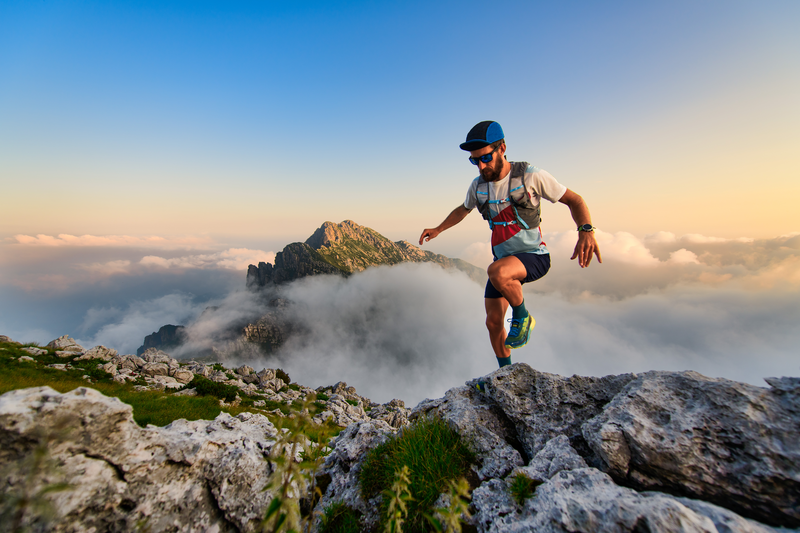
94% of researchers rate our articles as excellent or good
Learn more about the work of our research integrity team to safeguard the quality of each article we publish.
Find out more
ORIGINAL RESEARCH article
Front. Genet. , 04 August 2021
Sec. Genetics of Common and Rare Diseases
Volume 12 - 2021 | https://doi.org/10.3389/fgene.2021.677748
This article is part of the Research Topic Genetic and Environmental Factors in the Occurrence of Neonatal Disorders - Volume I View all 10 articles
Next-generation sequencing (NGS) has been used to detect severe combined immunodeficiency (SCID) in patients, and some patients with DNA cross-link repair 1C (DCLRE1C) variants have been identified. Moreover, some compound variants, such as copy number variants (CNV) and single nucleotide variants (SNV), have been reported. The purpose of this study was to expand the genetic data related to patients with SCID carrying the compound DCLRE1C variant. Whole-exome sequencing (WES) was performed for genetic analysis, and variants were verified by performing Sanger sequencing or quantitative PCR. Moreover, we searched PubMed and summarized the data of the reported variants. Four SCID patients with DCLRE1C variants were identified in this study. WES revealed a homozygous deletion in the DCLRE1C gene from exons 1–5 in patient 1, exons 1–3 deletion and a novel rare variant (c.92T>C, p.L31P) in patient 2, exons 1–3 deletion and a novel rare variant (c.328C>G, p.L110V) in patient 3, and exons 1–4 deletion and a novel frameshift variant (c.449dup, p.His151Alafs*20) in patient 4. Based on literature review, exons 1–3 was recognized as a hotspot region for deletion variation. Moreover, we found that compound variations (CNV + SNV) accounted for approximately 7% variations in all variants. When patients are screened for T-cell receptor excision circles (TRECs), NGS can be used to expand genetic testing. Deletion of the DCLRE1C gene should not be ignored when a variant has been found in patients with SCID.
Severe combined immunodeficiency (SCID), one of the most severe forms of primary immunodeficiency diseases, is characterized by a deficiency of T-cell, B-cell, and sometimes NK-cell responses to infections. The reported incidence of SCID ranges from one per 50,000 to one per 1,00,000 live births (Lipstein et al., 2010). The affected patients fail to clear infections and usually die early in life, even with treatment. Studies have shown that genetic abnormalities are associated with the development of SCID. To date, more than 40 genes have been reported to be associated with SCID.
The DNA cross-link repair 1C (DCLRE1C) gene, also known as ARTEMIS, is located on chromosome 10p13. It encodes the nuclease ARTEMIS, a protein with 5ꞌ–3ꞌ exonuclease activity for single-stranded DNA. ARTEMIS plays an important role in V(D)J recombination that occurs during B- and T-cell development. Variations in the DCLRE1C gene is associated with autosomal recessive SCID by affecting the V(D)J recombination. In 2001, Moshous et al. (2001) reported 13 patients with SCID who carried DCLRE1C gene point variants/exon deletions. Since then, an increasing number of patients have been reported, some of which were found during newborn screening (Rechavi et al., 2017). Notably, some compound variations [copy number variation (CNV) + single nucleotide variation (SNV)] in the DCLRE1C were identified by Sanger sequencing or PCR (Moshous et al., 2001, 2003). However, these traditional methods (Sanger or PCR) can only sequence short DNA fragments. The development of next-generation sequencing (NGS) has accelerated genomics studies. NGS can simultaneously sequence more than 100 genes and has a shorter turnaround time. To date, several studies (Luk et al., 2017; Sundin et al., 2018; Strand et al., 2020) have reported the use of NGS for the detection of SCID patients, and some patients with DCLRE1C variants were identified. However, the detection of CNV by NGS remains a challenge because of the issues intrinsic to the technology (such as short read lengths, etc.; Moreno-Cabrera et al., 2020). Thus, some deletions in DCLRE1C may be missed by NGS.
