- Department of Children and Adolescences Health, School of Public Health, Shanxi Medical University, Taiyuan, China
Polycyclic aromatic hydrocarbons (PAHs) are known environmental pollutants. Studies are very limited regarding the impacts of paternal PAHs exposure on birth outcomes as well as the underpinning mechanisms in human. In this study, 302 reproductive-aged males (22–46 years old) were enrolled and demographic informatics data were obtained by questionnaires. The levels of urinary hydroxylated PAHs (OH-PAHs) were assessed by ultra-high performance liquid chromatography-tandem mass spectrometry; and methylation levels of the imprinting genes H19, Meg3, and Peg3 of sperm DNA were evaluated via bisulfite pyrosequencing. The analysis of the correlation between OH-PAHs levels and methylation levels of imprinting genes showed that OH-PAHs are correlated with some CpG sites in H19, Peg3, and Meg3. To further investigate an association of urinary OH-PAHs with birth outcomes, follow-up study of wives of these subjects has been performed for 1–3 years. As the result, a total of 157 babies were born. The birth outcomes parameters including birth weight (BW), length (BL), and ponderal index (PI) were recorded. The further analysis of generalized estimating equation indicated a negative correlation between urinary total OH-PAHs levels and newborn BW (β = −0.081, p = 0.020); but this association has not been found for BL and PI. Furthermore, a logistic regression analysis was employed for examining associations of the methylation of imprinting genes with birth outcomes parameters, which indicated a negative correlation between BW and H19, namely, each unit percent (%) elevation in methylation of H19 (but not Peg3 and Meg3) was significantly associated with a 0.135 g reduction of BW (β = −0.135; 95% CI 0.781–0.978). Putting together, these results show that paternal non-occupational environmental exposure to PAHs is associated with newborn BW. And imprinting gene H19 methylation may be involved in the underlying mechanisms. This study in human population adds a support for previous animal study and implies that environmental impact on the offspring through paternal pathway.
Introduction
Recently, the impacts of environmental pollutants on reproduction and development in humans have been of increasing concern (Nassar et al., 2010; Shirangi et al., 2011). Polycyclic aromatic hydrocarbons (PAHs) represent molecules released during partial combustion of hydrocarbons (Mumtaz and George, 1995). Outdoor air and soil could contain PAHs produced by industries, wild fires, automobiles, and asphalt, while indoor air could also be polluted with PAHs by home heating and cooking emissions (Lewtas, 2007). Human exposure to PAHs occurs through air inhalation, ingested food (especially after meat grilling or smoking), and exposure to tobacco smoke (US Department of Health Human Services, 1995; Aquilina et al., 2010). PAHs are metabolically bio-transformed in the body to produce hydroxylated compounds that ultimately undergo urine excretion. In human study, urine contents of hydroxylated PAHs (OH-PAHs) have been broadly employed as surrogates for estimating exposure to PAHs (Ramesh et al., 2004). Since PAHs can cross the placental barrier into the fetus, these compounds have also been proven to lead detrimental effects such as congenital malformation and developmental disorders (Polanska et al., 2014; Rengarajan et al., 2015). For example, previous animal studies suggested that PAHs affect the length of gestation and intrauterine growth (Detmar et al., 2008). Further human studies showed direct maternal exposure to PAHs of pregnant women was associated with adverse birth outcomes (Dejmek et al., 2000; Choi et al., 2006, 2008; Lamichhane et al., 2016), including delayed intrauterine growth and preterm deliveries (Singh et al., 2008; Wilhelm et al., 2011; Guo et al., 2012), reduced newborn weights, head circumferences, and lengths (Dejmek et al., 2000; Perera et al., 2005; Choi et al., 2006; Tang et al., 2006; Jedrychowski et al., 2012, 2017; Duarte-Salles et al., 2013; Langlois et al., 2014; Padula et al., 2014). However, research on adverse birth outcomes from paternal PAHs exposure is very limited (Padula et al., 2014). Recently, paternal impacts on embryonic development is receiving increased attention. The mechanisms underlying the impact of paternal PAHs exposure on their offspring have not been fully characterized.
