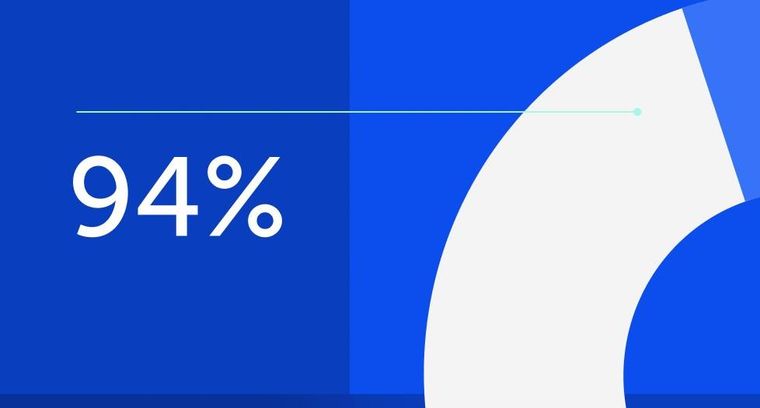
94% of researchers rate our articles as excellent or good
Learn more about the work of our research integrity team to safeguard the quality of each article we publish.
Find out more
ORIGINAL RESEARCH article
Front. Genet., 03 January 2022
Sec. Genomic Assay Technology
Volume 12 - 2021 | https://doi.org/10.3389/fgene.2021.769281
This article is part of the Research TopicMicrosatellite and Microsatellite InstabilityView all 5 articles
Determination of microsatellite instability (MSI) using molecular test and deficient mismatch repair (dMMR) using immunohistochemistry (IHC) has major implications on colorectal cancer (CRC) management. The HSP110 T17 microsatellite has been reported to be more monomorphic than the common markers used for MSI determination. Large deletion of HSP110 T17 has been associated with efficacy of adjuvant chemotherapy in dMMR/MSI CRCs. The aim of this study was to evaluate the interest of HSP110 deletion/expression as a diagnostic tool of dMMR/MSI CRCs and a predictive tool of adjuvant chemotherapy efficacy. All patients with MSI CRC classified by molecular testing were included in this multicenter prospective cohort (n = 381). IHC of the 4 MMR proteins was carried out. HSP110 expression was carried out by IHC (n = 343), and the size of HSP110 T17 deletion was determined by PCR (n = 327). In the 293 MSI CRCs with both tests, a strong correlation was found between the expression of HSP110 protein and the size of HSP110 T17 deletion. Only 5.8% of MSI CRCs had no HSP110 T17 deletion (n = 19/327). HSP110 T17 deletion helped to re-classify 4 of the 9 pMMR/MSI discordance cases as pMMR/MSS cases. We did not observe any correlation between HSP110 expression or HSP110 T17 deletion size with time to recurrence in patients with stage II and III CRC, treated with or without adjuvant chemotherapy. HSP110 is neither a robust prognosis marker nor a predictor tool of adjuvant chemotherapy efficacy in dMMR/MSI CRC. However, HSP110 T17 is an interesting marker, which may be combined with the other pentaplex markers to identify discordant cases between MMR IHC and MSI.
Approximately 15% of colorectal cancers (CRCs) have microsatellite instability (MSI) due to the deficient mismatch repair (dMMR) system (Umar et al., 2004; Evrard et al., 2019). Determination of MMR/MSI status is a key issue in the management of CRC patients. dMMR/MSI status is associated with Lynch syndrome but is also a prognostic and predictive factor of sensitivity to chemotherapy and immune checkpoint inhibitors. Indeed, dMMR/MSI status is associated with good prognosis in non-metastatic CRC, particularly for patients with stage II CRC, which in most cases does not require adjuvant chemotherapy (Sargent et al., 2010). Moreover, dMMR/MSI status has been associated with chemoresistance to adjuvant 5-fluorouracil (5-FU) in stage II CRC (Tougeron et al., 2016). Finally, the high rate of mutational load in dMMR/MSI tumors leads to multiple tumor-specific neo-antigens recruiting cytotoxic T-cells, which explain both the good prognosis and the high sensitivity to immune checkpoint inhibitors (Tougeron et al., 2009; Le et al., 2015; André et al., 2020).
HSP110 protein belongs to the family of large heat shock proteins (HSPs) (Zuo et al., 2016). In CRC, HSP110 promotes the proliferation of tumor cells by activating signal transducer and activator of transcription 3, STAT3 (Berthenet et al., 2017). In 2011, Dorard et al. identified HSP110 as a target of microsatellite instability (Dorard et al., 2011). In the intron 8 of HSP110, there exists a region of mononucleotide repeats of thymine, which is located upstream of the splice acceptor-site of exon 9. This region is well conserved across species with only two known alleles, 16 repeats (T16) and 17 repeats (T17), the latter being more frequent. The HSP110 T17 marker has been reported to be more monomorphic than the common mononucleotide markers used for the determination of MSI status (Buhard et al., 2016; Berardinelli et al., 2018; How-Kit et al., 2018). Indeed, about 97% of dMMR/MSI CRCs presented 1 to 7 base pairs (bp) deletion within the T17 region. These deletions, when they were bi-allelic and of large size (≥5 bp), led to complete inactivation of the HSP110 protein (Dorard et al., 2011). Large deletion of HSP110 T17 has been associated with efficacy of adjuvant chemotherapy, 5-FU alone or with oxaliplatin, in non-metastatic stage II and III dMMR/MSI CRCs by one team (Dorard et al., 2011; Collura et al., 2014). By contrast, neither significant survival difference nor impact on adjuvant chemotherapy efficacy between large and small HSP110 T17 deletions was observed in another study (Kim et al., 2014).
Kim JH et al. analyzed HSP110 expression by immunohistochemistry (IHC) in dMMR/MSI CRCs, and low expression of HSP110 was significantly associated with deletion of 4 bp or more of HSP110 thymine repeats (Kim et al., 2014). High expression of HSP110 was observed in normal tissue, pMMR/MSS CRCs and dMMR/MSI CRCs with short HSP110 T17 deletion. Low HSP110 expression was associated with better disease-free survival (DFS) but not the size of HSP110 T17 deletion. These results and other studies suggested discordant results concerning HSP110 expression by IHC and/or size of HSP110 T17 deletion as a prognostic factor and/or predictor of adjuvant chemotherapy efficacy in stage II and III dMMR/MSI CRCs (Dorard et al., 2011; Collura et al., 2014; Kim et al., 2014; Oh et al., 2017). dMMR/MSI CRCs have been associated with resistance to adjuvant 5FU, mostly in stage II CRC, as compared to pMMR/MSS tumors. Nevertheless, these studies have suggested that among dMMR/MSI, HSP110 T17 deletion size could be associated with sensitivity to adjuvant 5FU, a finding suggesting that non-metastatic dMMR/MSI CRC could have different levels of chemoresistance to 5FU due to HSP110 T17 deletion.
