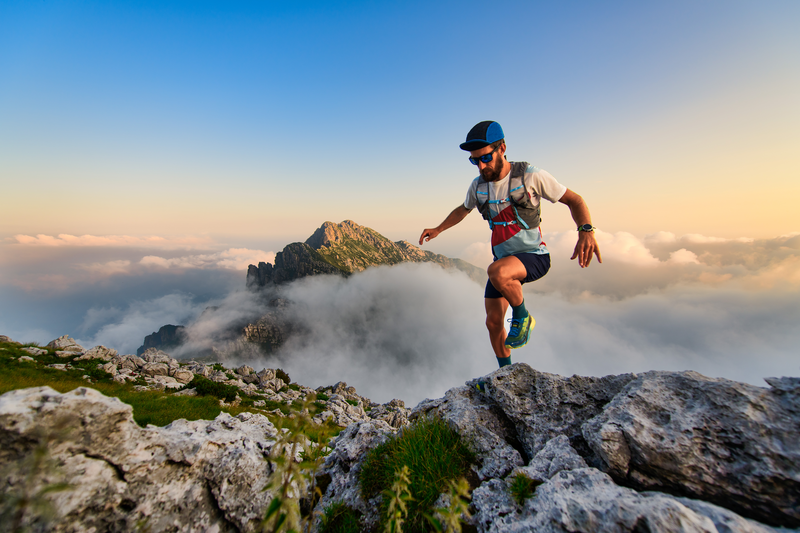
95% of researchers rate our articles as excellent or good
Learn more about the work of our research integrity team to safeguard the quality of each article we publish.
Find out more
ORIGINAL RESEARCH article
Front. Genet. , 08 March 2021
Sec. Evolutionary and Population Genetics
Volume 12 - 2021 | https://doi.org/10.3389/fgene.2021.615911
This article is part of the Research Topic Conservation Genomic Studies for Threatened Plants View all 9 articles
Mangrove forest ecosystems, which provide important ecological services for marine environments and human activities, are being destroyed worldwide at an alarming rate. The objective of our study was to use molecular data and analytical techniques to separate the effects of historical and contemporary processes on the distribution of mangroves and patterns of population genetic differentiation. Seven mangrove species (Acanthus ilicifolius, Aegiceras corniculatum, Avicennia marina, Bruguiera gymnorrhiza, Kandelia obovata, Lumnitzera racemosa, and Rhizophora stylosa), which are predominant along the coastlines of South China, were genotyped at nuclear (nSSR) and chloroplast (cpSSR) microsatellite markers. We estimated historical and contemporary gene flow, the genetic diversity and population structure of seven mangrove species in China. All of these seven species exhibited few haplotypes, low levels of genetic diversity (HE = 0.160–0.361, with the exception of K. obovata) and high levels of inbreeding (FIS = 0.104–0.637), which may be due to their marginal geographical distribution, human-driven and natural stressors on habitat loss and fragmentation. The distribution patterns of haplotypes and population genetic structures of seven mangrove species in China suggest historical connectivity between populations over a large geographic area. In contrast, significant genetic differentiation [FST = 0.165–0.629 (nSSR); GST = 0.173–0.923 (cpSSR)] indicates that populations of mangroves are isolated from one another with low levels of contemporary gene flow among populations. Our results suggest that populations of mangroves were historically more widely inter-connected and have recently been isolated, likely through a combination of ocean currents and human activities. In addition, genetic admixture in Beibu Gulf populations and populations surrounding Hainan Island and southern mainland China were attributed to asymmetric gene flow along prevailing oceanic currents in China in historical times. Even ocean currents promote genetic exchanges among mangrove populations, which are still unable to offset the effects of natural and anthropogenic fragmentation. The recent isolation and lack of gene flow among populations of mangroves may affect their long-term survival along the coastlines of South China. Our study enhances the understanding of oceanic currents contributing to population connectivity, and the effects of anthropogenic and natural habitat fragmentation on mangroves, thereby informing future conservation efforts and seascape genetics toward mangroves.
Mangrove forests form conspicuous wetland ecosystems, fringing extensive areas of coastlines in tropical and subtropical regions (Mitsch et al., 2009; Barbier et al., 2011). Approximately 80 species, from 20 plant families, have been recognized as characteristic of this plant community, primarily consisting of trees and shrubs that normally grow in the intertidal zone of marine coastal environments or estuarine margins (Duke, 1992). Mangrove forests are extremely productive ecosystems that provide important ecological services, both to the marine environment (e.g., coastal protection) and to industries including fisheries, timber and plant products; and tourism (Alongi, 2002; Barbier et al., 2011; Manez et al., 2014). Despite their ecological and economic importance, mangroves are being fragmented and destroyed worldwide at an alarming rate due to both climate change and human activity. They include sea-level fluctuations affected by climate change, urbanization, industrial pollution, farmland conversion, embankments for aquaculture ponds, and so on (Farnsworth and Ellison, 1997; Li and Lee, 1997; Ge and Sun, 2001; Stellman et al., 2003; Ong and Gong, 2013; Nguyen, 2014). This loss results in a serious ecological problem and requires urgent conservation in the face of further increases in climate fluctuation which is expected to have a dramatic effect on coastal areas (Ngeve et al., 2016; Yang et al., 2017). Recent phylogenetic and population genetics studies of Rhizophora mucronata in Southeast Asia, Rhizophora racemosa in East Atlantic, Rhizophora mangle in Florida, Sonneratia alba across the Indo-West-Pacific region, showed that historical events (e.g., climate change-induced sea level rise) and contemporary oceanographic conditions (e.g., surface currents) contributed to demographic histories and population genetic structure (Wee et al., 2014; Hodel et al., 2016; Ngeve et al., 2016; Yang et al., 2017). To better protect mangrove communities, a comprehensive understanding of the population dynamics and biographical processes associated with oceanic currents is necessary.
Ocean currents create a complex physical environment that influences dispersal, isolation, population connectivity and genetic structure of marine systems (Nakajima et al., 2014). Oceanic currents can act as gene-exchange corridors for the migration of marine organisms with long-distance dispersal (LDD) capability, and invisible physical barriers which may lead to genetic heterogeneity between adjacent populations (Palumbi, 1994; Gilg and Hilbish, 2003; Mitarai et al., 2009; Nakajima et al., 2014). Oceanic currents interact with historical events, environmental factors (e.g., temperature, salinity, and habitat availability) and biological traits (e.g., dispersal capability and life history) to determine species distribution range and phylogeographical structure in the sea (Nakajima et al., 2014; Li et al., 2017). Mangroves are characterized by a series of morphological and ecophysiological adaptations in thriving in tidally influenced areas (Van der Stocken and Menemenlis, 2017). Most mangroves have viviparous propagules which are buoyant and presumably capable of dispersal by ocean currents, which may play a role in the geographical distribution and population dynamics of mangrove plants (Yang et al., 2017). Mangroves are very dependent on LDD to allow for the colonization of unoccupied habitats, geographically distant areas and range expansion (Cain et al., 2000; Van der Stocken and Menemenlis, 2017). Therefore, it has been suggested that LDD and colonization can prevent speciation of geographically isolated populations (Cain et al., 2000). Several studies based on molecular markers have provided evidence that mangrove can detour around vast landmasses or across open seas and can effectively colonize geographically distant regions (Cain et al., 2000; Chiang et al., 2001; Liao et al., 2009; Takayama et al., 2013). In this study, we considered seven co-occurring mangrove species from five families [Acanthus ilicifolius (Acanthaceae), Aegiceras corniculatum (Myrsinaceae), Avicennia marina (Verbenaceae), Bruguiera gymnorrhiza (Rhizophoraceae), Kandelia obovata (Rhizophoraceae), Lumnitzera racemosa (Combretaceae), and Rhizophora stylosa (Rhizophoraceae)] across their entire distributional range in China. These seven species are dominantly distributed in the South China Sea (SCS). Owing to differences in pollination vectors and propagule types, these species are expected to have different potentials for gene flow. For this purpose, our main goals were to (i) investigate the patterns of genetic diversity and population genetic structure of seven dominant mangrove species with varying reproductive traits along the coastlines of southern China; (ii) examine whether and how oceanic currents along the South China Sea contributed to the biogeographic processes and population genetic structure of mangrove species. We then discuss the effects of major evolutionary processes, as well as the oceanic currents in the South China Sea that might have played dominant roles in shaping the current distribution and genetic patterns of mangrove populations. We further discuss the relative influence of historical versus contemporary processes on shaping the spatial distribution of genetic variation. This will allow us to assess the effects of anthropogenic habitat fragmentation on mangroves, thereby informing conservation efforts toward them.
Seven species, which are common to the coastal mangroves of southeastern China, were included in this study (A. ilicifolius, A. corniculatum, A. marina, B. gymnorrhiza, K. obovata, L. racemosa, and R. stylosa). Kandelia spp. is mainly distributed along the coastlines of Asia and has a wide distribution from western and eastern India, through the South China Sea region to southern China and southern Japan (Tomlinson, 1986). Kandelia spp. has been divided into two species, one of which is still considered as Kandelia candel (distributed from the oceanic coast of Indian Ocean to southern South China Sea), and the other is named as K. obovata (distributed from northern South China Sea to southern Japan) (Sheue et al., 2003). Although these seven species are adapted to the environmental and ecological conditions of mangrove habitats, they still have different morphological attributes.