Here, we present four cases of SCID caused by DCLRE1C variants. All patients were diagnosed with SCID by a physician (two of them were identified during newborn screening). The genetic information of SCID patients carrying the DCLRE1C variants was collected from reported studies. The purpose of this study was to expand knowledge related to the genetic information of SCID patients carrying the DCLRE1C variant.
Four patients were included in the present study. Informed consent was obtained from the parents or guardians of the patients. The study was approved by the Ethics Committee of the Children’s Hospital of Fudan University.
At least 2 ml peripheral blood was obtained from the patients and their parents into tubes with EDTA. Genomic DNA was extracted using a TIANGEN DNA Blood Mini Kit. Subsequently, the DNA fragments were enriched using the Agilent SureSelect XT Human All Exon V5 kit. Sequencing was performed on an Illumina HiSeq X10 platform. The average on-target sequencing depth was 120X. Burrows-Wheeler Aligner was used to align the sequencing reads to the reference genome hg19 (Li and Durbin, 2009). The variants were called based on the genome analysis toolkit Best Practices, and a variant call format file was generated. The detailed methods for the data analysis are present in our published study (Yang et al., 2019). For CNV analysis, pipeline for clinical NGS-involved CNV detection (PICNIC) was used to detect CNV from whole-exome sequencing (WES) data. The PICNIC can filter out high-frequency gene deletions/duplications (which occurred in >10% of the internal samples). In addition, we used Database of Genomic Variants, the Database of Chromosomal Imbalance and Phenotype in Humans using Ensemble Resources, and our internal database (Wang et al., 2014) for region-level annotation. More information about CNV detection can be seen in our previous study (Dong et al., 2020).
Deletions in the DCLRE1C were confirmed using quantitative PCR (qPCR). The primers were designed using Primer Premier 5.0. The details on the primers are shown in Supplementary Table S1. The qPCR mixture (10 μl) contained 5.0 μl SYBR Green mix (2X), 0.2 μl ROX Reference Dye II (50X), 0.5 μmol/L of each primer for the target region and for lactate dehydrogenase A as the reference gene, 20 μg DNA, and ddH2O. The following conditions were used for qPCR: an initial 45-s 95°C period followed by 40 cycles of amplification (5 s at 95°C, 30 s at 60°C, and 30 s at 72°C) using the real-time PCR system (Applied Biosystems StepOnePlus). Relative quantification was performed using the 2-ΔΔCt method. The copy numbers that were less than 0.8, between 0.8 and 1.4, and more than 1.4 mean deletion, normal, and duplication, respectively.
Sanger sequencing was performed to validate the identified variants. Sequencing was conducted using an automated sequencer (3500XL Genetic Analyzer, Applied Biosystems, United States). The primers were designed using Primer Premier 5.0. The Mutation Surveyor (SoftGenetics®, State College, PA, United States) was used for sequence analysis.
All the clinical features of our patients are listed in Table 1.
Patient 1 (P1) had severe pneumonia at 3 months of age. Her sputum test revealed an infection with Pneumocystis carinii and Candida albicans. Flow cytometry revealed a decrease in the expression of CD3 and CD19. She died at 4 months of age after unclear treatment at a local hospital. Blood samples were sent to the laboratory for genetic testing.
Patient 2 (P2) developed eczema all over the body without any known cause at 2 months of age. At 4 months of age, the patient was diagnosed with pneumonia due to cough and a recurrent fever. Subsequently, he was diagnosed with Acinetobacter baumannii infection and had a recurrent fever, which was identified at a local hospital. He was admitted to our hospital for further treatment at 6 months and 15 days of age. However, the family refused hemopoietic stem cell transplantation at 10 months and 14 days of age.
Patient 3 (P3) presented with fever and vomiting at 4 months of age. She was diagnosed with acute upper respiratory infection, septicemia, and low white blood cell count at a local hospital. She was treated with antibiotics, but the patient frequently had a fever. She visited our hospital for further treatment at 9 months and 3 days of age. However, her parents refused hematopoietic stem cell transplantation, and there was no further follow-up after 1 year and 10 months of age.
Patient 4 (P4) started coughing without a known reason at 2 months of age. Her parents used Chinese herbs, but her symptoms did not improve. At 4 months of age, she had a fever, which increased up to 39.3°C. In addition, an approximately 4-cm mass was found in the left axilla. She was diagnosed with immunodeficiency and tuberculous infection. Her genetic test at the local hospital showed no variation in RAG1 and RAG2. The patient was subsequently referred to our hospital for further treatment at the age of 6 months. She underwent hematopoietic stem cell transplantation at the age of 7 months. Follow-up at 15 months showed normal growth and development.