The objective of this study was to evaluate the correlation between HSP110 T17 deletion and dMMR/MSI status identified by standard procedures. We also evaluated both HSP110 T17 deletion and HSP110 expression as prognostic factors or predictors of response to adjuvant chemotherapy in dMMR/MSI CRCs.
From 2003 to 2015, all patients with a CRC classified as “MSI” by molecular testing (Pentaplex panel) from the cancer biology departments of the Poitiers and Tours University Hospitals were included in this multicenter prospective cohort (n = 381). Proficient MMR (pMMR) or dMMR statuses were determined secondarily (MLH1, PMS2, MSH2, and MSH6 protein expression). Most MMR IHC and MSI molecular tests were carried out in routine clinical practice. All cases, including discordant cases (pMMR/MSI), were evaluated in light of HSP110 T17 deletion results.
The study has been approved by the ethics committees of the Poitiers and Tours University Hospitals (Comité de protection des personnes Ouest III, n DC-2008-565 and n 2018-039). The study was performed according to the principles of the Declaration of Helsinki.
Main patients (gender and age) and tumor (tumor site, TNM stage, VELIPI criteria (vascular emboli, lymphatic invasion, or perineural invasion), tumor grade, tumor perforation, and initial bowel obstruction) characteristics were collected. Germline Lynch syndrome versus sporadic dMMR/MSI cases was determined, as previously described (Tougeron et al., 2020). Briefly, the molecular mechanism underlying the MMR deficiency was based on the detection of MMR gene germline mutation, Amsterdam II criteria, MMR protein expression, BRAF status, and MLH1 promoter methylation.
Tumor DNA was extracted in routine practice from formalin-fixed paraffin-embedded tumor tissue using a KAPA Express Extract© kit (ROCHE, Basel, Switzerland). The same tumor DNA was also used to determine KRAS, NRAS, and BRAF mutations using next-generation sequencing.
MSI phenotype was assessed by analyzing microsatellite loci consisting of 5 mononucleotide markers, BAT-25, BAT-26, NR21, NR24, and NR27 (Kit PROMEGA, ref MD1641, Madison, Wisconsin, United States), as previously described (Pentaplex panel) (Buhard et al., 2006; Tachon et al., 2018). Briefly, PCR fragments were separated according to their size by capillary electrophoresis using an ABI 3500Dx Genetic Analyzer© (Applied Biosystems, Foster City, United States). The results were analyzed using GeneMapper v4.1© software (Applied Biosystems) and interpreted by expert molecular biologists. MSI was defined by the presence of instability affecting at least 3 of the 5 markers. In the case of one or two markers with instability, a comparative analysis of normal colon tissue and tumor DNA was performed, as polymorphisms have been reported, especially in African ethnicities (Augustus and Ellis, 2018).
For all cases, both tissue microarray and whole tissue sections were available. For tissue microarray construction, nine biopsy cores of 0.6 mm diameter per patient (3 in tumor center, 3 in invasive front, and 3 in non-tumor tissue) have been performed using Alphelys© plateform (MTA Booster© version 1.01, Plaisir, France).
For determination of MMR status, most IHCs were carried out in routine clinical practice from formalin-fixed paraffin-embedded tumor tissue using antibodies directed against MLH1 (clone M1), MSH2 (clone G219-1129), MSH6 (clone 44), and PMS2 (clone EPR3947) proteins (Ventana Medical Systems, Tucson, AZ, United States), as previously described (Tachon et al., 2018; Singh et al., 2021). If IHC was not performed or was discordant with the MSI test (pMMR/MSI), a new MMR IHC was performed on tissue microarray. A tumor was considered dMMR if it presented loss of nuclear staining of at least one of the four MMR proteins.
HSP110 IHC was carried out on whole-section tissues with the antibody directed against the C-terminus part of HSP110 protein (Leica Biosystems, NCL-HSP105, RRID:AB_563775, Newcastle upon Tyne, United Kingdom), as described by Kim et al. (2014). This HSP110 antibody is designed to target only wild-type (wt) HSP110 protein and therefore does not recognize the truncated HSP110 protein caused by HSP110 intronic T17 deletions. The staining was mostly cytoplasmic and sometimes associated with nuclear staining, as previously described (Kim et al., 2014). The same intensity grading as that of Oh et al. was used (Oh et al., 2017), with four-tier intensity scores ranging from 0 (no expression) to 3 (stronger nuclear-to-cytoplasmic expression than the internal positive control) (Supplementary Figure S1). Internal non-tumor tissue, precisely normal colonic mucosal epithelial cells and/or lymphocytes, served as the positive control. The intensity score was considered when at least 5% of the tumor surface was stained. For each sample, the most represented score occupying the largest area of the tumor, called the “dominant intensity score” by Oh et al., was determined (Oh et al., 2017) (Supplementary Figure S2). Nuclear positivity was defined as nuclear staining of at least 10% of the tumor cells.
Two independent expert pathologists, unaware of other results and HSP110 T17 deletion, conducted the scoring in the 343 formalin-fixed paraffin-embedded samples available for HSP110 expression IHC. Conflicting results between the two observers were reviewed and discussed, and a consensus was reached. The tumor was classified as HSP110 “high” when the dominant intensity score was 2 + or 3+ and HSP110 “low” when the dominant intensity score was 0 or 1+.
The size of HSP110 T17 microsatellite deletion was determined by PCR followed by capillary electrophoresis on the 327 tumor DNA available. To amplify the region of interest, homemade primers (forward, 5′-TAMRACCCTGTCCATCCATTGGAATTGA-3′; reverse, 5′-GGAACTGCATCTGTGACGGAA-3′) and standard PCR procedure (initial denaturation step at 94°C for 10 min, then 40 cycles at 94°C for 30 s, 57°C for 30 s, and 72°C for 1 min) were used. Results were interpreted by two independent molecular biologists using GeneMapper v4.1© software (Applied Biosystems, Foster City, United States). To determine the polymorphic zone corresponding to the T16/T16, T16/T17, and T17/T17 genotypes (Dorard et al., 2011), 36 MSS CRCs were analyzed. The polymorphic zone corresponded to 147–148 base pairs (bp) fragment sizes, 147 bp for the T16 allele and 148 bp for the T17 allele (Figure 1A). Information about homozygous T16 or T17 or heterozygous T16/T17 was not collected in this work, in which only the polymorphic area was established.