Three of the seven species (B. gymnorrhiza, K. obovata, and R. stylosa) are viviparous, two species (A. corniculatum and A. marina) are crypto-viviparous, and the remaining species (A. ilicifolius and L. racemosa) are non-viviparous (Ye et al., 2004a; Duke, 2006). The propagules of A. corniculatum and L. racemosa are not buoyant (Clarke, 1995), but the propagules of A. corniculatum, B. gymnorrhiza, K. obovata, and R. stylosa can survive in seawater for more than 3 months (Clarke et al., 2001; Ye et al., 2004b). For the other three species, the propagules can withstand seawater for shorter periods of time, varying between 11 days for A. ilicifolius and 3–4 weeks for A. marina (Steinke, 1986; Clarke et al., 2001).
Our sampling targeted all mangrove communities throughout the coastlines of southeast China, including the provinces of Fujian, Hainan, Guangdong and Guangxi (Table 1). A total of 20 locations, as shown in Figure 1, were selected to collect samples. R. stylosa was present at 15 of these collection sites. In contrast, A. corniculatum and L. racemosa were only found at six of the locations. On average, 40 individuals of each species within each location were sampled, at an interval of at least 10 m. If the number of individuals at each location was less than 40, all of them were sampled. A total of 2,459 individuals were sampled for the seven mangrove species. The number of sampled individuals per population ranged between 8 and 49. All samples were immediately placed in plastic zip lock bags with silica gel for fast drying, and then stored at room temperature.
Table 1. Locations and sample number of the seven dominant mangrove species along the coastlines of South China (AI, Acanthus ilicifolius; AC, Aegiceras corniculatum; AM, Avicennia marina; BG, Bruguiera gymnorrhiza; KO, Kandelia obovata; LR, Lumnitzera racemosa; RS, Rhizophora stylosa).
Figure 1. Sampling locations of the seven dominant mangrove species along the coastline of South China. Location identification numbers are given in brackets. See Table 1 for details of the number of species that were sampled in each of these 20 locations. Dark arrows are South China Sea Warm Current (SCSWC) and Taiwan Warm current (TWC) in the summer, and gray arrows is the China Coastal Current (CCC) in the winter in the South China Sea (Wang et al., 1991; Longhurst, 2007).
Total genomic DNA was extracted from dried leaves, using a modified cetyltrimethylammonium bromide method (Lian et al., 2003). The DNA was dissolved in 200 μL of sterilized water and stored at −30°C.
For microsatellite analysis, samples of A. corniculatum, A. ilicifolius, A. marina, B. gymnorrhiza, K. obovata, L. racemosa, and R. stylosa were genotyped with 10, 6, 11, 8, 10, 9, and 10 polymorphic nuclear microsatellite (nSSR) markers, respectively, and 7, 5, 13, 11, 8, 16, and 8 chloroplast microsatellite (cpSSR) markers, respectively. Details of these nuclear SSR (nSSR) and chloroplast SSR (cpSSR) markers are presented in Supplementary Table 1 (Maguire et al., 2000a; Sugaya et al., 2002; Islam et al., 2004, 2006a, b, 2008; Geng et al., 2006, 2007, 2008b, 2009; Geng, 2007). The PCR conditions for each nSSR and cpSSR locus are described in previous reports, as shown in Supplementary Table 1. The DNA fragment analyses followed the methods reported by Geng et al. (2009) and Lian et al. (2008).
To calculate chloroplast haplotype variation within populations for each species, the number of haplotypes (nh), number of polymorphic loci (np), and Nei’s unbiased haplotype diversity estimates [HE; (Nei, 1987)] were computed using Arlequin version 3.5 (Excoffier and Lischer, 2010).
We calculated Nei’s unbiased genetic distance estimate for all population pairs [GST; (Nei, 1978)], and Nei’s genetic differentiation index among populations, using the PopGene 1.32 program (Yeh and Boyle, 1997). The significance of correlations between genetic (GST) and geographic distances, and between population pairs, was determined according to the Mantel test (Mantel, 1967), with 9,999 random permutations, using GenAlEx 6.5 software (Peakall and Smouse, 2006).
Population differentiation was estimated with ΦST and NST by using Permut & CpSSR version 2.0 (Pons and Petit, 1996; Burban et al., 1999) and significance tested with 10,000 permutations. ΦST is calculated only based on haplotype frequencies, however, NST also accounts for genetic distances between haplotypes. Phylogeographical structuring is usually indicated when NST value is higher than the ΦST values (10,000 permutations; P < 0.05) (Petit et al., 2005).
To determine the evolutionary relationships among haplotypes, a haplotype network based on the median-joining (MJ) algorithm was constructed using Network 5.0 (Bandelt et al., 1999).
For genetic diversity levels of each species, according to nuclear SSR markers, the mean number of alleles per locus (Ave), expected (HE) and observed (HO) heterozygosity, and the number of private alleles (PA) for each population were estimated using GenAlEx 6.5 (Peakall and Smouse, 2006). The allelic richness (R) and inbreeding coefficients (FIS) were estimated using FSTAT version 2.9.4 (Goudet, 2003). The deviation of FIS from zero was tested in each population by 1,000 permutation tests, with a sequential Bonferroni correction. Recent effective population sizes of each population (NE) were estimated using program ONeSAMP 2.0, which uses eight summary genetic statistics for approximate Bayesian computation (Tallmon et al., 2008). The lower and upper bounds of the prior distribution in all populations were two and 1,0000, respectively. Priors of 2–100 and 2–1,000 were also tested to assess whether the results were robust to changes in these assumed values. Analysis of molecular variance [AMOVA; (Excoffier et al., 1992)] was performed according to Arlequin version 3.5 (Excoffier and Lischer, 2010), with 1,000 permutations. We used all the populations of each species to estimate the variances attributable to differences between populations within species and among individuals within populations. The overall Wright’s FST values among all populations of each species were also estimated according to the Arlequin version 3.5 (Excoffier and Lischer, 2010). The pairwise FST (Weir and Cockerham, 1984) values between populations were estimated using GenAlEx6.5 (Peakall and Smouse, 2006). A random effects model, with 9,999 random permutations, was used to test the significance of each FST value.
To test isolation by distance, the relationship between genetic [FST/(1 − FST)] and geographical distances was assessed by Mantel test (Mantel, 1967), using GenAlEx 6.5 (Peakall and Smouse, 2006). Genetic distance was regressed against the straight-line geographic distances for all pairs of populations.
Bayesian methods were also used to recover the optimal number of clusters representing these populations. This analysis was performed using STRUCTURE 2.3 software (Pritchard et al., 2000). Twenty independent runs for each K (population numbers of each species) were performed using 1,000,000 Markov Chain Monte Carlo (MCMC) repetitions and 100,000 burn-in periods (Falush et al., 2003; Gilbert et al., 2012), no prior information, and assuming correlated allele frequencies and admixtures. The optimal K value was determined using the ΔK method of Evanno et al. (2005), as implemented in STRUCTURE HARVESTER (Earl and Vonholdt, 2012). To obtain the optimal alignment of the independent runs, the CLUMPP version 1.1 (Jakobsson and Rosenberg, 2007) was used, with 10,000 random input orders and 10,000 repeats, to calculate the average pairwise similarity (H′) of runs.
We used BAYESASS 3.0.4 (Wilson and Rannala, 2003) and Migrate-N version 3.6 (Beerli and Palczewski, 2010), respectively, to estimate contemporary (past few generations) and historical gene flow (much longer period of time, approximately 4 Ne generations in the past (Beerli and Felsenstein, 2001; Beerli, 2006).
BAYESASS uses a Bayesian approach and a Markov chain Monte Carlo (MCMC) algorithm to estimate migration rates (m) between populations without assuming genetic equilibrium, which reflects gene flow over the most recent several generations (Wilson and Rannala, 2003). We ran 5 independent replicates with different random starting seeds for each species. We used 5 × 107 iterations, with a burn-in of 5 × 106 generations, and a sampling interval of 2000. We optimized the mixing parameters for migration rates (m), allele frequencies (a), and inbreeding coefficients (f) so that posterior acceptance rates for each parameter were between 20 and 60% as suggested by Wilson and Rannala (2003). For A. corniculatum, A. ilicifolius, A. marina, L. racemosa, and R. stylosa, we used m = 0.15, a = 0.4, and f = 0.5; for B. gymnorrhiza and K. obovata, we used m = 0.15, a = 0.4, and f = 0.7, respectively. Convergence of the MCMCs was checked by comparing the traces of each run using TRACER v. 1.7 (Rambaut et al., 2018). Among the five independent runs, we chose the one with the best consistency of the posterior distribution.
We used Migrate-N version 3.6 to investigate historical migration rates (mh) among populations (Beerli and Palczewski, 2010). MIGRATE uses a coalescent theory with MCMC to estimate effective population sizes (Theta = θ, θ = 4 Neμ, where Ne is historical effective population size and μ is mutation rate per generation) and the mutation-scaled migration rate (M) (M = mh/μ) between population pairs. Migrate-N assumes that populations are in migration-drift equilibrium, that population sizes and migration rates are constant through time, and that populations are randomly sampled. To estimate migration, we ran five replicates for each population in Migrate-N using Brownian motion mutation model with constant mutation rates and starting parameters based on FST calculations. The program estimates the parameters θ and M using a Bayesian method (Beerli and Felsenstein, 2001; Beerli, 2006). Both of which could be used to estimate the number of migrants per generation (Nm) into each population using the equation 4 Nm = θ∗ M. We estimated θ and M with slice sampling and uniform prior distribution (for θ, range = 0–100.0, delta = 10; for M, range = 0–1000.0, delta = 100). Each of the five replicate runs was performed with 10,000-recorded genealogies at a sampling interval of 5 increments after discarding the first 100,000 genealogies (burn-in). Four-chain adaptive heating at temperatures (1.0, 1.5, 3.0, and 1,000,000) was used to increase the efficiency of the MCMC. The confidence interval for θ and the migration parameter M was calculated using a percentile approach (Beerli and Felsenstein, 2001). The mean value of M for each population pair in each direction was used.
