- 1Department of Pharmacology and Clinical Pharmacy, College of Health Sciences, Addis Ababa University, Addis Ababa, Ethiopia
- 2Division of Population Health and Genomics, Ninewells Hospital and School of Medicine, University of Dundee, Dundee, United Kingdom
Background: Adverse drug reactions (ADR) are a major clinical problem accounting for significant hospital admission rates, morbidity, mortality, and health care costs. One-third of people with diabetes experience at least one ADR. However, there is notable interindividual heterogeneity resulting in patient harm and unnecessary medical costs. Genomics is at the forefront of research to understand interindividual variability, and there are many genotype-drug response associations in diabetes with inconsistent findings. Here, we conducted a systematic review to comprehensively examine and synthesize the effect of genetic polymorphisms on the incidence of ADRs of oral glucose-lowering drugs in people with type 2 diabetes.
Methods: A literature search was made to identify articles that included specific results of research on genetic polymorphism and adverse effects associated with oral glucose-lowering drugs. The electronic search was carried out on 3rd October 2020, through Cochrane Library, PubMed, and Web of Science using keywords and MeSH terms.
Result: Eighteen articles consisting of 10, 383 subjects were included in this review. Carriers of reduced-function alleles of organic cation transporter 1 (OCT 1, encoded by SLC22A1) or reduced expression alleles of plasma membrane monoamine transporter (PMAT, encoded by SLC29A4) or serotonin transporter (SERT, encoded by SLC6A4) were associated with increased incidence of metformin-related gastrointestinal (GI) adverse effects. These effects were shown to exacerbate by concomitant treatment with gut transporter inhibiting drugs. The CYP2C9 alleles, *2 (rs1799853C>T) and *3 (rs1057910A>C) that are predictive of low enzyme activity were more common in subjects who experienced hypoglycemia after treatment with sulfonylureas. However, there was no significant association between sulfonylurea-related hypoglycemia and genetic variants in the ATP-binding cassette transporter sub-family C member 8 (ABCC8)/Potassium Inwardly Rectifying Channel Subfamily J Member 11 (KCNJ11). Compared to the wild type, the low enzyme activity C allele at CYP2C8*3 (rs1057910A>C) was associated with less weight gain whereas the C allele at rs6123045 in the NFATC2 gene was significantly associated with edema from rosiglitazone treatment.
Conclusion: In spite of limited studies investigating genetics and ADR in diabetes, some convincing results are emerging. Genetic variants in genes encoding drug transporters and metabolizing enzymes are implicated in metformin-related GI adverse effects, and sulfonylurea-induced hypoglycemia, respectively. Further studies to investigate newer antidiabetic drugs such as DPP-4i, GLP-1RA, and SGLT2i are warranted. In addition, pharmacogenetic studies that account for race and ethnic differences are required.
Introduction
Diabetes mellitus refers to a group of metabolic disorders characterized by hyperglycemia resulting from insufficient production and/or ineffective response of cells to insulin. It is one of the major contributors to morbidity and mortality globally, and its prevalence continues to rise. By 2019, an estimated 463 million adults aged 20–79 years were living with diabetes which accounts 9.3% of the global population in this age group (International Diabetes Federation, 2019). This figure is expected to surge to 578 million (10.2%) by 2030 and to 700 million (10.9%) by 2045, most of this in the form of type 2 diabetes (T2D) (International Diabetes Federation, 2019). Poorly controlled diabetes progressively leads to chronic microvascular, macrovascular and neuropathic complications which manifest as renal failure, blindness, lower limb amputation, and accelerated vascular disease.
Several drugs are available for the management of T2D, referred to as glucose lowering agents. These include: biguanides (metformin), insulin secretagogues (sulfonylureas, meglitinides), thiazolidinediones, alpha glucosidase inhibitors (acarbose), incretin mimetics (GLP-1RAs, DPP-4is), amylin antagonists, sodium-glucose co-transporter-2 inhibitors (SGLT-2i), and insulin. These classes of drugs are either prescribed as monotherapy or given in combination.
The management of T2D is guided by national and international evidence-based guidelines (Buse et al., 2020), but there is noticeable inter-individual variability in treatment response as defined by glycemic reduction and adverse drug reactions. This variation is the net effect of several environmental and clinical factors including age, sex, adherence, concomitant therapy, drug interactions, and disease severity. In addition to these, a patient's genotype can affect interindividual differences in drug response.
Adverse drug reactions are major clinical and public health problems world-wide. In the UK, around 6.5% of hospital admissions are due to adverse drug reactions (Pirmohamed et al., 2004), and that almost 15% of patients experience an ADR during their admission (Davies et al., 2009, 2010). The projected annual cost of such admissions to the NHS was £466m (Davies et al., 2009). Glucose lowering agents are one of the drugs of great concern for ADRs (Ducoffe et al., 2016). They have a well-described set of ADRs that are detrimental to individuals' health and quality of life (Table 1). In the US, from 2010 to 2013, there were 600,991 ADRs associated with glucose-lowering agents with an average hospital marginal cost of $4, 312 resulting in an annual hospital cost of $2.59 billion (Spector et al., 2017). Optimizing drug therapy through the avoidance of ADRs will dramatically improve patient health while generating millions of dollars by saving unnecessary medical costs.
However, there is inter-individual variability in the type and severity of ADRs experienced by individuals taking glucose-lowering drugs. While clinical and environmental factors influence this, genomic factors are also important. Here, we aim to undertake a systematic review of pharmacogenomic studies of ADRs related to oral glucose-lowering drugs.
