- 1Department of Neurology, The First Affiliated Hospital of Zhengzhou University, Zhengzhou, China
- 2Henan Key Laboratory of Cerebrovascular Diseases, The First Affiliated Hospital of Zhengzhou University, Zhengzhou, China
- 3Clinical Systems Biology Laboratories, The First Affiliated Hospital of Zhengzhou University, Zhengzhou, China
Background: Homocysteine (Hcy) is a toxic amino acid and hyperhomocysteinemia (HHcy) was reported to be associated with both cerebrovascular disease and neurodegenerative disease. Our aim was to assess the causal link between plasma Hcy level and cerebrovascular and neurodegenerative diseases through a Mendelian randomization (MR) study.
Methods: A two-sample MR study was performed to infer the causal link. We extracted the genetic variants (SNPs) associated with plasma Hcy level from a large genome-wide association study (GWAS) meta-analysis. The main MR analysis was performed using the inverse variance-weighted method. Additional analyses were further performed using MR-Egger intercept and Cochran’s Q statistic to detect the heterogeneity or pleiotropy of our findings.
Results: Thirteen Hcy-associated SNPs were selected as instrumental variables. The results showed evidence of a causal link between plasma Hcy level and ischemic stroke (IS) caused by small artery occlusion (SAS, OR = 1.329, 95% CI 1.047–1.612, p = 0.048). Meanwhile, there was no evidence of association between plasma Hcy level and other types of IS, transient ischemic attack (TIA), or neurodegenerative disease. The MR-Egger intercept test indicated no evidence of directional pleiotropy. Results of additional MR analysis indicated that blood pressure (BP) and type 2 diabetes mellitus (T2DM) serve as influencers in the association.
Conclusion: The MR study found a little causal link between plasma Hcy level and SAS. The link is likely to be influenced by other risk factors like BP and T2DM.
Introduction
Homocysteine (Hcy) is a sulfur-containing toxic amino acid that is harmful to the body or cells (Eikelboom et al., 1999; Faraci and Lentz, 2004). Hcy has been shown to induce endothelial dysfunction by DNA damage, oxidative stress, and promotion of coagulation (Hankey and Eikelboom, 2001). In addition, hyperhomocysteinemia (HHcy) also adversely affects vascular smooth muscle cells, leading to their proliferation (Mujumdar et al., 2000). Therefore, the toxicity of Hcy is considered a cause of vascular alterations and atherosclerosis. Hcy has been regarded as a risk factor for cardiovascular disease since 1969 (McCully, 1969; Eikelboom et al., 1999). However, lowering Hcy medications, including folate and B vitamin supplementation, remains limited as most clinical trials have shown conflicting results (Huang et al., 2012). Although studies have reported that lowering Hcy may reduce the risk of stroke (Martí-Carvajal et al., 2017), the utilization of lowering Hcy medication is not well determined based on the subtypes of ischemic stroke (IS), as demonstrated by The Trial of Org 10,172 in Acute Stroke Treatment (TOAST), including large artery atherosclerosis (LAS), cardioembolism (CES), and small artery occlusion (SAS; Adams et al., 1993).
In addition to cerebrovascular disease, HHcy was reported to be associated with other neurological disorders, especially neurodegenerative disease (Mattson and Shea, 2003; Dubchenko et al., 2020). Neurodegeneration is characterized by neuronal degeneration and apoptosis. Previous studies have reported the association of HHcy and Alzheimer’s disease (AD), Parkinson’s disease (PD), and amyotrophic lateral sclerosis (ALS; Seshadri et al., 2002; Wang and Fan, 2012; Fan et al., 2020). In addition, vascular origin was mentioned as a potential mechanism of neurodegeneration and demyelination (Kalaria et al., 2012; Zivadinov et al., 2012; Yamazaki and Kanekiyo, 2017). It is still unclear whether HHcy is the cause or merely a phenomenon accompanying cardiovascular disease.
Because of sampling errors, causal links between plasma Hcy level and risk of cerebrovascular or neurodegenerative disease cannot be found by observational studies. Mendelian randomization (MR) is a powerful tool for analyzing the causality of exposure factors and certain disorder, which utilizes genetic variations (i.e., SNPs, single nucleotide polymorphisms) as instrumental variables (IVs; Lawlor et al., 2008; Sekula et al., 2016). Thus, an MR study can overcome the limitations of observational studies such as confounding and reverse causation (Latvala and Ollikainen, 2016). Previous studies have investigated the causal link between Hcy and cardiovascular disease or AD, but few focused on the subtypes of IS or other neurodegenerative disorders (Casas et al., 2005; Larsson et al., 2017; Miao et al., 2019).
The aim of our study was to evaluate the causal association between plasma Hcy level and cerebrovascular disease, including IS (LAS, CES, SAS, and nonsubtyped) and transient ischemic attack (TIA), or neurodegenerative disease, including MS, AD, PD, ALS, and frontotemporal dementia (FTD) through a two-sample MR study.
Materials and Methods
Data Sources
All the genetic variants associated with plasma Hcy level were acquired from a large genome-wide association study (GWAS) meta-analysis with 44,147 subjects of European ancestry (van Meurs et al., 2013). For the cerebrovascular disease dataset, we obtained the corresponding genetic variants from the MEGASTROKE consortium (Malik et al., 2018). Their dataset included 440,328 subjects and 34,217 cases, which could be further divided into LAA (n = 4,373), CE (n = 7,193), small artery occlusion (SAO; n = 5,386), and nonsubtyped cases (n = 17,265; Malik et al., 2018). We obtained corresponding genetic variants with TIA from the UK Biobank, including 1,364 cases with TIA and 461,646 controls (Sudlow et al., 2015). For datasets of neurodegenerative disease, we obtained the corresponding genetic variants from the International Multiple Sclerosis Genetics Consortium (IMSGC) including 14,498 cases with MS and 24,091 controls [International Multiple Sclerosis Genetics Consortium (IMSGC) et al., 2013], the International Genomics of Alzheimer’s Project including 21,982 cases with AD and 41,944 controls (Kunkle et al., 2019), the International Parkinson’s Disease Genomics Consortium including 33,674 cases with PD and 449,056 controls (Nalls et al., 2019), the International Amyotrophic Lateral Sclerosis Genomics Consortium including 20,806 cases with ALS and 59,804 controls (Nicolas et al., 2018), and the International Frontotemporal Lobar Degeneration Collaboration including 515 cases with FTD and 2,509 controls (Van Deerlin et al., 2010). The subjects from both exposure and outcome datasets included in our study were of European ancestry. Ethics approval was not required as it was a secondary analysis of previously published data.
Study Design
A two-sample MR study was performed to investigate the potential causal impact of plasma Hcy level on the risk of cerebrovascular and neurodegenerative diseases. The MR study is established by three major assumptions (Emdin et al., 2017; Figure 1). First, the IVs are directly associated with the exposure (plasma Hcy level) with genome-wide significance. Second, there is no link between the IVs and the confounding factors. Lastly, the IVs affect the outcome merely through exposure.