Based on the genetic diversity of the mangrove species, as estimated by cpSSR markers, nh and np per locus per population ranged from 1.2 (L. racemosa) to 3.2 (A. marina), and from 0.3 (A. ilicifolius) to 2.1 (K. obovata), respectively (Table 2). Low levels of genetic diversity in cpSSRs were consistently observed in all species, as indicated by Nei’s unbiased haplotypic diversity (HE), ranging from 0.050 (R. stylosa) to 0.263 (A. marina; Table 2).
Table 2. Genetic diversity within different populations of the seven dominant mangrove species along the coastlines of South China as revealed by chloroplast microsatellite markers.
The mean number of alleles per locus (Ave) and allelic richness (R) per locus and per population, based on nSSR marker data, ranged from 1.8 (R. stylosa) to 7.2 (K. obovata), and 1.6 (R. stylosa) to 6.3 (K. obovata), respectively (Table 3). The observed (HO) and expected (HE) heterozygosities ranged from 0.088 (R. stylosa) to 0.544 (K. obovata), and 0.160 (R. stylosa) to 0.587 (K. obovata), respectively (Table 3). The lowest and highest genetic diversities were observed for R. stylosa and K. obovata, respectively. The mean values of recent effective population size (NE) ranged from 3.6 (A. ilicifolius) to 206.0 (A. corniculatum) (Table 3).
Table 3. Descriptive statistics over all nuclear microsatellite loci for each population of seven dominant mangrove species along the coastlines of South China.
Allelic richness (R) and expected heterozygosity (HE) data are shown in Supplementary Figure 1 and they suggest that levels of genetic diversity of the seven species were independent of location. Therefore, species co-occurring in a particular site displayed different R, HE, and PA values (Supplementary Figure 1). Most of the inbreeding coefficients were positive, ranging from 0.104 (K. obovata) to 0.637 (L. racemosa), indicating that most of these mangrove populations experienced at least moderate levels of inbreeding (Table 3).
The analysis of genetic differentiation between populations, based on cpSSR data, showed a strong population structure in each mangrove species throughout the coastlines of southeast China. The highest estimate of genetic differentiation (GST) among the populations was for R. stylosa (0.923), followed by A. ilicifolius (0.834), L. racemosa (0.787), A. marina (0.530), K. obovata (0.438), B. gymnorrhiza (0.274), and A. corniculatum (0.173). Significant correlations, between geographical distance and genetic distance (GST) estimates, were observed for A. marina and K. obovata (P < 0.01), but not among the other five species (P = 0.109 to 0.443; Supplementary Figure 2).
Hierarchical AMOVA of nSSR data for the seven species showed that most of the genetic variation was among populations within A. ilicifolius (62.9%), L. racemosa (50.7%), and R. stylosa (58.0%), while, for the other four species, most of the genetic variation was detected within populations (A. corniculatum, 72.9%; A. marina, 75.1%; B. gymnorrhiza, 83.5%; and K. candle, 79.9%; Table 4). The FST values computed across populations for each species, based on nSSR data, ranged from 0.165 (B. gymnorrhiza) to 0.629 (A. ilicifolius), which indicated a high level of genetic differentiation among populations for these species (Table 4). The pairwise FST values, between populations and within the seven species, ranged from 0.251 to 0.822 (A. ilicifolius), 0.025 to 0.373 (A. corniculatum), 0.040 to 0.314 (A. marina), 0.004 to 0.254 (B. gymnorrhiza), 0.015 to 0.351 (K. candle), 0.165 to 0.579 (L. racemosa), and 0.007 to 0.747 (R. stylosa; Supplementary Table 2), which further confirmed that genetic differentiation among the populations was extremely high within all of the species. No significant relationships, between geographical and genetic distance, were observed from the nSSR analysis (P = 0.089–0.607), with the exception of A. corniculatum (P = 0.0001; R2 = 0.622; Supplementary Figure 3).
Table 4. Hierarchical analysis of molecular variance (AMOVA), overall Wrights’s FST and number of migrants per generation (Nm) of the seven dominant mangrove species along the coastlines of South China estimated by nuclear microsatellite markers.
The inferred best K values from STRUCTURE cluster analysis were different between the species, with K = 4 clusters for A. ilicifolius, 3 clusters for A. corniculatum, 2 clusters for A. marina, 2 clusters for B. gymnorrhiza, 4 clusters for K. obovata, 2 clusters for L. racemosa, and 2 clusters for R. stylosa, respectively (Figure 2 and Supplementary Figure 4). These results showed that although different species aggregated in different clusters, populations within a similar geographical distance had similar genetic structures.
Figure 2. Geographic distribution of genetic groups detected from a STRUCTURE analysis of (A) Acanthus ilicifolius (ΔK = 4), (B) Aegiceras corniculatum (ΔK = 3), (C) Avicennia marina (ΔK = 2), (D) Bruguiera gymnorrhiza (ΔK = 2), (E) Kandelia obovata (ΔK = 4), (F) Lumnitzera racemosa (ΔK = 2), and (G) Rhizophora stylosa (ΔK = 2).
The polymorphic cpSSR markers yielded 3 to 11 haplotypes among the seven mangrove species. The highest number of haplotypes was found in A. marina (11). For all of the species, only a few haplotypes were detected in most of their populations (one type in A. ilicifolius, A. corniculatum, B. gymnorrhiza, K. obovata, and R. stylosa; two types in L. racemosa; and three types in A. marina). The remaining haplotypes were found in a few locations. Among seven mangrove species, significant phylogeographic structure was only observed in R. stylosa [NST (0.921) > ΦST (0.805), P < 0.05] (Figure 3 and Supplementary Table 3).
Figure 3. Geographic distribution of cpSSR haplotypes of (A) Acanthus ilicifolius (three haplotypes), (B) Aegiceras corniculatum (five haplotypes), (C) Avicennia marina (11 haplotypes), (D) Bruguiera gymnorrhiza (five haplotypes), (E) Kandelia obovata (10 haplotypes), (F) Lumnitzera racemosa (four haplotypes), and (G) Rhizophora stylosa (four haplotypes).
The contemporary migration rates estimated in BAYESASS were low (m < 0.1) for most population pairs of all seven mangrove species (Supplementary Table 4). However, the number of migrants per generation (Nm) for historical times as calculated by Migrate-N were high (Nm > 1) in seven mangrove species (Supplementary Table 5). Estimates of contemporary gene flow of seven mangrove species were lower than the historical times, which suggest that gene flow might be affected by recent habitat loss and fragmentation of these mangrove species.
Migration analyses inferred from BAYESASS and Migrate-N congruently revealed the similar asymmetric gene flow in all seven mangrove species along the coastlines of South China (Supplementary Tables 4, 5 and Figures 4, 5), and which also revealed that contemporary gene flow was much lower than the historical gene flow. For populations surrounding the Hainan Island and mainland China, strong directional differences in gene flow was detected from populations in eastern Hainan Island (11–17) to the eastern coastlines of South China (3, 4) in four species (A. ilicifolius, A. corniculatum, B. gymnorrhiza, and R. stylosa; Figures 1, 4, 5 and Supplementary Tables 4, 5). For all seven mangrove species, highly asymmetric gene flows were detected from south to north, almost two to thirty-seven times larger than that in the opposite direction, which was congruent with the direction of seasonal marine currents (Figures 1, 4, 5 and Supplementary Tables 4, 5). For northern populations near the Taiwan Strait, strong asymmetric gene flow was detected in five species (A. ilicifolius, A. corniculatum, B. gymnorrhiza, K. obovata, and R. stylosa) (Supplementary Tables 4, 5 and Figures 4, 5). For populations along Beibu Gulf (5–8) and Qiongzhou Strait (11–16), symmetric or asymmetric gene flow was bidirectional (Supplementary Tables 4, 5 and Figures 4, 5). Furthermore, strong directional differences in gene flow were detected between adjacent populations in Beibu Gulf, i.e., gene flow from population 8–10 was ten times higher than the opposite direction in A. corniculatum, A. marina, and K. obovata (Supplementary Tables 5, 6 and Figures 4, 5). Strong directional differences in gene flow were detected in seven species, respectively (highlighted in the Supplementary Tables 4, 5 and Figures 4, 5). In addition, recent and historical gene flow, as assessed in BAYESASS and Migrate-N, was all lowest in A. ilicifolius, and highest in B. gymnorrhiza of the seven mangrove species (Supplementary Tables 4, 5).
Figure 4. Contemporary gene flow estimated by BAYESASS between populations of seven dominant mangrove species along the coastline of South China based on nuclear SSR analysis. The width of each arrow is proportional to the relative level of gene flow.
Figure 5. Gene flow estimated by Migrate-N between populations of seven dominant mangrove species based on nuclear SSR analysis. The direction of gene flow shown by the levels of Nm (the effective number of migrants per generations). The width of each arrow is proportional to the relative level of gene flow.
Accurate estimates of genetic diversity are very useful for optimizing sampling strategies, and for conserving and managing the genetic resources of trees (Hamrick et al., 1991; Schaal et al., 1991; Chalmers et al., 1992; Cardoso et al., 1998). The current distribution of mangrove species in China is located in the most northerly boundary of mangroves distribution worldwide. Nuclear SSR markers revealed low levels of genetic diversity in the populations of most mangrove species in China (HE from 0.160–0.361), with the exception of K. obovata (HE = 0.587). High levels of inbreeding (FIS = 0.104–0.637) were also detected in most populations. Small recent effective population sizes for all mangrove species (3.6–206.0) were also detected. The cpSSR markers identified only a few haplotypes that were found in most populations, while the remaining haplotypes only occurred in a few populations (Figure 3).