Whole-exome sequencing was performed on peripheral blood sample obtained from the patients and their biological parents. In this study, both the CNV and SNV results were generated. Genetic testing revealed a homozygous deletion of the DCLRE1C gene from exons 1–5 in P1 (Figure 1A). P2 had exons 1–3 deletion and a mosaic novel rare variant (c.92T>C, p.L31P; Figure 1B); the short tandem repeat test showed that maternal cells accounted for 31.36% in patient 2. P3 had a compound heterozygous exons 1–3 deletion and a novel rare variant (c.328C>G, p.L110V; Figure 1C). P4 had a heterozygous exons 1–4 deletion and a novel frameshift variant (c.449dup, p.His151Alafs*20; Figure 1D). All variants and deletions of each patient were confirmed by Sanger sequencing and qPCR, respectively (Figure 1). Supplementary Table S2 shows the prediction of pathogenicity for novel variants.
Figure 1. (A) A family pedigree of patient 1. Whole-exome sequencing (WES) revealed a homozygous deletion of DNA cross-link repair 1C (DCLRE1C) gene from exons 1–5 in this patient, and quantitative PCR (qPCR) verified it. (B) A family pedigree of patient 2. WES revealed exons 1–3 deletion and a novel rare variant (c.92T>C, p.L31P). The qPCR verified exons 1–3 deletion, and Sanger sequencing confirmed the variant (c.92T>C, p.L31P). (C) A family pedigree of patient 3. WES revealed heterozygous exons 1–3 deletion and a novel rare variant (c.328C>G, p.L110V). The qPCR verified exons 1–3 deletion, and Sanger sequencing confirmed the variant (c.328C>G, p.L110V). (D) A family pedigree of patient 4. WES revealed heterozygous exons 1–4 deletion and a novel frameshift variant (c.449_450insT). The qPCR verified exons 1–4 deletion, and Sanger sequencing confirmed the variant (c.449dup, p.His151Alafs*20).
Combining Human Gene Mutation Database (HGMD) and PubMed, a total of 26 publications that reported SCID related to DCLRE1C until March 2021 were included for analysis. The genetic characteristics of patients from published studies (Moshous et al., 2001, 2003; Li et al., 2002; Kobayashi et al., 2003; Noordzij et al., 2003; Musio et al., 2005; Evans et al., 2006; Darroudi et al., 2007; van der Burg et al., 2007; Lagresle-Peyrou et al., 2008; Alsmadi et al., 2009; Pannicke et al., 2010; Woodbine et al., 2010; Ijspeert et al., 2011; Lee et al., 2011, 2013; Tomashov-Matar et al., 2012; Bajin et al., 2013; Halbrich et al., 2013; Schuetz et al., 2014; Felgentreff et al., 2015; Lobachevsky et al., 2015; Volk et al., 2015; Al-Mousa et al., 2016; Dvorak et al., 2017; Luk et al., 2017; Rechavi et al., 2017; Stray-Pedersen et al., 2017; Tahiat et al., 2017; Al-Herz et al., 2018; Sundin et al., 2018, 2019; Krantz et al., 2019; Wu et al., 2019; Dasouki et al., 2020; Fayez et al., 2020; Firtina et al., 2020; Kalina et al., 2020; Simon et al., 2020; Strand et al., 2020; Vignesh et al., 2020) are listed in Supplementary Table S3. In total, 87 variants were recorded in HGMD as SCID. A combined literature review was performed on studies published on PubMed, and 153 patients were reported with the DCLRE1C variants. A total of 116 patients had detailed zygosity information. Of them, 14 patients were compound heterozygous, 10 patients were heterozygous, and 92 patients were homozygous. In total, 37 studies indicated the test methods. Of these studies, 18 studies used PCR, 15 studies (including eight WES) used NGS, two studies used Sanger sequencing, one study used a genome-wide scan, and one study used multiplex ligation-dependent probe amplification. We observed that the exon deletions were approximately one-third (55/153) of the total variations in SCID patients carrying DCLRE1C variants. Among these deletions, more than 50% (33/55) were exons 1–3 deletion; exons 1–3 was recognized as a hotspot region for deletion variation. Moreover, compound variations (CNV + SNV) accounted for approximately 7% (11/153) of variations in all variants.
In this study, four SCID patients with deletions in exons 1–5 were identified. P1 had homozygous exon deletions, while P2, P3, and P4 carried compound heterozygous variants (CNV + SNV). In all the patients, these deletions were inherited from the father, while the point variants were inherited from the mother. We propose the following as a possible mechanism for the observations made in this study. P1 had homozygous exons 1–5 deletion, which is caused by homologous recombination and results in SCID. P2 and P3 carried compound heterozygous variants with exons 1–3 deletion and a missense variant. Exons 1–3 deletion can account for the absence of ARTEMIS protein expression due to homology and should be considered a null allele (Pannicke et al., 2010). These two novel missense variants (p.L31P and p.L110V) are located on exons 1 and 5, respectively. Exons 1 and 2 are important for ARTEMIS endonucleolytic features (Pannicke et al., 2004). Exons 5 and 6 are necessary for maintaining the ARTEMIS structure (Pannicke et al., 2004). Since exons 1–3 deletion is a null allele, p.L31P probably influences the active site of ARTEMIS, and p.L110V likely affects the protein structure, thereby leading to SCID. P4 had a heterozygous exons 1–4 deletion along with a frameshift variant (c.449dup) in exons 6. Felgentreff et al. (2015) verified that a frameshift variant can disrupt domains and almost completely abrogate the ARTEMIS function. We speculated that this frameshift variant probably affects the balance of the ARTEMIS structure and results in SCID.
