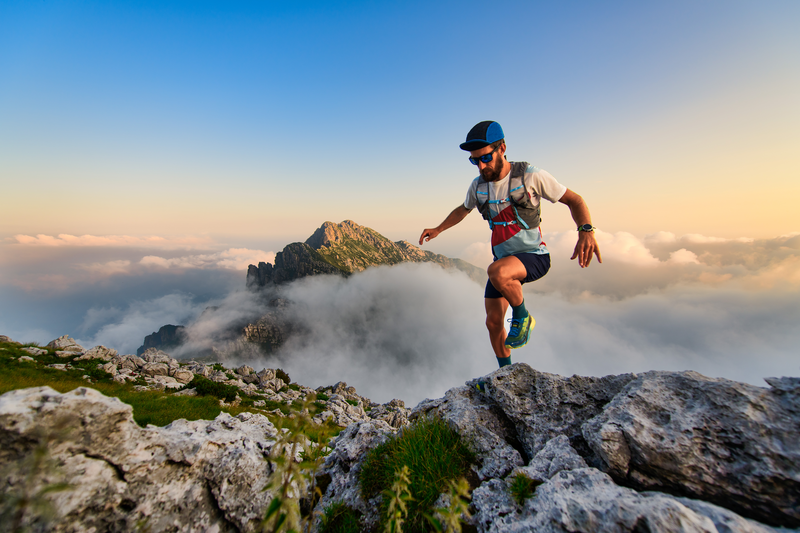
95% of researchers rate our articles as excellent or good
Learn more about the work of our research integrity team to safeguard the quality of each article we publish.
Find out more
CASE REPORT article
Front. Genet. , 18 November 2022
Sec. Genetics of Common and Rare Diseases
Volume 13 - 2022 | https://doi.org/10.3389/fgene.2022.1005573
A twelve-year-old patient with a previous clinical diagnosis of spondylocostal skeletal dysplasia and moderate intellectual disability was genetically analyzed through next generation sequencing of a targeted gene panel of 179 genes associated to skeletal dysplasia and mucopolysaccharidosis in order to stablish a precision diagnosis. A homozygous nonsense [c.62C>G; p.(Ser21Ter)] mutation in DYM gene was identified in the patient. Null mutations in DYM have been associated to Dyggve-Melchior-Clausen syndrome, which is a rare autosomal-recessive disorder characterized by skeletal dysplasia and mental retardation, compatible with the patient´s phenotype. To confirm the pathogenicity of this mutation, a segregation analysis was carried out, revealing that the mutation p(Ser21Ter) was solely inherited from the father, who is a carrier of the mutation, while the mother does not carry the mutation. With the suspicion that a paternal disomy could be causing the disease, a series of microsatellite markers in chromosome 18, where the DYM gene is harbored, was analyzed in all the members of the family. Haplotype analysis provided strong evidence of paternal isodisomy and heterodisomy in that chromosome, confirming the pathological effect of this mutation. Furthermore, the patient may have a compromised expression of the ELOA3 gene due to modifications in the genomic imprinting that may potentially increase the risk of digestive cancer. All these results highlight the importance of obtaining a precision diagnosis in rare diseases.
Dyggve-Melchior-Clausen (DMC) syndrome [MIM# 223800] is a rare disease included in the heterogenous group of spondylo-epi-metaphysal dysplasias disorders, all of them defined by the combination of vertebral, epiphyseal and metaphyseal abnormalities. DMC syndrome is characterized by short trunk dwarfism, microcephaly and mental retardation (Beighton, 1990). The prevalence of this syndrome is < 1/1,000,000, and there are about 100 cases reported worldwide (Orphanet, 2022).
DMC syndrome is caused by homozygous null mutations in the DYM gene (Dimitrov et al., 2009; Dupuis et al., 2015). This gene (NCBI ID: 54,808) is located in chromosome 18 (18q21.1) (Cohn et al., 2003; el Ghouzzi et al., 2003) and is consists of 17 exons which encode dymeclin, a 669-amino acid protein whose function remains unknown. However, it has been proposed that it may be an integral protein of the endoplasmic reticulum membrane that could play a role in the transport of intracellular compounds. Furthermore, it could also have a critical role in both the formation and function of the Golgi apparatus and in the tracking of associated vesicles.
Dymeclin is highly conserved across species, but it does not belong to any identified protein family. This protein is expressed at various embryonic stages of human brain development, chondrocytes, osteoblasts and skin fibroblasts (el Ghouzzi et al., 2003).
Uniparental disomy (UPD) refers to the inheritance of both copies of a chromosome from one single parent. This phenomenon usually occurs due to aberrations during meiosis, and two main subgroups can be distinguished: uniparental isodisomy (UPiD), where both allele copies are identical (they originated from the sister chromatids); and uniparental heterodisomy (UPhD), where the pair of homologous chromosomes of a parent is inherited. Recombination processes during meiosis can result in partial UPiD, partial UPhD or mixed UPiD-UPhD (Benn, 2021). The overall prevalence of UPD in the general population is estimated to be 1:2,000 births, whereas the prevalence of UPD as a cause of rare diseases ranges from 1:3,500 to 1:5,000 (Robinson, 2000; Liehr, 2022). To date, only one case of rare disease, concretely hearing loss, have been reported to be caused by a mutation in LOXHD1 gene and UPD of the chromosome 18 (Morgan et al., 2018).