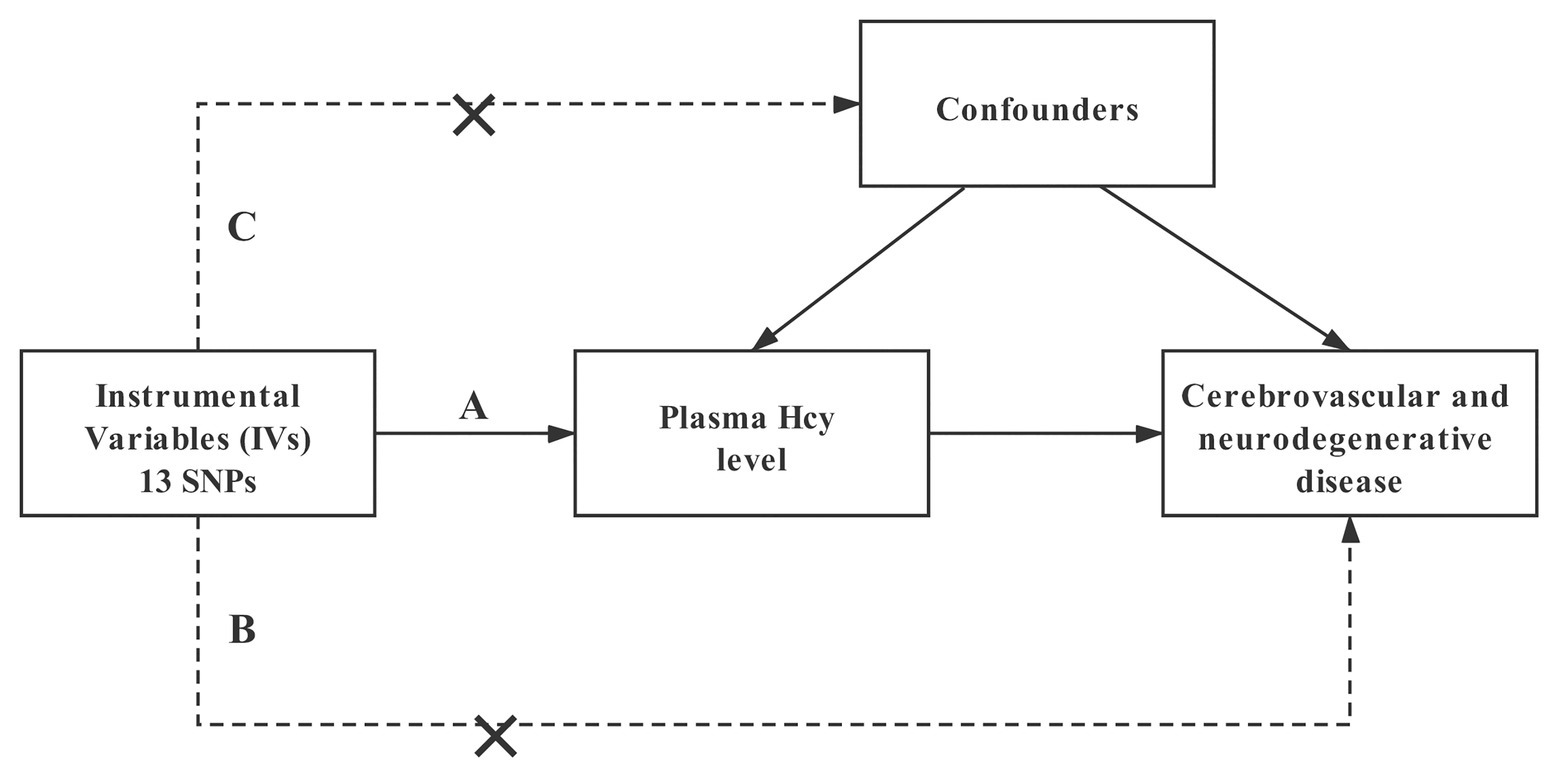
Figure 1. Design and main assumptions of our Mendelian randomization study. SNPs, single nucleotide polymorphisms; Hcy, homocysteine.
SNP Selection and Validation
We have selected genome-wide significant (p < 5 × 10−8) genetic variants (SNPs) associated with plasma Hcy level from a large GWAS meta-analysis as IVs (van Meurs et al., 2013). There were 18 SNPs in total, after testing for linkage disequilibrium: 5 SNPs (rs7422339, rs12134663, rs957140, rs12921383, and rs2851391) with r2 greater than 0.01 in the European samples of 1,000 Genomes were excluded and finally 13 SNPs remained (Supplementary Table S1). The 13 unique SNPs explained 5.9% of the variation in plasma Hcy level (van Meurs et al., 2013). Then, we have assessed the F-statistic of the selected IVs that was approximately 212.85, indicating strong instruments for our following MR study (Burgess et al., 2011). If the specified SNP was not available in the outcome dataset, we used a proxy SNP in linkage disequilibrium (r2 > 0.9) with the specified SNP. For the outcome dataset of TIA, MS, and FTD, there were no corresponding SNPs from the dataset, and thus, we would select a proxy variant with the existence of high linkage disequilibrium for further MR analysis.
Statistical Analysis
MR analysis was conducted in R (version 4.0.2) by the TwoSampleMR package (Hemani et al., 2018). The main analysis was performed by random-effects inverse variance-weighted (IVW) analysis (Hemani et al., 2018). We also performed fixed-effects IVW analysis, maximum likelihood analysis, simple median analysis, MR-Egger analysis, weighted median analysis, simple mode analysis, and weighted mode analysis as additional analyses (Bowden et al., 2015, 2016; Hartwig et al., 2017). Then, sensitivity tests were conducted using the heterogeneity test, pleiotropy test, and leave-one-out sensitivity test. Cochran’s Q test was calculated to assess the degree of heterogeneity across the individual effect estimates derived from every genetic variant. The MR-Egger intercept test was conducted to assess the horizontal pleiotropy and a funnel plot was plotted to provide a visual inspection (Bowden et al., 2015). Leave-one-out sensitivity analysis was performed to measure if the pooled estimate is being disproportionately influenced by each genetic variant. We used a Bonferroni correction [corrected p = 0.05/1 (traits considered)/10 (exposures) = 0.005] to account for multiple comparisons.
In the leave-one-out analysis of SAS (Supplementary Figure S1), we found six IVs (rs154657, rs7130284, rs234709, rs42648, rs1801222, and rs2275565) which had a greater impact on the result. In addition, by the forest plot (Supplementary Figure S2), we found three potential risk SNPs (rs9369898, rs548987, and rs1801133) for SAS through the impact on plasma Hcy level. Therefore, we performed an additional MR analysis for the three and seven SNPs (in addition to the six SNPs mentioned above) and SAS.
The other additional MR analysis was first performed to find the 13 SNPs which showed significant differences for the known risk factors of IS (here considered as potential pleiotropic SNPs), including body mass index (BMI; Hoffmann et al., 2018), years of schooling (Lee et al., 2018), numbers of moderate physical activity, alcohol consumption (Clarke et al., 2017), current smoking, high-density lipoprotein cholesterol (HDL; Willer et al., 2013), low-density lipoprotein cholesterol (LDL; Willer et al., 2013), total cholesterol (TC; Kettunen et al., 2016), triglycerides (TG; Willer et al., 2013), pulse rate (PR), systolic blood pressure (SBP; Evangelou et al., 2018), diastolic blood pressure (DBP; Evangelou et al., 2018), atrial fibrillation (AF; Nielsen et al., 2018), coronary heart disease (CHD; Nikpay et al., 2015), fasting blood glucose (FBG; Manning et al., 2012), and type 2 diabetes mellitus (T2DM; Bonàs-Guarch et al., 2018), respectively. Then we excluded the potential pleiotropic SNPs we found in the last step in each analysis, and the respective SNPs with no significant differences were included in the further additional MR analysis between plasma Hcy level and SAS. The methods of all additional MR analysis were random-effects IVW method.
Results
Plasma Hcy Level Was Associated With Ischemic Stroke Caused by Small Artery Occlusion
For cerebrovascular disease, we have included 13 genetic variants in most studies in addition to TIA (10 SNPs in addition to rs7422339, rs7130284, and rs548987). The result of the main MR analysis is shown in Table 1 and Figure 2. We found a causal link between plasma Hcy level and SAS (OR = 1.329, 95% CI 1.002–1.763, p = 0.048) but no causal link on TIA (OR = 1.000, 95% CI 0.999–1.001, p = 0.858) or LAS (OR = 1.093, 95% CI 0.878–1.312, p = 0.424), CES (OR = 0.920, 95% CI 0.785–1.080, p = 0.308), and nonsubtyped (OR = 1.098, 95% CI 0.980–1.229, p = 0.107). However, the causal link was not strong after multiple comparisons (corrected p = 0.005).