Our literature review analyzed 26 publications and summarized that exons 1–3 is a hotspot region for deletion. The major mechanism for this hotspot deletion is homologous recombination (Meek et al., 2004). Moreover, Pannicke et al. (2010) found that there was a series of short interspersed nuclear element, long interspersed nuclear element, long terminal repeat, simple repeat, and low complexity repeat sequences in this region that can enhance the recombination events. Although the above-mentioned mechanisms have been identified, more evidence from functional studies are needed.
In addition, our literature review found that compound variations (CNV + SNV) accounted for approximately 7% of the total variants, and deletion in DCLRE1C gene was mainly tested by PCR. Currently, NGS is a more powerful tool for obtaining genetic test results than PCR. However, small CNV calling remains challenging due to variable coverage and data analysis pipelines (Teo et al., 2012). WES analysis pipelines still leave a gap of small deletion calling between SNVs/small insertions/deletions and CNVs larger than 1 Mb. In recent years, the development of long-read sequencing, directly sequencing single molecules of DNA in real time, can help for the detection of complex chromosomal rearrangements including deletions, inversions, insertions, and duplications (McGinty et al., 2017). However, long-read sequencing has several limitations, such as high sequencing error rate, high systematic error, and higher cost than NGS (Xiao and Zhou, 2020).
Traditional exome sequencing mainly concentrates on the detection of SNVs. The small deletion may be missed by these methods. Subsequently, several algorithms, including CANOES (Backenroth et al., 2014), XHMM (Fromer et al., 2012), and Co NIFER (Krumm et al., 2012), have been developed for CNV analysis, and recent studies (Yang et al., 2013; Retterer et al., 2015; Ellingford et al., 2018) showed the good performance of CNV analysis through WES. In this study, we used PICNIC (Qin et al., 2018), which demonstrated 100% specificity and sensitivity to pathogenic/likely pathogenic CNVs, for the detection of CNVs. Totally, four cases were diagnosed with SCID, with three compounds heterozygous and one homozygous deletion of the DCLRE1C gene, which was detected by WES. Without PICNIC, these cases may have been left undiagnosed if the deletion calling was missed. In addition, qPCR can be further used for deletion validation due to the limitations of NGS. Notably, healthy individuals can also harbor variations in the DCLRE1C gene. Thus, healthcare professionals should consider the possibility of deletion when a variant is found in the DCLRE1C gene.
Currently, newborns are being screened for SCID in some countries by assessing T-cell receptor excision circles (TREC). Krantz et al. (2019) reported two familial patients diagnosed with SCID who carried the same compound DCLRE1C deletions. However, the outcomes for these patients were different: the one who underwent TREC screening and early genetic diagnosis survived with SCID prior to the onset of infections. Although TREC is useful for SCID screening, SCID diagnosis should be confirmed by genetic testing. Although only 5% of SCID patients carry a variant of DCLRE1C, these patients (87.5%, 7/8) had 10-year survival rate higher than that of RAG1/2 SCID patients (64.4%, 9/13) after hematopoietic stem cell transplantation (Hamid et al., 2018). Therefore, the early diagnosis of DCLRE1C SCID may provide patients with effective treatment and a better chance of survival.
In conclusion, we reported four SCID cases with five allelic deletions in exons 1–5. When patients are screened for the presence of TREC to diagnose SCID, NGS can be used for further genetic testing. Gross deletions in the DCLRE1C should not be ignored when a variant has been identified.
The datasets for this article are not publicly available due to concerns regarding participant/patient anonymity. Requests to access the datasets should be directed to the corresponding authors.
The studies involving human participants were reviewed and approved by the Ethics Committee of Children’s Hospital of Fudan University. Written informed consent to participate in this study was provided by the participants’ legal guardian/next of kin. Written informed consent was obtained from the minor(s)’ legal guardian/next of kin for the publication of any potentially identifiable images or data included in this article.
HW, FX, and WZ conceptualized the study and analyzed the data. YL, BW, BL, GL, PZ, QZ, and JS co-conceptualized the study and interpreted the data. All authors contributed to the drafting of the article and revised it critically for important intellectual content. All authors contributed to the article and approved the submitted version.
The authors declare that the research was conducted in the absence of any commercial or financial relationships that could be construed as a potential conflict of interest.
All claims expressed in this article are solely those of the authors and do not necessarily represent those of their affiliated organizations, or those of the publisher, the editors and the reviewers. Any product that may be evaluated in this article, or claim that may be made by its manufacturer, is not guaranteed or endorsed by the publisher.
