroids in menopause and perimenopause. Menopause 27, 238–242. doi:10.1097/GME.0000000000001438
Wei, J. J., Zhang, X. M., Chiriboga, L., Yee, H., Perle, M. A., and Mittal, K. (2006). Spatial differences in biologic activity of large uterine leiomyomata. Fertil. Steril. 85, 179–187. doi:10.1016/j.fertnstert.2005.07.1294
Wu, M., Yang, L. Z., and Chen, L. L. (2021). Long noncoding RNA and protein abundance in lncRNPs. Rna 27, 1427–1440. doi:10.1261/rna.078971.121
Yang, E., Xue, L., Li, Z., and Yi, T. (2019). Lnc-AL445665.1-4 may be involved in the development of multiple uterine leiomyoma through interacting with miR-146b-5p. Bmc Cancer 19, 709. doi:10.1186/s12885-019-5775-1
Yuan, Y., Chen, L., Zhao, T., and Yu, M. (2021). Pathogenesis, diagnosis and treatment of uterine lipoleiomyoma: A review. Biomed. Pharmacother. 142, 112013. doi:10.1016/j.biopha.2021.112013
Zhang, H., Cai, Y., Zheng, L., Zhang, Z., Lin, X., and Jiang, N. (2018). LncRNA BISPR promotes the progression of thyroid papillary carcinoma by regulating miR-21-5p. Int. J. Immunopathol. Pharmacol. 32, 2058738418772652. doi:10.1177/2058738418772652
Zhao, S., Heng, N., Weldegebriall, S. B., Wang, H., and Zhu, H. (2021). Long noncoding RNAs: Recent insights into their role in male infertility and their potential as biomarkers and therapeutic targets. Int. J. Mol. Sci. 22, 13579. doi:10.3390/ijms222413579
Keywords: uterine leiomyomas, long non-coding RNA, mRNAs, high-throughput sequencing, miRNAs
Citation: Meng F, Ji Y, Chen X, Wang Y and Hua M (2023) An integrative analysis of an lncRNA–mRNA competing endogenous RNA network to identify functional lncRNAs in uterine leiomyomas with RNA sequencing. Front. Genet. 13:1053845. doi: 10.3389/fgene.2022.1053845
Received: 29 September 2022; Accepted: 18 November 2022;
Published: 04 January 2023.
Edited by:
Xiao-Mei Li, The First Affiliated Hospital of University of Science and Technology of China (USTC), ChinaReviewed by:
Qi Shen, Wenzhou Medical University, ChinaYang Shen, Zhongda Hospital, Southeast University, China
Copyright © 2023 Meng, Ji, Chen, Wang and Hua. This is an open-access article distributed under the terms of the Creative Commons Attribution License (CC BY). The use, distribution or reproduction in other forums is permitted, provided the original author(s) and the copyright owner(s) are credited and that the original publication in this journal is cited, in accordance with accepted academic practice. No use, distribution or reproduction is permitted which does not comply with these terms.
*Correspondence: Maofang Hua, 18705130566@163.com