Low genetic diversity might arise from population substructures or founder events, in which locally, high level of inbreeding have led to demes of genetically similar individuals (Tan et al., 2005). During interglacial periods, the founder effect during recolonization led to a further decrease in the genetic diversity of peripheral populations, such as A. marina and Hibiscus tiliaceus (Takayama et al., 2006). The low level of genetic diversity of most mangrove species in our study, was also reported in some previous molecular studies of mangroves along the coastlines of China, such as B. gymnorrhiza (Ge et al., 2005) and Ceriops tagal (Ge and Sun, 2001). These results may support the central-marginal hypothesis (Sagarin et al., 2006), which predicts lower genetic diversity and higher genetic differentiation in marginal populations (Eckert et al., 2008). A similar trend in the genetic characteristics between marginal and central populations was revealed in widely distributed mangrove species, such as A. marina (Maguire et al., 2000b; Arnaud-Haond et al., 2006), Excoecaria agallocha (Zhang et al., 2008), R. mangle (Sandoval-Castro et al., 2012), and S. alba (Yang et al., 2017). Peripheral populations may undergo consequences of bottlenecks, founder effects, reduced gene flow, strong genetic drift, low effective population size, inbreeding and high environmental stress, which would lead to lower genetic diversity and higher genetic differentiation than in central populations (Eckstein et al., 2006).
Furthermore, nearly two-thirds of the mangrove community in China were destroyed by industrialization, urbanization and coastal development during the past century (Li and Lee, 1997). As a result, the habitat area of mangroves is drastically decreased and discontinuous. Fragmentation effects on species more strongly at the periphery of their distributions compared to the core, which will lead to the loss of genetic variation, the increase of genetic differentiation between populations and the decrease of gene flow (Henle et al., 2017).
Lakshmi et al. (1997) suggested that different mangrove species are likely to display varying degrees of polymorphism, depending on their edaphic preferences and adaptations. We identified species-specific patterns of genetic diversity for the mangrove species included in our study (Supplementary Figure 1), indicating that the genetic diversity of every species might not be location-dependent, but was rather attributed to species-specific differences in their history of establishment and physiological traits.
The South China Sea (SCS) is one of the world’s most diverse marine ecosystems (Trajano et al., 2017), and is a region comprised of diversified mangrove species. The current distribution of mangrove species in China is confined to the northern coastlines of the SCS, and it represents the most northern boundary of worldwide distribution of mangroves. Along the coastlines of southern China, there is the northward flowing South China Sea Warm Current (SCSWC) which converges with the Taiwan Warm current (TWC) in the East China Sea in the summer (Figure 1). As mangrove global discontinuous distributions (Duke et al., 2002), and it has been assumed that buoyant propagules can be passively dispersed by ocean currents over long distances, because of their buoyancy, longevity, and water-dispersal (Clarke, 1993; Steinke and Ward, 2003; Nathan, 2006). However, a long-distance dispersal event is rare, stochastic and complex (Nathan, 2006), which acts as a bridge to connect isolated and distant populations, and potentially contributing to a chaotic genetic pattern for mangroves at a regional level. Here, our integrative evidence suggests that the population genetic structures of seven dominant mangrove species were mainly shaped by oceanic currents along the coastlines of southern China, especially in historical times.
The patterns of cpSSR haplotype distribution and population genetic structures of the seven mangrove species showed that although different species aggregated in different clusters, populations within a similar geographical distance had similar genetic structures (Figures 2, 3), which suggests that propagule dispersal of the mangrove species mostly occurred within a mesoscale range. The importance of considering ocean current patterns in explaining the genetic structure of coastal ecosystems has also been postulated by Wee et al. (2014), who demonstrated that genetic discontinuity between mangrove populations of R. mucronata is maintained by ocean features. Stocken et al. (2019b) also found high rates of along-coast transport and transoceanic dispersal in mangroves across the Atlantic, Pacific and Indian oceans. The trend of historical gene flow follows the direction of ocean currents in mangroves in northern SCS (Supplementary Table 5 and Figure 5), which suggests that the primary ocean currents can drive population genetic connectivity of these seven mangroves in this region in historical times.
First, mangrove populations in Beibu Gulf were mainly affected by the ocean currents (Figures 1, 5). The Beibu Gulf (Gulf of Tonkin), is located in the northwest South China Sea. At the surface, the residual flow direction during winter is basically westward or southwestward, while during summer it is almost reversed in Beibu Gulf (Manh and Yanagi, 2000). A certain degree of genetic connectivity was evident among the populations of Beibu Gulf (5–8) and Qiongzhou Strait (11–16) for all mangrove species, based on both haplotype distributions and Bayesian clustering analysis (Figures 2, 3), which is not consistent with our expectation. Oceanic current-mediated strong asymmetric historical gene flow (Nm > 1) between the populations in the northeast Beibu Gulf around Hainan Island (11–16) and the northern Beibu Gulf (5–8) for five mangrove species were found (Supplementary Table 5 and Figure 5). The oceanic current might lead to a high level of population genetic homogeneity of mangroves across a mesoscale geographical range in historical times. Our results showed that historical gene flow affected the current genetic structure of mangroves to some extent. In addition, strong directional differences in gene flow were detected between adjacent populations in northern Beibu Gulf, i.e., gene flow from population 8 to 10 was ten times higher than the opposite direction in A. corniculatum, A. marina, and K. obovata (Supplementary Table 5 and Figure 5), suggesting that oceanic currents in Beibu Gulf contributed strongly to population connectivity of mangroves in this gulf.
Second, populations of mangroves surrounding the Hainan Island and mainland China were mainly affected by the SCSWC (Figure 1) (Wang et al., 1991). A strong directional differences in gene flow was detected from populations in eastern Hainan Island (11, 17) to the eastern coastlines of South China (3, 4) in four species (A. ilicifolius, A. corniculatum, B. gymnorrhiza, and R. stylosa), over 450 km across the Qiongzhou strait (Figures 1, 5 and Supplementary Table 5). The detected gene flow along the path of SCSWC was almost 2–37 times larger than that in the opposite direction (Supplementary Table 5 and Figure 5), suggesting that the SCSWC contributed strongly to population genetic structure of mangroves in the northern SCS. In addition, near the Taiwan Strait highly asymmetric gene flow was detected from south to north (from population 2 to population 1) along the SCSWC and TWC in A. corniculatum and K. obovata (Figure 5). Such a dispersal pattern has also been observed in seagrass, Sargassum thunbergii (Li et al., 2017), Sargassum horneri (Hu et al., 2011), and Sargassum fusiforme (Hu et al., 2013) in northern SCS, which suggest that the northward running SCSWC and TWC have had more profound impacts on phylogeographical structure of marine species along the China coasts than the southward flowing China Coastal Current (Li et al., 2017).
Our results contribute to the growing evidence that ocean circulation plays important roles in population genetic connectivity in mangroves in the coastlines of southern China. Similar phenomenon was also found in Rhizophora apiculata in the Indo-Malesian region (Guo et al., 2016), R. mangle populations in Brazil and Florida (Pil et al., 2011; Hodel et al., 2016), R. mucronata populations in Southeast Asia (Wee et al., 2014), and R. racemosa in Eastern Atlantic (Ngeve et al., 2016).
Furthermore, the extent of gene flow varied among each mangrove species. The quantification of the numbers of propagules available for dispersal, the timing of propagule release in combination with the tidal framework and seasonal changes in ocean circulation, propagule buoyancy, viability period of propagules in seawater are the potential factors for the mangrove propagule dispersal (Stocken et al., 2019a). Among these factors, dispersal distance and connectivity of mangroves may be highly sensitive to the minimum and maximum floating periods (Stocken et al., 2019b). Recent and historical gene flow, as inferred from BayesAss and Migrate-N, was congruent lowest in A. ilicifolius, while highest in B. gymnorrhiza among seven mangrove species (Supplementary Tables 4, 5). The gene flow comparisons from BayesAss and Migrate-N may mean that the larger, longer-lived propagules of B. gymnorrhiza are more successful in dispersing long distance via ocean currents than the smaller, shorter-lived and no buoyancy propagules of A. ilicifolius (Steinke, 1986; Clarke et al., 2001; Ye et al., 2004b; Stocken et al., 2019b), which was further supported by genetic differentiation in these two species. The FST values of A. ilicifolius (0.629) were higher than for B. gymnorrhiza (0.165).
Mangroves are being fragmented and destroyed, which were attributed primarily to human activities (Ngeve et al., 2016; Goldberg et al., 2020). As a result, mangroves were expected to experience erosion of genetic variation, reduced population size and increased population genetic differentiation and reduced gene flow.
In the present study, the contemporary effective population size (NE) ranged from 3.6 (A. ilicifolius) to 206.0 (A. corniculatum) (Table 3). This is consistent with increasing human activities, which led to a dramatic reduction in the number of habitats and the effective population size of mangroves (Barber et al., 2014; Yang et al., 2017). During a reduction in population size, the range in allele size would be expected to decrease as quickly as the number of alleles (Garza and Williamson, 2001), and as a result genetic diversity is lost very rapidly, which could explain the low genetic diversity of most mangroves in present study.
In the present study, both the nSSR and cpSSR markers revealed the relative high genetic differentiation among populations of the seven species [overall FST = 0.165-0.629 (nSSR); GST = 0.173-0.923 (cpSSR)] according to the AMOVA, especially the A. ilicifolius, L. racemosa and R. stylosa populations (Table 4). Similar results were obtained for previous mangroves studies in China, such as Heritiera littoralis (GST = 0.238) (Jian et al., 2004), C. tagal (GST = 0.529) (Ge and Sun, 2001), K. obovata (GST = 0.5548) (Chen et al., 2010). Furthermore, most of the pairwise FST values between populations within seven mangroves were also significantly high (P < 0.05, Supplementary Table 2). The high level of genetic differentiation seems to be the result of the reduced population size that induced the genetic drift.