The Supplementary Material for this article can be found online at: https://www.frontiersin.org/articles/10.3389/fgene.2021.677748/full#supplementary-material
Al-Herz, W., Massaad, M. J., Chou, J., Notarangelo, L. D., and Geha, R. S. (2018). DNA recombination defects in Kuwait: clinical, immunologic and genetic profile. Clin. Immunol. 187, 68–75. doi: 10.1016/j.clim.2017.10.006
Al-Mousa, H., Abouelhoda, M., Monies, D. M., Al-Tassan, N., Al-Ghonaium, A., Al-Saud, B., et al. (2016). Unbiased targeted next-generation sequencing molecular approach for primary immunodeficiency diseases. J. Allergy Clin. Immunol. 137, 1780–1787. doi: 10.1016/j.jaci.2015.12.1310
Alsmadi, O., Al-Ghonaium, A., Al-Muhsen, S., Arnaout, R., Al-Dhekri, H., Al-Saud, B., et al. (2009). Molecular analysis of T-B-NK+ severe combined immunodeficiency and Omenn syndrome cases in Saudi Arabia. BMC Med. Genet. 10:116. doi: 10.1186/1471-2350-10-116
Backenroth, D., Homsy, J., Murillo, L. R., Glessner, J., Lin, E., Brueckner, M., et al. (2014). CANOES: detecting rare copy number variants from whole exome sequencing data. Nucleic Acids Res. 42:e97. doi: 10.1093/nar/gku345
Bajin, İ. Y., Ayvaz, D., Ünal, S., Özgür, T. T., Çetin, M., Gümrük, F., et al. (2013). Atypical combined immunodeficiency due to Artemis defect: a case presenting as hyperimmunoglobulin M syndrome and with LGLL. Mol. Immunol. 56, 354–357. doi: 10.1016/j.molimm.2013.05.004
Darroudi, F., Wiegant, W., Meijers, M., Friedl, A. A., van der Burg, M., Fomina, J., et al. (2007). Role of Artemis in DSB repair and guarding chromosomal stability following exposure to ionizing radiation at different stages of cell cycle. Mutat. Res. 615, 111–124. doi: 10.1016/j.mrfmmm.2006.11.029
Dasouki, M., Jabr, A., AlDakheel, G., Elbadaoui, F., Alazami, A. M., Al-Saud, B., et al. (2020). TREC and KREC profiling as a representative of thymus and bone marrow output in patients with various inborn errors of immunity. Clin. Exp. Immunol. 202, 60–71. doi: 10.1111/cei.13484
Dong, X., Liu, B., Yang, L., Wang, H., Wu, B., Liu, R., et al. (2020). Clinical exome sequencing as the first-tier test for diagnosing developmental disorders covering both CNV and SNV: a Chinese cohort. J. Med. Genet. 57, 558–566. doi: 10.1136/jmedgenet-2019-106377
Dvorak, C. C., Patel, K., Puck, J. M., Wahlstrom, J., Dorsey, M. J., Adams, R., et al. (2017). Unconditioned unrelated donor bone marrow transplantation for IL7Rα- and Artemis-deficient SCID. Bone Marrow Transplant. 52, 1036–1038. doi: 10.1038/bmt.2017.74
Ellingford, J. M., Horn, B., Campbell, C., Arno, G., Barton, S., Tate, C., et al. (2018). Assessment of the incorporation of CNV surveillance into gene panel next-generation sequencing testing for inherited retinal diseases. J. Med. Genet. 55, 114–121. doi: 10.1136/jmedgenet-2017-104791
Evans, P. M., Woodbine, L., Riballo, E., Gennery, A. R., Hubank, M., and Jeggo, P. A. (2006). Radiation-induced delayed cell death in a hypomorphic Artemis cell line. Hum. Mol. Genet. 15, 1303–1311. doi: 10.1093/hmg/ddl050
Fayez, E. A., Qazvini, F. F., Mahmoudi, S. M., Khoei, S., Vesaltalab, M., and Teimourian, S. (2020). Diagnosis of radiosensitive severe combined immunodeficiency disease (RS-SCID) by comet assay, management of bone marrow transplantation. Immunobiology 225:151961. doi: 10.1016/j.imbio.2020.151961
Felgentreff, K., Lee, Y. N., Frugoni, F., Du, L., van der Burg, M., Giliani, S., et al. (2015). Functional analysis of naturally occurring DCLRE1C mutations and correlation with the clinical phenotype of ARTEMIS deficiency. J. Allergy Clin. Immunol. 136, 140.e7–150.e7. doi: 10.1016/j.jaci.2015.03.005
Firtina, S., Ng, Y. Y., Ng, O. H., Kiykim, A., Aydiner, E., Nepesov, S., et al. (2020). Mutational landscape of severe combined immunodeficiency patients from Turkey. Int. J. Immunogenet. 47, 529–538. doi: 10.1111/iji.12496
Fromer, M., Moran, J. L., Chambert, K., Banks, E., Bergen, S. E., Ruderfer, D. M., et al. (2012). Discovery and statistical genotyping of copy-number variation from whole-exome sequencing depth. Am. J. Hum. Genet. 91, 597–607. doi: 10.1016/j.ajhg.2012.08.005
Halbrich, M., Ben-Shoshan, M., and McCusker, C. (2013). Autoimmune hemolytic anemia in a teenager: a wolf in sheep's clothing. Eur. J. Haematol. 91, 262–264. doi: 10.1111/ejh.12148