On the other hand, imprinting is a phenomenon that occurs in some developmental genes and consists in the silencing of one of the copies of the gene (maternal or paternal), thus having a monoallelic expression under normal conditions. Genes with imprinting have been described in all human chromosomes (Geneimprint, 2022). When UPiD occurs in a region with imprinted genes, the expression of those can vary drastically and cause diseases such as the well-known Prader-Willi syndrome, Angelman syndrome or Silver-Russell syndrome, among others (Liehr, 2022). To date, only three imprinted genes (TCEB3C/ELOA3, ZNF396 and PARD6G) have been described in chromosome 18 (Geneimprint, 2022).
The case reported here describes the precision diagnosis performed in a patient with DMC syndrome in which the mutation p.(Ser21Ter) was identified in the DYM gene, a gene associated with this pathology. The genetic analysis of this result confirms the pathogenic nature of the mutation and reveals that the homozygous state of the mutation was caused by a paternal mixed UPiD-UPhD in chromosome 18, a mechanism that had not been described in this chromosome until now. In addition, the risk of digestive cancer could be particularly increased in this patient due to modifications of the paternal imprinting in the isodisomic region, specifically where a tumor suppressor gene (ELOA3) is located.
The subject was evaluated at the Complejo Hospitalario Universitario de Albacete (Spain).
First child of 23-year-old, healthy and non-consanguineous parents.
The pregnancy had a normal course until 33+2 weeks when preeclampsia was detected and corticosteroids were used to promote lung maturation in the fetus. Delivery occurred at 33+5 weeks by cesarean section with a cephalic presentation, clear amniotic fluid (Apgar score: 7/8) and a constitutional hypotony in relation to the prematurity.
The patient was admitted to Neonatology Service due to prematurity. The examination was normal, without dysmorphias and with parameters in accordance with prematurity. On the second day of life, jaundice was detected with a maximum bilirubin level of 12.6 mg/dl. The patient required phototherapy for 72 h and was discharged after 20 days of life with no other incidents. Before the patient was 1 year old, the family moved to their country of origin (Ecuador).
At 3 years old, the parents detected a significant developmental delay (free walking at 20 months and language delay limited to referential disyllables) and decided to return to Spain. Psychological examination revealed hyperactivity and an attention deficit disorder. Physical examination showed a weight of 9,600 g (<p3), a cranial perimeter of 46 cm (<p3), a height of 84 cm (<p3), short trunk and neck dwarfism. At that moment, the patient was diagnosed with microcephaly, kyphosis, peptum carinatum, flat feet and a spondylo-epi-metaphyseal dysplasia. Other complementary examinations were performed such as karyotype, hemogram and biochemistry, that were normal. The metabolomic study also detected normal levels of amino acids, organic acids, and sialotransferrins. Lysosomal diseases such as the Morquio syndrome were discarded due to the absence of corneal clouding, deafness, mucopolysaccharidosis or valvular disease. Pathologic skeletal X-rays showed generalized platyspondyly of the whole vertebral rachis, bilateral coxofemoral dysplasia, and metaphyseepiphyseal widening of long bones. According to this evidence, a Spondyloepiphyseal Dysplasia diagnosis was made. However, the patient did not yet have a precision diagnosis. Multidisciplinary follow-up was carried out in the Dysmorphology/Neuropediatrics, Endocrinology and Pediatric Orthopedics Services up to 9 years old. Two years later, he underwent surgery for flat feet and two subtalar implants were inserted in both feet. But it is not until the child is 12 years old that the patient returns to the Dysmorphology/Neuropediatrics consultation. The child had the same clinical characteristics that had been previously detected, but, in addition, he was diagnosed with moderate intellectual disability. At that time, a NGS panel of 179 genes associated with skeletal dysplasia and mucopolysaccharidosis was requested by the doctor.
The NGS company in charge of the sequencing reported a homozygous mutation in the DYM gene and classified it as a variant of uncertain significance. This variant was previously found in heterozygous state in a single Asian individual (frequency of 4 × 10−6) according gnomAD data base. A family segregation study was recommended by the company to confirm the pathogenic nature of the mutation.
In light of these results, in 2017 the case was referred to our Medical Genetics laboratory, where the mutation was analyzed, and other genetic studies were performed so as to offer a precision diagnosis and genetic counselling to the family. Thus, the variant was reclassified as pathogenic and DMC syndrome was confirmed. At the same time, the UPiD-UPhD and the genetic mechanism responsible for the disease in this patient, as well as the clinical consequences of this condition, were reported to the Dysmorphology/Neuropediatrics Service of the Complejo Hospitalario Universitario de Albacete, putting an end to 13 years of diagnostic odyssey (Figure 1).
FIGURE 1. Diagnostic odyssey timeline of the patient. The figure shows the different stages that this patient has undergone since the appearance of the first symptoms until a precise diagnosis has been achieved. Once the patient is given a diagnosis, the main challenge is to develop a precision treatment.
Four family members (parents and their two sons) were recruited in the Department of Pediatrics of the “Complejo Hospitalario Universitario de Albacete”, Spain, for genetic analysis. The study followed the tenets of the Declaration of Helsinki and informed consents were obtained from the parents. The patient and his family were clinically evaluated by experienced neuropediatricians. Except for the patient, the rest of the family members did not report any pathology of interest at the time of the study.
DNA extraction: Genomic DNA was obtained from peripheral blood leukocytes in all family members using the E.Z.N.A. Blood DNA kit (Omega Bio-tek) according to manufacturer’s protocol.
NGS technology: a whole exome sequencing (WES) was carried out and subsequently, a panel of 179 genes associated with skeletal dysplasia and mucopolysaccharidosis was filtered in the case reported by an external company using an Ion Proton (Life Technologies) platform (Supplementary Table S1). The exome capture was designed using the Ion AmpliSeq™ Exome technology (Life Technologies). The analysis was addressed to the identification of variants located in exonic regions or in splice-site junctions that involve a protein modification (nonsense/missense mutations, as well as nucleotide insertions, deletions or indels) with a frequency higher than 40% of the reads.
