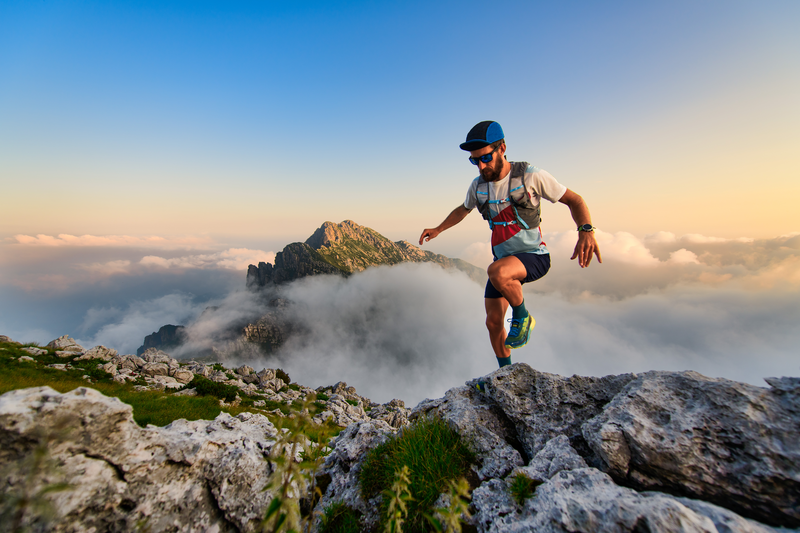
95% of researchers rate our articles as excellent or good
Learn more about the work of our research integrity team to safeguard the quality of each article we publish.
Find out more
REVIEW article
Front. Genet. , 02 February 2021
Sec. Stem Cell Research
Volume 12 - 2021 | https://doi.org/10.3389/fgene.2021.598855
This article is part of the Research Topic From Single Stem Cells to Organoids, Organ Repair, and Public Health View all 13 articles
Previous studies have reported an important role of c-kit in embryogenesis and adulthood. Activation of the SCF/KIT signal transduction pathway is customarily linked to cell proliferation, migration and survival thus influence hematopoiesis, pigmentation, and spermatogenesis. The role of c-kit in the liver is controversial, it is however argued that it is a double-edged sword in liver regeneration and diseases. First, liver c-kit+ cells, including oval cells, bile epithelial cells, and part of hepatocytes, participate in liver tissue repair by regenerating target cells according to the type of liver injury. At the same time, c-kit+ mast cells, act as immature progenitors in circulation, playing a critical role in liver fibrosis. Furthermore, c-kit is also a proto-oncogene. Notably, c-kit overexpression regulates gastrointestinal stromal tumors. Various studies have explored on c-kit and hepatocellular carcinoma, nevertheless, the intricate roles of c-kit in the liver are largely understudied. Herein, we extensively summarize previous studies geared toward providing hints for future clinical and basic research.
C-kit also known as CD117, is a type III receptor tyrosine kinase coded by the KIT gene. It has a stem cell growth factor (SCF) as its ligand, hence referred to as the stem cell growth factor receptor (SCFR) (Fujio et al., 1996). C-kit is encoded by white spotting (W) locus with more than 30 mutations while SCF is encoded by steel (Sl) locus also with natural mutations (Lev et al., 1994). In mice, total loss of c-kit or SCF function causes embryonic lethal, however, mice with one of these mutations (W or Sl mice) can survive.
Previous studies have demonstrated the fundamental role of c-kit in embryogenesis and adulthood. C-kit can be detected in several normal cells including, hematopoietic stem cells (HSCs), oval cells, intestinal cells of Cajal, germ cells, melanocytes among others. Activation of the SCF/c-kit signal transduction pathway is usually linked to cell proliferation, migration, and survival, and thus regulate crucial functions in hematopoiesis, pigmentation, and spermatogenesis (Besmer et al., 1993). The liver is the only visceral organ that possesses the capacity to regenerate. As long as 30–35% of the liver remains, the remnant liver will undergo hyperplasia until the normal liver is re-established. Approximately 20% of hepatocytes located mainly at the periportal and pericentral regions express c-kit located with a weak staining intensity participates in liver regeneration (Yushkov et al., 2011). Hepatic resident stem cells and bone marrow-derived stem cells (BMSCs), both of which express c-kit, can differentiate into various cells according to the specific injuries. Moreover, transplantation of c-kit positive (c-kit+) oval cells, and BMSCs is a potential factor of improving liver regeneration.
Under pathological conditions, excessive c-kit signaling is often associated with tumor formation and progression in gastrointestinal stromal tumors (GISTs), melanoma, small cell lung carcinoma, testicular carcinoma, etc. Imatinib, a relatively selective tyrosine inhibitor, is a target particularly of BCR-ABL, platelet-derived growth factor receptor (PDGFR), and c-kit. Imatinib is commonly used in the treatment of GIST. Piebaldism is a rare autosomal dominant disorder in which interstitial cells of Cajal are lost due to the loss-of-function mutation of c-kit (Giebel and Spritz, 1991). In liver, c-kit+ oval cells malignant transformation might be one of the possible mechanisms of hepatocarcinogenesis (Fang et al., 2004). Moreover, c-kit mutation or overexpression has been reported in hepatocellular carcinomas (HCC) (Potti et al., 2003; Yan et al., 2018). Besides, c-kit+ mast cells play a key role in fibrogenesis, specifically in cholestatic/biliary diseases associated with fibrosis.
Nonetheless, the complex roles of c-kit and liver are largely understudied, therefore, this review broadly summarizes previous research to provide clues for future basic and clinical studies.
Several cell surface markers have been utilized to identify stem/progenitor cells of the liver, such as c-kit, CD34, Thy-1 (Lemmer et al., 1998; Petersen et al., 1998; Baumann et al., 1999; Crosby et al., 2001). Even without a characteristic marker for liver progenitor cells, previous studies used c-kit as a potential marker for liver stem/progenitor cells (Fujio et al., 1994; Fujio et al., 1996; Baumann et al., 1999). Adult hepatic stem cells, also termed oval cells, express both SCF and c-kit (Fujio et al., 1994). While the proliferation of hepatocytes is inhibited, KIT-mediated signal transduction activates oval cells which then produce hepatocytes and bile epithelial cells (BECs) (Matsusaka et al., 1999). Besides, c-kit is expressed in oval cells and BECs (Fujio et al., 1996), whereas only 20% of hepatocytes located mainly at the periportal and pericentral regions express c-kit with a weak staining intensity (Yushkov et al., 2011). Moreover, liver sinusoidal endothelial cells (LSECs) are known to express c-kit (Luna et al., 2004). On the other hand, c-kit can be detected in early hematopoietic cells, mast cells, and dendritic cells. And c-kit+ mast cells are closely related to primary sclerosing cholangitis (PSC) (Metcalfe et al., 1997; Ishii et al., 2005; Ray et al., 2010). Kupffer cells and hepatic stellate cells are negative for c-kit (Ikarashi et al., 2013; Fu et al., 2015).
The liver is the only visceral organ with the capacity to regenerate. Liver c-kit+ cells, including part of hepatocytes, BECs, and oval cells participate in liver regeneration by differentiating into different types of cells depending on the type of the injury. Moreover, c-kit+ hematopoietic stem/progenitor cells (HSCs/HPCs) participate in liver regeneration. SCF/KIT signal transduction system regulates cell proliferation and survival. This review explains the relationship between c-kit and liver regeneration based on different types of cells (Figure 1).
Figure 1. The relationship between c-kit and different type of cells in liver regeneration and cancer. (1) C-kit express in 20% hepatocytes. After acute injury, solubilized SCF released and interact with c-kit, leading surrounding hepatocytes prolifer8ation. (2) In young rats after BDL, SCF bind to c-kit, leading to activation of the MAPK pathway; finally, BECs proliferation. (3) C-kit play a vital role in HCC. On the one hand, oval cells transformed to LCSCs are related with the c-kit gene overexpression. On the other hand, c-kit might associate with HCC by participating in angiogenesis. PH, Partial Hepatectomy; BEC, Bile Epithelial Cell; BDL, Bile Duct Ligation; 2AAF/PH, 2-Acetylaminofluorene/Partial Hepatectomy; LCSC, Liver Cancer Stem Cell; HCC, Hepatocellular Carcinoma.
Stem cell growth factor is localized in hepatocytes surrounding the BECs. And c-kit expressed with a weak staining intensity in 20% of hepatocytes located mainly at the periportal and pericentral regions (Yushkov et al., 2011).