In keeping with the above, relative low contemporary gene flow among most population pairs of all seven mangrove species (m < 0.1) was lower than historical gene flow (Nm > 1) (Supplementary Tables 4, 5). This finding shows that the recent habitat fragmentation in these mangroves populations is accompanied by decreased gene flow. This conclusion was confirmed by several studies in mangroves, such as A. corniculatum (Ge and Sun, 1999; Deng et al., 2009), C. tagal (Ge and Sun, 2001; Liao et al., 2011), H. littoralis (Jian et al., 2004; Jian S.-G. et al., 2010), K. candel (Huang, 1994; Sun et al., 1998; Chiang et al., 2001; Geng et al., 2008a), L. racemosa (Su et al., 2006), and Nypa fruticans (Jian S. et al., 2010). Reduced contemporary gene flow can further decrease genetic diversity and contribute to high levels of genetic differentiation in these mangrove species, which is consistent with the conclusions above. Although, the trend of recent gene flow follows the direction of ocean currents in mangroves in northern SCS, it is too little to offset the dramatic habitat fragmentation in the short term.
Overall, we found that most mangrove species in China have low levels of genetic variation within populations, except for K. obovata. Local population extinction could result in a decreased potential to adapt to environmental changes, through the loss of alleles with adaptive value. In addition, a reduction in the number of populations and their sizes could enhance genetic drift and inbreeding, leading to reduced fitness. The conservation of mangrove genetic resources involves not only preventing extinction, but also ensuring the preservation of alleles that could provide adaptive values in changing environments (Namkoong, 1997; Tan et al., 2005).
The patterns of cpSSR haplotype distribution, population genetic structure and strong asymmetric historical gene flow of the seven mangrove species along the coastlines of China, suggests that populations of mangroves were mainly driven by oceanic currents in historical times. In contrast, high genetic differentiation indicates that populations of mangroves are isolated with low levels of contemporary gene flow, which might be due to natural and anthropogenic habitat fragmentation. The recent isolation and lack of gene flow among populations of mangroves may affect their long-term survival along the coastlines of South China. Our results showed that the strong and marked genetic differentiation among populations of each of the species resulted in several geographically distinct genetic clusters, indicating the existence of genetically-similar bioregions. Four bioregions (Fujian, Guangdong, Guangxi, and Hainan) could be identified based on genetic structure results, and any activities resulting in gene mixing with each other ought to be prevented. We suggest that future ecological restoration initiatives should establish these bioregions as conservation units. Within the different bioregions of each species, plants could be transplanted to diminish the risk of fitness decline and loss of genetic diversity of the native plant populations.
The raw data supporting the conclusions of this article will be made available by the authors, without undue reservation.
QG, CL, and TH conceived the study. QG performed the experiments, analyzed the data, and wrote the manuscript. JT helped to collect the samples. ZW, MK, and HL provided useful suggestions on the manuscript. All authors contributed to the manuscript revision, read and approved the submitted version.
This work was supported by Grants-in-Aid from the Japan Society for the Promotion of Science (18405028).
The authors declare that the research was conducted in the absence of any commercial or financial relationships that could be construed as a potential conflict of interest.
We are grateful to R. L. Qiu, J. C. Huang, S. Q. Li, H. Li, M. M. Liang, K. Y. Lin, and F. H. Xu for their kind assistance in collecting samples from China. We thank M. S. Islam, M. A. Wadud, N. Miyashita, C. Chen, and J. T Sun for their kind technical help. We also thank S. Goto for his good suggestions on our manuscript.
The Supplementary Material for this article can be found online at: https://www.frontiersin.org/articles/10.3389/fgene.2021.615911/full#supplementary-material
Supplementary Figure 1 | The allelic richness (R), expected heterozygosity (HE), and number of private alleles (PA) over all nuclear SSR loci in each population of the seven dominant mangrove species along the coastline of South China.
Supplementary Figure 2 | The correlations between Nei’s unbiased genetic distance (GST) estimated by cpSSR loci and geographical distance of the seven dominant mangrove species along the coastline of South China.
Supplementary Figure 3 | Correlation between genetic FST/(1 − FST) estimated by nuclear SSR loci and geographic distances among populations of the seven dominant mangrove species along the coastline of South China.
Supplementary Figure 4 | The Delta K values (Delta K = mean[| L′′(K)|]/sd [L(K]) detected from STRUCTURE HARVESTER of seven dominant mangrove species: (A) Acanthus ilicifolius, (B) Aegiceras corniculatum, (C) Avicennia marina, (D) Bruguiera gymnorrhiza, (E) Kandelia obovata, (F) Lumnitzera racemosa, and (G) Rhizophora stylosa.
Supplementary Table 1 | Chloroplast and nuclear microsatellite loci of the seven mangrove species used in this experiment.
Supplementary Table 2 | Matrix of pairwise comparisons of population genetic differentiation (FST) of the seven dominant mangrove species along the coastline of South China from nuclear SSR analysis.
Supplementary Table 3 | Estimation of inter-population differentiation (GST) and the number of substitution types (NST) (mean ± SE in parentheses) of seven dominant mangrove species along the coastline of South China.
Supplementary Table 4 | Recent migration rate between populations estimated from BAYESASS of seven dominant mangrove species along the coastline of South China from nuclear SSR analysis.
Supplementary Table 5 | Mutation-scaled effective population size (Theta = 4Neμ) and mutation-scaled migration rate (M) estimated from Migrate-N of seven dominant mangrove species along the coastline of South China from nuclear SSR analysis.
Alongi, D. M. (2002). Present state and future of the world’s mangrove forests. Environ. Conserv. 29, 331–349. doi: 10.1017/S0376892902000231
Arnaud-Haond, S., Teixeira, S., Massa, S. I., Billot, C., Saenger, P., Coupland, G., et al. (2006). Genetic structure at range edge: low diversity and high inbreeding in Southeast Asian mangrove (Avicennia marina) populations. Mol. Ecol. 15, 3515–3525. doi: 10.1111/j.1365-294X.2006.02997.x
Bandelt, H. J., Forster, P., and Rohl, A. (1999). Median-joining networks for inferring intraspecific phylogenies. Mol. Biol. Evol. 16, 37–48. doi: 10.1093/oxfordjournals.molbev.a026036
Barber, C. P., Cochrane, M. A., Souza, C. M., and Laurance, W. F. (2014). Roads, deforestation, and the mitigating effect of protected areas in the Amazon. Biol. Conserv. 177, 203–209. doi: 10.1016/j.biocon.2014.07.004
Barbier, E. B., Hacker, S. D., Kennedy, C., Koch, E. W., Stier, A. C., and Silliman, B. R. (2011). The value of estuarine and coastal ecosystem services. Ecol. Monogr. 81, 169–193. doi: 10.1890/10-1510.1
Beerli, P. (2006). Comparison of Bayesian and maximum-likelihood inference of population genetic parameters. Bioinformatics 22, 341–345. doi: 10.1093/bioinformatics/bti803
Beerli, P., and Felsenstein, J. (2001). Maximum likelihood estimation of a migration matrix and effective population sizes in n subpopulations by using a coalescent approach. Proc. Natl. Acad. Sci. U.S.A. 98, 4563–4568. doi: 10.1073/pnas.081068098
Beerli, P., and Palczewski, M. (2010). Unified framework to evaluate panmixia and migration direction among multiple sampling locations. Genetics 185, 313–U463. doi: 10.1534/genetics.109.112532
Burban, C., Petit, R. J., Carcreff, E., and Jactel, H. (1999). Rangewide variation of the maritime pine bast scale Matsucoccus feytaudi Duc. (Homoptera : Matsucoccidae) in relation to the genetic structure of its host. Mol. Ecol. 8, 1593–1602. doi: 10.1046/j.1365-294x.1999.00739.x
Cain, M. L., Milligan, B. G., and Strand, A. E. (2000). Long-distance seed dispersal in plant populations. Am. J. Bot. 87, 1217–1227. doi: 10.2307/2656714
Cardoso, M. A., Provan, J., Powell, W., Ferreira, P. C. G., and De Oliveira, D. E. (1998). High genetic differentiation among remnant populations of the endangered Caesalpinia echinata Lam. (Leguminosae-Caesalpinioideae). Mol. Ecol. 7, 601–608. doi: 10.1046/j.1365-294x.1998.00363.x
Chalmers, K. J., Waugh, R., Sprent, J. I., Simons, A. J., and Powell, W. (1992). Detection of genetic variation between and within populations of Gliricidia sepium and G. maculata using RAPD markers. Heredity 69, 465–472. doi: 10.1038/hdy.1992.151
Chen, S. B., Ding, W. Y., Qiu, J. B., Wang, G. Y., Zhou, Z. M., Chen, J. F., et al. (2010). The genetic diversity of the mangrove Kandelia obovata in china revealed by ISSR analysis. Pakistan J. Bot. 42, 3755–3764.
Chiang, T. Y., Chiang, Y. C., Chen, Y. J., Chou, C. H., Havanond, S., Hong, T. N., et al. (2001). Phylogeography of Kandelia candel in East Asiatic mangroves based on nucleotide variation of chloroplast and mitochondrial DNAs. Mol. Ecol. 10, 2697–2710. doi: 10.1046/j.0962-1083.2001.01399.x
Clarke, P. J. (1993). Dispersal of gray mangrove (Avicennia marina) propagules in southeastern Australia. Aquat. Bot. 45, 195–204. doi: 10.1016/0304-3770(93)90021-N
Clarke, P. J. (1995). The population dynamics of the mangrove shrub Aegiceras corniculatum (Myrsinaceae): fecundity, dispersal, establishment and population structure. Proc. Linn. Soc. New South Wales 115, 35–44.