Sequencing data were aligned to the reference genome (hg19) using the TMAP-Ion-Aligment software (Life Technologies). Variants were further annotated and analyzed using the latest available version of ION Reporter Software (Life Technologies). 99% of targeted sequences were reliably sequenced, with at least 10X coverage.
Filtering out of variants was carried out following these criteria: 1) variants with a high frequency in population (Minor Allele Frequency (MAF) > 1%), and 2) variants detected in genes associated with clinical profiles not related with the observed in our patient were not prioritized to be disease-causing. Applying the first filtering, we eliminated single nucleotide polymorphisms (SNPs) and common variants that have been previously described as polymorphisms in population databases and no association with any clinical phenotype has been reported in the literature. Applying the second criteria, three missense mutations with a low allelic frequency (<0.01; gnomAD) present in three different genes (SLC34A3, TRPV4 and CTSK) were eliminated due to the lack of genotype-phenotype correlation in the patient.
Segregation analysis: p.(Ser21Ter) mutation was confirmed in the patient and analysed in the rest of the family members by PCR and Sanger sequencing. The following PCR primers were designed in the intronic regions flanking exon 2 of the DYM gene (transcript version ENST00000269445.10; DYM-201):
FW-5′GTTGGGATTACAGGCGTGAG3’; RV-5′TTCCAGAACGGGTCATTCTC3’.
Terminator cycle sequencing was carried out using the BigDye kit (version 3.1; Applied Biosystems) and the products of sequencing reactions were analysed in a 3730xl automated DNA analyser (Applied Biosystems). Chromas Pro software was used for sequence analysis.
Seventeen microsatellite markers were evaluated in all family members’ chromosome 18. Markers were amplified by PCR using the primers and conditions detailed in Table 1.
TABLE 1. Primer sequences and annealing temperatures used to amplify microsatellite markers of chromosome 18.
After amplification, 100 ng of PCR product was mixed with 30 µL of formamide and 0.3 µL of GENESCAN® 400HD size standard (Applied Biosystems). Fragment analyses were carried out in a 3730xl DNA sequencer (Applied Biosystems) and Peak Scanner v1.0 software was used to visualize fragment lengths.
Next generation sequencing company identified a variant of uncertain significance in the patient, NG_009239.2(NM_017653.6):c.62C>G, found in homozygous state in the exon 2 of the DYM gene. This change predicts the nonsense mutation NP_060123.3:p.(Ser21Ter) in the dymeclin protein. Although null DYM mutations cause DMC syndrome, whose phenotype is compatible with the clinical features of the patient, it was essential to confirm the pathogenicity of the mutation, as it had not been previously described. Therefore, a segregation study was carried out in all family members (Figure 2). The results revealed that the patient inherited the mutation only from his father, who is a carrier of the mutation, but not from his mother, suggesting a possible paternal UPiD. In such a scenario, the segregation pattern would still be consistent with the pathological condition of the mutation, but to achieve a precision diagnosis in the child it was necessary to clarify the molecular mechanism underlying the disease.
FIGURE 2. Segregation of the mutation in the family. Filled symbols represent affected individuals, and the arrow indicates the proband subject of this study (individual II:1). Genotypes are indicated below the symbols.
Paternal isodisomy is usually originated by an abnormal chromosomal segregation during meiosis. To confirm this hypothesis, a haplotype analysis with a total of 17 microsatellite markers located throughout chromosome 18 was carried out in all family members (Figure 3).
FIGURE 3. Haplotype genotyping for 17 microsatellite markers. (A) Location of markers covering chromosome 18 and haplotype results. UPiD: uniparental isodisomy; UPhD: uniparental heterodisomy. (B) Haplotype segregation in the family. The different haplotypes are visualized by color coding. Note that the proband contains a non-informative region delimited by dotted lines, which makes it imposible to establish the cut-off point between UPiD and UPhD. UPiD: uniparental isodisomy; UPhD: uniparental heterodisomy; WT: wildtype genotype; MUT: mutant genotype.
The results showed that the proband does not share any of the haplotypes of his mother but has the same as those of his father, proving the UPD. However, UPiD of chromosome 18 is not complete, spreading from the distal p-end (short arm) to approximately 51.574.222 pb position (18q21.32), long after the centromere, while both paternal homologous chromosomes (UPhD) spread approximately from this last position to the final downstream location (distal q-end arm). In addition, no small peaks from the mother were detected in the microsatellite analysis performed in the patient, assuming no mosaicism. Therefore, we can state that both UPiD and UPhD coexist in the patient´s chromosome 18. As expected, DYM gene is located in the isodisomic region, giving a molecular explanation for the homozygosity of the mutation in the proband.
With these findings and in accordance with the American College of Medical Genetics and Genomics (ACMG) recommendations on the classification of genetic variants (Richards et al., 2015), the mutation was reclassified from variant of uncertain significance (results provided by the NGS sequencing company) to pathogenic with a very strong weighting (PVS1 code in the ACMG classification) as it is a homozygous predicted null mutation originated by a premature stop codon. This loss of function is the mechanism by which the DMC syndrome is developed, and the segregation pattern (with the isodisomy in the DYM region) is also compatible with the disease.