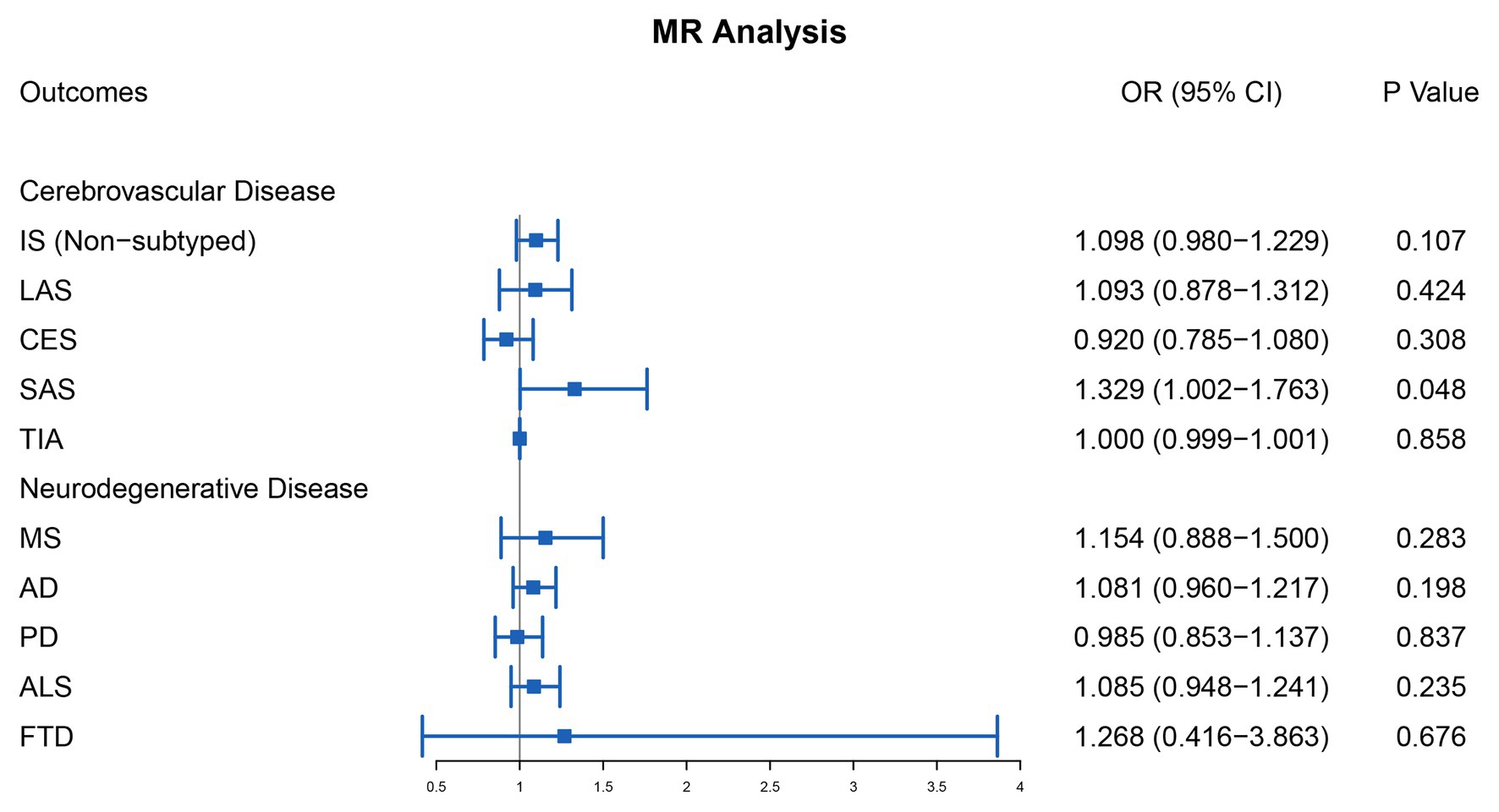
Figure 2. Mendelian randomization analysis of the association between plasma Hcy level and cerebrovascular and neurodegenerative diseases. OR, odds ratio; CI, confidential interval; IS, ischemic stroke; LAS, large artery atherosclerosis stroke; CES, cardioembolism stroke; SAS, small artery occlusion stroke; TIA, transient ischemic attack; MS, multiple sclerosis; AD, Alzheimer’s disease; PD, Parkinson’s disease; ALS, amyotrophic lateral sclerosis; FTD, frontotemporal dementia.
In the sensitivity analysis (Supplementary Table S2), our result indicated strong heterogeneities of the genetic variants for SAS (p = 0.011) and nonsubtyped IS (p = 0.042). We have not found any heterogeneities of plasma Hcy level and LAS (p = 0.291), CES (p = 0.301), and TIA (p = 0.416). By leave-one-out analysis (Supplementary Figure S1), we found that six SNPs (rs154657, rs7130284, rs234709, rs42648, rs1801222, and rs2275565) may affect the link.
Through the MR-Egger analysis (Supplementary Table S2), there was no evidence of pleiotropy SAS (MR-Egger intercept = 0.007, 95% CI 0.038–0.053, p = 0.758). Also, there was no evidence of pleiotropy for LAS (MR-Egger intercept = 0.001, 95% CI 0.034–0.037, p = 0.953), CES (MR-Egger intercept = −0.020, 95% CI 0.043–0.003, p = 0.110), and nonsubtyped IS (MR-Egger intercept = −0.004, 95% CI −0.022 to 0.022, p = 0.680) and TIA (MR-Egger intercept = 0.0001, 95% CI −3.91 × 10−5 to 2.65 × 10−4, p = 0.183).
As shown in Figure 3, the additional analysis indicated that a causal link between plasma Hcy level and SAS was observed using 3-SNPs (OR = 1.849, 95% CI 1.364–2.506, p = 7.48 × 10−5) and 7-SNPs (OR = 1.774, 95% CI 1.426–2.206, p = 2.59 × 10−7). Moreover, the causal link was still strong after multiple comparisons by Bonferroni correction (corrected value of p for 3-SNPs: 0.017 and corrected value of p for 7-SNPs: 0.007). In the sensitivity analyses (Supplementary Table S2), there was no evidence of significant associations observed for both 3-SNPs (p = 0.127) and 7-SNPs (p = 0.224), and there was no evidence of pleiotropy for IS caused by SAS (3-SNPs: MR-Egger intercept = 0.044, 95% CI 0.002–0.086, p = 0.291; 7-SNPs: MR-Egger intercept = 0.012, 95% CI −0.022 to 0.045, p = 0.529). Leave-one-out analysis showed that the causal link between plasma Hcy level and SAS was not substantially driven by any individual SNP (Supplementary Figure S3).