Partial hepatectomy (PH) is the most common model used to study liver regeneration. After PH, mature hepatocytes proliferation leads to liver regeneration. Following PH, there is a significant decline in liver SCF levels and an increase in serum SCF levels during the first 24 h (Ren et al., 2003). The level of liver SCF mRNA was upregulated from the 24th hour to the 7th day (Meng et al., 2012). The number of c-kit+ hepatocytes increased after PH, including medium and high staining intensity (Yushkov et al., 2011). In addition, exogenous SCF promotes the proliferation of primary hepatocytes in humans and mice (Meng et al., 2012). Moreover, a study by Ren et al. linked the interleukin-6 (IL-6) enzyme and tumor necrosis factor-α (TNF-α) with the SCF/C-kit in mice in 70% PH model. Results suggested that IL-6 stimulates the production and release of SCF, then SCF interacts with c-kit in activating the signal transducer and activator of transcription 3 (stat3) signal pathway thereby inducing hepatocyte proliferation (Ren et al., 2003; Figure 2). Besides, TNF-α-induced hepatocyte proliferation was partially mediated via SCF/c-kit, and the SCF-induced hepatocyte proliferation might also be mediated by the stat3 signal pathway (Ren et al., 2008; Figure 2). The possible mechanism occurs after acute injury where solubilized SCF is released and interact with c-kit, causing proliferation of the surrounding hepatocytes.
Figure 2. A scheme showing the SCF/KIT signal transduction. (1) SCF/KIT function in the APAP model might be mainly through increasing proliferation of hepatocyte and inhibiting hepatocyte apoptosis. Exogenous SCF activating c-kit and Akt, results in an increase in Bcl-2 protein and inhibiting hepatocyte apoptosis. (2) IL-6 stimulates the production and release of SCF, then SCF interacts with c-kit in activating the stat3 signal pathway thereby inducing hepatocyte proliferation. (3) Both SCF and c-kit are up-regulated in BECs in young rats after BDL, SCF binds to c-kit, causing the activation of the Ras/Raf/MAPK pathway, hence the proliferation of bile epithelial cells.
Acetaminophen or Tylenol overdose has become the most prevalent cause of acute liver failure in the United States (Larson et al., 2005). Overdose produces serious acute liver toxicity, leading to hepatocyte necrosis, acute liver failure, and even death. Acetaminophen (APAP) model is a widely used animal experimental model of drug-induced hepatic injury. Data from an examination by Hu and Colletti et al. on the c-kit and SCF expression of C57BL/6J mice, shows that c-kit mRNA increase begins at 6 h and peaks after 48 h of overdose APAP treated, while SCF mRNA increase begins at 6 h and peaks at 16 h post-administration. Treatment with exogenous SCF significantly reduces the mortality of mice in 750 mg/kg APAP model. These findings suggest that SCF/KIT’s function in the APAP model might be mainly through increasing proliferation of hepatocyte and inhibiting hepatocyte apoptosis. Exogenous SCF activating c-kit and Akt, results in an increase in B cell lymphoma-2 (Bcl-2) protein and inhibiting hepatocyte apoptosis (Hu and Colletti, 2008; Figure 2). In contrast, inhibiting c-kit will increase the mortality of the lethal dose APAP-treated model (Nassar et al., 2009; Shaker, 2014). In summary, these results indicate that c-kit is an important molecule in liver recovery according to the APAP model. Activation of the SCF/KIT system causes an increase in hepatocyte proliferation and a decrease in hepatocyte apoptosis.
Studies argue that the proliferation of BEC is associated with c-kit. A study by Fujio et al. (1996) reported that SCF and c-kit are co-expressed in BEC during the embryonic and adult stages of rat’s liver. Elsewhere, Omori et al. demonstrated that BECs in young liver react differently with adult liver in the bile duct ligation (BDL) model. In the liver of a young rat with 5 weeks of age, there is an upregulation in the expression of SCF and c-kit after BDL is performed. But in the adult liver of rats after BDL, no upregulation of SCF and c-kit is observed. And the pattern of BECs in young liver response to bile stasis is similar to that of the early stage of oval cell activation (Omori et al., 1997). Evidence reported by Satake et al. show that both SCF and c-kit are up-regulated in BECs in young rats after BDL, SCF binds to c-kit, causing the activation of the Ras/Raf/mitogen-activated protein kinase (MAPK) pathway, hence the proliferation of bile epithelial cells (Figure 2). Nevertheless, in the adult rats, KIT-mediated signal transduction plays a mirror role due to the low expression of SCF/c-kit (Satake et al., 2003).
Oval cells are the progenitor cells of the liver with c-kit as one of its markers (Crosby et al., 2001). Normally, after PH, mature hepatocytes proliferate causing liver regeneration. However, if PH is performed when the replicative ability of mature hepatocytes is impaired, there will occur proliferation and differentiation of oval cells (Thorgeirsson, 1996). PH integrated with 2-acetylaminofluorene (2-AAF)/PH is the model utilized to investigate the liver regeneration capability via oval cells. Further reports by Fujio et al. (1994) showed that in the embryonic and adult rats, both SCF and c-kit were expressed in oval cells. After 2-AAF/PH, the level of SCF transcripts increased within 12 h and reached a peak at day 4, the level of c-kit mRNA was upregulated from 12th hour to 11th day (Fujio et al., 1994). Additionally, Matsusaka et al. used Ws/Ws rats with 2-AAF/PH model to evaluate the role of SCF/KIT in the development of oval cells. Their results demonstrated that in at least one rat under the 2-AAF/PH model, KIT-mediated signal transduction activates the oval cells. However, in the proliferative activity and determining the phenotype of oval cells, the SCF/KIT signal transduction system plays a minor role (Matsusaka et al., 1999).
Circulating endothelial progenitors (CEPs) originated from bone marrow with markers c-kit or CD133. By combining bone marrow transplant with 70% PH, the investigators concluded that CEPs contribute to liver regeneration by differentiating into liver vasculature, and exogenous VEGF accelerates liver regeneration by increasing the recruitment of CEPs in 70% PH model (Beaudry et al., 2007; Figure 3A). Moreover, a study by Si et al. found that there were relatively more CD45+Lin–C-Kit+ HSCs/HPCs recruited in wide type (WT) liver than chemokine (C-C motif) receptor2 knock out (CCR2–/–) after APAP-induced injury. More importantly, after administration with exogenous CD45+Lin–C-Kit+ HSCs/HPCs, WT had greater resolution of APAP-induced injury than CCR2–/– mice, and these HSCs/HPCs express the macrophage M2 (repair phenotype) genes characteristic (Si et al., 2010; Figure 3B).
Figure 3. The role of c-kit+ circulating progenitor cells in liver diseases. (A) By combined bone marrow transplant with 70% PH, the investigators conclude that CEPs contribute to liver regeneration by differentiating into liver vasculature. (B) In APAP model, c-kit+ HSCs/HPCs were recruited into liver and these HSCs/HPCs express the macrophage M2 (repair phenotype) genes characteristic. (C) Cholestatic/biliary diseases-associated fibrosis. Damaged BECs express and secrete SCF to recruit mast cells, then mast cells migrate to the damage area and contribute to fibrogenesis. C-kit+ mast cells play a key role in fibrogenesis mainly through expressing fibrosis-associated factors including tryptase, b-FGF and TNF-α. HSCs/HPCs, Hematopoietic Stem/Progenitor Cells; CEPs, Circulating Endothelial Progenitors.
In summary, liver regeneration is a complex process, with multiple mechanisms and pathways, current literatures suggest that c-kit regulates liver regeneration. On the one hand, activation of the SCF/KIT signal transduction system leads to hepatocytes proliferation and apoptosis inhibition, BECs proliferation and oval cells activation. On the other hand, c-kit+ progenitor cells contribute to liver regeneration by differentiating into liver vasculature or alternatively activated macrophages.
C-kit is expressed on HSCs/HPCs and mast cells, and several liver diseases are relevant to c-kit. A strong relationship was observed between c-kit and primary liver cancer, besides, c-kit+ mast cells also participate in fibrogenesis. This section extensively summarizes the relationship.