In recent years, NGS technology is allowing precision genetic diagnosis to become one of the fundamental pillars on which pediatricians rely when diagnosing rare diseases. Since the frequency of these diseases is low, clinicians are not used to seeing many of these diseases in their practices, so precision genetic diagnosis is of vital importance. DMC syndrome was usually diagnosed by radiological evidence but complementing the diagnosis with genetic analysis can not only reveal the molecular cause and inheritance pattern of the disease, but also provide relevant information for prognosis, treatment and clinical management of the patient.
A panel of 179 genes associated to skeletal dysplasia and mucopolysaccharidosis in a patient with a clinical diagnosis of spondylocostal skeletal dysplasia and moderate intellectual disability revealed a rare mutation, [c.62C>G; p.(Ser21Ter)], in the DYM gene. In the present study, it is determined for the first time that this mutation causes DMC syndrome in the proband and that it is caused by a paternal isodisomy-heterodisomy of chromosome 18. This variant predicts a premature stop codon in exon 2 of the 17 exons of the DYM gene. Under these circumstances, and with no alternative splicing or second translation initiations beyond exon 2 having been described, the nonsense-mediated mRNA decay (NMD) mechanism would presumably act to eliminate the transcript, resulting in a complete absence of the dymeclin protein. The action of the NMD system in this gene is supported by the fact that there is no evidence of alternative translation initiations up to the C-terminal end, according to the ATGpr predictor software (data not shown). Furthermore, Abdullah and co-workers described a nonsense mutation in the DYM gene just one codon upstream of the 21 codon. They described a reduction in the expression of the mutated transcript by RT-PCR and suggested the acting of NMD system (Abdullah et al., 2020). In addition, null mutations such as this one in the DYM gene are associated with DMC syndrome (el Ghouzzi et al., 2003). The patient´s phenotype is totally compatible with this pathology, which confirms that the p(Ser21Ter) mutation is the genetic cause of this syndrome.
The reasons why the external NGS sequencing company classified this variant as of uncertain significance are unknown. It is true that they recommended a family segregation analysis in order to classify it as pathogenic, but according to ACMG recommendations (Richards et al., 2015), it would have been sufficient to have verified that there is no second translation start after exon 2 or a possible alternative splicing that would have overridden the action of the NMD system. This fact highlights the work of translational genetics laboratories since sometimes they are able to get where these companies do not. In this way, family segregation analyses, functional analyses of variants of uncertain significance or analyses to confirm isodisomies such as this case can be performed. Thanks to this second line of genetic research, it has been possible to provide the patient with a precise diagnosis, putting an end to a diagnostic odyssey that could have lasted for many more years.
Further analysis of family segregation and microsatellite markers revealed that the homozygous condition of the mutation is due to mixed UPiD/UPhD of chromosome 18. As the centromere is within the isodisomic region, the hypothesis proposed for the occurrence of this event would be that during paternal meiosis II, one of the male gametes mistakenly received both chromatids of one of the chromosomes 18 (Eggermann et al., 2018); but one of these chromatids had previously undergone a cross-linking with the other chromosome 18. Therefore, at the moment of fertilization, the female gamete may have contributed with a third chromosome, originating a trisomy 18 that would finally be compensated by randomly eliminating an extra chromosome, which in this case would be the maternal one. This compensatory mechanism is called trisomic rescue (Liehr, 2022) and would be the major mode of UPD formation (Eggermann et al., 2018) rather than gamete complementation. This mechanism would explain the homozygosity of the mutation, as it is located in the region where isodisomy occurs.
The incidence of UPD worldwide is not clearly determined due to, among others, publishing or methodological bias (Liehr, 2022), but it has been estimated to be around 1:3,500 (Robinson, 2000) and/or 1:2,000 (Nakka et al., 2019). Not all chromosomes undergo isodisomy with the same frequency, with chromosome 18 being one of the least reported. As far as we are concerned, there are only two studies reporting an UPD in chromosome 18, one of them segmental (Kariminejad et al., 2011; Morgan et al., 2018). Furthermore, mixed UPiD-UPhD from paternal origin in any chromosome is a very rare event (Scuffins et al., 2021). This evidence, together with the fact that the general paternal UPD is much less common than maternal UPD (Nakka et al., 2019; Liehr, 2022), shows that the case presented here must be an extremely rare event. However, this fact is quite surprising when trisomies on chromosome 18 causing the well-known Edwards Syndrome, have an incidence of 1/6,000 to 1/8,000 live births. This discrepancy between the frequencies of trisomies and the very rare UPD events described for the same chromosome could be explained by considering that the cases of UPD in chromosome 18 are being underestimated. Chromosome 18 is the one with the lowest gene density (Nusbaum et al., 2005). This could be an important reason to explain why this trisomy is compatible with a full-term pregnancy and even with a few months of life. This assumption is supported by the fact that chromosome 13 is also one of the chromosomes with the lowest gene density (Dunham et al., 2004), which could also allow babies with a trisomy on this chromosome to be born, the so-called Patau Syndrome. The lower gene density in chromosome 18 also reduces the frequency of variants and the consequent occurrence of recessive diseases, which until now have been the only clue to discovering UPD cases. For this reason, it is possible that UPD cases on chromosome 18 may be underestimated, as most of them go unnoticed by the clinical eye at birth, while a trisomy is always diagnosed given its relevant symptomatology.