Methods
This systematic review is reported according to the Preferred Reporting Items for the Systematic Reviews and Meta-analysis Protocols (PRISMA-P) 2015 Checklist (Shamseer et al., 2015).
Type of Participants
Participants included in eligible studies must be diagnosed with T2D and treated with oral glucose-lowering drugs.
Type of Exposure
We included studies in which participants genotype were investigated in relation to ADRs of oral glucose-lowering agents.
Outcomes
The primary outcome was the incidence of any of the adverse effects of glucose-lowering drugs (Table 1). For metformin, GI adverse effects were considered - hypoglycemia and weight gain for sulfonylureas and weight gain and edema for thiazolidinediones.
Eligibility Criteria
Inclusion Criteria
Studies assessing the effect of genetic variations on the incidence of adverse effects of oral glucose-lowering drugs in people with T2D, published up to 3rd October 2020 in English language without any geographical restriction were included in this review.
Exclusion Criteria
We did not consider news, qualitative studies, case reports, reviews, editorials, and comments; and all non-published studies and published in non-English languages. Studies in which relevant data on genetic polymorphisms and/or ADRs associated with oral glucose-lowering agents is lacking or impossible to extract were also excluded.
Search Strategy for Identifying Relevant Studies
A literature search was made to identify articles that included specific results of research on genetic polymorphisms and adverse effects associated with oral glucose-lowering agents. The electronic search was carried out on 3rd October 2020, through Cochrane Library, PubMed, and Web of Science using keywords and MeSH terms with no restriction for time of publication. The search strategy conducted in MEDLINE via PubMed, Cochrane, and Web of Science is shown in Supplementary Material. We also manually searched reference lists from relevant studies and contact experts in the field in order to identify additional eligible studies.
Selection of Studies for Inclusion in the Review
Two review authors (AMB and AYD) independently identified articles and then screened their titles and abstracts for eligibility. Thereafter, full texts of articles considered to be eligible were retrieved. Furthermore, the review authors independently assessed the eligibility for inclusion in the review based on the inclusion and exclusion criteria. Disagreements between the two authors was resolved by consensus. A PRISMA flow diagram (Shamseer et al., 2015) was employed to document the process of literature selection and the reasons for exclusion of articles were stated.
Data Extraction and Management
Two review authors (AMB and AYD) independently extracted data from the articles reviewed. A data extraction form for this purpose was designed. Data was collected on the first author's name, year of publication, geographical location (country where the study was performed), sample size, population characteristics, relevant genetic polymorphism, primary outcome measurements (incidence of adverse events after treatment with oral glucose-lowering agents). Any disagreements between the two review authors were resolved through discussion and consensus.
Study characteristics and the effect estimates of genetic polymorphisms on the incidence of adverse effects of oral glucose-lowering agents was presented in full, in tabular form. We summarized findings based on the type of oral glucose-lowering agent.
Result
Study Selection
Of the 362 studies identified, 66 were included for review of the full text. Of these studies, 18 studies met the inclusion criteria (Figure 1).
Characteristics of Included Studies
Eighteen articles comprised of 10, 383 subjects were included in this review (Table 2). Among these, two studies were multinational (Bailey et al., 2010; Dawed et al., 2019). While 12 studies were conducted in Europe (Holstein et al., 2005, 2011, 2012; Ragia et al., 2009, 2012, 2014; Tarasova et al., 2012; Dujic et al., 2015, 2016a,b, 2018; Dawed et al., 2016), three were in Asia (Kahn et al., 2006; Sato et al., 2010; Gökalp et al., 2011) and one was in the US (Ruaño et al., 2009).
Five of these studies comprising of 5,438 subjects were conducted to investigate association between genetic polymorphisms and metformin-related GI adverse effects (Tarasova et al., 2012; Dujic et al., 2015, 2016a,b; Dawed et al., 2019). Association between genetic polymorphisms and the risks of sulfonylurea-induced hypoglycemia was evaluated in nine studies comprising of 1,944 subjects (Holstein et al., 2005, 2011, 2012; Ragia et al., 2009, 2012, 2014; Sato et al., 2010; Gökalp et al., 2011; Dujic et al., 2018). Among the sulfonylureas, glimepiride was the most investigated (nine studies) (Holstein et al., 2005, 2012; Ragia et al., 2009, 2012, 2014; Sato et al., 2010; Gökalp et al., 2011; Dujic et al., 2018) followed by gliclazide (Ragia et al., 2009, 2012, 2014; Gökalp et al., 2011; Dujic et al., 2018) (five studies) and glibenclamide (Holstein et al., 2005, 2011, 2012; Sato et al., 2010; Dujic et al., 2018) (five studies). Four studies consisting of 3, 001 subjects evaluated the genetics of thiazolidinediones-induced edema and weight gain (Kang et al., 2006; Ruaño et al., 2009; Bailey et al., 2010; Dawed et al., 2016).
Metformin
Metformin is the first-line therapy for T2D. Around 30% of metformin treated subjects experience gastrointestinal (GI) side effects manifested as nausea, indigestion, abdominal cramps, bloating, diarrhea, or combination of these (Table 1) (Garber et al., 1997; Hirst J. A. et al., 2012). Metformin is an organic cation, and carrier proteins mediate its oral absorption, hepatic uptake, and renal elimination. Several solute carrier transporters, expressed in the membranes of the enterocytes, could be involved in the absorption of metformin from the intestinal lumen, including organic cation transporter (OCT) 1, plasma membrane monoamine transporter (PMAT), carnitine/cation transporter 1, OCT3 (encoded by SLC22A3), and serotonin reuptake transporter (SERT) (Han et al., 2015). Genetic variants in genes encoding these transporters have been reported in five articles (Table 3) (Tarasova et al., 2012; Dujic et al., 2015, 2016a,b; Dawed et al., 2019).