Liver cancer is the fourth leading cause of cancer death among sexes combined globally (Bray et al., 2018). Evidence shows that liver stem/progenitor cells are the potential source of HCC, also called liver cancer stem cells (LCSCs) (Dumble et al., 2002; Fujii et al., 2008). It is believed that the liver stem cells transformed into LCSCs are linked with the overexpression of the c-kit gene (Chen et al., 2007; Yan et al., 2018). The SCF/c-kit signal transduction system participates in the activation and proliferation of oval cells. On the other hand, overexpression of c-kit is relevant with micro-vessel density, therefore, c-kit might be associated with HCC by participating in angiogenesis (Yan et al., 2018). Concerning the hepatitis B virus (HBV) or hepatitis C virus (HCV) induced HCC, c-kit is involved. PreS1 protein of HBV stimulates the appearance and self-renewal of LCSCs by activating the expression of c-kit and is confirmed in human hepatoma cells and HCC tissues (Liu et al., 2017). And in HCV infection patients, c-kit overexpression is observed (Kwon et al., 2015; Nazzal et al., 2020). Data shows that HCV core protein upregulates the expression of c-kit in hepatocytes, inducing the epithelial-mesenchymal transition, and extends the life span of cells (Kwon et al., 2015). Further, evidence recommends that liver stem/progenitor cells are the potential source of intrahepatic cholangiocarcinoma (ICC) and SCF/c-kit system might contribute to ICC by promoting cell proliferation and survival (Nomoto et al., 2006; Komuta et al., 2008; Mansuroglu et al., 2009b). Again, several lines of evidence attest that liver stem/progenitor cells are the potential source of HCC-ICC (Tanaka et al., 2005; Zhang et al., 2008). And the recent classification of digestive system tumor (5th edition) by the World Health Organization (WHO) also agrees with this concept. A different follow-up model indicates that the recurrence of HCC-ICC after hepatectomy is associated with the expression of c-kit in both tumor and non-tumor livers (Cai et al., 2012).
HCC is the most prevalent primary liver cancer, accounting for 85∼90% of primary liver cancers. However, the positive rate of HCC has not been precisely clarified. A research executed by Chung et al. (2005) examined the protein expression of c-kit in 86 human HCC specimens. The results demonstrated that among the 86 specimens, 22 (25.6%) were positive. According to an article published by Yan et al. (2018), the percentage of positive c-kit expression is 48.1% in 206 HCC cases. Nevertheless, Becker et al. (2007) collected 258 human HCC samples and c-kit immunohistochemistry was performed, results reported that only 6 (2.3%) samples were positive. There are many reasons attributed to these results among them, antibody specificity (Went et al., 2004), cellular heterogeneity, different stages of cell differentiation, etc. In summary, more cases need to be included and more detailed etiology should be classified. Epidemiological data shows that ICC accounts for 15% of primary liver cancer, second to the HCC (Shaib et al., 2005). Clinicopathological study on c-kit positive immunohistochemistry staining of human ICC sample (1/32), suggesting that ICC originates from hepatic stem cell (Liu et al., 2004). Certainly, only one c-kit positive among 32 samples could not clarify the origin of ICC, implying that other mechanisms might also exist. Therefore, more cases need to be collected in future studies before a single-cell RNA sequence can be performed, this might solve the problem of origin. A study by Xu et al. (2018) showed that NCAM+ c-kit+ subset cells in RBE cell lines might have properties of hepatic progenitor cells. And NCAM combined with c-Kit might be a valuable marker for isolating and purifying ICC stem/progenitor cells. Therefore, c-kit can be a candidate marker of ICC stem/progenitor cells used in single cell RNA sequence in the future. HCC-ICC is a rare subtype of primary liver cancer with poor prognosis, comprising <1% of all liver carcinomas, and epidemiological data shows it accounts for 1.6–6.5% of surgically resected primary liver cancer (Koh et al., 2005; Yu et al., 2011; Akiba et al., 2013). Unlike the low c-kit positive rate of HCC, the presence of the c-kit in HCC-ICC is 71.4% (10/14), which might explain the poor prognosis of HCC-ICC (Yu et al., 2011).
In addition, it is reported that there is positive feedback in transforming growth factor β (TGF-β) and c-kit, which induce carcinogenesis in HepG2 cells. TGF-β activated SMAD2 transcriptionally induces SCF expression, SCF expression and secretion result in stimulation of the c-kit receptor, followed by activation of JAK1/STAT3 signaling. pTyr705STAT3 trans-locates to the nucleus where it binds directly to the TGF-β ligand gene, positively regulating its expression. Following activation of secreted TGF-β precursor, it can activate the TGF-β receptor. Disruption of the TGF-β/KIT signaling loop on the level of the SCF/STAT3 axis restores TGF-β tumor suppressor function by inhibition of epithelial-mesenchymal transition (EMT), tumor cell migration, and invasion, and restoration of its cell cycle inhibitory functions (Rojas et al., 2016). Besides, a recent study showed that in HCV-infected patients, their c-kit gene mutation in exons 9 but not 11, are often bound up with high- viremia HCV. And these patients might be resistant to TGF-β, thus promoting the development of HCC (El-Houseini et al., 2019). These studies indicate that TGF-β/KIT signaling plays an important role in the development of HCC and is a key target for the prevention and treatment of HCC.
Hepatic fibrosis is a wound healing process involving multiple cell types. There are many causes of liver fibrosis, including viral, alcoholic, cholestasis, etc. The role of c-kit in liver fibrosis is quite complex. Mast cells increase in liver fibrosis, but decrease in other chronic liver diseases, suggesting that mast cells take part in fibrogenesis particularly in cholestatic/biliary diseases (Weiskirchen et al., 2019).
Most of hematopoietic cells lose expression of c-kit when they mature, except mast cells and dendritic cells. Mast cells are derived from the bone marrow, and they act as immature progenitors in circulation. In the liver, mast cells are primarily located in the periportal area. Primary sclerosing cholangitis (PSC) or primary biliary cirrhosis is an autoimmune disease characterized by infiltration of mast cell (Nakamura et al., 1997; Ishii et al., 2005). In contrast, there is no significant increase in acute viral or drug-induced hepatitis (Yamashiro et al., 1998).
PSC is a disease characterized by infiltration of mast cell, biliary damage, and liver fibrosis. SCF/KIT signaling plays a critical role in the development of mast cell, survival and homing. Animal models have shown that inhibiting the c-kit system releases allergic reactions in the lungs (Berlin et al., 2004). In the liver, data suggests that c-kit+ mast cells play a key role in fibrogenesis from the early stage of PSC (Ishii et al., 2005; Meadows et al., 2019). First, damaged BECs express and secrete SCF to recruit mast cells. Secondly, mast cells migrate to the damaged area and cause fibrogenesis. Therefore, c-kit+ mast cells contribute to fibrogenesis primarily through expressing fibrosis-associated factors including tryptase, basic fibroblast growth factor (b-FGF), and TNF-α (Kanbe et al., 2000; Gaca et al., 2002; Figure 3C). BDL-induced biliary hyperplasia, hepatic injury, and fibrosis are reduced in KitW–sh mice, which are mast cell deficient (Hargrove et al., 2017). As for ameliorating progression of PSC, targeting mast cell infiltration might be an efficient option. Furthermore, in systemic mastocytosis, mastocytosis-derived extracellular vesicles transfer c-kit to liver stellate cells, causing activation, proliferation, cytokine production, and differentiation of liver stellate cells (Kim et al., 2018). This might be an alternative mechanism of c-kit+ mast cells-induced fibrogenesis.
Additionally, mast cells take part in the progress of biliary atresia (BA). It is reported that the increased mast cells adversely affects liver function, perhaps through type I allergic reaction (Uddin Ahmed et al., 2000). However, there is no specific study about the SCF/c-kit system and BA. Given the relationship between mast cells and BA, SCF/c-kit system should be considered.
The number of mast cells in other chronic liver diseases-associated fibrosis is increased, and the intensity of c-kit immunostaining is slightly higher in cirrhotic non-tumorous liver than in non-cirrhotic non-tumorous liver, but the relationship between c-kit and fibrosis has not been extensively evaluated (Mansuroglu et al., 2009a). C-kit expressed in fibroblasts and SCF/c-kit plays a vital role in scar pathogenesis, thus we can use a c-kit selective inhibitor to block it (Mukhopadhyay et al., 2011).
In summary, the role of c-kit in liver fibrosis is obscure. In cholestatic/biliary diseases-associated fibrosis, c-kit+ mast cells regulate fibrogenesis. However, in other chronic liver diseases-associated fibrosis, despite the increase of mast cells, the relationship between c-kit and fibrosis is largely understudied. Therefore, further studies are necessary to elaborate on the relationship between c-kit and hepatic fibrosis.
The roles of c-kit+ cells in chronic hepatitis B and C have been described in HCC (Kara et al., 2008; Kwon et al., 2015; Liu et al., 2017; Nazzal et al., 2020). Also, it is reported that there is an increase of mast cells in alcoholic hepatitis, but reports on the relationship between c-kit and alcoholic hepatitis are insufficient (Farrell et al., 1995). Alcoholic hepatitis impairs intestinal barrier and activate the mast cell causing fibrogenesis (Ferrier et al., 2006). Besides, a study by Hisada et al. (2017) mentioned that the percentage of c-kit+ cells was dramatically decreased in alcohol-fed rats compared to non-alcohol-fed rats. These findings indicate that BMSCs might be damaged by the consumption of alcohol. Nonetheless, the relationship between c-kit+ cells and alcohol has not been fully elucidated, hence this represents an important topic for future research.