Consequences of UPiDs are well known such as disrupting imprinting (Robinson, 2000; Eggermann et al., 2018; Nakka et al., 2019; Benn, 2021), and they are closely intermingled with imprinting disorders (Liehr, 2014, 2022). For these reasons, we analyzed the possible imprinting consequences of the UPiD-UPhD in the proband and we discovered a described gene with paternal imprinting (Morison et al., 2005; Li et al., 2010; Edfeldt et al., 2014; Chen et al., 2015; Hsu et al., 2016; Nieser, 2016; Geneimprint, 2022), ELOA3 -previously called TCEB3C- with possible and important clinical consequences. It is located in the isodysomic region of the patient, specifically in the same cytogenetic band as the DYM gene, 18q21, and it has a monoallelic tissue-specific expression. ELOA3 was considered as a putative tumor suppressor gene (Edfeldt et al., 2014; Nieser, 2016) which is involved in small intestinal neuroendocrine tumors (SI-NETs) development (Edfeldt et al., 2014; Stålberg et al., 2016; Scarpa, 2019; Hofland et al., 2020). This gene encodes elongin A3 (Yamazaki et al., 2002), a transcription elongation factor, that forms a stable complex with elongin B and C, encoded by ELOB -previously called TCEB2- and ELOC -previously called TCEB1-, respectively. It has been described that ELOB and ELOC play an important role in tumors suppression by von Hippel–Lindau (Edfeldt et al., 2014), supporting the role of ELOA3 as a tumor suppressor gene.
Frequent loss of one copy of chromosome 18 in primary tumors and metastases has been observed in SI-NETs (Andersson et al., 2009; Cunningham et al., 2011; D. H. Kim et al., 2008; Kulke et al., 2008; Kytölä et al., 2001; Löllgen et al., 2001; Stancu et al., 2003; Tonnies et al., 2001; Walsh et al., 2011; Wang et al., 2005). Edfeldt and co-workers identified only one copy of ELOA3 in most primary SI-NETs tumors (89%) as well (Edfeldt et al., 2014). Regarding the ELOA3 gene, it would be reasonable to think that the tumors that lose one of the chromosomes 18 are those of maternal origin, since they are the only ones that are potentially expressed. This hypothesis is supported by the fact that these types of tumors are epigenetically deregulated, while genetically they remain very homogeneous (Banck et al., 2013; Karpathakis et al., 2016; Stålberg et al., 2016). In the case of our patient, having both copies of ELOA3 of paternal origin, it is possible that there is no expression of elongin A3 and this fact can particularly affect the intestinal tissue, causing, in part, a similar situation to that of SI-NETs patients who have lost part of or all of chromosome 18. Unfortunately, this hypothesis could not be confirmed as it is not possible to biopsy the patient’s intestinal tissue. We also can not state that the loss of function of ELOA3 gene is a determinant in SI-NETs development although a loss of heterozygosity exists on chromosome 18. Further studies are required to better understand the development mechanism of SI-NETs.
In any case, it seems advisable to carry out a genetic counseling for the patient to have revisions with the digestive specialist in order to prevent or mitigate this potential severe pathology. These results highlight the importance of characterizing the nature of the mutations in each individual patient to obtain an accurate diagnosis.
Currently, as in almost all rare diseases, there are no targeted molecular therapies for DMC syndrome. However, increasingly, precise drugs are being designed to act specifically depending on the mutation that the patient has. In that sense, we already have read-through agents (Pranke et al., 2018) and inhibitors of the NMD system, such as gentamicin, amlexanox and escin, used in patients with cystic fibrosis with class I mutations (Lopes-Pacheco, 2020). It would be interesting to investigate whether this type of drugs could be recycled for use in other diseases with the same molecular problem as in the case here presented. Although it may be too late for our patient, it is possible that by administering this type of drugs from birth, the development of the pathology could be mitigated or delayed.
Similarly, epigenetic therapies have already proved their effectiveness in the oncological field and other pathologies such as infectious diseases, metabolic and cardiovascular disorders (Das et al., 2009; Dunn & Rao, 2017). As other authors note for Prader-Willi or Beckwith-Wiedemann syndromes (Y. Kim et al., 2019; Papulino et al., 2020), it is possible to reduce CpG island methylation on paternal and/or maternal allele to restore the gene expression (Bartolomei & Ferguson-Smith, 2011). Further studies are needed to determinate whether these epidrugs might become a valid chance for UPD cases with aberrations in imprinting regions.
After the precision genetic diagnosis in this family, a good and accurate genetic counseling is also essential, especially when parents wish to have more children, as was the case with this family. In this case, the genetic counseling was radically different than if a classical autosomal recessive inheritance pattern had been found. The risk of having another child with DMC would be now equal to that of the general population, being well below the 25% that it would have been in the case of a recessive inheritance. In fact, the parents are currently expecting another baby and the pregnancy is proceeding normally.
In conclusion, it is worth highlighting the diagnostic odyssey that most patients with rare diseases and their families undergo, where they do not obtain a definitive diagnosis until 3–12 years after the appearance of the first symptoms. This odyssey rests in the fact that most UPDs are not detected unless there is a translational genetics laboratory supporting the national health system that decides to perform a family segregation test beforehand. In order to minimize these risks, it is desirable that NGS companies choose to perform trio exomes. This approach would make it possible to detect homozygous mutations that are due to UPD, making the most of these technologies and, ultimately, improving diagnosis so that it is faster and more precise.
The datasets for this article are not publicly available due to concerns regarding participant/patient anonymity. Requests to access the datasets should be directed to the corresponding author.
Ethical review and approval was not required for the study on human participants in accordance with the local legislation and institutional requirements. Written informed consent to participate in this study was provided by the participants’ legal guardian/next of kin.
M-PL-G carried out the analysis, interpretation of the data, and manuscript writing. M-CC-R recruited the family, participated in the data acquisition, did the clinical examination to the individuals and contributed to the conception and design of the study. MM-H and C-MS-M contributed to the data analysis and elaboration of figures and tables. FS-S elaborated the conception and design of the study, and interpretation of the results. All the authors contributed to manuscript revisions and read and approved the final manuscript.