Association between genetic variants in SLC22A1 (a gene encoding OCT1), and GI intolerance related to metformin therapy have been reported in three studies (Tarasova et al., 2012; Dujic et al., 2015, 2016a). A study conducted using the GoDARTS cohort (Hébert et al., 2018), in 251 metformin-intolerant and 1,915 metformin-tolerant individuals showed that the presence of two or more reduced-function alleles at R61C, C88R, G401S, M420del, or G465R increased the odds of GI side effects of metformin by more than 2-fold (Dujic et al., 2015). This effect was over 4-fold with concomitant use of OCT1-inhibiting drugs. The findings were replicated in another prospective observational cohort study from Bosnia and Herzegovina that included 92 newly diagnosed subjects in the first 6 months of metformin treatment (Dujic et al., 2016a). Likewise, Tarasova et al. showed significant associations between a SNP (rs628031) and an 8 bp insertion (rs36056065) in the SLC22A1, with GI side effects of metformin (Tarasova et al., 2012).
Dawed et al. reported association between rs3889348, a variant that alters intestinal expression of the SLC9A4 (a gene encoding PMAT), with metformin related GI intolerance in 286 severe intolerant and 1,128 tolerant subjects. The G allele that reduces expression of SLC29A4 was associated with 34% higher odds of intolerance. Concomitant administration of metformin transporter inhibiting drugs exacerbate GI intolerance by more than 3-folds (Dawed et al., 2019).
Considering involvement of serotonin reuptake transporter (SERT) in metformin intestinal absorption, Dujic et al. investigated association between the low-expressing S* allele derived from a composite SERT (SLC6A4)-5-HTTLPR/rs25531 genotypes and metformin intolerance. In this study, each S* allele was associated with 30% higher odds of intolerance. Interestingly, a multiplicative interaction between OCT1 and SERT genotypes was observed (Dujic et al., 2016b). Carriers of reduced function alleles in OCT1 at the background of the wild type SERT (L*L*) genotype had greater odds of intolerance (OR 9.25 [3.18–27.0]) compared to carriers of the S* allele.
Sulfonylureas
Sulfonylureas were the first oral glucose-lowering therapy introduced into clinical practice and along with metformin, are the most prescribed drugs for the management of T2D (Inzucchi et al., 2015). SU are transported into the liver by OATP1B1 (encoded by SLCO1B1) and metabolized mainly by the polymorphic CYP2C9 enzyme and to a lesser extent by CYP2C19 enzyme (Becker et al., 2008). CYP2C9*2 (R144C, rs1799853) and CYP2C9*3 (I359L, rs1057910) are the two most common variants that have been associated with poor metabolism of SU (Semiz et al., 2010). Sulfonylureas induce glucose-independent insulin release from the pancreatic β-cells by binding to the ATP-sensitive potassium (KATP) channels, SUR1 and Kir6.2, that are encoded by the ABCC8 and KCNJ11 genes, respectively.
Hypoglycemia is the most common adverse effect of SU. In a systematic review consisting of 22 randomized controlled trials, 10.1 and 5.9% of SU treated subjects experienced hypoglycemia as defined by blood glucose levels of ≤ 3.1 or ≤ 2.8 mmol/L, respectively (Schopman et al., 2014). Severe hypoglycemia with SU therapy is less common, with reported incidence of 0.8%. SU treatment also results in weight gain of 1–3 kg (Schopman et al., 2014). Risk of hypoglycemia and weight gain may vary with age, gender, renal function, disease progression, drug exposure, and genetic constitution.
In this systematic review, we have included studies that investigated association between genetic variants in genes, CYP2C9 and ABCC8/KCNJ11, that encode CYP2C9 and SUR1/Kir6.2 with risk of hypoglycemia (Holstein et al., 2005, 2011, 2012; Ragia et al., 2009, 2012, 2014; Gökalp et al., 2011) (Table 4). An association between reduced function CYP2C9*2 and CYP2C9*3 alleles with higher risk of SU related hypoglycemia was reported confirming earlier functional and pharmacokinetic data (Ragia et al., 2009; Gökalp et al., 2011). However, another study could not confirm the findings (Holstein et al., 2011). In the later study subjects within the control arm that carry slow metabolizing alleles were found to be treated with significantly lower doses than carriers of the wild type, whereas in the group with severe hypoglycemia, the dose was the same for all genotype groups. Another small study suggested a possible interaction between P450 oxidoreductase (POR) and CYP2C9 genotypes (Ragia et al., 2014), where POR*28 allele could mask the effect of CYP2C9*2 allele on sulfonylurea-induced hypoglycemia. Indeed, a bigger study from the GoDARTS cohort confirmed this and therefore it is worth considering CYP2C9 and POR genotypes jointly in studies involving the pharmacogenetics of SU (Dujic et al., 2018).
Three other studies investigated association between two strongly linked non-synonymous polymorphisms, S1369A (rs757110) and E23K (rs5219), in the ABCC8 and KCNJ11 genes, respectively with hypoglycemia (Sato et al., 2010; Holstein et al., 2012; Ragia et al., 2012). None of these studies showed statistically significant association between SU treatment and risk of hypoglycemia suggesting these polymorphisms may not play a major role in the etiology of hypoglycemia.