In summary, c-kit is relevant to primary liver cancer. It is believed that liver stem cells transformed into LCSCs are linked with the overexpression of the c-kit gene, causing liver cancer. Besides, c-kit+ mast cells participate in fibrogenesis particularly in cholestatic/biliary diseases. C-kit+ mast cells contribute to fibrogenesis primarily through expressing fibrosis-associated factors.
Few reports are suggested that c-kit can be used as a diagnostic factor in liver diseases. For instance, Kara et al. (2008) recommended that c-kit can be used as an early diagnostic factor for HBV-related HCC. However, it is unclear whether c-kit can be used as an indicator in HCC caused by other factors. Furthermore, it is reported that c-kit+ mast cells increase after liver allograft rejection (El-Refaie and Burt, 2005), but the increased c-kit+ mast cells cannot distinguish rejection from recurrent HCV infection in transplantation of liver (Doria et al., 2006). Seemingly, c-kit is a good prognostic parameter in several diseases. First, one article has mentioned that c-kit can be used as a prognostic factor for HCC (Chung et al., 2005). Moreover, Yan et al. suggested that c-kit is an independent prognostic indicator for HBV-related HCC patients. In addition, Kaplan–Meier survival analysis shows that the c-kit expression was linked to poor disease-free survival (DFS) (P < 0.001) in HBV-related HCC patients (Yan et al., 2018). Besides, in a cohort of 70 HCC-ICC patients who underwent resection for treatment, overall survival (OS) and DFS were associated with expression of c-kit in both tumor and non-tumor livers (Cai et al., 2012). Secondly, the increased number of c-kit+ mast cells in chronic HCV patients might be used as an indicator of liver fibrosis (Koruk et al., 2011). Thirdly, it is reported that the number of mast cells adversely affects liver function in biliary atresia, but the authors did not stain mast cells with c-kit. Therefore, more patients should be enrolled when conducting c-kit+ mast cell statistics (Uddin Ahmed et al., 2000). Finally, due to the role of c-kit+ mast cells in fibrogenesis among PSC patients and animal models, the number of c-kit+ mast cells might reflect the prognosis of PSC patients (Ishii et al., 2005; Meadows et al., 2019). However, only 4 samples were observed, therefore more clinical data need to be counted.
Oval cell transplantation after liver transplantation might be useful for liver regeneration. A study by Li et al. (2013) isolated oval cells, of which 99.8% were positive for c-kit. After primary culture, oval cells were injected into the rats who underwent liver transplantation. Data shows that compared to controls, oval cell transplantation significantly improved the liver function, prolonged median survival time and reduced acute allograft rejection (Li et al., 2013).
BMSCs are closely related to liver fibrosis. In carbon tetrachloride (CCl4)-induced liver fibrosis mice, the differentiation potential of circulation of endothelial progenitor cells (EPCs) is attenuated due to the impairment of the endothelial lineage differentiation potential of bone marrow-C-kit+, Sca-1+, and Lin–(BM-KSL) cells (Shirakura et al., 2011). Transplantation of HSCs has been reported to be effective in mice. Some authors proved that CCl4 treated liver fibrosis mice administered with the HSCs (Lin–Sca-1+c-kit+) had less fibrosis and better liver function compared with the group not inject (Cho et al., 2011). Moreover, King et al. arrived at the same conclusion and pointed out that HSCs (Lin–Sca-1+c-kit+) participate in anti-fibrotic by promoting the recruitment of endogenous macrophages and neutrophils. And the retention of HSCs can be increased by reducing sphingosine-1-phosphate (S1P) signaling to antifibrosis (King et al., 2017). Furthermore, it has been reported that in CCl4-induced liver injury, transplantation of very small embryonic-like stem cells (VSELs), which express c-kit, directly into the liver can significantly lower the levels of serum alanine aminotransferase (ALT) and aspartate aminotransferase (AST) (Chen et al., 2015). Transplantation of BMSCs has been reported to be effective in humans. Research by Terai et al. reported 9 patients with liver cirrhosis that received the therapy of autologous bone marrow cell infusion (ABMI). The cell characteristics were confirmed by fluorescence-activated cell sorting analysis (CD34+, CD45+, c-kit+). And after infusing, significantly improved serum albumin levels and total protein were witnessed. Besides, significant improvements in Child-Pugh scores were observed (Terai et al., 2006; Figure 4).
Figure 4. The role of c-kit+ cell in stem cell therapy. (A) CCl4 treated liver fibrosis mice administered with the HSCs (Lin–Sca-1+c-kit+) had less fibrosis and better liver function compared with the group not inject. (B) Compare to controls, oval cells transplantation significantly improved the liver function, prolonged median survival time and reduced acute allograft rejection. (C) After infusing, significantly improved serum albumin levels and total protein were observed. ABMI, Autologous Bone Marrow cell Infusion.
By and large, we can speculate that c-kit+ oval cell transplantation might be useful in therapeutic liver regeneration after orthotopic liver transplantation. And c-kit+ BMSCs or HSCs transplantation is a promising treatment of patients with liver cirrhosis. Nevertheless, considering the role of c-kit in liver cancer, further studies are essential to evaluate the relationship between c-kit+ cell, hepatic fibrosis, and liver cancer. Additionally, we summarized the clinical implications of c-kit in liver (Table 1).
Besides its implication in prognosis and stem cell therapy, overexpression of c-kit in liver primary cancer has potential therapeutic implications because it can inhibit the kinase activity of c-kit. Imatinib mesylate (STI-571 or Gleevecs) is a relatively selective tyrosine inhibitor of BCR-ABL, PDGF-R, and c-kit, which is usually used in the treatment of chronic myelocytic leukemia (CML) and GIST. Animal experiments point out that imatinib treatment can inhibit c-kit-expressing liver progenitor cells proliferation and early fibrosis induced by short-term choline-deficient, ethionine-supplemented (CDE) diet. In the long run, imatinib treatment can reduce the degree of fibrosis and significantly inhibit the formation of tumor (Knight et al., 2008). Furthermore, Nazzal et al. recently showed that HCC-patient-derived xenograft (PDX) tumors respond well following imatinib treatment. Treatment of HCC-PDX xenograft tumor-bearing mice with imatinib significantly reduced tumor growth and c-kit downstream molecules expression (Nazzal et al., 2020). In clinical, Becker et al. (2007) discovered that the positive rate of c-kit is 2.3% and that there was no role for imatinib. Similarly, in a clinical phase II trial of imatinib, Eckel et al. (2005) found no positive case for c-kit among 15 HCC patients, and imatinib showed no therapeutic effect. Another phase II study of imatinib in unresectable HCC concluded that imatinib is unsuitable as a monotherapy in the treatment of unresectable HCC (Lin et al., 2008). Interestingly, Ramadori et al. (2004) effectively treated one HCC patient with imatinib. Sorafenib is a multi-kinase inhibitor that is broadly applied in unresectable HCC as a first-line treatment. C-kit is among the molecular targets of sorafenib. In one case report, a patient diagnosed with HCC-ICC and highly c-kit positive, responded to sorafenib (Seino et al., 2014). As we know, sorafenib targets c-kit, and the high concentration of soluble c-kit group has a greater response to sorafenib than the low concentration group (Llovet et al., 2012). Since primary liver tumors are regulated by multiple signaling pathways, imatinib is hardly used in patients with HCC. But going by the results of sorafenib and imatinib, the c-kit pathway should be of concern. Additionally, we summarized c-kit signal involved drugs and clinical trials in unresectable hepatocellular carcinoma(uHCC)(Table 2).
On the other hand, due to the role of c-kit+ mast cells in cholestatic/biliary diseases-associated fibrosis, c-kit receptor tyrosine inhibitor can be used to prevent fibrosis. It is reported that a c-kit/PDGF receptor tyrosine kinase inhibitor-masitinib, plays an effective role in the treatment of corticosteroid-dependent asthma by inhibiting c-kit+ mast cells (Humbert et al., 2009). However, to validate these findings, further in vitro and in vivo experiments are essential.