Authors are extremely grateful to the family participant in the study. We would like to thank the nurses of the Pediatrics Service of University Hospital of Albacete for blood extractions. Authors also acknowledge funding by the Health Service of Castilla-La Mancha, which has a collaboration agreement with the Medical Genetics Group (University of Castilla-La Mancha) to financial support of genetic studies of the patients. MM-H has been rewarded with a collaboration grant awarded by the non-profit association ADAO.
The authors declare that the research was conducted in the absence of any commercial or financial relationships that could be construed as a potential conflict of interest.
All claims expressed in this article are solely those of the authors and do not necessarily represent those of their affiliated organizations, or those of the publisher, the editors and the reviewers. Any product that may be evaluated in this article, or claim that may be made by its manufacturer, is not guaranteed or endorsed by the publisher.
The Supplementary Material for this article can be found online at: https://www.frontiersin.org/articles/10.3389/fgene.2022.1005573/full#supplementary-material
Abdullah, , , Shah, P. W., Nawaz, S., Hussain, S., Ullah, A., Basit, S., et al. (2020). A homozygous nonsense variant in DYM underlies Dyggve–Melchior–Clausen syndrome associated with ectodermal features. Mol. Biol. Rep. 47 (9), 7083–7088. doi:10.1007/s11033-020-05774-z
Andersson, E., Swärd, C., Stenman, G., Ahlman, H., and Nilsson, O. (2009). High-resolution genomic profiling reveals gain of chromosome 14 as a predictor of poor outcome in ileal carcinoids. Endocr. Relat. Cancer 16 (3), 953–966. doi:10.1677/ERC-09-0052
Banck, M. S., Kanwar, R., Kulkarni, A. A., Boora, G. K., Metge, F., Kipp, B. R., et al. (2013). The genomic landscape of small intestine neuroendocrine tumors. J. Clin. Invest. 123 (6), 2502–2508. doi:10.1172/JCI67963
Bartolomei, M. S., and Ferguson-Smith, A. C. (2011). Mammalian genomic imprinting. Cold Spring Harb. Perspect. Biol. 3 (7), a002592. doi:10.1101/cshperspect.a002592
Beighton, P. (1990). Dyggve-Melchior-Clausen syndrome. J. Med. Genet. 27 (8), 512–515. doi:10.1136/jmg.27.8.512
Benn, P. (2021). “Uniparental disomy: Origin, frequency, and clinical significance,” in Prenatal diagnosis (New York, United States: John Wiley & Sons), 41 (5), 564–572. doi:10.1002/pd.5837
Chen, Z., Hagen, D. E., Elsik, C. G., Ji, T., Morris, C. J., Moon, L. E., et al. (2015). Characterization of global loss of imprinting in fetal overgrowth syndrome induced by assisted reproduction. Proc. Natl. Acad. Sci. U. S. A. 112 (15), 4618–4623. doi:10.1073/pnas.1422088112
Cohn, D. H., Ehtesham, N., Krakow, D., Unger, S., Shanske, A., Reinker, K., et al. (2003). Mental retardation and abnormal skeletal development (Dyggve-Melchior-Clausen dysplasia) due to mutations in a novel, evolutionarily conserved gene. Am. J. Hum. Genet. 72, 419–428. doi:10.1086/346176
Cunningham, J. L., Díaz de Ståhl, T., Sjöblom, T., Westin, G., Dumanski, J. P., and Janson, E. T. (2011). Common pathogenetic mechanism involving human chromosome 18 in familial and sporadic ileal carcinoid tumors. Genes Chromosom. Cancer 50 (2), 82–94. doi:10.1002/gcc.20834
Das, R., Hampton, D. D., and Jirtle, R. L. (2009). Imprinting evolution and human health. Mamm. Genome 20 (9–10), 563–572. doi:10.1007/s00335-009-9229-y
Dimitrov, A., Paupe, V., Gueudry, C., Sibarita, J.-B., Raposo, G., Vielemeyer, O., et al. (2009). The gene responsible for Dyggve-Melchior-Clausen syndrome encodes a novel peripheral membrane protein dynamically associated with the Golgi apparatus. Hum. Mol. Genet. 18 (3), 440–453. doi:10.1093/hmg/ddn371
Dunham, A., Matthews, L. H., Burton, J., Ashurst, J. L., Howe, K. L., Ashcroft, K. J., et al. (2004). The DNA sequence and analysis of human chromosome 13. Nature 428 (6982), 522–528. doi:10.1038/nature02379
Dunn, J., and Rao, S. (2017). Epigenetics and immunotherapy: The current state of play. Mol. Immunol. 87, 227–239. doi:10.1016/j.molimm.2017.04.012
Dupuis, N., Fafouri, A., Bayot, A., Kumar, M., Lecharpentier, T., Ball, G., et al. (2015). Dymeclin deficiency causes postnatal microcephaly, hypomyelination and reticulum-to-Golgi trafficking defects in mice and humans. Hum. Mol. Genet. 24 (10), 2771–2783. doi:10.1093/hmg/ddv038
Edfeldt, K., Ahmad, T., Åkerström, G., Janson, E. T., Hellman, P., Stålberg, P., et al. (2014). TCEB3C a putative tumor suppressor gene of small intestinal neuroendocrine tumors. Endocr. Relat. Cancer 21 (2), 275–284. doi:10.1530/ERC-13-0419
Eggermann, T., Mackay, J. G. D., and Tümer, Z. (2018). Uniparental disomy and imprinting disorders. obm Genet. 2 (3), 1. doi:10.21926/obm.genet.1803031
Geneimprint (2022). Human imprintome genomic map. Available at: https://www.geneimprint.com/(Accessed July 19, 2022).