FIGURE 1. Molecular determination of HSP110 T17 deletion. (A) Homozygous T16/T16 and HSP110 T17/T17 and heterozygous T16/T17 HSP110 profiles from MSS CRC tissues. Peak of 147bp is indicated in black. (B) MSI tumor with a large deletion (−5bp) of HSP110 T17. (C) MSI tumor with a small deletion (−2bp) of HSP110 T17. The gray frame is the polymorphic area. Arrows indicate the size of the deleted transcript. (D) Distribution of samples according to the size of HSP110 T17 deletion.
Deletions on the HSP110 T17 microsatellite resulted in peaks smaller than the polymorphic zone of 147 bp (Figures 1A–C). For the majority of the analyzed MSI tumor samples, only one mutated allele type was detected, corresponding to the main clonal population present in the tumors, as described by Collura et al. (2014). For cases that displayed multiallelic profiles, the peak associated with the larger T17 deletion, which did not appear to result from the Taq polymerase stuttering, was considered. Two different thresholds for the scoring of HSP110 T17 deletion were used according to previous publications, 5 bp (Collura et al., 2014) and 4 bp (Kim et al., 2014). For all analyses, 5 bp was used first as the primary endpoint.
Two independent expert molecular biologists, unaware of each other’s results and IHC HSP110 results, conducted the molecular interpretation. Conflicting results between the two observers were reviewed and discussed, and a consensus was reached.
Follow-up was calculated by reverse Kaplan–Meier estimation. Some patients with sporadic MSI CRC were elderly with comorbidities and could die from causes other than CRC; consequently, time to recurrence (TTR) and disease-free survival (DFS), cancer-specific survival (CSS), and overall survival (OS) were determined. For non-metastatic patients with curative surgery, TTR was defined as the time between surgery and the date of recurrence or death from the same cancer, whichever occurred first (Birgisson et al., 2011). DFS was defined as the time between curative surgery and the date of recurrence or death, whatever the cause and whichever occurred first. OS was defined as the time between CRC diagnosis and the date of death, whatever the cause. Alive patients were censored at the date of last assessment. CSS was defined as the time between CRC diagnosis and the date of death from the same cancer. Alive patients without recurrence were censored at the date of last assessment. Survival curves were estimated using the Kaplan–Meier method and compared using the log-rank test.
Variables with p values of 0.10 or less in univariate analysis were eligible for the Cox multivariable regression model. A p value of less than 0.05 was considered statistically significant. All analyses were performed using Statview© software (SAS Institute, Cary, NC).
A total of 381 patients with MSI CRC were included in this study. The mean age was 69.9 years with 60.1% being women (Table 1). The tumor was localized in the ascending colon in 80.6% of cases and the majority of tumors were stage II or stage III (43.9 and 32.2%, respectively). Tumors were mainly pT3 (60.5%) and moderately or poorly differentiated (43.9 and 44.2%, respectively).
RAS and BRAF mutations were observed in 25.0 and 54.0% of tumors, respectively. Most CRCs had MLH1/PMS2 loss (79.9%) and only 9 (2.9%) were pMMR. MLH1 promoter hypermethylation was determined in 112 tumors in order to identify sporadic MSI CRCs and was detected in 60.7% (n = 68/112). Only 8.9% of patients had a proven germline MMR mutation, and 16.6% had suspected Lynch syndrome.
Most patients had a resection of their primary tumors (99.0%). Among patients with non-metastatic tumors (n = 317), six patients had non-curative resection of their tumor and were excluded for survival analyses. Among the stage II and III patients with curative strategy (n = 280), 37.0% had received adjuvant chemotherapy, 16.0 and 67.0% in stage II and III, respectively (Table 2). Most adjuvant treatment was 5-FU associated with oxaliplatin (80.6%, n = 83/103) and others, fluoropyrimidine alone.
Median follow-up of the overall population was 79.2 ± 3.5 months. Overall, 48.3% of patients died, of which 59.2% were cancer-related. Among stage II and III CRC patients with curative surgery, recurrence rates were 13.2 and 23.7%, respectively. Median TTR was not reached. The 3-year TTR rates of stage II and III CRC patients were 88.8 and 76.2%, respectively. Median CSS was not reached. The 5-year CSS rates of stage II and III were 88.8 and 75.0%, respectively.
Concerning patients with metastatic tumors (n = 59), median OS and CSS were 12.5 ± 3.8 months and 14.3 ± 3.7 months, respectively.
Among the 381 patients, 343 formalin-fixed paraffin-embedded samples were available for IHC testing. The most represented intensity score in each tumor, called the “dominant intensity score,” was 3+ (54.2%), followed by 2+ (24.5%) (Supplementary Table S1). A positive HSP110 nuclear staining was found in 36.4%, mainly in tumors with a 3 + dominant intensity score (n = 95) (p < 0.001). In addition, a majority of tumors (74.0%) had intratumoral heterogeneity of HSP110 staining, defined by at least two different identified intensity scores. The majority of tumors presented high HSP110 expression (78.7%) defined by a “dominant intensity score” of 2 + or 3+.
Deletion of HSP110 T17 was determined for 327 MSI CRC. Most samples (n = 308, 94.2%) showed deletion of 1–7 bp of the HSP110 T17 microsatellite (Figure 1D). Few tumors had a large HSP110 T17 deletion, 27.8% using a cut-off at 5 bp, 55.6% using a cut-off at 4 bp.
Nineteen cases had no HSP110 T17 deletion (5.8%). When looking at these tumors, they were more frequently located in the descending colon (44.4 versus 13.8%, p = 0.002), BRAF wt (84.2 versus 43.8%, p < 0.001), with no MLH1 promoter hypermethylation (78.6 versus 32.9%, p < 0.001), and all but one had high HSP110 expression. Most of these tumors were MSI/dMMR/no HSP110 T17 deletion (n = 15), 14 with MLH1/PMS2 loss and 1 with MSH6 loss, and 4 were MSI/pMMR/no HSP110 T17 deletion. After re-examination and new MSI/MMR IHC testing on new tumor material with special care given to tissue quality and percentage of tumor cells in these 19 cases, the 4 MSI/pMMR/no HSP110 T17 deletion cases were finally considered as MSS with no unstable markers (n = 0/5) (Table 3). Regarding the remaining 5 MSI/pMMR/no HSP110 T17 deletion cases, discordance remained despite re-examination and new MSI/MMR IHC tests. None of these patients had MMR germline testing.
Finally, out of 327 cases and after re-examination, 20 were discordant with either MSI/pMMR/HSP110 T17 deletion (n = 5) or MSI/dMMR/no HSP110 T17 wt (n = 15) (Table 3). All in all, HSP110 T17 deletion has a sensitivity of 95.5% to detect dMMR/MSI cases and proves useful in detecting false MSI results.