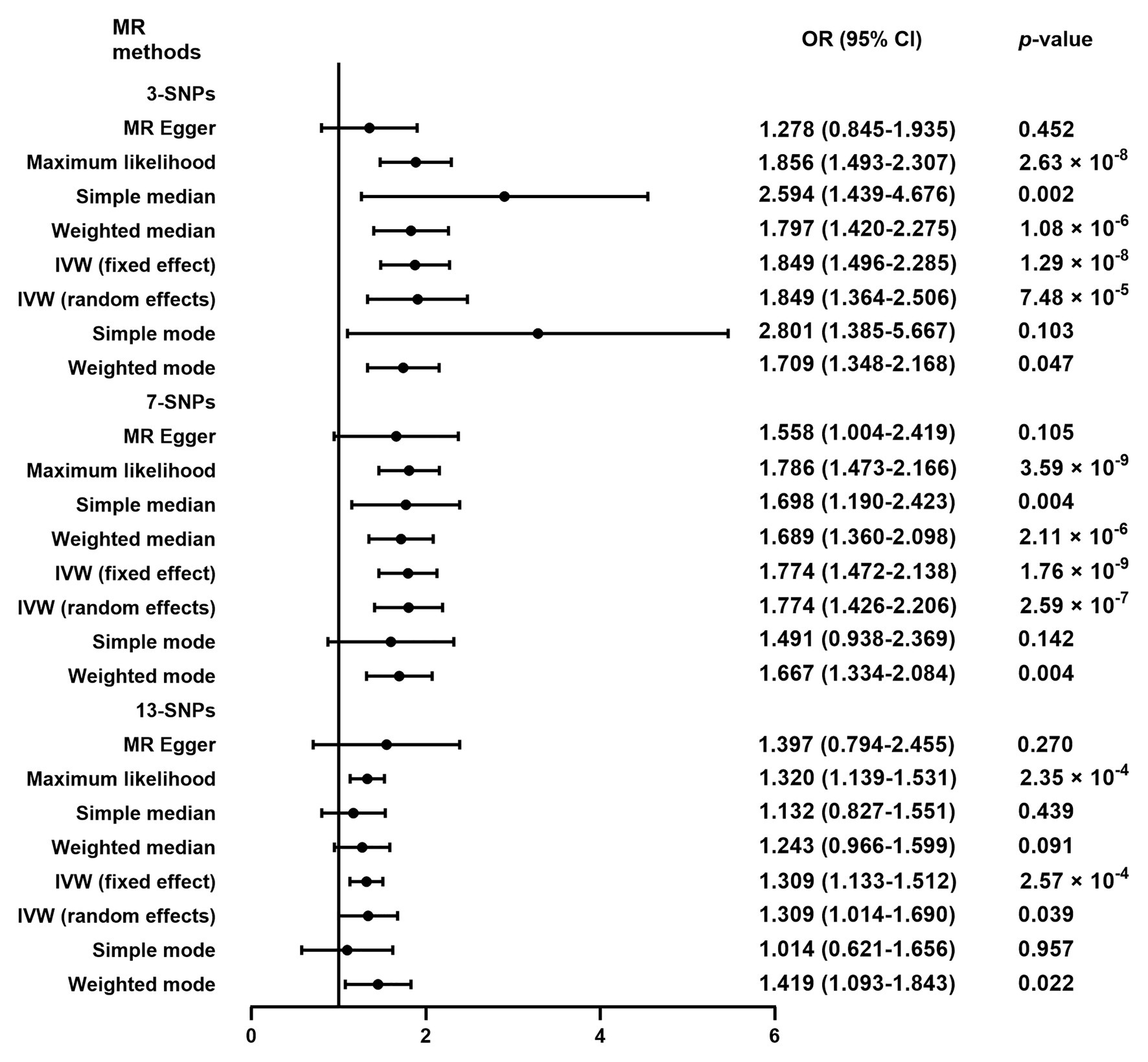
Figure 3. Mendelian randomization analysis of plasma Hcy level on the risk of ischemic stroke caused by small artery occlusion. OR, odds ratio; CI, confidential interval; MR, Mendelian randomization; SNPs, single nucleotide polymorphisms; IVW, inverse variance-weighted. 3-SNPs: rs1801133, rs9369898, and rs548987. 7-SNPs: rs1801133, rs9369898, rs548987, rs4660306, rs2251468, rs838133, and rs12780845. 13-SNPs: rs1801133, rs9369898, rs548987, rs4660306, rs2251468, rs838133, rs12780845, rs2275565, rs7130284, rs154657, rs234709, rs42648, and rs1801222.
To analyze the causal link between Hcy and SAS deeply, first, we performed two-sample MR analysis of plasma Hcy level and the known risk factors for IS (including BMI, years of schooling, etc.), respectively, to find out the SNP with significant differences, which were considered as potential pleiotropic SNP (Supplementary Table S3). We then excluded potential pleiotropic SNPs and included SNPs with no significant differences. The results indicated that after the multipotent SNPs were excluded, SBP (OR = 1.218, 95% CI 0.685–1.750, p = 0.469), DBP (OR = 0.949, 95% CI 0.675–1.223, p = 0.706), and T2DM (OR = 1.071, 95% CI 0.748–1.395, p = 0.677) were potential influencers of the link between Hcy and SAS (Figure 4).
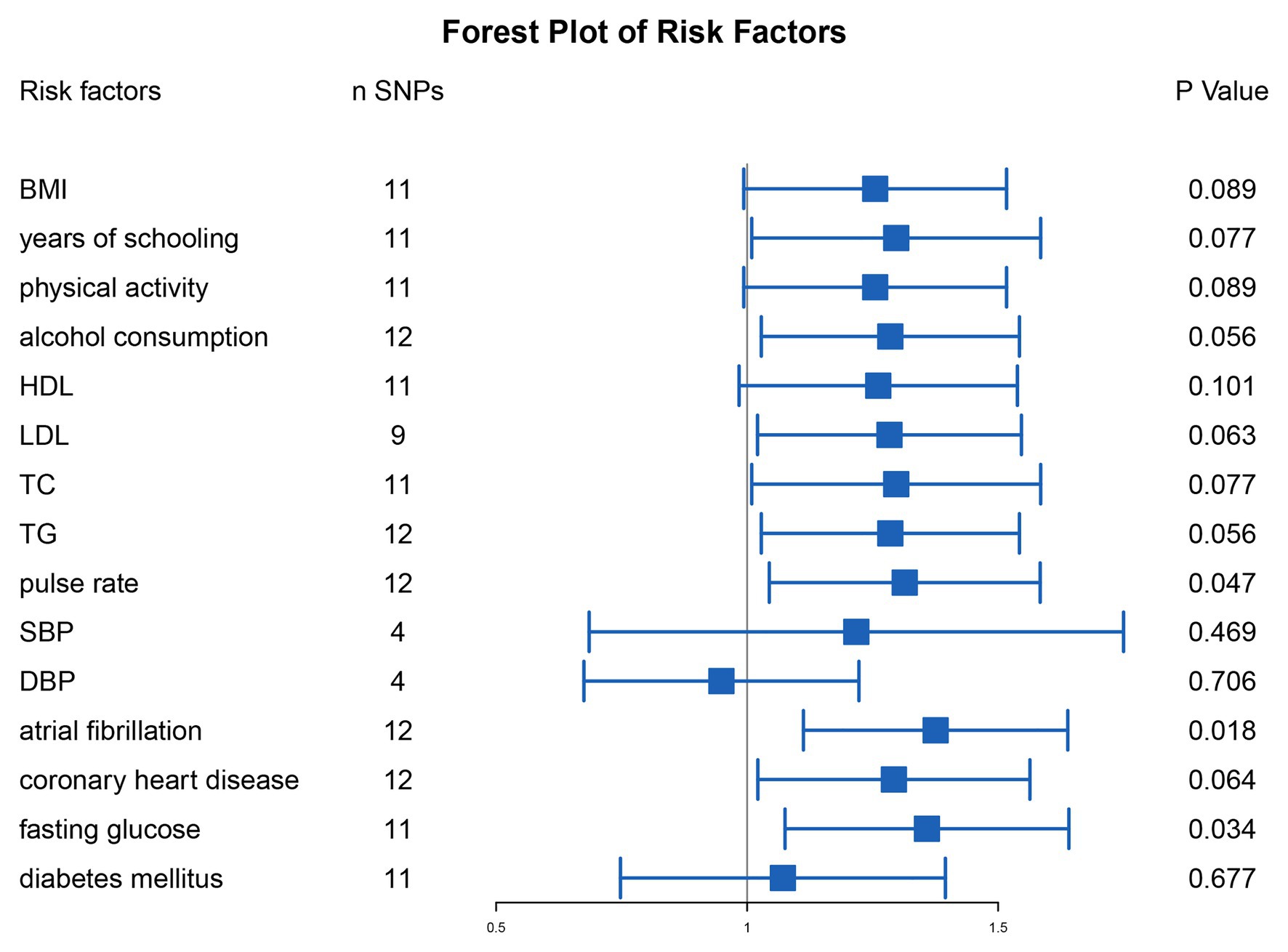
Figure 4. Mendelian randomization analysis of plasma Hcy level on the risk of ischemic stroke caused by small artery occlusion after exclusion for the SNPs with significant differences of the known risk factors of ischemic stroke. SNPs, single nucleotide polymorphisms; BMI, body mass index; HDL, high-density lipoprotein cholesterol; LDL, low-density lipoprotein cholesterol; TC, total cholesterol; TG, triglycerides; SBP, systolic blood pressure; DBP, diastolic blood pressure.