Ghouzzi, V. E., Dagoneau, N., Kinning, E., Thauvin-Robinet, C., Chemaitilly, W., Prost-Squarcioni, C., et al. (2003). Mutations in a novel gene Dymeclin (FLJ20071) are responsible for Dyggve-Melchior-Clausen syndrome. Hum. Mol. Genet. 12 (3), 357–364. doi:10.1093/hmg/ddg029
Hofland, J., Kaltsas, G., and de Herder, W. W. (2020). Advances in the diagnosis and management of well-differentiated neuroendocrine neoplasms. Endocr. Rev. 41 (2), bnz004. doi:10.1210/endrev/bnz004
Hsu, C.-M., Lin, P.-M., Lin, H.-C., Lai, C.-C., Yang, C.-H., Lin, S.-F., et al. (2016). Altered expression of imprinted genes in squamous cell carcinoma of the head and neck. Anticancer Res. 36 (5), 2251–2258.
Kariminejad, A., Kariminejad, R., Moshtagh, A., Zanganeh, M., Kariminejad, M. H., Neuenschwander, S., et al. (2011). Pericentric inversion of chromosome 18 in parents leading to a phenotypically normal child with segmental uniparental disomy 18. Eur. J. Hum. Genet. 19 (5), 555–560. doi:10.1038/ejhg.2010.252
Karpathakis, A., Dibra, H., Pipinikas, C., Feber, A., Morris, T., Francis, J., et al. (2016). Prognostic impact of novel molecular subtypes of small intestinal neuroendocrine tumor. Clin. Cancer Res. 22 (1), 250–258. doi:10.1158/1078-0432.CCR-15-0373
Kim, D. H., Nagano, Y., Choi, I.-S., White, J. A., Yao, J. C., and Rashid, A. (2008). Allelic alterations in well-differentiated neuroendocrine tumors (carcinoid tumors) identified by genome-wide single nucleotide polymorphism analysis and comparison with pancreatic endocrine tumors. Genes Chromosom. Cancer 47 (1), 84–92. doi:10.1002/gcc.20510
Kim, Y., Wang, S. E., and Jiang, Y. (2019). Epigenetic therapy of prader–willi syndrome. Transl. Res. 208, 105–118. doi:10.1016/j.trsl.2019.02.012
Kulke, M. H., Freed, E., Chiang, D. Y., Philips, J., Zahrieh, D., Glickman, J. N., et al. (2008). High-resolution analysis of genetic alterations in small bowel carcinoid tumors reveals areas of recurrent amplification and loss. Genes Chromosom. Cancer 47 (7), 591–603. doi:10.1002/gcc.20561
Kytölä, S., Höög, A., Nord, B., Cedermark, B., Frisk, T., Larsson, C., et al. (2001). Comparative genomic hybridization identifies loss of 18q22-qter as an early and specific event in tumorigenesis of midgut carcinoids. Am. J. Pathol. 158 (5), 1803–1808. doi:10.1016/S0002-9440(10)64136-3
Li, S. S., Yu, S-L., and Singh, S. (2010). Epigenetic states and expression of imprinted genes in human embryonic stem cells. World J. Stem Cells 2 (4), 97–102. doi:10.4252/wjsc.v2.i4.97
Liehr, T., Heng, H., Yurov, Y., Meloni-Ehrig, A., and Iourov, I. (2022). Molecular cytogenetics: The first impact factor (2.36). Mol. Cytogenet. 15 (1), 28. doi:10.1186/1755-8166-6-28
Liehr, T. (2014). “UPD related syndromes caused by imprinting,” in Uniparental disomy (UPD) in clinical genetics (Berlin, Germany: Springer Berlin Heidelberg), 49–77. doi:10.1007/978-3-642-55288-5_4
Löllgen, R.-M., Hessman, O., Szabo, E., Westin, G., and Akerström, G. (2001). Chromosome 18 deletions are common events in classical midgut carcinoid tumors. Int. J. Cancer 92 (6), 812–815. doi:10.1002/ijc.1276
Lopes-Pacheco, M. (2020). CFTR modulators: The changing face of cystic fibrosis in the era of precision medicine. Front. Pharmacol. 10, 1662. doi:10.3389/fphar.2019.01662
Morgan, A., Lenarduzzi, S., Cappellani, S., Pecile, V., Morgutti, M., Orzan, E., et al. (2018). Genomic studies in a large cohort of hearing impaired Italian patients revealed several new alleles, a rare case of uniparental disomy (UPD) and the importance to search for copy number variations. Front. Genet. 9, 681. doi:10.3389/fgene.2018.00681
Morison, I. M., Ramsay, J. P., and Spencer, H. G. (2005). A census of mammalian imprinting. Trends Genet. 21 (8), 457–465. doi:10.1016/j.tig.2005.06.008
Nakka, P., Pattillo Smith, S., O’Donnell-Luria, A. H., McManus, K. F., Agee, M., Auton, A., et al. (2019). Characterization of prevalence and health consequences of uniparental disomy in four million individuals from the general population. Am. J. Hum. Genet. 105 (5), 921–932. doi:10.1016/j.ajhg.2019.09.016
Nieser, M. (2016). Identification and characterization of the genetic alterations underlying neuroendocrine tumors of the small intestine. Tübingen, Germany: Tübingen University.