Among the 381 MSI CRCs, 293 were analyzed for HSP110 both by IHC and molecular tests. When comparing HSP110 expression (low versus high) and HSP110 T17 deletion (large versus small) using 5 bp cut-off, a strong correlation was identified (p < 0.001), with 76.4% concordance (n = 224/293) (Figure 2). A close result with 59.7% concordance (n = 175/293) was observed using a cut-off of 4 bp (p < 0.001). There was no correlation between the presence of nuclear staining and the deletion size of HSP110 T17 using a cut-off at 5 bp (p = 0.18) contrary to 4 bp (p = 0.01).
No correlation was found between HSP110 expression (high versus low) and HSP110 T17 deletion (cut-off at 5 or 4 bp) with main patient characteristics (age, sex, and LS), histopathological features (pTNM stage, tumor site, perforation, initial tumor obstruction, T stage, grade, N stage, mucinous component, and VELIPI criteria), MMR immunohistochemistry, and BRAF mutation. By contrast, RAS mutations were associated with high HSP110 expression (28.2 versus 12.5%, p = 0.006) and small HSP110 T17 deletion using a cut-off at 5 bp (28.0 versus 16.7%, p = 0.044). When using a cut-off at 4 bp for HSP110 T17 deletion, a strong correlation with RAS mutations was also identified (p = 0.003).
In patients with stage II and III MSI CRC, recurrence rates did not differ according to HSP110 T17 deletion size, 19.7% in the large deletion group versus 17.7% in the small deletion group using 5 bp cut-off (p = 0.72), or HSP110 expression (p = 0.79). TTR and DFS did not differ according to HSP110 T17 deletion size (p = 0.45 and p = 0.91, respectively) or HSP110 expression (p = 0.78 and p = 0.64, respectively) (Figures 3A,B). The 3-year TTR rates in the large deletion group versus the small deletion group were 76.7 and 84.1%, respectively. Results were similar when using a cut-off at 4 bp for HSP110 T17 deletion or according to HSP110 nuclear staining.
FIGURE 3. Time to recurrence. Kaplan–Meier curves showing the time to recurrence in patients with stage II or III MSI CRC according to (A) size of HSP110 T17 deletion and (B) HSP110 expression and time to recurrence in patients with stage II or III MSI CRC who received adjuvant chemotherapy according to (C) size of HSP110 T17 deletion and (D) HSP110 expression.
OS and CSS did not differ according to size of HSP110 T17 deletion using a cut-off at 5 bp (p = 0.83 and p = 0.29, respectively) or HSP110 expression (p = 0.84 and p = 0.82, respectively). The 5-year CSS rates in case of large deletion versus small deletion were 79.3 and 83.4%, respectively.
In patients with stage II or III CRC who received adjuvant chemotherapy (n = 103), recurrence rates did not differ according to HSP110 T17 deletion size, 23.8% in the large deletion group versus 25.0% in the small deletion group (p = 0.91) or HSP110 expression, 28.0% in the high expression group versus 30.8% in the low expression group (p = 0.50). The TTR and DFS did not differ according to HSP110 T17 deletion size (p = 0.93 and p = 0.78, respectively) or HSP110 expression (p = 0.78 and p = 0.45, respectively) (Figures 3C,D). OS and CSS did not differ according to size of HSP110 T17 deletion (p = 0.75 and p = 0.49, respectively) or HSP110 expression (p = 0.44 and p = 0.83, respectively).
Concerning metastatic tumors (n = 59), rates of death did not differ according to HSP110 T17 deletion size (p = 0.21) or HSP110 expression (p = 0.23), and OS did not differ according to the size of HSP110 T17 deletion (p = 0.91) or HSP110 expression (p = 0.15).
There were conflicting results concerning the HSP110 T17 microsatellite for MSI determination as compared to the standard method (Pentaplex) but also concerning its prognostic value and its predictive value for adjuvant chemotherapy efficacy in MSI CRCs. Our study is the first to analyze both the expression of HSP110 and the size of the deletion of the HSP110 T17 microsatellite in a large multicenter study of 381 MSI CRCs. We identified a strong correlation between expression of HSP110 protein and size of deletion of HSP110 T17. With 95.5% sensitivity to identify dMMR/MSI CRCs, HSP110 T17 performance seems similar to actual standard tests, namely, MMR IHC and MSI molecular testing. That said, we did not observe any prognostic value or predictive value of adjuvant chemotherapy efficacy of HSP110 expression or deletion size of the HSP110 T17 microsatellite.
In our study, we performed both molecular testing and MMR IHC for MSI determination. In most studies on HSP110, dMMR/MSI CRCs were defined according to either MMR IHC or molecular testing but not both (Dorard et al., 2011; Collura et al., 2014; Buhard et al., 2016; Oh et al., 2017; Berardinelli et al., 2018; How-Kit et al., 2018). This important point is a major limitation to the evaluation of HSP110 T17 deletion as a diagnostic tool for MSI determination. MMR IHC presents sensitivity between 85 and 100% and specificity between 85 and 92% (Zhang and Li, 2013; Snowsill et al., 2017). Sensitivity and specificity of the Pentaplex panel range between 90 and 100% (Xicola et al., 2007; Goel et al., 2010). Moreover, some studies have shown discordance between MMR IHC and MSI molecular testing, ranging from 1 to 10% (Lindor et al., 2002; Watson et al., 2007; Jaffrelot et al., 2019; Guyot; De Salins et al., 2021). In our cohort, all tumors were initially determined as MSI using a robust test (Pentaplex panel) (Suraweera et al., 2002; Wong et al., 2006). Most tumors had MMR IHC tests (98.2%) and only 9 MSI/pMMR cases were identified (2.4%). These results were concordant with recent series (Lindor et al., 2002; Watson et al., 2007; Fazzalari, 2017; Cohen et al., 2018; Jaffrelot et al., 2019; De Salins et al., 2021). A recent work showed that ∼6% of MSI cases retained mismatch repair protein expression and would consequently be missed by IHC testing alone, thereby hindering patient access to immunotherapy (Hechtman et al., 2020). The majority of these cases harbor germline or somatic mismatch repair gene missense mutations; as a result, inactive mutant proteins remain detected by IHC (Peltomäki and Vasen, 2004). Consistently with previous studies, MSI CRCs were associated with female sex, proximal tumor sites, poorly differentiated tumors, and BRAF mutation (Tougeron et al., 2015).