Plasma Hcy Level Was Not Associated With Neurodegenerative Disease
For neurodegenerative disease, 13 genetic variants were included in most studies in addition to MS (6 SNPs, namely rs2275565, rs548987, rs7130284, rs154657, rs22251468, and rs12780845) and FTD (6 SNPs, namely rs9369898, rs154657, rs42648, rs12780845, rs4660306, and rs7130284). The outcomes of the main MR analysis are shown in Table 2 and Figure 2. We have not identified a significant causal link between plasma Hcy level and MS (OR = 1.154, 95% CI 0.888–1.500, p = 0.283), AD (OR = 1.081, 95% CI 0.960–1.217, p = 0.198), PD (OR = 0.985, 95% CI 0.853–1.137, p = 0.837), ALS (OR = 1.085, 95% CI 0.948–1.241, p = 0.235), and FTD (OR = 1.268, 95% CI 0.416–3.863, p = 0.676). By forest plot (Supplementary Figure S2), we neither have not found any SNP as a risk factor for neurodegenerative disease.
As shown in Supplementary Table S2, the sensitivity analysis indicated no heterogeneities among individual SNPs (MS: p = 0.236; AD: p = 0.857; PD: p = 0.856; ALS: p = 0.129; FTD: p = 0.213), and there was no evidence of pleiotropy for the outcomes of neurodegenerative disease (MS: MR-Egger intercept = −0.0002, 95% CI −0.065 to 0.065, p = 0.996; AD: MR-Egger intercept = −0.005, 95% CI −0.024 to 0.013, p = 0.593; PD: MR-Egger intercept = −0.016, 95% CI −0.038 to 0.007, p = 0.206; ALS: MR-Egger intercept = −0.021, 95% CI −0.040 to −0.002, p = 0.053; FTD: MR-Egger intercept = 0.014, 95% CI −0.188 to 0.217, p = 0.897). The leave-one-out sensitivity analysis suggested that all MR analysis results for neurodegenerative disease were not driven dramatically by any single SNP (Supplementary Figure S1).
Discussion
In our two-sample MR study, there was little evidence of the causal link between high plasma Hcy and high risk of SAS. However, after multiple comparisons, no statistically significant impact was found. Additional analysis showed strong evidence by both 3-SNP and 7-SNP methods. We also found that SBP, DBP, and T2DM were potential influencers in the link between Hcy and SAS. For other types of IS, genetically higher plasma Hcy level was not associated with higher risk of LAS, CES, and nonsubtyped. In addition, our results showed no causal links between plasma Hcy level and neurodegenerative disease.
Previous studies have reported the link between HHcy and atherosclerotic vascular disease and neurodegenerative disease (Hankey and Eikelboom, 2001; Morris, 2003; Zhang et al., 2018). Some studies demonstrated that Hcy contributed to endothelial dysfunction by oxidative stress, DNA damage, and apoptosis (Currò et al., 2014). Hcy could induce an inflammatory environment by upregulating inflammatory factors, including C-reactive protein and intracellular adhesion molecule-1 (Durga et al., 2005). Because of the high susceptibility to disease of cerebral small vessels, the harmful effect of Hcy can be more obvious (Wardlaw et al., 2019).
Epidemiologic observational studies have demonstrated Hcy as a risk factor for cardiovascular disease. A meta-analysis including 10 studies detected a significant dose–response association of Hcy with the risk of IS (Wu et al., 2020). Previous studies also reported that HHcy was associated with microbleeds and leukoaraiosis (Feng et al., 2013; Yoo et al., 2020). By profiling the genetic variant MTHFR C677T among IS with different origins, Rutten-Jacobs et al. (2016) reported that MTHFR C677T was associated with lacune and higher white hyperintensity, but not LAS or CES, which partly explained the uncertainty about the efficacy of lowering Hcy treatment for stroke patients. A meta-analysis including 13 case–control studies of Chinese patients reported that all subtypes of IS had higher plasma Hcy than healthy controls (Zhang et al., 2020). Previous MR studies have reported the association between Hcy and cardiovascular disease. Casas et al. found a causal link between Hcy and IS by profiling MTHFR C677T polymorphism (Casas et al., 2005). MTHFR gene mutations were associated with lacunes and cerebral atrophy, too (Cao et al., 2020). An MR study investigating the level of Hcy, folate, and B vitamins and IS or CHD reported a similar result (Larsson et al., 2019). Our main analysis had a consistent result. A possible mechanism of the link between Hcy and SAS was the small vessels’ higher sensitivity to stimulus like high pressure and oxidative stress (Wardlaw et al., 2019). In addition, HHcy was associated with endothelial dysfunction, thus leading to blood–brain barrier (BBB) dysfunction and even disruption (Nam et al., 2019). The disruption of the BBB and chronic hypoperfusion finally cause the development of white matter hyperintensity, cerebral microbleeds, and enlarged perivascular space (Nam et al., 2019). We found that, after multiple comparisons, the effect was not strong. But through an additional analysis using 3-SNP and 7-SNP methods, even after the correction, the effect was still strong. Thus, some candidate risk genes, like MTHFR, which provided a novel monogenic cause of SAS, still require further investigation, and the mechanism needs to be further clarified.
Another main concern is the use of lowering Hcy medication. HHcy was relatively common in atherosclerotic cardiovascular disease patients. A meta-analysis demonstrated a linear inverse link between dietary intake of Hcy metabolism-related B vitamins (Chen et al., 2020). However, the rate of use of folate or B vitamin supplementation for lowering Hcy was not as high as expected partly due to the controversial results of previous clinical trials. The VITAmins TO Prevent Stroke (VITATOPS) trial indicated that B vitamin supplementation was a protective factor for SAS but not for other subtypes (VITATOPS Trial Study Group, 2010), and a substudy of VITATOPS showed that a 2-year B vitamin medication could significantly reduce the volume of white matter hyperintensities (Cavalieri et al., 2012). Besides, the China Stroke Primary Prevention Trial concluded that folic acid supplementation could significantly reduce the risk of first stroke among subjects with hypertension (Huo et al., 2015). Therefore, lowering Hcy treatment may benefit individuals with specific origins of cardiovascular disease. For neurodegeneration, most clinical trials obtained negative results of lowering Hcy treatment (Chen et al., 2016; Kwok et al., 2020). Here, we performed an additional analysis by excluding the potential pleiotropic SNPs, and our results indicated that the link between genetically high Hcy and SAS could be influenced by the multipotent capacity of the Hcy-related genetic variants, particularly by the effect on blood pressure and T2DM for the first time by the MR method. The effect may originate from both horizontal and vertical pleiotropy of the genetic variants. Perhaps the genetic variants associated with Hcy influence blood pressure and T2DM or Hcy affects the risk of SAS through blood pressure and T2DM. This effect can affect the occurrence of SAS and the therapeutic efficacy of lowering Hcy. SAS, T2DM, and hypertension all show small vessel lesions and the long-term metabolic disturbance is associated with dysfunction of the endothelium and activation and irritation of the inflammatory environment, which were potential mechanisms of SAS. Therefore, lowering Hcy treatments could be considered for subjects with HHcy and metabolic syndrome including hypertension and T2DM. Moreover, clinical trials will focus on the diversity and accuracy of lowering Hcy treatment and find out the population who benefit from it.
In addition to the adverse effect on vasculature, previous studies have shown that HHcy was associated with neurodegeneration, so we tried to find the link between Hcy and neurodegeneration. The toxicity of Hcy on neurons may contribute to the accumulation of β-amyloid, calcium influx, and apoptosis of neurons, making subjects more prone to develop dementia (Obeid and Herrmann, 2006). Despite several studies reporting the links between Hcy and neurodegenerative disease (Ostrakhovitch and Tabibzadeh, 2019), we have not found any evidence of the link between Hcy and AD from our results, which was consistent with a previous MR study (Larsson et al., 2017). We also found no causal link between Hcy and other neurodegenerative disease, including PD, ALS, MS, and FTD. Therefore, the common pathogenesis of SAS and dementia remains to be validated and investigated.