Nusbaum, C., Zody, M. C., Borowsky, M. L., Kamal, M., Kodira, C. D., Taylor, T. D., et al. (2005). DNA sequence and analysis of human chromosome 18. Nature 437 (7058), 551–555. doi:10.1038/nature03983
Orphanet (2022). Orphanet. Available at: Https://Www.Orpha.Net/Consor/Cgi-Bin/OC_Exp.Php?Lng=EN&Expert=239.
Papulino, C., Chianese, U., Nicoletti, M. M., Benedetti, R., and Altucci, L. (2020). Preclinical and clinical epigenetic-based reconsideration of beckwith-wiedemann syndrome. Front. Genet. 11, 563718. doi:10.3389/fgene.2020.563718
Pranke, I., Bidou, L., Martin, N., Blanchet, S., Hatton, A., Karri, S., et al. (2018). Factors influencing readthrough therapy for frequent cystic fibrosis premature termination codons. ERJ Open Res. 4 (1), 00080-0.2017doi:10.1183/23120541.00080-2017
Richards, S., Aziz, N., Bale, S., Bick, D., Das, S., Gastier-Foster, J., et al. (2015). Standards and guidelines for the interpretation of sequence variants: A joint consensus recommendation of the American College of medical genetics and genomics and the association for molecular pathology. Genet. Med. 17 (5), 405–424. doi:10.1038/gim.2015.30
Robinson, W. P. (2000). Mechanisms leading to uniparental disomy and their clinical consequences. BioEssays 22 (5), 452–459. doi:10.1002/(SICI)1521-1878(200005)22:5<452:AID-BIES7>3.0.CO;2-K
Scarpa, A. (2019). The landscape of molecular alterations in pancreatic and small intestinal neuroendocrine tumours. Ann. Endocrinol. 80 (3), 153–158. doi:10.1016/j.ando.2019.04.010
Scuffins, J., Keller-Ramey, J., Dyer, L., Douglas, G., Torene, R., Gainullin, V., et al. (2021). Uniparental disomy in a population of 32, 067 clinical exome trios. Genet. Med. 23 (6), 1101–1107. doi:10.1038/s41436-020-01092-8
Stålberg, P., Westin, G., and Thirlwell, C. (2016). Genetics and epigenetics in small intestinal neuroendocrine tumours. J. Intern. Med. 280 (6), 584–594. doi:10.1111/joim.12526
Stancu, M., Wu, T. T., Wallace, C., Houlihan, P. S., Hamilton, S. R., and Rashid, A. (2003). Genetic alterations in goblet cell carcinoids of the vermiform appendix and comparison with gastrointestinal carcinoid tumors. Mod. Pathol. 16 (12), 1189–1198. doi:10.1097/01.MP.0000097362.10330.B1
Tonnies, H., Toliat, M., Ramel, C., Pape, U., Neitzel, H., Berger, W., et al. (2001). Analysis of sporadic neuroendocrine tumours of the enteropancreatic system by comparative genomic hybridisation. Gut 48 (4), 536–541. doi:10.1136/gut.48.4.536
Walsh, K. M., Choi, M., Oberg, K., Kulke, M. H., Yao, J. C., Wu, C., et al. (2011). A pilot genome-wide association study shows genomic variants enriched in the non-tumor cells of patients with well-differentiated neuroendocrine tumors of the ileum. Endocr. Relat. Cancer 18 (1), 171–180. doi:10.1677/ERC-10-0248
Wang, G. G., Yao, J. C., Worah, S., White, J. A., Luna, R., Wu, T.-T., et al. (2005). Comparison of genetic alterations in neuroendocrine tumors: Frequent loss of chromosome 18 in ileal carcinoid tumors. Mod. Pathol. 18 (8), 1079–1087. doi:10.1038/modpathol.3800389
Keywords: UPD chromosome 18, DMC syndrome, precision medicine, imprinting chromosome 18, diagnostic odyssey, ELOA3, DYM gene mutation, TCEB3C
Citation: López-Garrido M-P, Carrascosa-Romero M-C, Montero-Hernández M, Serrano-Martínez C-M and Sánchez-Sánchez F (2022) Case Report: Precision genetic diagnosis in a case of Dyggve-Melchior-Clausen syndrome reveals paternal isodisomy and heterodisomy of chromosome 18 with imprinting clinical implications. Front. Genet. 13:1005573. doi: 10.3389/fgene.2022.1005573
Received: 28 July 2022; Accepted: 02 November 2022;
Published: 18 November 2022.
Edited by:
Erika Fernandez-Vizarra, University of Padua, ItalyReviewed by:
Masayo Kagami, National Center for Child Health and Development (NCCHD), JapanCopyright © 2022 López-Garrido, Carrascosa-Romero, Montero-Hernández, Serrano-Martínez and Sánchez-Sánchez. This is an open-access article distributed under the terms of the Creative Commons Attribution License (CC BY). The use, distribution or reproduction in other forums is permitted, provided the original author(s) and the copyright owner(s) are credited and that the original publication in this journal is cited, in accordance with accepted academic practice. No use, distribution or reproduction is permitted which does not comply with these terms.
*Correspondence: Francisco Sánchez-Sánchez, ZnJhbmNpc2NvLnNzYW5jaGV6QHVjbG0uZXM=
Disclaimer: All claims expressed in this article are solely those of the authors and do not necessarily represent those of their affiliated organizations, or those of the publisher, the editors and the reviewers. Any product that may be evaluated in this article or claim that may be made by its manufacturer is not guaranteed or endorsed by the publisher.
Research integrity at Frontiers
Learn more about the work of our research integrity team to safeguard the quality of each article we publish.