Imprinted genes play critical roles in normal fetal growth and placental function (Tunster et al., 2013). It has been suggested that epigenetic events might underlie the risk of adverse birth outcomes through phenotypic plasticity (Vidal et al., 2014; Montoya-Williams et al., 2017). A previous study revealed that paternal life behavior and the effects of environmental exposure are passed on to future generations through sperm genetic imprinting (Abbasi, 2017). The large number of imprinted genes known to affect fetal and placental growth. Among them, research reports showed that disturbance of the methylation status of the imprinted genes H19 and Mest leads to decreased sperm function and embryo development failure, as well as with the risk of passing this defect on to the offspring (Marques et al., 2004). Paternally expressed genes, such as Peg3, is susceptible to epigenetic modification, are highly expressed in the placenta, which is critical for fetal growth (Joomyeong et al., 2013). In additional, previous study showed that a small group of imprinted genes including Meg3 were affected in IUGR placentas (McMinn et al., 2006). Therefore, the current study will address epigenetical mechanisms underlying the associations of paternal urine PAHs contents with adverse birth outcomes by examining the relevant changes of the imprinting genes H19, Peg3, and Meg3 in human sperm DNA.
Our previous animal experimental studies of paternal B(a)P exposure showed a disorder in the methylation levels of four imprinting genes in sperm DNA. Importantly, a similar pattern in methylation change of these genes was seen in their unexposed F1-2 male mice (Zhang et al., 2019). In our recent human studies, paternal Hg and PAHs exposures are associated with altered methylation pattern in sperm (Lu et al., 2018; Ma et al., 2019). However, whether paternal exposure to PAHs and the altered DNA methylation levels of imprinting genes in sperm are linked, and eventually lead to adverse birth outcomes, remains unknown. Shanxi Province of China represents an industrialized region with relatively elevated amounts of PAHs in air, especially due to coal-processing waste. By investigating the father and their newborns in this area, current study aimed to assess potential associations of paternal PAHs exposure with birth outcomes.
Materials and Methods
Study Participants and Data Collection
A total of 302 reproductive-age men were enrolled at the Reproductive Clinic of Maternal and Child Care Service Center in Shanxi Province from April 2015 to March 2016. They visited the center for pre-pregnancy check or family infertility exam. After enrollment, the participants completed a validated questionnaire including sociodemographic information (age, occupation, education level, history of abnormal pregnancy, and health condition) and lifestyles (smoking and consumption of alcohol, and frequencies of consuming fried and barbecued meats). The exclusion criterion was a family history of birth defects. The included individuals were followed up until their children were born, and newborn conditions (birth outcome, vital signs, and delivery conditions). All participating individuals provided written informed consent. The study protocol had approval from the Research Ethics Committee of Shanxi Medical University.
Urine and Semen Collection and Analysis
Specimen Collection
A total of 50 ml midstream urine was obtained in clean vessels followed by immediate transport to the laboratory, and repackaging into and storage in 10 mL vials at −80°C. Meanwhile, semen specimens were collected after refraining from ejaculation for 3–7 days, by masturbation with no lubricant use into sterile tubes. Upon liquefaction, semen specimens underwent centrifugation (200 g, 15 min), and the pelleted sperm was kept at −80°C for subsequent DNA methylation measurement.
Assessment of Urinary Monohydroxylated PAHs Levels
The PAHs metabolites measured in the present study included 1-hydroxynaphthalene (1-OH NAP), 2-hydroxynaphthalene (2-OH NAP), 3-hydroxyfluoren (3-OH FLU), 2-hydroxyfluoren (2-OH FLU), 2-hydroxyphenanthrene (2-OH PHE), 1-hydroxyphenanthrene (1-OH PHE), 1-hydroxypyrene (1-OH PYR). For assessment, enzyme digestion and solid phase extraction were carried out, followed by Ultra-high Performance Liquid Chromatography with tandem Mass Spectrometry (UHPLC-MS/MS; Shimadzu, Japan). For enzymatic hydrolysis, 4 mL of thawed urine sample was mixed with 4 mL of acetic acid-ammonium acetate buffer solution (pH = 5) and 20 μL of β-glucuronidase (Sigma, United States), and then incubated at 37°C for 12 h. Then, the mixture was loaded onto a Sep-Pak C18 (6 cc, 500 mg) cartridge (Waters, United States), which was activated with 5 mL of methanol and 5 mL of distilled water. The hydrolyzate was enriched using a C18 solid-phase extraction column eluted with 5 mL of 30% methanol, and slowly eluted with 5 mL of methanol. The eluate was concentrated by dry nitrogen purge and fixed by methanol to yield the extract (0.5 ml). UHPLC-MS/MS was performed to simultaneously measure the seven OH-PAHs as described previously (Zhao et al., 2013; Lankova et al., 2016; Urbancova et al., 2017). The limit of detection (LOD) was the lowest standard concentration yielding a signal-to-noise ratio (S/N) of three. Linearity (R2), LOD, precision (relative standard deviation or RSD), and mean recovery rate were 0.9930–0.9998, 0.02–0.094 ng/mL, 2.7–11.6%, and 71.4–109.4%, respectively. Reagent blanks and urine specimens were assessed, and none of the PAH metabolites were found, suggesting the absence of contamination.