Our MR study still has some limitations. First of all, one major limitation of MR is the bias because of pleiotropy, indicating one genetic variant influences various phenotypes. Moreover, we can hardly exclude that all the SNPs in our study probably had an impact on the risk of cerebrovascular or neurodegenerative disease through other mechanisms except for influencing plasma Hcy level. Despite that we have not found any evidence of pleiotropy in the MR-Egger intercept analysis, this result may be hindered by a relatively low number of SNPs, and a low number of SNPs may overestimate the effect of exposure on the outcome. Therefore, more SNPs associated with plasma Hcy level and exposures including IS need to be identified in studies with a larger sample size with higher resolution. Besides, some risk factors for SAS, including hypertension, dyslipidemia, diabetes mellitus, smoking, and obesity, are risk factors for other subtypes of stroke as well. Thus, such risk factors may partially influence plasma Hcy level or contribute to the effect of Hcy on SAS via other mechanisms. Despite having excluded the SNPs with such properties in an additional analysis, fewer SNPs were included and a multivariable MR analysis may present a more meaningful suggestion. Finally, our MR study was originated from subjects with European ancestry, so population stratification inevitably existed. As a result, the conclusion is not supposed to be generalized to other ethnicities around the globe.
Conclusion
Through a two-sample MR study, we found that there was a causal link between plasma Hcy level and SAS. Another additional analysis indicated that SBP, DBP, and T2DM serve as influencers in this association. However, no causal links were identified between Hcy and other subtypes of IS, TIA, or neurodegenerative disease. For the prevention of IS, patients with T2DM or hypertension may benefit more from lowering Hcy treatment. Revealing the underlying common pathways of HHcy, hypertension, T2DM, and SAS would be of importance.
Data Availability Statement
The original contributions presented in the study are included in the article/Supplementary Material, further inquiries can be directed to the corresponding authors.
Ethics Statement
Written informed consent was obtained from the individual(s) for the publication of any potentially identifiable images or data included in this article.
Author Contributions
ZX and YX conceived and designed the study. SL, CL, and YT collected the data. WL and LZ analyzed the data. BS, ZX, and YX were involved in the supervision of the study. HF, RZ, and BS were involved in the interpretation of the data. WL drafted the manuscript. All authors contributed to the article and approved the submitted version.
Funding
This work was funded by the National Natural Science Foundation of China to YX (81530037 and 91849115) and ZX (31970158).
Conflict of Interest
The authors declare that the research was conducted in the absence of any commercial or financial relationships that could be construed as a potential conflict of interest.The reviewer YP declared a past co-authorship with the authors HF, BS and YX to the handling editor.
Acknowledgments
We gratefully thanked van Meurs et al. (2013), the MEGASTROKE consortium, UK Biobank, the International Multiple Sclerosis Genetics Consortium, the International Genomics of Alzheimer’s Project, the International Parkinson’s Disease Genomics Consortium, the International Amyotrophic Lateral Sclerosis Genomics Consortium, the International Frontotemporal Lobar Degeneration Collaboration, Hoffmann et al. (2018), Manning et al. (2012), the Global Lipids Genetics Consortium, Kettunen et al. (2016), Bonàs-Guarch et al. (2018), Clarke et al. (2017), Nikpay et al. (2015), the International Consortium of Blood Pressure, and Nielsen et al. (2018) for providing statistical data.
Supplementary Material
The Supplementary Material for this article can be found online at: https://www.frontiersin.org/articles/10.3389/fgene.2021.653032/full#supplementary-material
References
Adams, H. P., Bendixen, B. H., Kappelle, L. J., Biller, J., Love, B. B., Gordon, D. L., et al. (1993). Classification of subtype of acute ischemic stroke. Definitions for use in a multicenter clinical trial. TOAST. Trial of org 10172 in acute stroke treatment. Stroke 24, 35–41. doi: 10.1161/01.STR.24.1.35
Bonàs-Guarch, S., Guindo-Martínez, M., Miguel-Escalada, I., Grarup, N., Sebastian, D., Rodriguez-Fos, E., et al. (2018). Re-analysis of public genetic data reveals a rare X-chromosomal variant associated with type 2 diabetes. Nat. Commun. 9:321. doi: 10.1038/s41467-017-02380-9
Bowden, J., Davey Smith, G., and Burgess, S. (2015). Mendelian randomization with invalid instruments: effect estimation and bias detection through Egger regression. Int. J. Epidemiol. 44, 512–525. doi: 10.1093/ije/dyv080
Bowden, J., Davey Smith, G., Haycock, P. C., and Burgess, S. (2016). Consistent estimation in Mendelian randomization with some invalid instruments using a weighted median estimator. Genet. Epidemiol. 40, 304–314. doi: 10.1002/gepi.21965
Burgess, S., and Thompson, S. G., CRP CHD Genetics Collaboration (2011). Avoiding bias from weak instruments in Mendelian randomization studies. Int. J. Epidemiol. 40, 755–764. doi: 10.1093/ije/dyr036
Cao, Y., Su, N., Zhang, D., Zhou, L., Yao, M., Zhang, S., et al. (2020). Correlation between total homocysteine and cerebral small vessel disease: a Mendelian randomization study. Eur. J. Neurol. doi: 10.1111/ene.14708 [Epub ahead of print]
Casas, J. P., Bautista, L. E., Smeeth, L., Sharma, P., and Hingorani, A. D. (2005). Homocysteine and stroke: evidence on a causal link from mendelian randomisation. Lancet 365, 224–232. doi: 10.1016/S0140-6736(05)70152-5
Cavalieri, M., Schmidt, R., Chen, C., Mok, V., de Freitas, G. R., Song, S., et al. (2012). B vitamins and magnetic resonance imaging-detected ischemic brain lesions in patients with recent transient ischemic attack or stroke: the VITAmins TO Prevent Stroke (VITATOPS) MRI-substudy. Stroke 43, 3266–3270. doi: 10.1161/STROKEAHA.112.665703
Chen, L., Li, Q., Fang, X., Wang, X., Min, J., and Wang, F. (2020). Dietary intake of homocysteine metabolism-related B-vitamins and the risk of stroke: a dose-response meta-analysis of prospective studies. Adv. Nutr. 11, 1510–1528. doi: 10.1093/advances/nmaa061
Chen, H., Liu, S., Ji, L., Wu, T., Ji, Y., Zhou, Y., et al. (2016). Folic acid supplementation mitigates Alzheimer’s disease by reducing inflammation: a randomized controlled trial. Mediat. Inflamm. 2016:5912146. doi: 10.1155/2016/5912146
Clarke, T.-K., Adams, M. J., Davies, G., Howard, D. M., Hall, L. S., Padmanabhan, S., et al. (2017). Genome-wide association study of alcohol consumption and genetic overlap with other health-related traits in UK Biobank (N=112117). Mol. Psychiatry 22, 1376–1384. doi: 10.1038/mp.2017.153