Hamid, I. J. A., Slatter, M. A., McKendrick, F., Pearce, M. S., and Gennery, A. R. (2018). Long-term health outcome and quality of life post-HSCT for IL7Ralpha-, Artemis-, RAG1- and RAG2-deficient severe combined immunodeficiency: a single center report. J. Clin. Immunol. 38, 727–732. doi: 10.1007/s10875-018-0540-9
Ijspeert, H., Lankester, A. C., van den Berg, J. M., Wiegant, W., van Zelm, M. C., Weemaes, C. M., et al. (2011). Artemis splice defects cause atypical SCID and can be restored in vitro by an antisense oligonucleotide. Genes Immun. 12, 434–444. doi: 10.1038/gene.2011.16
Kalina, T., Bakardjieva, M., Blom, M., Perez-Andres, M., Barendregt, B., Kanderová, V., et al. (2020). Euroflow standardized approach to diagnostic immunopheneotyping of severe PID in newborns and young children. Front. Immunol. 11:371. doi: 10.3389/fimmu.2020.00371
Kobayashi, N., Agematsu, K., Sugita, K., Sako, M., Nonoyama, S., Yachie, A., et al. (2003). Novel Artemis gene mutations of radiosensitive severe combined immunodeficiency in Japanese families. Hum. Genet. 112, 348–352. doi: 10.1007/s00439-002-0897-x
Krantz, M. S., Stone, C. A., Connelly, J. A., Norton, A. E., and Khan, Y. W. (2019). The effect of delayed and early diagnosis in siblings, and importance of newborn screening for SCID. Ann. Allergy Asthma Immunol. 122, 211–213. doi: 10.1016/j.anai.2018.11.002
Krumm, N., Sudmant, P. H., Ko, A., O’Roak, B. J., Malig, M., Coe, B. P., et al. (2012). Copy number variation detection and genotyping from exome sequence data. Genome Res. 22, 1525–1532. doi: 10.1101/gr.138115.112
Lagresle-Peyrou, C., Benjelloun, F., Hue, C., Andre-Schmutz, I., Bonhomme, D., Forveille, M., et al. (2008). Restoration of human B-cell differentiation into NOD-SCID mice engrafted with gene-corrected CD34+ cells isolated from Artemis or RAG1-deficient patients. Mol. Ther. 16, 396–403. doi: 10.1038/sj.mt.6300353
Lee, P. P., Chan, K. W., Chen, T. X., Jiang, L. P., Wang, X. C., Zeng, H. S., et al. (2011). Molecular diagnosis of severe combined immunodeficiency--identification of IL2RG, JAK3, IL7R, DCLRE1C, RAG1, and RAG2 mutations in a cohort of Chinese and southeast Asian children. J. Clin. Immunol. 31, 281–296. doi: 10.1007/s10875-010-9489-z
Lee, P. P., Woodbine, L., Gilmour, K. C., Bibi, S., Cale, C. M., Amrolia, P. J., et al. (2013). The many faces of Artemis-deficient combined immunodeficiency—two patients with DCLRE1C mutations and a systematic literature review of genotype-phenotype correlation. Clin. Immunol. 149, 464–474. doi: 10.1016/j.clim.2013.08.006
Li, H., and Durbin, R. (2009). Fast and accurate short read alignment with burrows-wheeler transform. Bioinformatics 25, 1754–1760. doi: 10.1093/bioinformatics/btp324
Li, L., Moshous, D., Zhou, Y., Wang, J., Xie, G., Salido, E., et al. (2002). A founder mutation in Artemis, an SNM1-like protein, causes SCID in Athabascan-speaking native Americans. J. Immunol. 168, 6323–6329. doi: 10.4049/jimmunol.168.12.6323
Lipstein, E. A., Vorono, S., Browning, M. F., Green, N. S., Kemper, A. R., Knapp, A. A., et al. (2010). Systematic evidence review of newborn screening and treatment of severe combined immunodeficiency. Pediatrics 125, e1226–e1235. doi: 10.1542/peds.2009-1567
Lobachevsky, P., Woodbine, L., Hsiao, K. C., Choo, S., Fraser, C., Gray, P., et al. (2015). Evaluation of severe combined immunodeficiency and combined immunodeficiency pediatric patients on the basis of cellular radiosensitivity. J. Mol. Diagn. 17, 560–575. doi: 10.1016/j.jmoldx.2015.05.004
Luk, A. D. W., Lee, P. P., Mao, H., Chan, K. W., Chen, X. Y., Chen, T. X., et al. (2017). Family history of early infant death correlates with earlier age at diagnosis but not shorter time to diagnosis for severe combined immunodeficiency. Front. Immunol. 8:808. doi: 10.3389/fimmu.2017.00808
McGinty, R. J., Rubinstein, R. G., Neil, A. J., Dominska, M., Kiktev, D., Petes, T. D., et al. (2017). Nanopore sequencing of complex genomic rearrangements in yeast reveals mechanisms of repeat-mediated double-strand break repair. Genome Res. 27, 2072–2082. doi: 10.1101/gr.228148.117
Meek, K., Gupta, S., Ramsden, D. A., and Lees-Miller, S. P. (2004). The DNA-dependent protein kinase: the director at the end. Immunol. Rev. 200, 132–141. doi: 10.1111/j.0105-2896.2004.00162.x