In this study, we based our technical exploration of HSP110 on previous publications. For instance, Kim et al. developed specific IHC interpretation of HSP110 wt staining based on 3 different scores (Kim et al., 2014). We reproduced the same IHC technique and compared our results with theirs. We highlighted the high percentage of intra-tumor heterogeneity of HSP110 staining in MSI CRC (74.0%), which is similar to the Oh et al. study (81%) (Oh et al., 2017). It could be interesting to study whether HSP110 IHC heterogeneity is the reflection of molecular heterogeneity, and if so, whether it is the consequence of the variable deletion size of HSP110 T17 or of post-transcriptional modifications. Nevertheless, despite the intra-tumor heterogeneity, HSP110 groups were comparable between ours and the two published studies with 21.3, 19, and 24% low expression of HSP110 and 78.7, 81, and 76% high expression of HSP110, respectively (Kim et al., 2014; Oh et al., 2017). Since low HSP110 expression is observed in a part of dMMR/MSI CRCs with large HSP110 T17 deletion, while high HSP110 expression is observed not only in normal tissue and in pMMR/MSS CRCs but also in most dMMR/MSI CRCs with small HSP110 T17 deletion, HSP110 expression is not relevant as a diagnostic tool of dMMR/MSI status.
In our cohort, 94.2% of the MSI CRCs had HSP110 T17 deletion. This percentage was lower than in the Collura et al. study (97%) but equivalent to the Berardinelli et al. study (94%) and higher than the 88% obtained by Kim et al. (Collura et al., 2014; Kim et al., 2014; Berardinelli et al., 2018). The difference with Duval et al. and Berardinelli et al. results could partly be explained by their use of a ratio calculation method. This quantitative method consisted, in the absence of a clear HSP110 T17 deletion peak, of calculating ratios of the heights of peaks from the Taq polymerase stutter located at -1 and -2 bp of the polymorphic zone (Buhard et al., 2016). We chose not to use this calculation method because more than 5% of MSS CRC cases explored during our technical development of the HSP110 T17 deletion test would have then presented HSP110 T17 deletion. In addition, it was uneasy to clearly discriminate the peak corresponding to the deleted fragment from the stutter peaks of the Taq polymerase. Indeed, discrepancy among different studies could be explained by the difficulties of determining HSP110 T17 deletion size. Enrichment techniques have recently been described, including modification of the denaturation temperature during the PCR step, which may improve the sensitivity of the analysis of HSP110 T17 deletion (Baudrin et al., 2018a; Baudrin et al., 2018b; How-Kit et al., 2018).
HSP110 T17 successfully identified 4 cases that were wrongly classified MSI by standard procedure. Our study had not a MSS/pMMR group to determine the specificity of HSP110 T17 deletion in detection of dMMR/MSI CRC. Nevertheless, as previously mentioned, determination of small HSP110 T17 deletion (–1 or –2 bp) is complex, and we recorded some false negative results. Considering all of these limitations, the Pentaplex panel seems easier and more apt than HSP110 T17 deletion to determine MSI status. Buhard et al. suggested that compared with the Pentaplex panel, HSP110 T17 deletion showed better sensitivity (0.984 versus 0.951) and similar specificity (0.997) for MSI detection (Buhard et al., 2016). It is worth noting that in this study, only MSI test was performed and not both molecular and MMR IHC. In the Berardinelli et al. study, most cases underwent both MSI and MMR IHC tests and eight cases had discordant status (Berardinelli et al., 2018). Finally, HSP110 T17 could be interesting, not to replace MSI and MMR IHC testing, but rather as a complement to the five microsatellites used in the Pentaplex panel to improve detection of MSI cases. Moreover, this marker may be of major interest to investigate discordant cases (dMMR/MSS or pMMR/MSI).
Our study confirmed the correlation between HSP110 wt expression and HSP110 T17 deletion size, first pointed out by Kim et al. (2014). In addition, there was a correlation between the presence of nuclear staining and the deletion size of HSP110 T17 using a cut-off at 4 bp. For the first time, we identified a correlation between RAS mutations with both high HSP110 expression and small HSP110 T17 deletion. RAS mutations are rare in dMMR/MSI CRC as compared to pMMR/MSS tumors and we have no explanation concerning the association between RAS mutations with HSP110 T17 deletion size and HSP110 expression level. RAS testing is an important biomarker to predict sensitivity of anti-EGFR in both pMMR/MSS and dMMR/MSI metastatic CRC. However, by contrast to BRAF mutation, RAS mutation is not a prognosis factor in dMMR/MSI (Rimbert et al., 2017; Tougeron et al., 2020).
Survival and recurrence rates observed in our series were in accordance with previously published studies on dMMR/MSI CRCs (Ribic et al., 2003; Tougeron et al., 2015; Tougeron et al., 2016; Tougeron et al., 2020). Kim et al. assessed HSP110 IHC on 168 MSI CRC tissues, among which 167 were analyzed for HSP110 T17 deletion. Associations with clinicopathological, molecular, and survival parameters were statistically analyzed and Kim et al. found that low expression of HSP110 was significantly associated with better DFS in the whole cohort, in 5-FU–based adjuvant chemotherapy-treated patients and in the stage III/IV cohort. Analyzing stage III/IV simultaneously is questionable. Besides, the authors did not look specifically at stage II/III patients who received adjuvant 5-FU–based chemotherapy. Moreover, they did not reproduce these results in their second study, in which no correlation was found in either stage II/III patients who received fluoropyrimidine-based adjuvant chemotherapy or in the stage II/III patients who received a combination of 5-FU and oxaliplatin (Kim et al., 2015). In our series, we did not find an association between HSP110 expression and TTR, DFS, OS, or CSS in patients with MSI stage II and III CRC, regardless of whether or not they received adjuvant chemotherapy.
Large deletion of HSP110 T17 has been associated with efficacy of adjuvant chemotherapy (relapse-free survival, RFS), 5-FU alone or with oxaliplatin, in a cohort of 329 patients with stage II and III dMMR/MSI CRCs (Dorard et al., 2011; Collura et al., 2014). It is worth noting that in elderly patients with dMMR/MSI CRC, RFS or DFS is not the most relevant endpoint since some elderly patients could die from other diseases and since dMMR/MSI is associated with good prognosis (Tougeron et al., 2016). TTR seems most relevant. In our series, we did not found any correlation between large HSP110 T17 deletion and TTR/DFS in stage II and III patients receiving adjuvant chemotherapy. Similar to us, no significant survival difference between large and small HSP110 T17 deletions was observed in the Kim et al. study (Kim et al., 2014). In addition, only 39% of patients (n = 30/77) were treated with the adjuvant 5-FU plus oxaliplatin regimen in the Collura et al. cohort versus 80.6% (n = 83/103) in our study (Collura et al., 2014). This difference could be explained by the fact that 5-FU plus oxaliplatin became the standard adjuvant regimen in CRC only in 2004. Regarding these discordant results, HSP110 IHC score or size of HSP110 T17 deletion are neither robust prognostic biomarkers nor predictive factors of adjuvant chemotherapy efficacy in dMMR/MSI CRC.