Currò, M., Gugliandolo, A., Gangemi, C., Risitano, R., Ientile, R., and Caccamo, D. (2014). Toxic effects of mildly elevated homocysteine concentrations in neuronal-like cells. Neurochem. Res. 39, 1485–1495. doi: 10.1007/s11064-014-1338-7
Dubchenko, E., Ivanov, A., Spirina, N., Smirnova, N., Melnikov, M., Boyko, A., et al. (2020). Hyperhomocysteinemia and endothelial dysfunction in multiple sclerosis. Brain Sci. 10:637. doi: 10.3390/brainsci10090637
Durga, J., van Tits, L. J. H., Schouten, E. G., Kok, F. J., and Verhoef, P. (2005). Effect of lowering of homocysteine levels on inflammatory markers: a randomized controlled trial. Arch. Intern. Med. 165, 1388–1394. doi: 10.1001/archinte.165.12.1388
Eikelboom, J. W., Lonn, E., Genest, J., Hankey, G., and Yusuf, S. (1999). Homocyst(e)ine and cardiovascular disease: a critical review of the epidemiologic evidence. Ann. Intern. Med. 131, 363–375. doi: 10.7326/0003-4819-131-5-199909070-00008
Emdin, C. A., Khera, A. V., and Kathiresan, S. (2017). Mendelian randomization. JAMA 318, 1925–1926. doi: 10.1001/jama.2017.17219
Evangelou, E., Warren, H. R., Mosen-Ansorena, D., Mifsud, B., Pazoki, R., Gao, H., et al. (2018). Genetic analysis of over 1 million people identifies 535 new loci associated with blood pressure traits. Nat. Genet. 50, 1412–1425. doi: 10.1038/s41588-018-0205-x
Fan, X., Zhang, L., Li, H., Chen, G., Qi, G., Ma, X., et al. (2020). Role of homocysteine in the development and progression of Parkinson’s disease. Ann. Clin. Transl. Neurol. 7, 2332–2338. doi: 10.1002/acn3.51227
Faraci, F. M., and Lentz, S. R. (2004). Hyperhomocysteinemia, oxidative stress, and cerebral vascular dysfunction. Stroke 35, 345–347. doi: 10.1161/01.STR.0000115161.10646.67
Feng, C., Bai, X., Xu, Y., Hua, T., Huang, J., and Liu, X.-Y. (2013). Hyperhomocysteinemia associates with small vessel disease more closely than large vessel disease. Int. J. Med. Sci. 10, 408–412. doi: 10.7150/ijms.5272
Hankey, G. J., and Eikelboom, J. W. (2001). Homocysteine and stroke. Curr. Opin. Neurol. 14, 95–102. doi: 10.1097/00019052-200102000-00015
Hartwig, F. P., Davey Smith, G., and Bowden, J. (2017). Robust inference in summary data Mendelian randomization via the zero modal pleiotropy assumption. Int. J. Epidemiol. 46, 1985–1998. doi: 10.1093/ije/dyx102
Hemani, G., Zheng, J., Elsworth, B., Wade, K. H., Haberland, V., Baird, D., et al. (2018). The MR-base platform supports systematic causal inference across the human phenome. eLife 7:e34408. doi: 10.7554/eLife.34408
Hoffmann, T. J., Choquet, H., Yin, J., Banda, Y., Kvale, M. N., Glymour, M., et al. (2018). A large multiethnic genome-wide association study of adult body mass index identifies novel loci. Genetics 210, 499–515. doi: 10.1534/genetics.118.301479
Huang, T., Chen, Y., Yang, B., Yang, J., Wahlqvist, M. L., and Li, D. (2012). Meta-analysis of B vitamin supplementation on plasma homocysteine, cardiovascular and all-cause mortality. Clin. Nutr. 31, 448–454. doi: 10.1016/j.clnu.2011.01.003
Huo, Y., Li, J., Qin, X., Huang, Y., Wang, X., Gottesman, R. F., et al. (2015). Efficacy of folic acid therapy in primary prevention of stroke among adults with hypertension in China: the CSPPT randomized clinical trial. J. Am. Med. Assoc. 313, 1325–1335. doi: 10.1001/jama.2015.2274
International Multiple Sclerosis Genetics Consortium (IMSGC)., Beecham, A. H., Patsopoulos, N. A., Xifara, D. K., Davis, M. F., Kemppinen, A., et al. (2013). Analysis of immune-related loci identifies 48 new susceptibility variants for multiple sclerosis. Nat. Genet. 45, 1353–1360. doi: 10.1038/ng.2770
Kalaria, R. N., Akinyemi, R., and Ihara, M. (2012). Does vascular pathology contribute to Alzheimer changes? J. Neurol. Sci. 322, 141–147. doi: 10.1016/j.jns.2012.07.032
Kettunen, J., Demirkan, A., Würtz, P., Draisma, H. H. M., Haller, T., Rawal, R., et al. (2016). Genome-wide study for circulating metabolites identifies 62 loci and reveals novel systemic effects of LPA. Nat. Commun. 7:11122. doi: 10.1038/ncomms11122
Kunkle, B. W., Grenier-Boley, B., Sims, R., Bis, J. C., Damotte, V., Naj, A. C., et al. (2019). Genetic meta-analysis of diagnosed Alzheimer’s disease identifies new risk loci and implicates Aβ, tau, immunity and lipid processing. Nat. Genet. 51, 414–430. doi: 10.1038/s41588-019-0358-2
Kwok, T., Wu, Y., Lee, J., Lee, R., Yung, C. Y., Choi, G., et al. (2020). A randomized placebo-controlled trial of using B vitamins to prevent cognitive decline in older mild cognitive impairment patients. Clin. Nutr. 39, 2399–2405. doi: 10.1016/j.clnu.2019.11.005
Larsson, S. C., Traylor, M., Malik, R., Dichgans, M., Burgess, S., Markus, H. S., et al. (2017). Modifiable pathways in Alzheimer’s disease: Mendelian randomisation analysis. BMJ 359:j5375. doi: 10.1136/bmj.j5375
Larsson, S. C., Traylor, M., and Markus, H. S. (2019). Homocysteine and small vessel stroke: a mendelian randomization analysis. Ann. Neurol. 85, 495–501. doi: 10.1002/ana.25440
Latvala, A., and Ollikainen, M. (2016). Mendelian randomization in (epi)genetic epidemiology: an effective tool to be handled with care. Genome Biol. 17:156. doi: 10.1186/s13059-016-1018-9
Lawlor, D. A., Harbord, R. M., Sterne, J. A. C., Timpson, N., and Davey Smith, G. (2008). Mendelian randomization: using genes as instruments for making causal inferences in epidemiology. Stat. Med. 27, 1133–1163. doi: 10.1002/sim.3034
Lee, J. J., Wedow, R., Okbay, A., Kong, E., Maghzian, O., Zacher, M., et al. (2018). Gene discovery and polygenic prediction from a genome-wide association study of educational attainment in 1.1 million individuals. Nat. Genet. 50, 1112–1121. doi: 10.1038/s41588-018-0147-3
Malik, R., Chauhan, G., Traylor, M., Sargurupremraj, M., Okada, Y., Mishra, A., et al. (2018). Multiancestry genome-wide association study of 520,000 subjects identifies 32 loci associated with stroke and stroke subtypes. Nat. Genet. 50, 524–537. doi: 10.1038/s41588-018-0058-3
Manning, A. K., Hivert, M.-F., Scott, R. A., Grimsby, J. L., Bouatia-Naji, N., Chen, H., et al. (2012). A genome-wide approach accounting for body mass index identifies genetic variants influencing fasting glycemic traits and insulin resistance. Nat. Genet. 44, 659–669. doi: 10.1038/ng.2274
Martí-Carvajal, A. J., Solà, I., Lathyris, D., and Dayer, M. (2017). Homocysteine-lowering interventions for preventing cardiovascular events. Cochrane Database Syst. Rev. 8:CD006612. doi: 10.1002/14651858.CD006612.pub5
Mattson, M. P., and Shea, T. B. (2003). Folate and homocysteine metabolism in neural plasticity and neurodegenerative disorders. Trends Neurosci. 26, 137–146. doi: 10.1016/S0166-2236(03)00032-8
McCully, K. S. (1969). Vascular pathology of homocysteinemia: implications for the pathogenesis of arteriosclerosis. Am. J. Pathol. 56, 111–128.