Moreno-Cabrera, J. M., Del Valle, J., Castellanos, E., Feliubadaló, L., Pineda, M., Brunet, J., et al. (2020). Evaluation of CNV detection tools for NGS panel data in genetic diagnostics. Eur. J. Hum. Genet. 28, 1645–1655. doi: 10.1038/s41431-020-0675-z
Moshous, D., Callebaut, I., de Chasseval, R., Corneo, B., Cavazzana-Calvo, M., Le Deist, F., et al. (2001). Artemis, a novel DNA double-strand break repair/V(D)J recombination protein, is mutated in human severe combined immune deficiency. Cell 105, 177–186. doi: 10.1016/S0092-8674(01)00309-9
Moshous, D., Pannetier, C., de Chasseval Rd, R., le Deist Fl, F., Cavazzana-Calvo, M., Romana, S., et al. (2003). Partial T and B lymphocyte immunodeficiency and predisposition to lymphoma in patients with hypomorphic mutations in Artemis. J. Clin. Invest. 111, 381–387. doi: 10.1172/JCI16774
Musio, A., Marrella, V., Sobacchi, C., Rucci, F., Fariselli, L., Giliani, S., et al. (2005). Damaging-agent sensitivity of Artemis-deficient cell lines. Eur. J. Immunol. 35, 1250–1256. doi: 10.1002/eji.200425555
Noordzij, J. G., Verkaik, N. S., van der Burg, M., van Veelen, L. R., de Bruin-Versteeg, S., Wiegant, W., et al. (2003). Radiosensitive SCID patients with Artemis gene mutations show a complete B-cell differentiation arrest at the pre-B-cell receptor checkpoint in bone marrow. Blood 101, 1446–1452. doi: 10.1182/blood-2002-01-0187
Pannicke, U., Hönig, M., Schulze, I., Rohr, J., Heinz, G. A., Braun, S., et al. (2010). The most frequent DCLRE1C (ARTEMIS) mutations are based on homologous recombination events. Hum. Mutat. 31, 197–207. doi: 10.1002/humu.21168
Pannicke, U., Ma, Y., Hopfner, K. P., Niewolik, D., Lieber, M. R., and Schwarz, K. (2004). Functional and biochemical dissection of the structure-specific nuclease ARTEMIS. EMBO J. 23, 1987–1997. doi: 10.1038/sj.emboj.7600206
Qin, Q., Liu, B., Yang, L., Wu, B., Wang, H., Dong, X., et al. (2018). Application of copy number variation screening analysis process based on high-throughput sequencing technology. Chin. J. Evid. Based Pediatr. 13, 275–279. doi: 10.3969/j.issn.1673-5501.2018.04.007
Rechavi, E., Lev, A., Simon, A. J., Stauber, T., Daas, S., Saraf-Levy, T., et al. (2017). First year of Israeli newborn screening for severe combined immunodeficiency-clinical achievements and insights. Front. Immunol. 8:1448. doi: 10.3389/fimmu.2017.01448
Retterer, K., Scuffins, J., Schmidt, D., Lewis, R., Pineda-Alvarez, D., Stafford, A., et al. (2015). Assessing copy number from exome sequencing and exome array CGH based on CNV spectrum in a large clinical cohort. Genet. Med. 17, 623–629. doi: 10.1038/gim.2014.160
Schuetz, C., Neven, B., Dvorak, C. C., Leroy, S., Ege, M. J., Pannicke, U., et al. (2014). SCID patients with Artemis vs. RAG deficiencies following HCT: increased risk of late toxicity in Artemis-deficient SCID. Blood 123, 281–289. doi: 10.1182/blood-2013-01-476432
Simon, A. J., Golan, A. C., Lev, A., Stauber, T., Barel, O., Somekh, I., et al. (2020). Whole exome sequencing (WES) approach for diagnosing primary immunodeficiencies (PIDs) in a highly consanguineous community. Clin. Immunol. 214:108376. doi: 10.1016/j.clim.2020.108376
Strand, J., Gul, K. A., Erichsen, H. C., Lundman, E., Berge, M. C., Trømborg, A. K., et al. (2020). Second-tier next generation sequencing integrated in nationwide newborn screening provides rapid molecular diagnostics of severe combined immunodeficiency. Front. Immunol. 11:1417. doi: 10.3389/fimmu.2020.01417
Stray-Pedersen, A., Sorte, H. S., Samarakoon, P., Gambin, T., Chinn, I. K., Akdemir, Z. H. C., et al. (2017). Primary immunodeficiency diseases: genomic approaches delineate heterogeneous Mendelian disorders. J. Allergy Clin. Immunol. 139, 232–245. doi: 10.1016/j.jaci.2016.05.042
Sundin, M., Marits, P., Ramme, K., Kolios, A. G. A., and Nilsson, J. (2019). Severe combined immunodeficiency (SCID) presenting in childhood, with agammaglobulinemia, associated with novel compound heterozygous mutations in DCLRE1C. Clin. Immunol. 200, 16–18. doi: 10.1016/j.clim.2018.12.019
Sundin, M., Uhlin, M., Gaballa, A., Ramme, K., Kolios, A. G., Marits, P., et al. (2018). Late presenting atypical severe combined immunodeficiency (SCID) associated with a novel missense mutation in DCLRE1C. Pediatr. Allergy Immunol. 29, 108–111. doi: 10.1111/pai.12812
Tahiat, A., Badran, Y. R., Chou, J., Cangemi, B., Lefranc, G., Labgaa, Z. M., et al. (2017). Epidermodysplasia verruciformis as a manifestation of ARTEMIS deficiency in a young adult. J. Allergy Clin. Immunol. 139, 372.e4–375.e4. doi: 10.1016/j.jaci.2016.07.024