Thiazolidinediones
Thiazolidinediones are insulin sensitizers that act by increasing the transactivation activity of Peroxisome Proliferators Activated Receptors (PPARs). The clinically used TZDs, rosiglitazone and pioglitazone, suffer from serious side effects. Concerns about the cardiovascular safety of rosiglitazone due to fluid retention led suspension in the European market and several restrictions in the US (Woodcock et al., 2010; Shukla and Kalra, 2011). Unlike rosiglitazone, pioglitazone did not show any risk of cardiovascular side effects. However, concerns were raised on the apparent risk of bladder cancer with pioglitazone and hence it is not recommended in people with active or prior history of bladder cancer (Shukla and Kalra, 2011). TZDs are associated with an average of 2–4 kg weight gain in the first year of management (Yki-Järvinen, 2004). In addition, these agents result in peripheral edema in 4–6% (Graham et al., 2010).
This systematic review identified four articles that assessed association between genetic variants in candidate genes and weight gain and/or oedema after treatment with TZDs (Kang et al., 2006; Ruaño et al., 2009; Bailey et al., 2010; Dawed et al., 2016) (Table 5). A post-hoc analysis from the DREAM (Diabetes REduction Assessment with ramipril and rosiglitazone Medication) trial that consist of 4,197 participants showed higher rate of roziglitazone-induced edema (OR = 1.89 [95% CI = 1.47–2.42], P = 0.017) in subjects homozygous for the C allele at rs6123045, a variant at the Nuclear Factor of Activated T-cells, Cytoplasmic, Calcineurin-Dependent 2 (NFATC2) locus (Bailey et al., 2010). We have previously showed association between the CYP2C8*3 variant with less weight gain compared to the wild type (P = 0.02) in the GoDARTS (Dawed et al., 2016). Another study by Ruaño et al. investigated 384 SNPs in 87 subjects treated with TZDs and reported significant association between an intronic SNP, rs903361, in Adenosine A1 Receptor (ADORA1) and BMI after correcting for multiple testing (Ruaño et al., 2009). The A allele at Perilipin 1, PLIN 11482G>A (rs894160), was also associated with less weight gain in Korean subjects treated with rosiglitazone compared to the G allele (Kang et al., 2006). Given these findings replicate in well-powered independent studies, we could potentially identify individuals who can benefit from the considerable therapeutic advantages of TZDs and who are least at risk for the side effects.
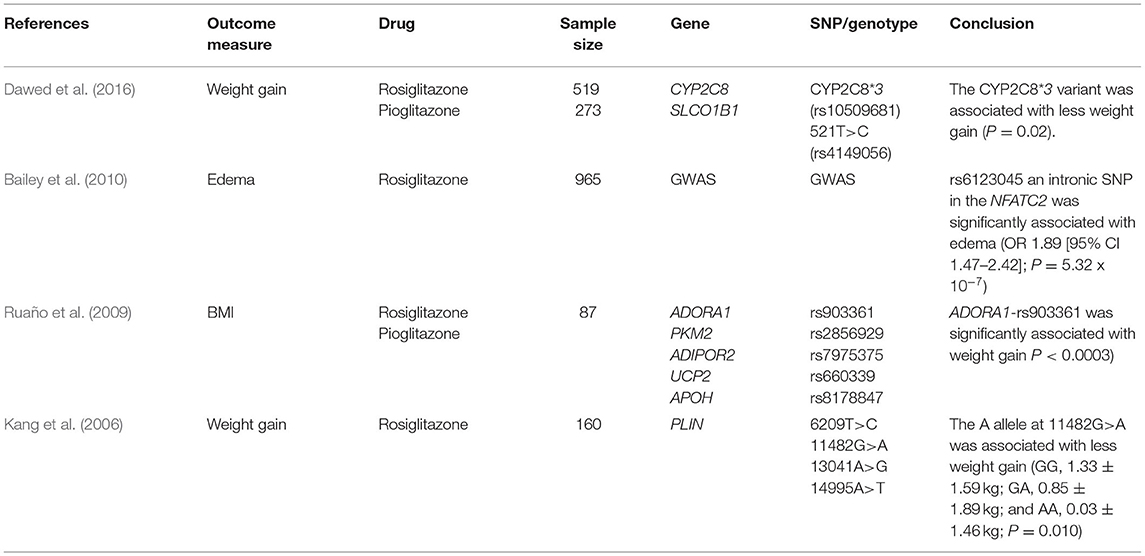
Table 5. Association between thiazolidinediones and selected SNPs for the incidence of weight gain and edema.
Discussion
This systematic review considers the adverse effects of glucose lowering drugs and their relationship to genetic variability. It presents the up-to-date knowledge of genetic variants that could influence ADRs related to drugs for the management of type 2 diabetes. Comprehensive understanding of genetic variants associated with ADRs have clinical utility in risk stratification of patients and precision therapeutics. Although numerous associations of genetic variants with ADR have been discovered, replication has proven difficult. This could mainly be due to a smaller sample which may not be sufficiently powered to detect the desired effect, the lack of consistent phenotypic definitions used, presence of possible drug-drug interactions, and related comorbidities.
This systematic review suggests that at present the clinical translation of genetic variants associated with ADR in diabetes therapy are limited.
Inter-ethnic differences in the susceptibility to ADRs and response to drugs are under-investigated. However, inter-ethnic differences have long been recognized as a crucial aspect of the genetics of variation in drug response. This could be due to differing background frequencies of risk alleles. For example, the frequency of 420del allele, that causes reduced OCT1 function and associated with higher odds of GI side effects of metformin, is much higher amongst European (~17%) than East Asian (~0.5%) or African (~6%) populations (Karczewski et al., 2020). The same is true for the loss of function variant, CYP2C9*3 (European ~6.7%, African ~1.3%, South Asian 1.2%) (Karczewski et al., 2020).