Clarke, P. J., Kerrigan, R. A., and Westphal, C. J. (2001). Dispersal potential and early growth in 14 tropical mangroves: do early life history traits correlate with patterns of adult distribution? J. Ecol. 89, 648–659. doi: 10.1046/j.0022-0477.2001.00584.x
Deng, S., Huang, Y., He, H., Tan, F., Ni, X., Jayatissa, L. P., et al. (2009). Genetic diversity of Aegiceras corniculatum (Myrsinaceae) revealed by amplified fragment length polymorphism (AFLP). Aquat. Bot. 90, 275–281. doi: 10.1016/j.aquabot.2008.11.002
Duke, N. C. (1992). “Mangrove floristics and biogeography,” in Tropical Mangrove Ecosystem, eds A. Robertson and D. Alongi (Washington, DC: American Geographical Union), 63–100. doi: 10.1029/CE041p0063
Duke, N. C. (2006). Australia’s Mangroves. The Authoritative Guide to Australia’s Mangrove Plants. Brisbane, QLD: University of Queensland.
Duke, N. C., Lo, E. Y. Y., and Sun, M. (2002). Global distribution and genetic discontinuities of mangroves – emerging patterns in the evolution of Rhizophora. Trees Struct. Funct. 16, 65–79. doi: 10.1007/s00468-001-0141-7
Earl, D. A., and Vonholdt, B. M. (2012). STRUCTURE HARVESTER: a website and program for visualizing STRUCTURE output and implementing the Evanno method. Conserv. Genet. Resour. 4, 359–361. doi: 10.1007/s12686-011-9548-7
Eckert, C. G., Samis, K. E., and Lougheed, S. C. (2008). Genetic variation across species’ geographical ranges: the central-marginal hypothesis and beyond. Mol. Ecol. 17, 1170–1188. doi: 10.1111/j.1365-294X.2007.03659.x
Eckstein, R. L., O’Neill, R. A., Danihelka, J., Otte, A., and Kohler, W. (2006). Genetic structure among and within peripheral and central populations of three endangered floodplain violets. Mol. Ecol. 15, 2367–2379. doi: 10.1111/j.1365-294X.2006.02944.x
Evanno, G., Regnaut, S., and Goudet, J. (2005). Detecting the number of clusters of individuals using the software STRUCTURE: a simulation study. Mol. Ecol. 14, 2611–2620. doi: 10.1111/j.1365-294X.2005.02553.x
Excoffier, L., and Lischer, H. E. L. (2010). Arlequin suite ver 3.5: a new series of programs to perform population genetics analyses under Linux and Windows. Mol. Ecol. Resour. 10, 564–567. doi: 10.1111/j.1755-0998.2010.02847.x
Excoffier, L., Smouse, P. E., and Quattro, J. M. (1992). Analysis of molecular variance inferred from metric distances among DNA haplotypes application to human mitochondrial DNA restriction data. Genetics 131, 479–491. doi: 10.1093/genetics/131.2.479
Falush, D., Stephens, M., and Pritchard, J. K. (2003). Inference of population structure using multilocus genotype data: Linked loci and correlated allele frequencies. Genetics 164, 1567–1587.
Farnsworth, E. J., and Ellison, A. M. (1997). The global conservation status of mangroves. Ambio 26, 328–334.
Garza, J. C., and Williamson, E. G. (2001). Detection of reduction in population size using data from microsatellite loci. Mol. Ecol. 10, 305–318. doi: 10.1046/j.1365-294x.2001.01190.x
Ge, J. P., Cai, B. Y., Ping, W. X., Song, G., Ling, H. Z., and Lin, P. (2005). Mating system and population genetic structure of Bruguiera gymnorrhiza (Rhizophoraceae), a viviparous mangrove species in China. J. Exp. Mar. Biol. Ecol. 326, 48–55. doi: 10.1016/j.jembe.2005.05.015
Ge, X. J., and Sun, M. (1999). Reproductive biology and genetic diversity of a cryptoviviparous mangrove Aegiceras corniculatum (Myrsinaceae) using allozyme and intersimple sequence repeat (ISSR) analysis. Mol. Ecol. 8, 2061–2069. doi: 10.1046/j.1365-294x.1999.00821.x
Ge, X. J., and Sun, M. (2001). Population genetic structure of Ceriops tagal (Rhizophoraceae) in Thailand and China. Wetlands Ecol. Manag. 9, 203–209. doi: 10.1023/A:1011156707160
Geng, Q. F. (2007). Development of microsatellite markers in a mangrove tree species Aegiceras corniculatum (Myrsinaceae) (vol 6, pg 1231, 2006). Mol. Ecol. Notes 7, 176–176. doi: 10.1111/j.1471-8286.2006.01499.x
Geng, Q. F., Kimura, M., Lian, C. L., Tao, J. M., and Hogetsu, T. (2009). Isolation and characterization of chloroplast microsatellite (cpSSR) markers in four mangrove species, Acanthus ilicifolius, Aegiceras corniculatum, Avicennia marina and Lumnitzera racemosa. Conserv. Genet. 10, 1133–1140. doi: 10.1007/s10592-008-9727-6
Geng, Q. F., Lian, C. L., Goto, S., Tao, J. M., Kimura, M., Islam, M. D. S., et al. (2008a). Mating system, pollen and propagule dispersal, and spatial genetic structure in a high-density population of the mangrove tree Kandelia candel. Mol. Ecol. 17, 4724–4739. doi: 10.1111/j.1365-294X.2008.03948.x
Geng, Q. F., Lian, C. L., Tao, J. M., and Hogetsu, T. (2008b). Development of microsatellite markers for two nonviviparous mangrove species, Acanthus ilicifolius and Lumnitzera racemosa. Mol. Ecol. Resour. 8, 377–380. doi: 10.1111/j.1471-8286.2007.01962.x
Geng, Q. F., Lian, C. L., Tao, J. M., Li, Q., and Hogetsu, T. (2007). Isolation and characterization of 10 new compound microsatellite markers for a mangrove tree species, Avicennia marina (Forsk.) Vierh. (Avicenniaceae). Mol. Ecol. Notes 7, 1208–1210. doi: 10.1111/j.1471-8286.2007.01834.x
Geng, Q. F., Lian, C. L., Tao, J. M., Qiu, R. L., and Hogetsu, T. (2006). Development of microsatellite markers in a mangrove tree species Aegiceras corniculatum (Myrsinaceae). Mol. Ecol. Notes 6, 1231–1233. doi: 10.1111/j.1471-8286.2006.01499.x
Gilbert, K. J., Andrew, R. L., Bock, D. G., Franklin, M. T., Kane, N. C., Moore, J.-S., et al. (2012). Recommendations for utilizing and reporting population genetic analyses: the reproducibility of genetic clustering using the program STRUCTURE. Mol. Ecol. 21, 4925–4930. doi: 10.1111/j.1365-294X.2012.05754.x
Gilg, M. R., and Hilbish, T. J. (2003). The geography of marine larval dispersal: coupling genetics with fine-scale physical oceanography. Ecol. Lett. 84, 2989–2998. doi: 10.1890/02-0498
Goldberg, L., Lagomasino, D., Thomas, N., and Fatoyinbo, T. (2020). Global declines in human-driven mangrove loss. Glob. Change Biol. 26, 5844–5855. doi: 10.1111/gcb.15275
Goudet, J. (2003). FSTAT (ver. 2.9.4), A Program to Estimate and Test Population Genetics Parameters. Available online at: http://www.unil.ch/izea/softwares/fstat.html. Updated from Goudet (1995).
Guo, Z. X., Huang, Y. L., Chen, Y. M., Duke, N. C., Zhong, C. R., and Shi, S. H. (2016). Genetic discontinuities in a dominant mangrove Rhizophora apiculata (Rhizophoraceae) in the Indo-Malesian region. J. Biogeogr. 43, 1856–1868. doi: 10.1111/jbi.12770
Hamrick, J. L., Godt, M. J. W., Murawski, D. A., and Loveless, M. D. (1991). “Correlations between species traits and allozyme diversity implications for conservation biology,” in Genetics and Conservation of Rare Plants, eds D. A. Falk and K. E. Holsinger (New York, NY: Oxford University Press), 75–86.
Henle, K., Andres, C., Bernhard, D., Grimm, A., Stoev, P., Tzankov, N., et al. (2017). Are species genetically more sensitive to habitat fragmentation on the periphery of their range compared to the core? A case study on the sand lizard (Lacerta agilis). Lands. Ecol. 32, 131–145. doi: 10.1007/s10980-016-0418-2
Hodel, R. G. J., Cortez, M. B. D. S., Soltis, P. S., and Soltis, D. E. (2016). Comparative phylogeography of black mangroves (Avicennia germinans) and red mangroves (Rhizophora mangle) in Florida: testing the maritime discontinuity in coastal plants. Am. J. Bot. 103, 1–10. doi: 10.3732/ajb.1500260
Hu, Z. M., Uwai, S., Yu, S. H., Komatsu, T., Ajisaka, T., and Duan, D. L. (2011). Phylogeographic heterogeneity of the brown macroalga Sargassum horneri (Fucaceae) in the northwestern Pacific in relation to late Pleistocene glaciation and tectonic configurations. Mol. Ecol. 20, 3894–3909. doi: 10.1111/j.1365-294X.2011.05220.x
Hu, Z. M., Zhang, J., Lopez-Bautista, J., and Duan, D. L. (2013). Asymmetric genetic exchange in the brown seaweed Sargassum fusiforme (Phaeophyceae) driven by oceanic currents. Mar. Biol. 160, 1407–1414. doi: 10.1007/s00227-013-2192-x
Huang, S. (1994). “Genetic variation of Kandelia candel (L) Druce (Rhizophoraceae) In Taiwan,” in Proceedings of the International Symposium on Genetic Conservation and Production of Tropical Forest Tree Seed, Ching Mai, Thailand, 1993, eds R. M. Drysdale, S. E. T. John, and A. C. Yapa (Saraburi: ASEAN-Canada Forest Tree Seed Centre Project), 165–172.