Teo, S. M., Pawitan, Y., Ku, C. S., Chia, K. S., and Salim, A. (2012). Statistical challenges associated with detecting copy number variations with next-generation sequencing. Bioinformatics 28, 2711–2718. doi: 10.1093/bioinformatics/bts535
Tomashov-Matar, R., Biran, G., Lagovsky, I., Kotler, N., Stein, A., Fisch, B., et al. (2012). Severe combined immunodeficiency (SCID): from the detection of a new mutation to preimplantation genetic diagnosis. J. Assist. Reprod. Genet. 29, 687–692. doi: 10.1007/s10815-012-9765-3
van der Burg, M., Verkaik, N. S., den Dekker, A. T., Barendregt, B. H., Pico-Knijnenburg, I., Tezcan, I., et al. (2007). Defective Artemis nuclease is characterized by coding joints with microhomology in long palindromic-nucleotide stretches. Eur. J. Immunol. 37, 3522–3528. doi: 10.1002/eji.200737624
Vignesh, P., Rawat, A., Kumrah, R., Singh, A., Gummadi, A., Sharma, M., et al. (2020). Clinical, immunological, and molecular features of severe combined immune deficiency: a multi-institutional experience from India. Front. Immunol. 11:619146. doi: 10.3389/fimmu.2020.619146
Volk, T., Pannicke, U., Reisli, I., Bulashevska, A., Ritter, J., Björkman, A., et al. (2015). DCLRE1C (ARTEMIS) mutations causing phenotypes ranging from atypical severe combined immunodeficiency to mere antibody deficiency. Hum. Mol. Genet. 24, 7361–7372. doi: 10.1093/hmg/ddv437
Wang, H., Bi, W., Wu, B., Zhou, W., and Cheung, S. (2014). Application and explanation of chromosome microarray (CMA) analysis in the diagnosis of clinical genetic disease. Chin. J. Evid. Based Pediatr. 9, 227–235. doi: 10.3969/j.issn.1673-5501.2014.03.013
Woodbine, L., Grigoriadou, S., Goodarzi, A. A., Riballo, E., Tape, C., Oliver, A. W., et al. (2010). An Artemis polymorphic variant reduces Artemis activity and confers cellular radiosensitivity. DNA Repair 9, 1003–1010. doi: 10.1016/j.dnarep.2010.07.001
Wu, Z., Subramanian, N., Jacobsen, E. M., Sampaio, K. L., van der Merwe, J., Hönig, M., et al. (2019). NK cells from RAG- or DCLRE1C-deficient patients inhibit HCMV. Microorganisms 7:546. doi: 10.3390/microorganisms7110546
Xiao, T., and Zhou, W. (2020). The third generation sequencing: the advanced approach to genetic diseases. Transl. Pediatr. 9, 163–173. doi: 10.21037/tp.2020.03.06
Yang, L., Kong, Y., Dong, X., Hu, L., Lin, Y., Chen, X., et al. (2019). Clinical and genetic spectrum of a large cohort of children with epilepsy in China. Genet. Med. 21, 564–571. doi: 10.1038/s41436-018-0091-8
Keywords: severe combined immunodeficiency, DCLRE1C gene, copy number variation, single nucleotide variation, sequencing
Citation: Xiao F, Lu Y, Wu B, Liu B, Li G, Zhang P, Zhou Q, Sun J, Wang H and Zhou W (2021) High-Frequency Exon Deletion of DNA Cross-Link Repair 1C Accounting for Severe Combined Immunodeficiency May Be Missed by Whole-Exome Sequencing. Front. Genet. 12:677748. doi: 10.3389/fgene.2021.677748
Received: 08 March 2021; Accepted: 28 June 2021;
Published: 04 August 2021.
Edited by:
Desheng Liang, Central South University, ChinaReviewed by:
María Eugenia De La Morena-Barrio, University of Murcia, SpainCopyright © 2021 Xiao, Lu, Wu, Liu, Li, Zhang, Zhou, Sun, Wang and Zhou. This is an open-access article distributed under the terms of the Creative Commons Attribution License (CC BY). The use, distribution or reproduction in other forums is permitted, provided the original author(s) and the copyright owner(s) are credited and that the original publication in this journal is cited, in accordance with accepted academic practice. No use, distribution or reproduction is permitted which does not comply with these terms.
*Correspondence: Huijun Wang, huijunwang@fudan.edu.cn; Wenhao Zhou, zhouwenhao@fudan.edu.cn
Disclaimer: All claims expressed in this article are solely those of the authors and do not necessarily represent those of their affiliated organizations, or those of the publisher, the editors and the reviewers. Any product that may be evaluated in this article or claim that may be made by its manufacturer is not guaranteed or endorsed by the publisher.
Research integrity at Frontiers
Learn more about the work of our research integrity team to safeguard the quality of each article we publish.