It is also likely that adverse drug reactions are polygenic. In polygenic effects, risk is conferred by combinations between several variants each of which could have small individual effects that are summarized as polygenic risk scores. We have previously shown a better prediction of metformin-induced GI intolerance by combining SNPs in the OCT1 (SLC22A1) and PMAT (SLC29A4) (Dawed et al., 2019), pioglitazone-related weight gain and SNPs in the SLCO1B1 and CYP2C9 (Dawed et al., 2016). Comprehensive studies encompassing data from hypothesis free genome-wide associations are required to identify susceptibility loci. In addition, next generation sequencing that allows the analysis of rare variants that have been postulated to have larger effects are likely to reveal functionally relevant genomic variations for ADRs.
Clinical, anthropometric, and environmental factors such as age, sex, weight, concomitant use of other drugs was also shown to contribute to ADRs in diabetes. Older people, women, and concomitant use of gut metformin transporter inhibiting drugs were previously shown to increase the likelihood of GI side effects of metformin (Dujic et al., 2015; Dawed et al., 2019). In addition, longer diabetes duration, impaired renal function, lower body mass index, lower triglyceride levels and old age were identified as major risk factors for hypoglycemia in people with type 2 diabetes (Schloot et al., 2016).
Even though this review is comprehensive, it is subjected to limitations. First, the studies included were heterogeneous in design with regards to treatment, adverse effect outcomes definitions, and population (ethnicity). The timing to measure primary endpoint (adverse effects of oral glucose-lowering agents) is also not uniform.
Poor adherence to treatment is a well-known phenomenon in patients with diabetes and is associated with inadequate glycaemic control leading to rapid disease progression and complications (Polonsky and Henry, 2016). Moderate and severe ADRs such as hypoglycemia and GI intolerance are previously shown to be key contributors of poor adherence in diabetes.
In conclusion, there are few pharmacogenomic studies of ADRs in type 2 diabetes that have been undertaken. Most of the studies have not been externally replicated, except OCT1 and metformin induced GI intolerance, CYP2C9 and SU-induced hypoglycemia. In the future, well-powered pharmacogenomic studies in T2D should collect standardized ADR data in multi-ethnic populations.
Data Availability Statement
The original contributions presented in the study are included in the article/Supplementary Material, further inquiries can be directed to the corresponding author.
Author Contributions
AMB and AYD conceived and designed this research, executed the analysis procedure, and analyzed the results. AMB, MKS, and AYD contributed to the writing of the manuscript. All authors reviewed the manuscript.
Funding
This work was partly supported by the internal Global Challenges Research Fund (GCRF) from the University of Dundee and the National Institute for Health Research (NIHR) using Official Development Assistance funding (INSPIRED 16/136/102).
Conflict of Interest
The authors declare that the research was conducted in the absence of any commercial or financial relationships that could be construed as a potential conflict of interest.
Supplementary Material
The Supplementary Material for this article can be found online at: https://www.frontiersin.org/articles/10.3389/fgene.2021.675053/full#supplementary-material
References
Aroda, V. R., Edelstein, S. L., Goldberg, R. B., Knowler, W. C., Marcovina, S. M., Orchard, T. J., et al. (2016). Long-term metformin use and vitamin b12 deficiency in the diabetes prevention program outcomes study. J. Clin. Endocrinol. Metab. 101, 1754–1761. doi: 10.1210/jc.2015-3754
Bailey, C. J., and Turner, R. C. (1996). Metformin. N. Engl. J. Med. 334, 574–579. doi: 10.1056/NEJM199602293340906
Bailey, S. D., Xie, C., Do, R., Montpetit, A., Diaz, R., Mohan, V., et al. (2010). Variation at the NFATC2 locus increases the risk of thiazolidinedione-induced edema in the Diabetes REduction Assessment with ramipril and rosiglitazone Medication (DREAM) study. Diabetes Care 33, 2250–2253. doi: 10.2337/dc10-0452
Becker, M. L., Visser, L. E., Trienekens, P. H., Hofman, A., van Schaik, R. H., and Stricker, B. H. (2008). Cytochrome P450 2C9 *2 and *3 polymorphisms and the dose and effect of sulfonylurea in type II diabetes mellitus. Clin. Pharmacol. Ther. 83, 288–292. doi: 10.1038/sj.clpt.6100273
Bell, D. S. (2010). Metformin-induced vitamin B12 deficiency presenting as a peripheral neuropathy. South. Med. J. 103, 265–267. doi: 10.1097/SMJ.0b013e3181ce0e4d
Buse, J. B., Wexler, D. J., Tsapas, A., Rossing, P., Mingrone, G., Mathieu, C., et al. (2020). 2019 update to: Management of hyperglycaemia in type 2 diabetes, 2018. A consensus report by the American Diabetes Association (ADA) and the European Association for the Study of Diabetes (EASD). Diabetologia 63, 221–228. doi: 10.1007/s00125-019-05039-w
Davies, E. C., Green, C. F., Mottram, D. R., Rowe, P. H., and Pirmohamed, M. (2010). Emergency re-admissions to hospital due to adverse drug reactions within 1 year of the index admission. Br. J. Clin. Pharmacol. 70, 749–755. doi: 10.1111/j.1365-2125.2010.03751.x
Davies, E. C., Green, C. F., Taylor, S., Williamson, P. R., Mottram, D. R., and Pirmohamed, M. (2009). Adverse drug reactions in hospital in-patients: a prospective analysis of 3695 patient-episodes. PLoS ONE 4:e4439. doi: 10.1371/journal.pone.0004439