Despite its retrospective nature and the prolonged period of the patient’s inclusion, there were few missing clinical data (10%) in our series. In addition, recent prognostic factors were available, like RAS and BRAF mutations and VELIPI status. One limitation of our study is that we performed a pooled analysis of patients with stage II and III MSI CRC for survival analyses to obtain greater statistical power; however, other series did the same (Collura et al., 2014; Kim et al., 2014). A meta-analysis of all series on HSP110 in MSI CRCs should be performed to confirm the absence of prognostic impact or predictive impact on response to chemotherapy. Up until now, our series is the largest published on MSI CRC patients with simultaneous molecular and IHC analyses of HSP110.
In conclusion, we observed a strong correlation between deletion size of HSP110 T17 and expression of HSP110. While the HSP110 T17 microsatellite had high sensitivity to detect dMMR/MSI CRC, false negative cases existed. Even though the gold standard for determination of MSI status remains a combination of Pentaplex panel and MMR IHC, the HSP110 T17 microsatellite could help to classify discordant cases. In our series, as in others, we did not identify HSP110 expression or size of HSP110 T17 deletion as prognostic markers or predictors of adjuvant chemotherapy efficacy in MSI CRC. Based on our series and the literature, input of HSP110 for diagnosis or prognosis of dMMR/MSI CRC seems low.
The original contributions presented in the study are included in the article/Supplementary Material; further inquiries can be directed to the corresponding author.
The studies involving human participants were reviewed and approved by the Comité de protection des personnes Ouest III. Written informed consent for participation was not required for this study in accordance with the national legislation and the institutional requirements.
DT was responsible for the conceptualization of the study. DT and LK-T secured funding and designed the experiment. GT, DT, and LK-T designed the methodology. AC-S-T, TL, AJ, EF, EM-S, JG, CE, VR, RC, M-LA, VM, SG, GF, and DT participated in the resource production and data curation. GT, AC-S-T, and DT wrote the original draft preparation. All authors contributed to manuscript revision and read and approved the submitted version. DT is the guarantor of this work and, as such, had full access to all of the data in the study and takes responsibility for the integrity of the data and the accuracy of the data analysis.
This work was supported by a grant from the associations “Sport et Collection” and “Ligue Contre le Cancer, Comités départementaux de la Vienne, Charente et Charente-Maritime.”
The authors declare that the research was conducted in the absence of any commercial or financial relationships that could be construed as a potential conflict of interest.
All claims expressed in this article are solely those of the authors and do not necessarily represent those of their affiliated organizations, or those of the publisher, the editors, and the reviewers. Any product that may be evaluated in this article, or claim that may be made by its manufacturer, is not guaranteed or endorsed by the publisher.
The authors thank all of the clinical research technicians, Céline Archaimbault, Marine Martel, Mélanie Chaffaud, Roxane Aguillon, Sébastien Martin, and Pierre Rivet, who participated in MMR IHC, MSI determination, and HSP110 exploration. Our original English-language manuscript was reread and revised by Jeffrey Arsham, an American medical translator.
The Supplementary Material for this article can be found online at: https://www.frontiersin.org/articles/10.3389/fgene.2021.769281/full#supplementary-material
Bp, base pairs; CRC, colorectal cancer; CSS, cancer-specific survival; DFS, disease-free survival; dMMR, deficient mismatch repair system; HSP, heat shock proteins; IHC, immunohistochemistry; MSI, microsatellite instability; OS, overall survival; pMMR, proficient MMR; STAT3, signal transducer and activator of transcription 3; TTR, time to recurrence; VELIPI, vascular emboli or lymphatic invasion or perinervous invasion; wt, wild-type.
André, T., Shiu, K.-K., Kim, T. W., Jensen, B. V., Jensen, L. H., Punt, C., et al. (2020). Pembrolizumab in Microsatellite-Instability-High Advanced Colorectal Cancer. N. Engl. J. Med. 383, 2207–2218. doi:10.1056/NEJMoa2017699
Augustus, G. J., and Ellis, N. A. (2018). Colorectal Cancer Disparity in African Americans. Am. J. Pathol. 188, 291–303. doi:10.1016/j.ajpath.2017.07.023
Baudrin, L. G., Deleuze, J.-F., and How-Kit, A. (2018a). Molecular and Computational Methods for the Detection of Microsatellite Instability in Cancer. Front. Oncol. 8, 621. doi:10.3389/fonc.2018.00621
Baudrin, L. G., Duval, A., Daunay, A., Buhard, O., Bui, H., Deleuze, J.-F., et al. (2018b). Improved Microsatellite Instability Detection and Identification by Nuclease-Assisted Microsatellite Instability Enrichment Using HSP110 T17. Clin. Chem. 64, 1252–1253. doi:10.1373/clinchem.2018.287490
Berardinelli, G. N., Scapulatempo-Neto, C., Durães, R., Antônio de Oliveira, M., Guimarães, D., and Reis, R. M. (2018). Advantage of HSP110 (T17) Marker Inclusion for Microsatellite Instability (MSI) Detection in Colorectal Cancer Patients. Oncotarget 9, 28691–28701. doi:10.18632/oncotarget.25611
Berthenet, K., Bokhari, A. d., Lagrange, A., Marcion, G., Boudesco, C., Causse, S., et al. (2017). HSP110 Promotes Colorectal Cancer Growth through STAT3 Activation. Oncogene 36, 2328–2336. doi:10.1038/onc.2016.403