The role of c-kit in the liver is conflicting, it is a two-edged sword in liver regeneration and diseases. First, as a liver stem cell marker, c-kit+ cells, such as oval cells, BECs, and part of hepatocytes are closely linked to liver regeneration. And c-kit signaling participates in cell proliferation, migration, and survival based on different types of cells. At the final stage of chronic liver cirrhosis, liver transplantation is the most effective treatment. But there are many challenges such as limited donors, surgical complications, etc. And c-kit+ BMSCs or HSCs transplantation is a potential treatment of patients in the final stage of chronic liver cirrhosis. Nevertheless, further research is crucial to explore the relationship between c-kit+ cell, hepatic fibrosis, and liver cancer. Secondly, mast cells express c-kit, and damaged BECs express and secrete SCF to recruit c-kit+ mast cells, resulting in fibrogenesis. Eventually, c-kit also is a proto-oncogene, and its overexpression is associated with primary liver cancer. Sorafenib, a multi-kinase inhibitor with c-kit included, is used as a first-line treatment for HCC. Since primary liver tumors are regulated by multiple signaling pathways, imatinib is hardly used in patients with HCC. But going by the results of sorafenib and imatinib, the c-kit pathway should be of concern.
Conventional research methods cannot reveal the dynamic process of stem cell differentiation in vivo, but genetic lineage tracing can address this challenge. Previously, we used Kit-CreERxRosa26-RFP mouse to trace the role of c-kit+ stem/progenitor cells in vascular injury-induced neointimal lesions, and found that c-kit+ cells are majorly converted to immune-inflammatory cells during neointima formation (Chen et al., 2018). Another laboratory utilized KitMerCreMer/+x Rosa26-eGFP lineage-tracing mouse to examine endothelial cell formation in the heart (Vagnozzi et al., 2020). Furthermore, several laboratories used single-cell RNA sequences to reveal the cell heterogeneity of the liver, including all liver cells, immune cells, and cancer stem cells (Zheng et al., 2018; Aizarani et al., 2019; Zhang et al., 2019). Recently, lineage-tracing combine RNA sequence analysis has revealed the mechanisms of endothelial repair by progenitors (Deng et al., 2020). In future research, this technology can be adopted in the liver to expose the role of c-kit+ cells in various liver diseases.
WW wrote the manuscript and prepared figures. MZ, YL, and LS provided the expert comments and edited the manuscript. All authors reviewed the manuscript.
This work was supported by the State S&T Project of 13th Five Year (No. 2018ZX10302206), the National Natural Science Foundation of China (No. 81871646), and the Key R&D Projects of Zhejiang Province (2018C03019).
The authors declare that the research was conducted in the absence of any commercial or financial relationships that could be construed as a potential conflict of interest.
Aizarani, N., Saviano, A., Sagar, M. L., Durand, S., and Herman, J. S. (2019). A human liver cell atlas reveals heterogeneity and epithelial progenitors. Nature 572, 199–204. doi: 10.1038/s41586-019-1373-2
Akiba, J., Nakashima, O., Hattori, S., Tanikawa, K., Takenaka, M., Nakayama, M., et al. (2013). Clinicopathologic analysis of combined hepatocellular-cholangiocarcinoma according to the latest WHO classification. Am. J. Surg. Pathol. 37, 496–505. doi: 10.1097/PAS.0b013e31827332b0
Baumann, U., Crosby, H. A., Ramani, P., Kelly, D. A., and Strain, A. J. (1999). Expression of the stem cell factor receptor c-kit in normal and diseased pediatric liver: identification of a human hepatic progenitor cell? Hepatology 30, 112–117. doi: 10.1002/hep.510300140
Beaudry, P., Hida, Y., Udagawa, T., Alwayn, I. P., Greene, A. K., Arsenault, D., et al. (2007). Endothelial progenitor cells contribute to accelerated liver regeneration. J. Pediatr. Surg. 42, 1190–1198. doi: 10.1016/j.jpedsurg.2007.02.034
Becker, G., Schmitt-Graeff, A., Ertelt, V., Blum, H. E., and Allgaier, H. P. (2007). CD117 (c-kit) expression in human hepatocellular carcinoma. Clin. Oncol. 19, 204–208. doi: 10.1016/j.clon.2006.12.009
Berlin, A. A., Lincoln, P., Tomkinson, A., and Lukacs, N. W. (2004). Inhibition of stem cell factor reduces pulmonary cytokine levels during allergic airway responses. Clin. Exp. Immunol. 136, 15–20. doi: 10.1111/j.1365-2249.2004.02404.x
Besmer, P., Manova, K., Duttlinger, R., Huang, E. J., Packer, A., Gyssler, C., et al. (1993). The kit-ligand (steel factor) and its receptor c-kit/W: pleiotropic roles in gametogenesis and melanogenesis. Dev. Suppl. 119, 125–137.
Bray, F., Ferlay, J., Soerjomataram, I., Siegel, R. L., Torre, L. A., and Jemal, A. (2018). Global cancer statistics 2018: GLOBOCAN estimates of incidence and mortality worldwide for 36 cancers in 185 countries. CA Cancer J. Clin. 68, 394–424. doi: 10.3322/caac.21492
Bruix, J., Qin, S., Merle, P., Granito, A., Huang, Y. H., Bodoky, G., et al. (2017). Regorafenib for patients with hepatocellular carcinoma who progressed on sorafenib treatment (RESORCE): a randomised, double-blind, placebo-controlled, phase 3 trial. Lancet 389, 56–66. doi: 10.1016/S0140-6736(16)32453-9
Cai, X., Zhai, J., Kaplan, D. E., Zhang, Y., Zhou, L., Chen, X., et al. (2012). Background progenitor activation is associated with recurrence after hepatectomy of combined hepatocellular-cholangiocarcinoma. Hepatology 56, 1804–1816. doi: 10.1002/hep.25874
Chen, L., Shen, R., Ye, Y., Pu, X. A., Liu, X., Duan, W., et al. (2007). Precancerous stem cells have the potential for both benign and malignant differentiation. PLoS One 2:e293. doi: 10.1371/journal.pone.0000293
Chen, Q., Yang, M., Wu, H., Zhou, J., Wang, W., Zhang, H., et al. (2018). Genetic lineage tracing analysis of c-kit(+) stem/progenitor cells revealed a contribution to vascular injury-induced neointimal lesions. J. Mol. Cell Cardiol. 121, 277–286. doi: 10.1016/j.yjmcc.2018.07.252
Chen, Z. H., Lv, X., Dai, H., Liu, C., Lou, D., Chen, R., et al. (2015). Hepatic regenerative potential of mouse bone marrow very small embryonic-like stem cells. J. Cell Physiol. 230, 1852–1861. doi: 10.1002/jcp.24913
Cheng, A. L., Kang, Y. K., Chen, Z., Tsao, C. J., Qin, S., Kim, J. S., et al. (2009). Efficacy and safety of sorafenib in patients in the Asia-Pacific region with advanced hepatocellular carcinoma: a phase III randomised, double-blind, placebo-controlled trial. Lancet Oncol. 10, 25–34. doi: 10.1016/S1470-2045(08)70285-7
Cheng, A. L., Thongprasert, S., Lim, H. Y., Sukeepaisarnjaroen, W., Yang, T. S., Wu, C. C., et al. (2016). Randomized, open-label phase 2 study comparing frontline dovitinib versus sorafenib in patients with advanced hepatocellular carcinoma. Hepatology 64, 774–784. doi: 10.1002/hep.28600
Cho, K. A., Lim, G. W., Joo, S. Y., Woo, S. Y., Seoh, J. Y., Cho, S. J., et al. (2011). Transplantation of bone marrow cells reduces CCl4 -induced liver fibrosis in mice. Liver Int. 31, 932–939. doi: 10.1111/j.1478-3231.2010.02364.x
Chung, C. Y., Yeh, K. T., Hsu, N. C., Chang, J. H., Lin, J. T., Horng, H. C., et al. (2005). Expression of c-kit protooncogene in human hepatocellular carcinoma. Cancer Lett. 217, 231–236. doi: 10.1016/j.canlet.2004.06.045