Islam, M. S., Lian, C., Kameyama, N., Wu, B., and Hogetsu, T. (2004). Development of microsatellite markers in Rhizophora stylosa using a dual-suppression-polymerase chain reaction technique. Mol. Ecol. Notes 4, 110–112. doi: 10.1046/j.1471-8286.2003.00585.x
Islam, M. S., Lian, C. L., Geng, Q. F., Kameyama, N., and Hogetsu, T. (2008). Chloroplast microsatellite markers for the mangrove tree species Bruguiera gymnorrhiza, Kandelia candel, and Rhizophora stylosa, and cross-amplification in other mangrove species. Conserv. Genet. 9, 989–993. doi: 10.1007/s10592-007-9424-x
Islam, M. S., Lian, C. L., Kameyama, N., Wu, B., and Hogetsu, T. (2006a). Development and characterization of ten new microsatellite markers in a mangrove tree species Bruguiera gymnorrhiza (L.). Mol. Ecol. Notes 6, 30–32. doi: 10.1111/j.1471-8286.2005.01127.x
Islam, M. S., Tao, J. M., Geng, Q. F., Lian, C. L., and Hogetsu, T. (2006b). Isolation and characterization of eight compound microsatellite markers in a mangrove tree Kandelia candel (L.) Druce. Mol. Ecol. Notes 6, 1111–1113. doi: 10.1111/j.1471-8286.2006.01453.x
Jakobsson, M., and Rosenberg, N. A. (2007). CLUMPP: a cluster matching and permutation program for dealing with label switching and multimodality in analysis of population structure. Bioinformatics 23, 1801–1806. doi: 10.1093/bioinformatics/btm233
Jian, S., Ban, J., Ren, H., and Yan, H. (2010). Low genetic variation detected within the widespread mangrove species Nypa fruticans (Palmae) from Southeast Asia. Aquat. Bot. 92, 23–27. doi: 10.1016/j.aquabot.2009.09.003
Jian, S. G., Tang, T., Zhong, Y., and Shi, S. B. (2004). Variation in inter-simple sequence repeat (ISSR) in mangrove and non-mangrove populations of Heritiera littoralis (Sterculiaceae) from China and Australia. Aquat. Bot. 79, 75–86. doi: 10.1016/j.aquabot.2004.01.005
Jian, S.-G., Tang, T., Zhong, Y., and Shi, S.-H. (2010). Conservation genetics of Heritiera littoralis (Sterculiaceae), a threatened mangrove in China, based on AFLP and ISSR markers. Biochem. Syst. Ecol. 38, 924–930. doi: 10.1016/j.bse.2010.09.016
Lakshmi, M., Rajalakshmi, S., Parani, M., Anuratha, C. S., and Parida, A. (1997). Molecular phylogeny of mangroves. 1. Use of molecular markers in assessing the intraspecific genetic variability in the mangrove species Acanthus ilicifolius Linn (Acanthaceae). Theoret. Appl. Genet. 94, 1121–1127. doi: 10.1007/s001220050525
Li, J. J., Hu, Z. M., Gao, X., Sun, Z. M., Choi, H. G., Duan, D. L., et al. (2017). Oceanic currents drove population genetic connectivity of the brown alga Sargassum thunbergii in the north-west Pacific. J. Biogeogr. 44, 230–242. doi: 10.1111/jbi.12856
Li, M. S., and Lee, S. Y. (1997). Mangroves of China: a brief review. For. Ecol. Manag. 96, 241–259. doi: 10.1016/S0378-1127(97)00054-6
Lian, C. L., Goto, S., Kubo, T., Takahashi, Y., Nakagawa, M., and Hogetsu, T. (2008). Nuclear and chloroplast microsatellite analysis of Abies sachalinensis regeneration on fallen logs in a subboreal forest in Hokkaido, Japan. Mol. Ecol. 17, 2948–2962. doi: 10.1111/j.1365-294X.2008.03802.x
Lian, C. L., Oishi, R., Miyashita, N., Nara, K., Nakaya, H., Wu, B. Y., et al. (2003). Genetic structure and reproduction dynamics of Salix reinii during primary succession on Mount Fuji, as revealed by nuclear and chloroplast microsatellite analysis. Mol. Ecol. 12, 609–618. doi: 10.1046/j.1365-294X.2003.01756.x
Liao, P. C., Chiang, Y. C., Huang, S., and Wang, J. C. (2009). Gene flow of Ceriops tagal (Rhizophoraceae) across the Kra Isthmus in the Thai Malay Peninsula. Botan. Stud. 50, 193–204.
Liao, P.-C., Hwang, S.-Y., Huang, S., Chiang, Y.-C., and Wang, J.-C. (2011). Contrasting demographic patterns of Ceriops tagal (Rhizophoraceae) populations in the South China Sea. Austr. J. Bot. 59, 523–532. doi: 10.1071/bt10290
Longhurst, A. R. (2007). Ecological Geography of the Sea. Oxford: Elsevier Academic Press. doi: 10.1016/B978-012455521-1/50002-4
Maguire, T. L., Edwards, K. J., Saenger, P., and Henry, R. (2000a). Characterisation and analysis of microsatellite loci in a mangrove species, Avicennia marina (Forsk.) Vierh. (Avicenniaceae). Theoret. Appl. Genet. 101, 279–285. doi: 10.1007/s001220051480
Maguire, T. L., Saenger, P., Baverstock, P., and Henry, R. (2000b). Microsatellite analysis of genetic structure in the mangrove species Avicennia marina (Forsk.) Vierh. (Avicenniaceae). Mol. Ecol. 9, 1853–1862. doi: 10.1046/j.1365-294x.2000.01089.x
Manez, K. S., Krause, G., Ring, I., and Glaser, M. (2014). The Gordian knot of mangrove conservation: disentangling the role of scale, services and benefits. Glob. Environ. Change Hum. Policy Dimens. 28, 120–128. doi: 10.1016/j.gloenvcha.2014.06.008
Manh, D. V., and Yanagi, T. (2000). A study on residual flow in the gulf of Tongking. J. Oceanogr. 56, 59–68. doi: 10.1023/A:1011162524466
Mantel, N. (1967). Detection of disease clustering and a generalized regression approach. Cancer Res. 27, 209–220.
Mitarai, S., Siegel, D. A., Watson, J. R., Dong, C., and McWilliams, J. C. (2009). Quantifying connectivity in the coastal ocean with application to the Southern California Bight. J. Geophys. Res. Oceans 114:21. doi: 10.1029/2008JC005166
Mitsch, W. J., Gosselink, J. G., Anderson, C. J., and Zhang, L. (2009). Wetland Ecosystems. Hoboken, NJ: John Wiley & Sons, Inc.
Nakajima, Y., Matsuki, Y., Lian, C. L., Fortes, M. D., Uy, W. H., Campos, W. L., et al. (2014). The kuroshio current influences genetic diversity and population genetic structure of a tropical seagrass, Enhalus acoroides. Mol. Ecol. 23, 6029–6044. doi: 10.1111/mec.12996
Nathan, R. (2006). Long-distance dispersal of plants. Science 313, 786–788. doi: 10.1126/science.1124975
Nei, M. (1978). Estimation of average heterozygosity and genetic distance from a small number of individuals. Genetics 89, 583–590.
Nei, M. (1987). Molecular Evolutionary Genetics. New York, NY: Columbia University Press, 512. doi: 10.7312/nei-92038
Ngeve, M. N., Van der Stocken, T., Menemenlis, D., Koedam, N., and Triest, L. (2016). Contrasting effects of historical sea level rise and contemporary ocean currents on regional gene flow of rhizophora racemosa in eastern atlantic mangroves. PLos One 11:e0150950. doi: 10.1371/journal.pone.0150950
Nguyen, H. H. (2014). The relation of coastal mangrove changes and adjacent land-use: a review in Southeast Asia and Kien Giang, Vietnam. Ocean Coast. Manag. 90, 1–10. doi: 10.1016/j.ocecoaman.2013.12.016
Ong, J. E., and Gong, W. K. (2013). Stucture, Function and Management of Mangrove Ecosystems. Okinawa: Tropical Timber Organization (ITTO).
Palumbi, S. R. (1994). Genetic divergence, reproductive isolation, and marine speciation. Annu. Rev. Ecol. Systemat. 25, 547–572. doi: 10.1146/annurev.es.25.110194.002555
Peakall, R., and Smouse, P. E. (2006). GenAlEx 6: genetic analysis in Excel. Population genetic software for teaching and research. Mol. Ecol. Notes 6, 288–295. doi: 10.1111/j.1471-8286.2005.01155.x
Petit, R. J., Duminil, J., Fineschi, S., Hampe, A., Salvini, D., and Vendramin, G. G. (2005). Comparative organization of chloroplast, mitochondrial and nuclear diversity in plant populations. Mol. Ecol. 14, 689–701. doi: 10.1111/j.1365-294X.2004.02410.x
Pil, M. W., Boeger, M. R. T., Muschner, V. C., Pie, M. R., Ostrensky, A., and Boeger, W. A. (2011). Postglacial north-south expansion of populations of Rhizophora Mangle (Rhizophoraceae) along the brazilian coast revealed by microsatellite analysis. Am. J. Bot. 98, 1031–1039. doi: 10.3732/ajb.1000392
Pons, O., and Petit, R. J. (1996). Measuring and testing genetic differentiation with ordered versus unordered alleles. Genetics 144, 1237–1245. doi: 10.1093/genetics/144.3.1237
Pritchard, J. K., Stephens, M., and Donnelly, P. (2000). Inference of population structure using multilocus genotype data. Genetics 155, 945–959.