Miao, L., Deng, G.-X., Yin, R.-X., Nie, R.-J., Yang, S., Wang, Y., et al. (2019). No causal effects of plasma homocysteine levels on the risk of coronary heart disease or acute myocardial infarction: a Mendelian randomization study. Eur. J. Prev. Cardiol. doi: 10.1177/2047487319894679 [Epub ahead of print]
Morris, M. S. (2003). Homocysteine and Alzheimer’s disease. Lancet Neurol. 2, 425–428. doi: 10.1016/S1474-4422(03)00438-1
Mujumdar, V. S., Hayden, M. R., and Tyagi, S. C. (2000). Homocyst(e)ine induces calcium second messenger in vascular smooth muscle cells. J. Cell. Physiol. 183, 28–36. doi: 10.1002/(SICI)1097-4652(200004)183:1<28::AID-JCP4>3.0.CO;2-O
Nalls, M. A., Blauwendraat, C., Vallerga, C. L., Heilbron, K., Bandres-Ciga, S., Chang, D., et al. (2019). Expanding Parkinson’s disease genetics: novel risk loci, genomic context, causal insights and heritable risk. bioRxiv [Preprint], 388165. doi: 10.1101/388165
Nam, K.-W., Kwon, H.-M., Jeong, H.-Y., Park, J.-H., Kwon, H., and Jeong, S.-M. (2019). Serum homocysteine level is related to cerebral small vessel disease in a healthy population. Neurology 92, e317–e325. doi: 10.1212/WNL.0000000000006816
Nicolas, A., Kenna, K. P., Renton, A. E., Ticozzi, N., Faghri, F., Chia, R., et al. (2018). Genome-wide analyses identify KIF5A as a novel ALS gene. Neuron 97, 1268.e6–1283.e6. doi: 10.1016/j.neuron.2018.02.027
Nielsen, J. B., Thorolfsdottir, R. B., Fritsche, L. G., Zhou, W., Skov, M. W., Graham, S. E., et al. (2018). Biobank-driven genomic discovery yields new insight into atrial fibrillation biology. Nat. Genet. 50, 1234–1239. doi: 10.1038/s41588-018-0171-3
Nikpay, M., Goel, A., Won, H.-H., Hall, L. M., Willenborg, C., Kanoni, S., et al. (2015). A comprehensive 1,000 genomes-based genome-wide association meta-analysis of coronary artery disease. Nat. Genet. 47, 1121–1130. doi: 10.1038/ng.3396
Obeid, R., and Herrmann, W. (2006). Mechanisms of homocysteine neurotoxicity in neurodegenerative diseases with special reference to dementia. FEBS Lett. 580, 2994–3005. doi: 10.1016/j.febslet.2006.04.088
Ostrakhovitch, E. A., and Tabibzadeh, S. (2019). Homocysteine and age-associated disorders. Ageing Res. Rev. 49, 144–164. doi: 10.1016/j.arr.2018.10.010
Rutten-Jacobs, L. C., Traylor, M., Adib-Samii, P., Thijs, V., Sudlow, C., Rothwell, P. M., et al. (2016). Association of MTHFR C677T genotype with ischemic stroke is confined to cerebral small vessel disease subtype. Stroke 47, 646–651. doi: 10.1161/STROKEAHA.115.011545
Sekula, P., Fabiola Del Greco, M., Pattaro, C., and Köttgen, A. (2016). Mendelian randomization as an approach to assess causality using observational data. J. Am. Soc. Nephrol. 27, 3253–3265. doi: 10.1681/ASN.2016010098
Seshadri, S., Beiser, A., Selhub, J., Jacques, P. F., Rosenberg, I. H., D’Agostino, R. B., et al. (2002). Plasma homocysteine as a risk factor for dementia and Alzheimer’s disease. N. Engl. J. Med. 346, 476–483. doi: 10.1056/NEJMoa011613
Sudlow, C., Gallacher, J., Allen, N., Beral, V., Burton, P., Danesh, J., et al. (2015). UK biobank: an open access resource for identifying the causes of a wide range of complex diseases of middle and old age. PLoS Med. 12:e1001779. doi: 10.1371/journal.pmed.1001779
Van Deerlin, V. M., Sleiman, P. M. A., Martinez-Lage, M., Chen-Plotkin, A., Wang, L.-S., Graff-Radford, N. R., et al. (2010). Common variants at 7p21 are associated with frontotemporal lobar degeneration with TDP-43 inclusions. Nat. Genet. 42, 234–239. doi: 10.1038/ng.536
van Meurs, J. B. J., Pare, G., Schwartz, S. M., Hazra, A., Tanaka, T., Vermeulen, S. H., et al. (2013). Common genetic loci influencing plasma homocysteine concentrations and their effect on risk of coronary artery disease. Am. J. Clin. Nutr. 98, 668–676. doi: 10.3945/ajcn.112.044545
VITATOPS Trial Study Group (2010). B vitamins in patients with recent transient ischaemic attack or stroke in the VITAmins TO Prevent Stroke (VITATOPS) trial: a randomised, double-blind, parallel, placebo-controlled trial. Lancet Neurol. 9, 855–865. doi: 10.1016/S1474-4422(10)70187-3
Wang, H., and Fan, D. (2012). The relation between plasma homocysteine level and amyotrophic lateral sclerosis. Zhonghua Nei Ke Za Zhi 51, 308–310. doi: 10.3760/cma.j.issn.0578-1426.2012.04.015
Wardlaw, J. M., Smith, C., and Dichgans, M. (2019). Small vessel disease: mechanisms and clinical implications. Lancet Neurol. 18, 684–696. doi: 10.1016/S1474-4422(19)30079-1
Willer, C. J., Schmidt, E. M., Sengupta, S., Peloso, G. M., Gustafsson, S., Kanoni, S., et al. (2013). Discovery and refinement of loci associated with lipid levels. Nat. Genet. 45, 1274–1283. doi: 10.1038/ng.2797
Wu, X., Zhou, Q., Chen, Q., Li, Q., Guo, C., Tian, G., et al. (2020). Association of homocysteine level with risk of stroke: a dose-response meta-analysis of prospective cohort studies. Nutr. Metab. Cardiovasc. Dis. 30, 1861–1869. doi: 10.1016/j.numecd.2020.07.026
Yamazaki, Y., and Kanekiyo, T. (2017). Blood-brain barrier dysfunction and the pathogenesis of Alzheimer’s disease. Int. J. Mol. Sci. 18:1965. doi: 10.3390/ijms18091965
Yoo, J. S., Ryu, C.-H., Kim, Y. S., Kim, H.-J., Bushnell, C. D., and Kim, H. Y. (2020). Homocysteinemia is associated with the presence of microbleeds in cognitively impaired patients. J. Stroke Cerebrovasc. Dis. 29:105302. doi: 10.1016/j.jstrokecerebrovasdis.2020.105302
Zhang, T., Jiang, Y., Zhang, S., Tie, T., Cheng, Y., Su, X., et al. (2020). The association between homocysteine and ischemic stroke subtypes in Chinese: a meta-analysis. Medicine 99:e19467. doi: 10.1097/MD.0000000000019467
Zhang, Y.-J., Zhang, L., Huang, X.-L., Duan, Y., Yang, L.-J., and Wang, J. (2018). The association between homocysteine and systemic sclerosis: a review of the literature and meta-analysis. Mod. Rheumatol. 28, 681–689. doi: 10.1080/14397595.2017.1386844
Keywords: Mendelian randomization, homocysteine, cerebrovascular disease, neurodegenerative disease, small artery occlusion, blood pressure, diabetes mellitus
Citation: Liu W, Zhang L, Li S, Liu C, Tong Y, Fang H, Zhang R, Song B, Xia Z and Xu Y (2021) A Mendelian Randomization Study of Plasma Homocysteine Levels and Cerebrovascular and Neurodegenerative Diseases. Front. Genet. 12:653032. doi: 10.3389/fgene.2021.653032
Edited by:
Raymond Noordam, Leiden University Medical Center, NetherlandsReviewed by:
Yuesong Pan, Capital Medical University, ChinaStella Trompet, Leiden University Medical Center, Netherlands
Copyright © 2021 Liu, Zhang, Li, Liu, Tong, Fang, Zhang, Song, Xia and Xu. This is an open-access article distributed under the terms of the Creative Commons Attribution License (CC BY). The use, distribution or reproduction in other forums is permitted, provided the original author(s) and the copyright owner(s) are credited and that the original publication in this journal is cited, in accordance with accepted academic practice. No use, distribution or reproduction is permitted which does not comply with these terms.
*Correspondence: Yuming Xu, eHV5dW1pbmdAenp1LmVkdS5jbg==; Zongping Xia, enhpYTIwMThAenp1LmVkdS5jbg==