Dawed, A. Y., Donnelly, L., Tavendale, R., Carr, F., Leese, G., Palmer, C. N., et al. (2016). CYP2C8 and SLCO1B1 variants and therapeutic response to thiazolidinediones in patients with type 2 diabetes. Diabetes Care 39, 1902–1908. doi: 10.2337/dc15-2464
Dawed, A. Y., Zhou, K., van Leeuwen, N., Mahajan, A., Robertson, N., Koivula, R., et al. (2019). Variation in the plasma membrane monoamine transporter (PMAT) (Encoded by SLC29A4) and organic cation transporter 1 (OCT1) (Encoded by SLC22A1) and gastrointestinal intolerance to metformin in type 2 diabetes: an IMI DIRECT study. Diabetes Care 42, 1027–1033. doi: 10.2337/dc18-2182
de Jager, J., Kooy, A., Lehert, P., Wulffelé M. G., van der Kolk, J., Bets, D., et al. (2010). Long term treatment with metformin in patients with type 2 diabetes and risk of vitamin B-12 deficiency: randomised placebo controlled trial. Bmj 340:c2181. doi: 10.1136/bmj.c2181
Donnelly, L. A., Dennis, J. M., Coleman, R. L., Sattar, N., Hattersley, A. T., Holman, R. R., et al. (2020). Risk of anemia with metformin use in type 2 diabetes: a MASTERMIND study. Diabetes Care 43, 2493–2499. doi: 10.2337/dc20-1104
Ducoffe, A. R., York, A., Hu, D. J., Perfetto, D., and Kerns, R. D. (2016). National action plan for adverse drug event prevention: recommendations for safer outpatient opioid use. Pain Med. 17, 2291–2304. doi: 10.1093/pm/pnw106
Dujic, T., Causevic, A., Bego, T., Malenica, M., Velija-Asimi, Z., Pearson, E. R., et al. (2016a). Organic cation transporter 1 variants and gastrointestinal side effects of metformin in patients with Type 2 diabetes. Diabet. Med. 33, 511–514. doi: 10.1111/dme.13040
Dujic, T., Zhou, K., Donnelly, L. A., Leese, G., Palmer, C. N. A., and Pearson, E. R. (2018). Interaction between variants in the CYP2C9 and POR genes and the risk of sulfonylurea-induced hypoglycaemia: a GoDARTS Study. Diabetes Obes. Metab. 20, 211–214. doi: 10.1111/dom.13046
Dujic, T., Zhou, K., Donnelly, L. A., Tavendale, R., Palmer, C. N., and Pearson, E. R. (2015). Association of organic cation transporter 1 with intolerance to metformin in type 2 diabetes: a GoDARTS study. Diabetes 64, 1786–1793. doi: 10.2337/db14-1388
Dujic, T., Zhou, K., Tavendale, R., Palmer, C. N., and Pearson, E. R. (2016b). Effect of serotonin transporter 5-HTTLPR polymorphism on gastrointestinal intolerance to metformin: a GoDARTS study. Diabetes Care 39, 1896–1901. doi: 10.2337/dc16-0706
Garber, A. J., Duncan, T. G., Goodman, A. M., Mills, D. J., and Rohlf, J. L. (1997). Efficacy of metformin in type II diabetes: results of a double-blind, placebo-controlled, dose-response trial. Am. J. Med. 103, 491–497. doi: 10.1016/S0002-9343(97)00254-4
Gökalp, O., Gunes, A., Cam, H., Cure, E., Aydin, O., Tamer, M. N., et al. (2011). Mild hypoglycaemic attacks induced by sulphonylureas related to CYP2C9, CYP2C19 and CYP2C8 polymorphisms in routine clinical setting. Eur. J. Clin. Pharmacol. 67, 1223–1229. doi: 10.1007/s00228-011-1078-4
Goodman, L. S. (2017). Goodman and Gilman's The Pharmacological Basis of Therapeutics, 13 Edn. New York, NY: McGraw-Hill.
Graham, D. J., Ouellet-Hellstrom, R., MaCurdy, T. E., Ali, F., Sholley, C., Worrall, C., et al. (2010). Risk of acute myocardial infarction, stroke, heart failure, and death in elderly Medicare patients treated with rosiglitazone or pioglitazone. Jama 304, 411–418. doi: 10.1001/jama.2010.920
Guardado-Mendoza, R., Prioletta, A., Jiménez-Ceja, L. M., Sosale, A., and Folli, F. (2013). The role of nateglinide and repaglinide, derivatives of meglitinide, in the treatment of type 2 diabetes mellitus. Arch. Med. Sci. 9, 936–943. doi: 10.5114/aoms.2013.34991
Han, T. K., Proctor, W. R., Costales, C. L., Cai, H., Everett, R. S., and Thakker, D. R. (2015). Four cation-selective transporters contribute to apical uptake and accumulation of metformin in Caco-2 cell monolayers. J. Pharmacol. Exp. Ther. 352, 519–528. doi: 10.1124/jpet.114.220350
Hébert, H. L., Shepherd, B., Milburn, K., Veluchamy, A., Meng, W., Carr, F., et al. (2018). Cohort profile: genetics of diabetes audit and research in Tayside Scotland (GoDARTS). Int. J. Epidemiol. 47:380-1j. doi: 10.1093/ije/dyx140
Hirst, J. A., Farmer, A. J., Ali, R., Roberts, N. W., and Stevens, R. J. (2012). Quantifying the effect of metformin treatment and dose on glycemic control. Diabetes Care 35, 446–454. doi: 10.2337/dc11-1465
Holstein, A., Beil, W., and Kovacs, P. (2012). CYP2C metabolism of oral antidiabetic drugs–impact on pharmacokinetics, drug interactions and pharmacogenetic aspects. Expert Opin. Drug Metab. Toxicol. 8, 1549–1563. doi: 10.1517/17425255.2012.722619
Holstein, A., Hahn, M., Patzer, O., Seeringer, A., Kovacs, P., and Stingl, J. (2011). Impact of clinical factors and CYP2C9 variants for the risk of severe sulfonylurea-induced hypoglycemia. Eur. J. Clin. Pharmacol. 67, 471–476. doi: 10.1007/s00228-010-0976-1
Holstein, A., Plaschke, A., Ptak, M., Egberts, E. H., El-Din, J., Brockmöller, J., et al. (2005). Association between CYP2C9 slow metabolizer genotypes and severe hypoglycaemia on medication with sulphonylurea hypoglycaemic agents. Br. J. Clin. Pharmacol. 60, 103–106. doi: 10.1111/j.1365-2125.2005.02379.x
Holstein, J. D., Kovacs, P., Patzer, O., Stumvoll, M., and Holstein, A. (2012). The Ser1369Ala variant of ABCC8 and the risk for severe sulfonylurea-induced hypoglycemia in German patients with Type 2 diabetes. Pharmacogenomics 13, 5–7; author reply 9–10. doi: 10.2217/pgs.11.150
International Diabetes Federation (2019). IDF Diabetes Atlas, 9th Edn. Brussels: International Diabetes Federation.