Rambaut, A., Drummond, A. J., Xie, D., Baele, G., and Suchard, M. A. (2018). Posterior summarization in bayesian phylogenetics using tracer 1.7. Syst. Biol. 8:syy032. doi: 10.1093/sysbio/syy032
Sagarin, R. D., Gaines, S. D., and Gaylord, B. (2006). Moving beyond assumptions to understand abundance distributions across the ranges of species. Trends Ecol. Evol. 21, 524–530. doi: 10.1016/j.tree.2006.06.008
Sandoval-Castro, E., Muniz-Salazar, R., Enriquez-Paredes, L. M., Rios-mena-Rodriguez, R., Dodd, R. S., Tovilla-Hernandez, C., et al. (2012). Genetic population structure of red mangrove (Rhizophora mangle L.) along the northwestern coast of Mexico. Aquat. Bot. 99, 20–26. doi: 10.1016/j.aquabot.2012.01.002
Schaal, B. A., Leverich, W. J., and Rogstad, S. H. (1991). “Comparison of methods for assessing genetic variation in plant conservation biology,” in Genetics and Conservation of Rare Plants, eds K. E. Holsinger and D. A. Falk (New York, NY: Oxford University Press), 123–134.
Sheue, C. R., Liu, H. Y., and Yong, J. W. H. (2003). Kandelia obovata (Rhizophoraceae), a new mangrove species from Eastern Asia. Taxon 52, 287–294. doi: 10.2307/3647398
Steinke, T. D. (1986). A preliminary-study of buoyancy behavior in Avicennia marina propagules. South Afr. J. Bot. 52, 559–565. doi: 10.1016/S0254-6299(16)31492-2
Steinke, T. D., and Ward, C. J. (2003). Use of plastic drift cards as indicators of possible dispersal of propagules of the mangrove avicennia marina by ocean currents. Afr. J. Mar. Sci. 25, 169–176. doi: 10.2989/18142320309504007
Stellman, J. M., Stellman, S. D., Christian, R., Weber, T., and Tomasallo, C. (2003). The extent and patterns of usage of Agent Orange and other herbicides in Vietnam. Nature 422, 681–687. doi: 10.1038/nature01537
Stocken, T. V. D., AlisonWee, A. K. S., Ryck, D. J. R. D., Vanschoenwinkel, B., Friess, D. A., Dahdouh-Guebas, F., et al. (2019a). A general framework for propagule dispersal in mangroves. Biol. Rev. 94, 1547–1575. doi: 10.1111/brv.12514
Stocken, T. V. D., Carroll, D., Menemenlis, D., Simard, M., and Koedam, N. (2019b). Global-scale dispersal and connectivity in mangroves. Proc. Natl. Acad. Sci. U.S.A. 116, 915–922. doi: 10.1073/pnas.1812470116
Su, G. H., Huang, Y. L., Tan, F. X., Ni, X. W., Tang, T., and Shi, S. H. (2006). Genetic variation in Lumnitzera racemosa, a mangrove species from the Indo-West Pacific. Aquat. Bot. 84, 341–346. doi: 10.1016/j.aquabot.2006.01.001
Sugaya, T., Takeuchi, T., Yoshimaru, H., and Katsuta, M. (2002). Development and polymorphism of simple sequence repeat DNA markers for Kandelia candel (L.) Druce. Mol. Ecol. Notes 2, 65–66. doi: 10.1046/j.1471-8286.2002.00151.x
Sun, M., Wong, K. C., and Lee, J. S. Y. (1998). Reproductive biology and population genetic structure of Kandelia candel (Rhizophoraceae), a viviparous mangrove species. Am. J. Bot. 85, 1631–1637. doi: 10.2307/2446492
Takayama, K., Kajita, T., Murata, J., and Tateishi, Y. (2006). Phylogeography and genetic structure of Hibiscus tiliaceus – speciation of a pantropical plant with sea-drifted seeds. Mol. Ecol. 15, 2871–2881. doi: 10.1111/j.1365-294X.2006.02963.x
Takayama, K., Tamura, M., Tateishi, Y., Webb, E. L., and Kajita, T. (2013). Strong genetic structure over the American continents and transoceanic dispersal in the mangrove genus rhizophora (Rhizophoraceae) revealed by broad-scale nuclear and chloroplast DNA analysis. Am. J. Bot. 100, 1191–1201. doi: 10.3732/ajb.1200567
Tallmon, D. A., Koyuk, A., Luikart, G., and Beaumont, M. A. (2008). ONeSAMP: a program to estimate effective population size using approximate Bayesian computation. Mol. Ecol. Resour. 8, 299–301. doi: 10.1111/j.1471-8286.2007.01997.x
Tan, F. X., Huang, Y. L., Ge, X. J., Su, G. H., Ni, X. W., and Shi, S. H. (2005). Population genetic structure and conservation implications of Ceriops decandra in Malay Peninsula and North Australia. Aquat. Bot. 81, 175–188. doi: 10.1016/j.aquabot.2004.11.004
Tomlinson, P. B. (1986). Cambridge Tropical Biology Series: The Botany of Mangroves. New York, NY: Cambridge University Press.
Trajano, J. C., Gong, L., Sembiring, M., and Astuti, R. (2017). Marine environmental protection in the South China Sea: challenges and prospects part I. NTS Insight 17, 1–16.
Van der Stocken, T., and Menemenlis, D. (2017). Modelling mangrove propagule dispersal trajectories using high-resolution estimates of ocean surface winds and currents. Biotropica 49, 472–481. doi: 10.1111/btp.12440
Wang, W., Huang, J., and Mao, S. (1991). Modern Sedimentation of the Southern China Coastal Area. Beijing: Science Press.
Wee, A. K. S., Takayama, K., Asakawa, T., Thompson, B., Onrizal Sungkaew, S., et al. (2014). Oceanic currents, not land masses, maintain the genetic structure of the mangrove Rhizophora mucronata Lam. (Rhizophoraceae) in Southeast Asia. J. Biogeogr. 41, 954–964. doi: 10.1111/jbi.12263
Weir, B. S., and Cockerham, C. C. (1984). Estimating F-statistics for the analysis of population structure. Evolution 38, 1358–1370. doi: 10.1111/j.1558-5646.1984.tb05657.x
Wilson, G. A., and Rannala, B. (2003). Bayesian inference of recent migration rates using multilocus genotypes. Genetics 163, 1177–1191.
Yang, Y. C., Li, J. F., Yang, S. H., Li, X. N., Fang, L., Zhong, C. R., et al. (2017). Effects of Pleistocene sea-level fluctuations on mangrove population dynamics: a lesson from Sonneratia alba. BMC Evolut. Biol. 17:22. doi: 10.1186/s12862-016-0849-z
Ye, Y., Lu, C. Y., Wong, Y. S., and Tam, N. F. Y. (2004a). Diaspore traits and inter-tidal zonation of non-viviparous mangrove species. Acta Bot. Sin. 46, 896–906.
Ye, Y., Tam, N. F. Y., Wong, Y. S., and Lu, C. Y. (2004b). Does sea level rise influence propagule establishment, early growth and physiology of Kandelia candel and Bruguiera gymnorrhiza? J. Exp. Mar. Biol. Ecol. 306, 197–215. doi: 10.1016/j.jembe.2004.01.011
Yeh, F. C., and Boyle, T. J. B. (1997). Population genetic analysis of co-dominant and dominant markers and quantitative traits. Belg. J. Bot. 129:157.
Keywords: conservation, gene flow, genetic diversity, mangrove, ocean current, population genetic structure
Citation: Geng Q, Wang Z, Tao J, Kimura MK, Liu H, Hogetsu T and Lian C (2021) Ocean Currents Drove Genetic Structure of Seven Dominant Mangrove Species Along the Coastlines of Southern China. Front. Genet. 12:615911. doi: 10.3389/fgene.2021.615911
Received: 10 October 2020; Accepted: 09 February 2021;
Published: 08 March 2021.
Edited by:
Jian-Feng Mao, Beijing Forestry University, ChinaReviewed by:
Renchao Zhou, Sun Yat-sen University, ChinaCopyright © 2021 Geng, Wang, Tao, Kimura, Liu, Hogetsu and Lian. This is an open-access article distributed under the terms of the Creative Commons Attribution License (CC BY). The use, distribution or reproduction in other forums is permitted, provided the original author(s) and the copyright owner(s) are credited and that the original publication in this journal is cited, in accordance with accepted academic practice. No use, distribution or reproduction is permitted which does not comply with these terms.
*Correspondence: Chunlan Lian, bGlhbkBhbmVzYy51LXRva3lvLmFjLmpw; Jianmin Tao, dGFvamlhbm1pbkBuamF1LmVkdS5jbg==
†These authors have contributed equally to this work
Disclaimer: All claims expressed in this article are solely those of the authors and do not necessarily represent those of their affiliated organizations, or those of the publisher, the editors and the reviewers. Any product that may be evaluated in this article or claim that may be made by its manufacturer is not guaranteed or endorsed by the publisher.
Research integrity at Frontiers
Learn more about the work of our research integrity team to safeguard the quality of each article we publish.