Birgisson, H., Wallin, U., Holmberg, L., and Glimelius, B. (2011). Survival Endpoints in Colorectal Cancer and the Effect of Second Primary Other Cancer on Disease Free Survival. BMC Cancer 11, 438. doi:10.1186/1471-2407-11-438
Buhard, O., Cattaneo, F., Wong, Y. F., Yim, S. F., Friedman, E., Flejou, J.-F., et al. (2006). Multipopulation Analysis of Polymorphisms in Five Mononucleotide Repeats Used to Determine the Microsatellite Instability Status of Human Tumors. Jco 24, 241–251. doi:10.1200/JCO.2005.02.7227
Buhard, O., Lagrange, A., Guilloux, A., Colas, C., Chouchène, M., Wanherdrick, K., et al. (2016). HSP110T17 Simplifies and Improves the Microsatellite Instability Testing in Patients with Colorectal Cancer. J. Med. Genet. 53, 377–384. doi:10.1136/jmedgenet-2015-103518
Cohen, R., Hain, E., Buhard, O., Guilloux, A., Bardier, A., Kaci, R., et al. (2018). Assessment of Local Clinical Practice for Testing of Mismatch Repair Deficiency in Metastatic Colorectal Cancer: The Need for New Diagnostic Guidelines Prior to Immunotherapy. Ann. Oncol. 29, viii179–viii180. doi:10.1093/annonc/mdy281.083
Collura, A., Lagrange, A., Svrcek, M., Marisa, L., Buhard, O., Guilloux, A., et al. (2014). Patients with Colorectal Tumors with Microsatellite Instability and Large Deletions in HSP110 T17 Have Improved Response to 5-Fluorouracil-Based Chemotherapy. Gastroenterology 146, 401–411. doi:10.1053/j.gastro.2013.10.054
De Salins, A. G. D. A., Tachon, G., Cohen, R., Karayan-Tapon, L., Junca, A., Frouin, E., et al. (2021). Discordance between Immunochemistry of Mismatch Repair Proteins and Molecular Testing of Microsatellite Instability in Colorectal Cancer. ESMO Open 6, 100120. doi:10.1016/j.esmoop.2021.100120
Dorard, C., de Thonel, A., Collura, A., Marisa, L., Svrcek, M., Lagrange, A., et al. (2011). Expression of a Mutant HSP110 Sensitizes Colorectal Cancer Cells to Chemotherapy and Improves Disease Prognosis. Nat. Med. 17, 1283–1289. doi:10.1038/nm.2457
Evrard, C., Tachon, G., Randrian, V., Karayan-Tapon, L., and Tougeron, D. (2019). Microsatellite Instability: Diagnosis, Heterogeneity, Discordance, and Clinical Impact in Colorectal Cancer. Cancers 11, 1567. doi:10.3390/cancers11101567
Fazzalari, L. (2017). Étude des discordances entre les analyses d’immunohistochimie et de biologie moléculaire à la recherche d’une instabilité microsatellitaire avec les analyses de génétique constitutionnelle dans le cadre du syndrome de Lynch. Medical thesis. 132.
Goel, A., Nagasaka, T., Hamelin, R., and Boland, C. R. (2010). An Optimized Pentaplex PCR for Detecting DNA Mismatch Repair-Deficient Colorectal Cancers. PLoS ONE 5, e9393. doi:10.1371/journal.pone.0009393
Hechtman, J. F., Rana, S., Middha, S., Stadler, Z. K., Latham, A., Benayed, R., et al. (2020). Retained Mismatch Repair Protein Expression Occurs in Approximately 6% of Microsatellite Instability-High Cancers and Is Associated with Missense Mutations in Mismatch Repair Genes. Mod. Pathol. 33, 871–879. doi:10.1038/s41379-019-0414-6
How-Kit, A., Daunay, A., Buhard, O., Meiller, C., Sahbatou, M., Collura, A., et al. (2018). Major Improvement in the Detection of Microsatellite Instability in Colorectal Cancer Using HSP110 T17 E-Ice -COLD-PCR. Hum. Mutat. 39, 441–453. doi:10.1002/humu.23379
Jaffrelot, M., Selves, J., Fares, N., Staub, A., Laurenty, A. P., Danjoux, M., et al. (2019). Characterization of Atypical dMMR (Deficient MisMatch Repair) Tumors: A Study from a Large Cohort of 4948 Cases. Ann. Oncol. 30, v807. doi:10.1093/annonc/mdz269.033
Kim, J. H., Bae, J. M., Oh, H. J., Lee, H. S., and Kang, G. H. (2015). Pathologic Factors Associated with Prognosis after Adjuvant Chemotherapy in Stage II/III Microsatellite-Unstable Colorectal Cancers. J. Pathol. Transl Med. 49, 118–128. doi:10.4132/jptm.2015.02.05
Kim, J. H., Kim, K.-J., Rhee, Y.-Y., Oh, S., Cho, N.-Y., Lee, H. S., et al. (2014). Expression Status of Wild-type HSP110 Correlates with HSP110 T17 Deletion Size and Patient Prognosis in Microsatellite-Unstable Colorectal Cancer. Mod. Pathol. 27, 443–453. doi:10.1038/modpathol.2013.160
Le, D. T., Uram, J. N., Wang, H., Bartlett, B. R., Kemberling, H., Eyring, A. D., et al. (2015). PD-1 Blockade in Tumors with Mismatch-Repair Deficiency. N. Engl. J. Med. 372, 2509–2520. doi:10.1056/NEJMoa1500596
Lindor, N. M., Burgart, L. J., Leontovich, O., Goldberg, R. M., Cunningham, J. M., Sargent, D. J., et al. (2002). Immunohistochemistry versus Microsatellite Instability Testing in Phenotyping Colorectal Tumors. Jco 20, 1043–1048. doi:10.1200/JCO.2002.20.4.1043
Oh, H. J., Kim, J. H., Lee, T. H., Park, H. E., Bae, J. M., Lee, H. S., et al. (2017). Dominant High Expression of Wild-type HSP110 Defines a Poor Prognostic Subgroup of Colorectal Carcinomas with Microsatellite Instability: a Whole-Section Immunohistochemical Analysis. APMIS 125, 1076–1083. doi:10.1111/apm.12770
Peltomäki, P., and Vasen, H. (2004). Mutations Associated with HNPCC Predisposition - Update of ICG-HNPCC/INSiGHT Mutation Database. Dis. Markers 20, 269–276. doi:10.1155/2004/305058
Ribic, C. M., Sargent, D. J., Moore, M. J., Thibodeau, S. N., French, A. J., Goldberg, R. M., et al. (2003). Tumor Microsatellite-Instability Status as a Predictor of Benefit from Fluorouracil-Based Adjuvant Chemotherapy for colon Cancer. N. Engl. J. Med. 349, 247–257. doi:10.1056/NEJMoa022289
Rimbert, J., Tachon, G., Junca, A., Villalva, C., Karayan-Tapon, L., and Tougeron, D. (2017). Association between Clinicopathological Characteristics and RAS Mutation in Colorectal Cancer. Mod. Pathol. 31, 517–526. doi:10.1038/modpathol.2017.119
Sargent, D. J., Marsoni, S., Monges, G., Thibodeau, S. N., Labianca, R., Hamilton, S. R., et al. (2010). Defective Mismatch Repair as a Predictive Marker for Lack of Efficacy of Fluorouracil-Based Adjuvant Therapy in colon Cancer. Jco 28, 3219–3226. doi:10.1200/JCO.2009.27.1825