Inzucchi, S. E., Bergenstal, R. M., Buse, J. B., Diamant, M., Ferrannini, E., Nauck, M., et al. (2015). Management of hyperglycemia in type 2 diabetes, 2015: a patient-centered approach: update to a position statement of the American Diabetes Association and the European Association for the Study of Diabetes. Diabetes Care 38, 140–149. doi: 10.2337/dc14-2441
Jearath, V., Vashisht, R., Rustagi, V., Raina, S., and Sharma, R. (2016). Pioglitazone-induced congestive heart failure and pulmonary edema in a patient with preserved ejection fraction. J. Pharmacol. Pharmacother. 7, 41–43. doi: 10.4103/0976-500X.179363
Juurlink, D. N., Gomes, T., Lipscombe, L. L., Austin, P. C., Hux, J. E., and Mamdani, M. M. (2009). Adverse cardiovascular events during treatment with pioglitazone and rosiglitazone: population based cohort study. Bmj 339:b2942. doi: 10.1136/bmj.b2942
Kahn, S. E., Haffner, S. M., Heise, M. A., Herman, W. H., Holman, R. R., Jones, N. P., et al. (2006). Glycemic durability of rosiglitazone, metformin, or glyburide monotherapy. N. Engl. J. Med. 355, 2427–2443. doi: 10.1056/NEJMoa066224
Kang, E. S., Cha, B. S., Kim, H. J., Kim, H. J., Kim, S. H., Hur, K. Y., et al. (2006). The 11482G >A polymorphism in the perilipin gene is associated with weight gain with rosiglitazone treatment in type 2 diabetes. Diabetes Care 29, 1320–1324. doi: 10.2337/dc05-2466
Karczewski, K. J., Francioli, L. C., Tiao, G., Cummings, B. B., Alföldi, J., Wang, Q., et al. (2020). The mutational constraint spectrum quantified from variation in 141,456 humans. Nature 581, 434–443. doi: 10.1038/s41586-020-2308-7
Katzung, B. G., Kruidering-Hall, M., and Trevor, A. J. (2019). Katzung and Trevor's Pharmacology: Examination and Board Review, 12Edn. New York, NY: McGraw-Hill Companies, Incorporated.
Madsbad, S., Kielgast, U., Asmar, M., Deacon, C. F., Torekov, S. S., and Holst, J. J. (2011). An overview of once-weekly glucagon-like peptide-1 receptor agonists–available efficacy and safety data and perspectives for the future. Diabetes Obes. Metab. 13, 394–407. doi: 10.1111/j.1463-1326.2011.01357.x
Meneilly, G. S. (2011). Effect of repaglinide versus glyburide on postprandial glucose and insulin values in elderly patients with type 2 diabetes. Diabetes Technol. Ther. 13, 63–65. doi: 10.1089/dia.2010.0105
Misbin, R. I. (2004). The phantom of lactic acidosis due to metformin in patients with diabetes. Diabetes Care 27, 1791–1793. doi: 10.2337/diacare.27.7.1791
Pirmohamed, M., James, S., Meakin, S., Green, C., Scott, A. K., Walley, T. J., et al. (2004). Adverse drug reactions as cause of admission to hospital: prospective analysis of 18 820 patients. Bmj 32:15. doi: 10.1136/bmj.329.7456.15
Polonsky, W. H., and Henry, R. R. (2016). Poor medication adherence in type 2 diabetes: recognizing the scope of the problem and its key contributors. Patient Prefer. Adherence 10, 1299–1307. doi: 10.2147/PPA.S106821
Ragia, G., Petridis, I., Tavridou, A., Christakidis, D., and Manolopoulos, V. G. (2009). Presence of CYP2C9*3 allele increases risk for hypoglycemia in Type 2 diabetic patients treated with sulfonylureas. Pharmacogenomics 10, 1781–1787. doi: 10.2217/pgs.09.96
Ragia, G., Tavridou, A., Elens, L., Van Schaik, R. H., and Manolopoulos, V. G. (2014). CYP2C9*2 allele increases risk for hypoglycemia in POR*1/*1 type 2 diabetic patients treated with sulfonylureas. Exp. Clin. Endocrinol. Diabetes 122, 60–63. doi: 10.1055/s-0033-1361097
Ragia, G., Tavridou, A., Petridis, I., and Manolopoulos, V. G. (2012). Association of KCNJ11 E23K gene polymorphism with hypoglycemia in sulfonylurea-treated type 2 diabetic patients. Diabetes Res. Clin. Pract. 98, 119–124. doi: 10.1016/j.diabres.2012.04.017
Ruaño, G., Bernene, J., Windemuth, A., Bower, B., Wencker, D., Seip, R. L., et al. (2009). Physiogenomic comparison of edema and BMI in patients receiving rosiglitazone or pioglitazone. Clin. Chim. Acta 400, 48–55. doi: 10.1016/j.cca.2008.10.009