Crosby, H. A., Kelly, D. A., and Strain, A. J. (2001). Human hepatic stem-like cells isolated using c-kit or CD34 can differentiate into biliary epithelium. Gastroenterology 120, 534–544. doi: 10.1053/gast.2001.21175
Deng, J., Ni, Z., Gu, W., Chen, Q., Nowak, W. N., Chen, T., et al. (2020). Single-cell gene profiling and lineage tracing analyses revealed novel mechanisms of endothelial repair by progenitors. Cell Mol. Life Sci. 77, 5299–5320. doi: 10.1007/s00018-020-03480-4
Doria, C., di Francesco, F., Marino, I. R., Ramirez, C. B., Frank, A., Iaria, M., et al. (2006). c-Kit-positive mast cells in portal tracts cannot be used to distinguish acute cellular rejection from recurrent hepatitis C infection in liver allografts. Transpl. Proc. 38, 3597–3600. doi: 10.1016/j.transproceed.2006.10.175
Dumble, M. L., Croager, E. J., Yeoh, G. C., and Quail, E. A. (2002). Generation and characterization of p53 null transformed hepatic progenitor cells: oval cells give rise to hepatocellular carcinoma. Carcinogenesis 23, 435–445. doi: 10.1093/carcin/23.3.435
Eckel, F., von Delius, S., Mayr, M., Dobritz, M., Fend, F., Hosius, C., et al. (2005). Pharmacokinetic and clinical phase II trial of imatinib in patients with impaired liver function and advanced hepatocellular carcinoma. Oncology 69, 363–371. doi: 10.1159/000089990
El-Houseini, M. E., Ismail, A., Abdelaal, A. A., El-Habashy, A. H., Abdallah, Z. F., Mohamed, M. Z., et al. (2019). Role of TGF-beta1 and C-Kit mutations in the development of Hepatocellular carcinoma in hepatitis C virus-infected patients: in vitro study. Biochemistry 84, 941–953. doi: 10.1134/S0006297919080108
El-Refaie, A. M., and Burt, A. D. (2005). Mast cells and c-Kit expression in liver allograft rejection. Histopathology 47, 375–381. doi: 10.1111/j.1365-2559.2005.02239.x
Faivre, S., Raymond, E., Boucher, E., Douillard, J., Lim, H. Y., Kim, J. S., et al. (2009). Safety and efficacy of sunitinib in patients with advanced hepatocellular carcinoma: an open-label, multicentre, phase II study. Lancet Oncol. 10, 794–800. doi: 10.1016/S1470-2045(09)70171-8
Fang, C. H., Gong, J. Q., and Zhang, W. (2004). Function of oval cells in hepatocellular carcinoma in rats. World J. Gastroenterol. 10, 2482–2487. doi: 10.3748/wjg.v10.i17.2482
Farrell, D. J., Hines, J. E., Walls, A. F., Kelly, P. J., Bennett, M. K., and Burt, A. D. (1995). Intrahepatic mast-cells in chronic liver-diseases. Hepatology 22, 1175–1181. doi: 10.1002/hep.1840220425
Ferrier, L., Berard, F., Debrauwer, L., Chabo, C., Langella, P., Bueno, L., et al. (2006). Impairment of the intestinal barrier by ethanol involves enteric microflora and mast cell activation in rodents. Am. J. Pathol. 168, 1148–1154. doi: 10.2353/ajpath.2006.050617
Fu, S., Wang, F., Cao, Y., Huang, Q., Xiao, J., Yang, C., et al. (2015). Telocytes in human liver fibrosis. J. Cell Mol. Med. 19, 676–683. doi: 10.1111/jcmm.12542
Fujii, T., Zen, Y., Harada, K., Niwa, H., Masuda, S., Kaizaki, Y., et al. (2008). Participation of liver cancer stem/progenitor cells in tumorigenesis of scirrhous hepatocellular carcinoma–human and cell culture study. Hum. Pathol. 39, 1185–1196. doi: 10.1016/j.humpath.2007.12.010
Fujio, K., Evarts, R. P., Hu, Z., Marsden, E. R., and Thorgeirsson, S. S. (1994). Expression of stem cell factor and its receptor, c-kit, during liver regeneration from putative stem cells in adult rat. Lab. Invest. 70, 511–516.
Fujio, K., Hu, Z., Evarts, R. P., Marsden, E. R., Niu, C. H., and Thorgeirsson, S. S. (1996). Coexpression of stem cell factor and c-kit in embryonic and adult liver. Exp. Cell Res. 224, 243–250. doi: 10.1006/excr.1996.0134
Gaca, M. D., Zhou, X., and Benyon, R. C. (2002). Regulation of hepatic stellate cell proliferation and collagen synthesis by proteinase-activated receptors. J. Hepatol. 36, 362–369. doi: 10.1016/s0168-8278(01)00285-9
Giebel, L. B., and Spritz, R. A. (1991). Mutation of the KIT (mast/stem cell growth factor receptor) protooncogene in human piebaldism. Proc. Natl. Acad. Sci. U.S.A. 88, 8696–8699. doi: 10.1073/pnas.88.19.8696
Hargrove, L., Kennedy, L., Demieville, J., Jones, H., Meng, F., DeMorrow, S., et al. (2017). Bile duct ligation-induced biliary hyperplasia, hepatic injury, and fibrosis are reduced in mast cell-deficient Kit(W-sh) mice. Hepatology 65, 1991–2004.
Hisada, M., Zhang, X., Ota, Y., Cameron, A. M., Burdick, J., Gao, B., et al. (2017). Fibrosis in small syngeneic rat liver grafts because of damaged bone marrow stem cells from chronic alcohol consumption. Liver Transpl. 23, 1564–1576. doi: 10.1002/lt.24820
Hu, B., and Colletti, L. M. (2008). Stem cell factor and c-kit are involved in hepatic recovery after acetaminophen-induced liver injury in mice. Am. J. Physiol. Gastrointest. Liver Physiol. 295, G45–G53. doi: 10.1152/ajpgi.00024.2008
Humbert, M., de Blay, F., Garcia, G., Prud’homme, A., Leroyer, C., Magnan, A., et al. (2009). Masitinib, a c-kit/PDGF receptor tyrosine kinase inhibitor, improves disease control in severe corticosteroid-dependent asthmatics. Allergy 64, 1194–1201. doi: 10.1111/j.1398-9995.2009.02122.x
Ikarashi, M., Nakashima, H., Kinoshita, M., Sato, A., Nakashima, M., Miyazaki, H., et al. (2013). Distinct development and functions of resident and recruited liver Kupffer cells/macrophages. J. Leukoc. Biol. 94, 1325–1336. doi: 10.1189/jlb.0313144
Ishii, M., Iwai, M., Harada, Y., Morikawa, T., Okanoue, T., Kishikawa, T., et al. (2005). A role of mast cells for hepatic fibrosis in primary sclerosing cholangitis. Hepatol. Res. 31, 127–131. doi: 10.1016/j.hepres.2005.01.007
Kanbe, N., Kurosawa, M., Nagata, H., Yamashita, T., Kurimoto, F., and Miyachi, Y. (2000). Production of fibrogenic cytokines by cord blood-derived cultured human mast cells. J. Allergy Clin. Immunol. 106(1 Pt 2), S85–S90. doi: 10.1067/mai.2000.106777
Kang, Y. K., Yau, T., Park, J. W., Lim, H. Y., Lee, T. Y., Obi, S., et al. (2015). Randomized phase II study of axitinib versus placebo plus best supportive care in second-line treatment of advanced hepatocellular carcinoma. Ann. Oncol. 26, 2457–2463. doi: 10.1093/annonc/mdv388
Kara, B., Doran, F., Kara, I. O., Akkiz, H., and Sandikci, M. (2008). Expression of c-kit protooncogen in hepatitis B virus-induced chronic hepatitis, cirrhosis and hepatocellular carcinoma: has it a diagnostic role? Int. J. Clin. Pract. 62, 1206–1211. doi: 10.1111/j.1742-1241.2007.01675.x
Kim, D. K., Cho, Y. E., Komarow, H. D., Bandara, G., Song, B. J., Olivera, A., et al. (2018). Mastocytosis-derived extracellular vesicles exhibit a mast cell signature, transfer KIT to stellate cells, and promote their activation. Proc. Natl. Acad. Sci. U.S.A. 115, E10692–E10701. doi: 10.1073/pnas.1809938115
King, A., Houlihan, D. D., Kavanagh, D., Haldar, D., Luu, N., Owen, A., et al. (2017). Sphingosine-1-phosphate prevents egress of hematopoietic stem cells from liver to reduce fibrosis. Gastroenterology 153, 233–248.e16. doi: 10.1053/j.gastro.2017.03.022