Singh, N., Wong, R., Tchrakian, N., Allen, S.-G., Clarke, B., and Gilks, C. B. (2021). Interpretation of mismatch repair protein expression using obsolete criteria results in discrepancies with microsatellite instability and mutational testing results. Comment on Hechtman et al. Mod Pathol 2020; 33:871-879. Mod. Pathol. 34, 1031–1032. doi:10.1038/s41379-020-00680-y
Snowsill, T., Coelho, H., Huxley, N., Jones-Hughes, T., Briscoe, S., Frayling, I. M., et al. (2017). Molecular Testing for Lynch Syndrome in People with Colorectal Cancer: Systematic Reviews and Economic Evaluation. Health Technol. Assess. 21, 1–238. doi:10.3310/hta21510
Suraweera, N., Duval, A., Reperant, M., Vaury, C., Furlan, D., Leroy, K., et al. (2002). Evaluation of Tumor Microsatellite Instability Using Five Quasimonomorphic Mononucleotide Repeats and Pentaplex PCR. Gastroenterology 123, 1804–1811. doi:10.1053/gast.2002.37070
Tachon, G., Frouin, E., Karayan-Tapon, L., Auriault, M.-L., Godet, J., Moulin, V., et al. (2018). Heterogeneity of Mismatch Repair Defect in Colorectal Cancer and its Implications in Clinical Practice. Eur. J. Cancer 95, 112–116. doi:10.1016/j.ejca.2018.01.087
Tougeron, D., Fauquembergue, E., Rouquette, A., Le Pessot, F., Sesboüé, R., Laurent, M., et al. (2009). Tumor-infiltrating Lymphocytes in Colorectal Cancers with Microsatellite Instability Are Correlated with the Number and Spectrum of Frameshift Mutations. Mod. Pathol. 22, 1186–1195. doi:10.1038/modpathol.2009.80
Tougeron, D., Mouillet, G., Trouilloud, I., Lecomte, T., Coriat, R., Aparicio, T., et al. (2016). Efficacy of Adjuvant Chemotherapy in Colon Cancer with Microsatellite Instability: A Large Multicenter AGEO Study. JNCI.J 108, djv438. doi:10.1093/jnci/djv438
Tougeron, D., Sickersen, G., Mouillet, G., Zaanan, A., Trouilloud, I., Coriat, R., et al. (2015). Predictors of Disease-free Survival in Colorectal Cancer with Microsatellite Instability: An AGEO Multicentre Study. Eur. J. Cancer 51, 925–934. doi:10.1016/j.ejca.2015.03.011
Tougeron, D., Sueur, B., Zaanan, A., Fouchardiére, C., Sefrioui, D., Lecomte, T., et al. (2020). Prognosis and Chemosensitivity of Deficient MMR Phenotype in Patients with Metastatic Colorectal Cancer: An AGEO Retrospective Multicenter Study. Int. J. Cancer 147, 285–296. doi:10.1002/ijc.32879
Umar, A., Boland, C. R., Terdiman, J. P., Syngal, S., Chapelle, A. d. l., Rüschoff, J., et al. (2004). Revised Bethesda Guidelines for Hereditary Nonpolyposis Colorectal Cancer (Lynch Syndrome) and Microsatellite Instability. JNCI J. Natl. Cancer Inst. 96, 261–268. doi:10.1093/jnci/djh034
Watson, N., Grieu, F., Morris, M., Harvey, J., Stewart, C., Schofield, L., et al. (2007). Heterogeneous Staining for Mismatch Repair Proteins during Population-Based Prescreening for Hereditary Nonpolyposis Colorectal Cancer. J. Mol. Diagn. 9, 472–478. doi:10.2353/jmoldx.2007.060162
Wong, Y. F., Cheung, T. H., Lo, K. W. K., Yim, S. F., Chan, L. K. Y., Buhard, O., et al. (2006). Detection of Microsatellite Instability in Endometrial Cancer: Advantages of a Panel of Five Mononucleotide Repeats over the National Cancer Institute Panel of Markers. Carcinogenesis 27, 951–955. doi:10.1093/carcin/bgi333
Xicola, R. M., Llor, X., Pons, E., Castells, A., Alenda, C., Piñol, V., et al. (2007). Performance of Different Microsatellite Marker Panels for Detection of Mismatch Repair-Deficient Colorectal Tumors. J. Natl. Cancer Inst. 99, 244–252. doi:10.1093/jnci/djk033
Zhang, X., and Li, J. (2013). Era of Universal Testing of Microsatellite Instability in Colorectal Cancer. Wjgo 5, 12–19. doi:10.4251/wjgo.v5.i2.12
Keywords: colorectal cancer, microsatellite instability, deficient mismatch repair, HSP110, adjuvant chemotherapy, biomarker, Lynch syndrome
Citation: Tachon G, Chong-Si-Tsaon A, Lecomte T, Junca A, Frouin É, Miquelestorena-Standley E, Godet J, Evrard C, Randrian V, Chautard R, Auriault M-L, Moulin V, Guyetant S, Fromont G, Karayan-Tapon L and Tougeron D (2022) HSP110 as a Diagnostic but Not a Prognostic Biomarker in Colorectal Cancer With Microsatellite Instability. Front. Genet. 12:769281. doi: 10.3389/fgene.2021.769281
Received: 01 September 2021; Accepted: 30 November 2021;
Published: 03 January 2022.
Edited by:
Kai Ye, Xi’an Jiaotong University, ChinaReviewed by:
Richarda Maria De Voer, Radboud University Nijmegen Medical Centre, NetherlandsCopyright © 2022 Tachon, Chong-Si-Tsaon, Lecomte, Junca, Frouin, Miquelestorena-Standley, Godet, Evrard, Randrian, Chautard, Auriault, Moulin, Guyetant, Fromont, Karayan-Tapon and Tougeron. This is an open-access article distributed under the terms of the Creative Commons Attribution License (CC BY). The use, distribution or reproduction in other forums is permitted, provided the original author(s) and the copyright owner(s) are credited and that the original publication in this journal is cited, in accordance with accepted academic practice. No use, distribution or reproduction is permitted which does not comply with these terms.
*Correspondence: David Tougeron, ZGF2aWR0b3VnZXJvbkBob3RtYWlsLmZy, ZGF2aWQudG91Z2Vyb25AY2h1LXBvaXRpZXJzLmZy
Disclaimer: All claims expressed in this article are solely those of the authors and do not necessarily represent those of their affiliated organizations, or those of the publisher, the editors and the reviewers. Any product that may be evaluated in this article or claim that may be made by its manufacturer is not guaranteed or endorsed by the publisher.
Research integrity at Frontiers
Learn more about the work of our research integrity team to safeguard the quality of each article we publish.