Salvo, F., Moore, N., Arnaud, M., Robinson, P., Raschi, E., De Ponti, F., et al. (2016). Addition of dipeptidyl peptidase-4 inhibitors to sulphonylureas and risk of hypoglycaemia: systematic review and meta-analysis. Bmj 353:i2231. doi: 10.1136/bmj.i2231
Sato, R., Watanabe, H., Genma, R., Takeuchi, M., Maekawa, M., and Nakamura, H. (2010). ABCC8 polymorphism (Ser1369Ala): influence on severe hypoglycemia due to sulfonylureas. Pharmacogenomics 11, 1743–1750. doi: 10.2217/pgs.10.135
Schloot, N. C., Haupt, A., Schütt, M., Badenhoop, K., Laimer, M., Nicolay, C., et al. (2016). Risk of severe hypoglycemia in sulfonylurea-treated patients from diabetes centers in Germany/Austria: How big is the problem? Which patients are at risk? Diabetes Metab. Res. Rev. 32, 316–324. doi: 10.1002/dmrr.2722
Schopman, J. E., Simon, A. C., Hoefnagel, S. J., Hoekstra, J. B., Scholten, R. J., and Holleman, F. (2014). The incidence of mild and severe hypoglycaemia in patients with type 2 diabetes mellitus treated with sulfonylureas: a systematic review and meta-analysis. Diabetes Metab. Res. Rev. 30, 11–22. doi: 10.1002/dmrr.2470
Semiz, S., Dujic, T., Ostanek, B., Prnjavorac, B., Bego, T., Malenica, M., et al. (2010). Analysis of CYP2C9*2, CYP2C19*2, and CYP2D6*4 polymorphisms in patients with type 2 diabetes mellitus. Bosn J. Basic Med. Sci. 10, 287–291. doi: 10.17305/bjbms.2010.2662
Shamseer, L., Moher, D., Clarke, M., Ghersi, D., Liberati, A., Petticrew, M., et al. (2015). Preferred reporting items for systematic review and meta-analysis protocols (PRISMA-P) 2015: elaboration and explanation. Bmj 350:g7647. doi: 10.1136/bmj.g7647
Shukla, R., and Kalra, S. (2011). Pioglitazone: Indian perspective. Indian J. Endocrinol. Metab. 15, 294–297. doi: 10.4103/2230-8210.85581
Spector, W. D., Limcangco, R., Furukawa, M. F., and Encinosa, W. E. (2017). The marginal costs of adverse drug events associated with exposures to anticoagulants and hypoglycemic agents during hospitalization. Med. Care 55, 856–863. doi: 10.1097/MLR.0000000000000780
Tarasova, L., Kalnina, I., Geldnere, K., Bumbure, A., Ritenberga, R., Nikitina-Zake, L., et al. (2012). Association of genetic variation in the organic cation transporters OCT1, OCT2 and multidrug and toxin extrusion 1 transporter protein genes with the gastrointestinal side effects and lower BMI in metformin-treated type 2 diabetes patients. Pharmacogenet. Genomics 22, 659–666. doi: 10.1097/FPC.0b013e3283561666
Winkelmayer, W. C., Setoguchi, S., Levin, R., and Solomon, D. H. (2008). Comparison of cardiovascular outcomes in elderly patients with diabetes who initiated rosiglitazone vs pioglitazone therapy. Arch. Intern. Med. 168, 2368–2375. doi: 10.1001/archinte.168.21.2368
Woodcock, J., Sharfstein, J. M., and Hamburg, M. (2010). Regulatory action on rosiglitazone by the U.S. Food and Drug Administration. N. Engl. J. Med. 363, 1489–1491. doi: 10.1056/NEJMp1010788
Keywords: pharmacogenomics, type 2 diabetes, adverse drug outcomes, oral glucose-lowering drugs, gastrointestinal side effects, hypoglycemia, weight gain
Citation: Baye AM, Fanta TG, Siddiqui MK and Dawed AY (2021) The Genetics of Adverse Drug Outcomes in Type 2 Diabetes: A Systematic Review. Front. Genet. 12:675053. doi: 10.3389/fgene.2021.675053
Received: 02 March 2021; Accepted: 21 May 2021;
Published: 14 June 2021.
Edited by:
Celine Verstuyft, Université Paris-Saclay, FranceReviewed by:
Zhiguo Xie, Central South University, ChinaVera Ribeiro, University of Algarve, Portugal
Copyright © 2021 Baye, Fanta, Siddiqui and Dawed. This is an open-access article distributed under the terms of the Creative Commons Attribution License (CC BY). The use, distribution or reproduction in other forums is permitted, provided the original author(s) and the copyright owner(s) are credited and that the original publication in this journal is cited, in accordance with accepted academic practice. No use, distribution or reproduction is permitted which does not comply with these terms.
*Correspondence: Adem Y. Dawed, a.y.dawed@dundee.ac.uk