Knight, B., Tirnitz-Parker, J. E., and Olynyk, J. K. (2008). C-kit inhibition by imatinib mesylate attenuates progenitor cell expansion and inhibits liver tumor formation in mice. Gastroenterology 135, 969–979.e1. doi: 10.1053/j.gastro.2008.05.077
Koh, K. C., Lee, H., Choi, M. S., Lee, J. H., Paik, S. W., Yoo, B. C., et al. (2005). Clinicopathologic features and prognosis of combined hepatocellular cholangiocarcinoma. Am. J. Surg. 189, 120–125. doi: 10.1016/j.amjsurg.2004.03.018
Komuta, M., Spee, B., Vander Borght, S., De Vos, R., Verslype, C., Aerts, R., et al. (2008). Clinicopathological study on cholangiolocellular carcinoma suggesting hepatic progenitor cell origin. Hepatology 47, 1544–1556. doi: 10.1002/hep.22238
Koruk, S. T., Ozardali, I., Dincoglu, D., and Bitiren, M. (2011). Increased liver mast cells in patients with chronic hepatitis C. Indian J. Pathol. Microbiol. 54, 736–740. doi: 10.4103/0377-4929.91510
Kudo, M., Finn, R. S., Qin, S., Han, K. H., Ikeda, K., Piscaglia, F., et al. (2018). Lenvatinib versus sorafenib in first-line treatment of patients with unresectable hepatocellular carcinoma: a randomised phase 3 non-inferiority trial. Lancet 391, 1163–1173. doi: 10.1016/S0140-6736(18)30207-1
Kwon, Y. C., Bose, S. K., Steele, R., Meyer, K., Di Bisceglie, A. M., Ray, R. B., et al. (2015). Promotion of cancer stem-like cell properties in hepatitis C virus-infected hepatocytes. J. Virol. 89, 11549–11556. doi: 10.1128/JVI.01946-15
Larson, A. M., Polson, J., Fontana, R. J., Davern, T. J., Lalani, E., Hynan, L. S., et al. (2005). Acetaminophen-induced acute liver failure: results of a United States multicenter, prospective study. Hepatology 42, 1364–1372. doi: 10.1002/hep.20948
Lemmer, E. R., Shepard, E. G., Blakolmer, K., Kirsch, R. E., and Robson, S. C. (1998). Isolation from human fetal liver of cells co-expressing CD34 haematopoietic stem cell and CAM 5.2 pancytokeratin markers. J. Hepatol. 29, 450–454. doi: 10.1016/s0168-8278(98)80064-0
Lev, S., Blechman, J. M., Givol, D., and Yarden, Y. (1994). Steel factor and c-kit protooncogene: genetic lessons in signal transduction. Crit. Rev. Oncog. 5, 141–168. doi: 10.1615/critrevoncog.v5.i2-3.30
Li, Z., Chen, J., Li, L., Ran, J. H., Liu, J., Gao, T. X., et al. (2013). In vitro proliferation and differentiation of hepatic oval cells and their potential capacity for intrahepatic transplantation. Braz. J. Med. Biol. Res. 46, 681–688. doi: 10.1590/1414-431X20132620
Lin, A. Y., Fisher, G. A., So, S., Tang, C., and Levitt, L. (2008). Phase II study of imatinib in unresectable hepatocellular carcinoma. Am. J. Clin. Oncol. 31, 84–88. doi: 10.1097/COC.0b013e3181131db9
Liu, C., Wang, J., and Ou, Q. J. (2004). Possible stem cell origin of human cholangiocarcinoma. World J. Gastroenterol. 10, 3374–3376. doi: 10.3748/wjg.v10.i22.3374
Liu, Z., Dai, X., Wang, T., Zhang, C., Zhang, W., Zhang, W., et al. (2017). Hepatitis B virus PreS1 facilitates hepatocellular carcinoma development by promoting appearance and self-renewal of liver cancer stem cells. Cancer Lett. 400, 149–160. doi: 10.1016/j.canlet.2017.04.017
Llovet, J. M., Pena, C. E., Lathia, C. D., Shan, M., Meinhardt, G., Bruix, J., et al. (2012). Plasma biomarkers as predictors of outcome in patients with advanced hepatocellular carcinoma. Clin. Cancer Res. 18, 2290–2300. doi: 10.1158/1078-0432.CCR-11-2175
Llovet, J. M., Ricci, S., Mazzaferro, V., Hilgard, P., Gane, E., Blanc, J. F., et al. (2008). Sorafenib in advanced hepatocellular carcinoma. N. Engl. J. Med. 359, 378–390. doi: 10.1056/NEJMoa0708857
Luna, G., Paez, J., and Cardier, J. E. (2004). Expression of the hematopoietic stem cell antigen Sca-1 (LY-6A/E) in liver sinusoidal endothelial cells: possible function of Sca-1 in endothelial cells. Stem Cells Dev. 13, 528–535. doi: 10.1089/scd.2004.13.528
Mansuroglu, T., Baumhoer, D., Dudas, J., Haller, F., Cameron, S., Lorf, T., et al. (2009a). Expression of stem cell factor receptor c-kit in human nontumoral and tumoral hepatic cells. Eur. J. Gastroenterol. Hepatol. 21, 1206–1211. doi: 10.1097/MEG.0b013e328317f4ef
Mansuroglu, T., Ramadori, P., Dudas, J., Malik, I., Hammerich, K., Fuzesi, L., et al. (2009b). Expression of stem cell factor and its receptor c-Kit during the development of intrahepatic cholangiocarcinoma. Lab. Invest. 89, 562–574. doi: 10.1038/labinvest.2009.15
Matsusaka, S., Tsujimura, T., Toyosaka, A., Nakasho, K., Sugihara, A., Okamoto, E., et al. (1999). Role of c-kit receptor tyrosine kinase in development of oval cells in the rat 2-acetylaminofluorene/partial hepatectomy model. Hepatology 29, 670–676. doi: 10.1002/hep.510290304
Meadows, V., Kennedy, L., Hargrove, L., Demieville, J., Meng, F., Virani, S., et al. (2019). Downregulation of hepatic stem cell factor by vivo-morpholino treatment inhibits mast cell migration and decreases biliary damage/senescence and liver fibrosis in Mdr2(-/-) mice. Biochim. Biophys. Acta Mol. Basis Dis. 1865:165557. doi: 10.1016/j.bbadis.2019.165557
Meng, F., Francis, H., Glaser, S., Han, Y., DeMorrow, S., Stokes, A., et al. (2012). Role of stem cell factor and granulocyte colony-stimulating factor in remodeling during liver regeneration. Hepatology 55, 209–221. doi: 10.1002/hep.24673
Metcalfe, D. D., Baram, D., and Mekori, Y. A. (1997). Mast cells. Physiol. Rev. 77, 1033–1079. doi: 10.1152/physrev.1997.77.4.1033
Mukhopadhyay, A., Do, D. V., Ong, C. T., Khoo, Y. T., Masilamani, J., Chan, S. Y., et al. (2011). The role of stem cell factor and c-KIT in keloid pathogenesis: do tyrosine kinase inhibitors have a potential therapeutic role? Br. J. Dermatol. 164, 372–386. doi: 10.1111/j.1365-2133.2010.10035.x
Nakamura, A., Yamazaki, K., Suzuki, K., and Sato, S. (1997). Increased portal tract infiltration of mast cells and eosinophils in primary biliary cirrhosis. Am. J. Gastroenterol. 92, 2245–2249.
Nassar, I., Pasupati, T., Judson, J. P., and Segarra, I. (2009). Reduced exposure of imatinib after coadministration with acetaminophen in mice. Indian J. Pharmacol. 41, 167–172. doi: 10.4103/0253-7613.56071
Nazzal, M., Sur, S., Steele, R., Khatun, M., Patra, T., Phillips, N., et al. (2020). Establishment of a patient-derived xenograft tumor from hepatitis C-associated liver cancer and evaluation of imatinib treatment efficacy. Hepatology 72, 379–388. doi: 10.1002/hep.31298
Nomoto, K., Tsuneyama, K., Cheng, C., Takahashi, H., Hori, R., Murai, Y., et al. (2006). Intrahepatic cholangiocarcinoma arising in cirrhotic liver frequently expressed p63-positive basal/stem-cell phenotype. Pathol. Res. Pract. 202, 71–76. doi: 10.1016/j.prp.2005.10.011
Omori, M., Evarts, R. P., Omori, N., Hu, Z., Marsden, E. R., and Thorgeirsson, S. S. (1997). Expression of alpha-fetoprotein and stem cell factor/c-kit system in bile duct ligated young rats. Hepatology 25, 1115–1122. doi: 10.1002/hep.510250512
Petersen, B. E., Goff, J. P., Greenberger, J. S., and Michalopoulos, G. K. (1998). Hepatic oval cells express the hematopoietic stem cell marker Thy-1 in the rat. Hepatology 27, 433–445. doi: 10.1002/hep.510270218
Potti, A., Ganti, A. K., Tendulkar, K., Chitajallu, S., Sholes, K., Koch, M., et al. (2003). HER-2/neu and CD117 (C-kit) overexpression in hepatocellular and pancreatic carcinoma. Anticancer Res. 23, 2671–2674.
Pressiani, T., Boni, C., Rimassa, L., Labianca, R., Fagiuoli, S., Salvagni, S., et al. (2013). Sorafenib in patients with Child-Pugh class A and B advanced hepatocellular carcinoma: a prospective feasibility analysis. Ann. Oncol. 24, 406–411. doi: 10.1093/annonc/mds343
Ramadori, G., Fuzesi, L., Grabbe, E., Pieler, T., and Armbrust, T. (2004). Successful treatment of hepatocellular carcinoma with the tyrosine kinase inhibitor imatinib in a patient with liver cirrhosis. Anticancer Drugs 15, 405–409. doi: 10.1097/00001813-200404000-00014
Ray, P., Krishnamoorthy, N., Oriss, T. B., and Ray, A. (2010). Signaling of c-kit in dendritic cells influences adaptive immunity. Ann. N.Y. Acad. Sci. 1183, 104–122. doi: 10.1111/j.1749-6632.2009.05122.x
Ren, X., Hogaboam, C., Carpenter, A., and Colletti, L. (2003). Stem cell factor restores hepatocyte proliferation in IL-6 knockout mice following 70% hepatectomy. J. Clin. Invest. 112, 1407–1418. doi: 10.1172/JCI17391
Ren, X., Hu, B., and Colletti, L. (2008). Stem cell factor and its receptor, c-kit, are important for hepatocyte proliferation in wild-type and tumor necrosis factor receptor-1 knockout mice after 70% hepatectomy. Surgery 143, 790–802. doi: 10.1016/j.surg.2008.03.021
Rojas, A., Zhang, P., Wang, Y., Foo, W. C., Munoz, N. M., Xiao, L., et al. (2016). A Positive TGF-beta/c-KIT feedback loop drives tumor progression in advanced primary liver cancer. Neoplasia 18, 371–386. doi: 10.1016/j.neo.2016.04.002
Satake, M., Shimano, K., Yamamoto, T., Okaya, A., Iwasaki, T., Kakihana, M., et al. (2003). Role of c-kit receptor tyrosine kinase-mediated signal transduction in proliferation of bile epithelial cells in young rats after ligation of bile duct: a study using Ws/Ws c-kit mutant rats. J. Hepatol. 39, 86–92. doi: 10.1016/s0168-8278(03)00149-1
Seino, S., Tsuchiya, A., and Watanabe, M. (2014). A rare primary liver tumor that responded to sorafenib. Gastroenterology 147, 1226–1227. doi: 10.1053/j.gastro.2014.07.035
Shaib, Y. H., El-Serag, H. B., Davila, J. A., Morgan, R., and McGlynn, K. A. (2005). Risk factors of intrahepatic cholangiocarcinoma in the United States: a case-control study. Gastroenterology 128, 620–626. doi: 10.1053/j.gastro.2004.12.048
Shaker, M. E. (2014). Nilotinib interferes with the signalling pathways implicated in acetaminophen hepatotoxicity. Basic Clin. Pharmacol. Toxicol. 114, 263–270. doi: 10.1111/bcpt.12144
Shirakura, K., Masuda, H., Kwon, S. M., Obi, S., Ito, R., Shizuno, T., et al. (2011). Impaired function of bone marrow-derived endothelial progenitor cells in murine liver fibrosis. Biosci. Trends 5, 77–82. doi: 10.5582/bst.2011.v5.2.77
Si, Y., Tsou, C. L., Croft, K., and Charo, I. F. (2010). CCR2 mediates hematopoietic stem and progenitor cell trafficking to sites of inflammation in mice. J. Clin. Invest. 120, 1192–1203. doi: 10.1172/JCI40310
Tanaka, S., Yamamoto, T., Tanaka, H., Kodai, S., Ogawa, M., Ichikawa, T., et al. (2005). Potentiality of combined hepatocellular and intrahepatic cholangiocellular carcinoma originating from a hepatic precursor cell: immunohistochemical evidence. Hepatol. Res. 32, 52–57. doi: 10.1016/j.hepres.2005.01.012
Terai, S., Ishikawa, T., Omori, K., Aoyama, K., Marumoto, Y., Urata, Y., et al. (2006). Improved liver function in patients with liver cirrhosis after autologous bone marrow cell infusion therapy. Stem Cells 24, 2292–2298. doi: 10.1634/stemcells.2005-0542
Uddin Ahmed, A. F., Ohtani, H., Nio, M., Funaki, N., Sasaki, H., Nagura, H., et al. (2000). Intrahepatic mast cell population correlates with clinical outcome in Biliary atresia. J. Pediatr. Surg. 35, 1762–1765. doi: 10.1053/jpsu.2000.19245
Vagnozzi, R. J., Maillet, M., Sargent, M. A., Khalil, H., Johansen, A. K. Z., Schwanekamp, J. A., et al. (2020). An acute immune response underlies the benefit of cardiac stem cell therapy. Nature 577, 405–409. doi: 10.1038/s41586-019-1802-2
Weiskirchen, R., Meurer, S. K., Liedtke, C., and Huber, M. (2019). Mast cells in liver fibrogenesis. Cells 8:1429. doi: 10.3390/cells8111429
Went, P. T., Dirnhofer, S., Bundi, M., Mirlacher, M., Schraml, P., Mangialaio, S., et al. (2004). Prevalence of KIT expression in human tumors. J. Clin. Oncol. 22, 4514–4522. doi: 10.1200/JCO.2004.10.125
Xu, J., Tan, Y., Shao, X., Zhang, C., He, Y., Wang, J., et al. (2018). Evaluation of NCAM and c-Kit as hepatic progenitor cell markers for intrahepatic cholangiocarcinomas. Pathol. Res. Pract. 214, 2011–2017. doi: 10.1016/j.prp.2018.09.005
Yamashiro, M., Kouda, W., Kono, N., Tsuneyama, K., Matsui, O., and Nakanuma, Y. (1998). Distribution of intrahepatic mast cells in various hepatobiliary disorders - An immunohistochemical study. Virchows Arch. 433, 471–479. doi: 10.1007/s004280050276
Yan, W., Zhu, Z., Pan, F., Huang, A., and Dai, G. H. (2018). Overexpression of c-kit(CD117), relevant with microvessel density, is an independent survival prognostic factor for patients with HBV-related hepatocellular carcinoma. Oncol. Targets Ther. 11, 1285–1292. doi: 10.2147/OTT.S157545
Yu, X. H., Xu, L. B., Zeng, H., Zhang, R., Wang, J., and Liu, C. (2011). Clinicopathological analysis of 14 patients with combined hepatocellular carcinoma and cholangiocarcinoma. Hepatobil. Pancreat. Dis. Int. 10, 620–625. doi: 10.1016/s1499-3872(11)60105-7
Yushkov, B. G., Danilova, I. G., Ponezheva, Zh B, Brykina, I. A., Abidov, M. T., and Kalyuzhin, O. V. (2011). Modulation of reparative regeneration and CD117 expression by liver cells after partial hepatectomy in mice. Bull. Exp. Biol. Med. 150, 352–354. doi: 10.1007/s10517-011-1140-3
Zhang, F., Chen, X. P., Zhang, W., Dong, H. H., Xiang, S., Zhang, W. G., et al. (2008). Combined hepatocellular cholangiocarcinoma originating from hepatic progenitor cells: immunohistochemical and double-fluorescence immunostaining evidence. Histopathology 52, 224–232. doi: 10.1111/j.1365-2559.2007.02929.x
Zhang, Q., He, Y., Luo, N., Patel, S. J., Han, Y., Gao, R., et al. (2019). Landscape and dynamics of single immune cells in hepatocellular carcinoma. Cell 179, 829–845.e20. doi: 10.1016/j.cell.2019.10.003
Keywords: c-kit, liver regeneration, stem cell, liver disease, HCC
Citation: Wang W, Shui L, Liu Y and Zheng M (2021) C-Kit, a Double-Edged Sword in Liver Regeneration and Diseases. Front. Genet. 12:598855. doi: 10.3389/fgene.2021.598855
Received: 25 August 2020; Accepted: 08 January 2021;
Published: 02 February 2021.
Edited by:
Lon J. Van Winkle, Rocky Vista University, United StatesReviewed by:
Isabel Fabregat, Institut d’Investigacio Biomedica de Bellvitge (IDIBELL), SpainCopyright © 2021 Wang, Shui, Liu and Zheng. This is an open-access article distributed under the terms of the Creative Commons Attribution License (CC BY). The use, distribution or reproduction in other forums is permitted, provided the original author(s) and the copyright owner(s) are credited and that the original publication in this journal is cited, in accordance with accepted academic practice. No use, distribution or reproduction is permitted which does not comply with these terms.
*Correspondence: Yanning Liu, bGl1eWFubmluZ0B6anUuZWR1LmNu; Min Zheng, bWluemhlbmdAemp1LmVkdS5jbg==
Disclaimer: All claims expressed in this article are solely those of the authors and do not necessarily represent those of their affiliated organizations, or those of the publisher, the editors and the reviewers. Any product that may be evaluated in this article or claim that may be made by its manufacturer is not guaranteed or endorsed by the publisher.
Research integrity at Frontiers
Learn more about the work of our research integrity team to safeguard the quality of each article we publish.