DNA Extraction and Bisulfite Treatment
Genomic DNA from each individual sperm sample was extracted with QIAamp DNA micro kit (Qiagen, United States) as previously proposed (Yuan et al., 2015). DNA quantitation was carried out on a Nanodrop 2000 Spectrophotometer (Thermo Fisher Scientific). Then, genomic DNA (1,000 ng) underwent treatment with sodium bisulfite for converting unmethylated cytosine moieties into uracil while leaving methylated cytosine unaltered, with EZ Methylation Gold-Kit (Zymo Research, United States) as directed by the manufacturer. After the converted reaction, DNA elution was performed with 15 μl of TE buffer (10 mM Tris–HCl, 0.1 mM EDTA, pH 7.5), and employed for PCR amplification.
PCR Amplification of Bisulfite-Treated Sperm DNA and Pyrosequencing
The experimental protocols were described in a previous report (Lu et al., 2018). Briefly, PCR was performed with Pyro Mark PCR Kit (Qiagen, CA, United States) with about 50 ng of bisulfite-DNA and 0.2 μM each of forward and reverse primers in 25 μl PCR reactions, as directed by the manufacturer. Reverse primers were linked to biotin; pyrosequencing was carried out on a Pyro Mark Q96 MD pyrosequencing instrument (Qiagen). PCR and pyrosequencing primers are shown in Table 1.
The biotinylated amplicons were obtained with streptavidin-Sepharose beads (Amersham, United States) and underwent sequencing with PyroMark Gold Q96 kit (Biotage, Sweden). Methylation levels at various CpG sites were examined with the PyroMark CpG Software (Biotage). Duplicate pyrosequencing assays were carried out in sequential runs. The numbers of CpGs sites assessed at various differentially methylated regions (DMRs) were: H19, 7; Meg3, 8; Peg3, 8 (Edwards et al., 2006).
Anthropometric Assessment of Newborns
Birth outcomes from follow-up investigation were assessed. The anthropometric parameters of newborns were birth weight (BW), birth length (BL), and ponderal index (PI). The PI {BW (g)/[BL (cm)] 3 × 102} is a body mass index that is most commonly used in pediatrics (Leitner et al., 2007).
Statistical Analysis
Hydroxylated PAHs levels were presented as median and interquartile range (IQR). The concentrations of PAHs metabolites below the LOD were considered to be half the indicated LOD. The Pearson correlation method was performed for evaluating associations of methylation at various CpG sites. Generalized estimating equation (GEE) analysis was performed for determining associations of urine OH-PAHs levels with the offspring’s birth outcomes after adjusting for potential confounding factors. The associations of paternal OH-PAHs levels with the methylation levels of the imprinting genes H19, Meg3, and Peg3 in sperm DNA were examined with Logistic regression analysis with the above co-variates to rule out potential confounders of BW after controlling for potential confounders using a logistic regression model. SPSS for Windows 22.0 (SPSS, United States) was employed for statistical analyses. Bilateral p < 0.05 indicated statistical significance.
Results
Demographic Features of the Participants
A total of 302 male participants in reproductive-age from 22 to 46 years old (median age of 30 years) were recruited. Their baseline features are presented in Table 2. Of all participant individuals, 54.0% had a college education level or higher; there were 30.1% with drinking status, and 55.7% were overweight (BMI > 24). More than half of the participants (167) were smokers, with 158 having three or more years of smoking experience; only 10.9% (n = 33) ate smoked food.
Concentration Distributions of the Seven OH-PAHs in Urine
To examine PAH exposure level, seven OH-PAHs were measured in urinary samples from the 302 participants. The concentrations corrected by urine specific gravity (Leitner et al., 2007; Gluckman et al., 2009; El Hajj et al., 2014) are shown in Table 3. The medians and IQRs of these OH-PAHs were as follows: 2-OH NAP, 0.058 (0.020–0.142); 1-OH NAP, 0.027 (0.009–0.049); 3-OH FLU, 0.025 (0.015–0.044); 2-OH FLU, 0.032 (0.019–0.062); 2-OH PHE, 0.092 (0.044–0.187); 1-OH PHE, 0.006 (0.001–0.027); 1-OH PYR, 0.015 (0.002–0.049). Of these OH-PAHs, the median value of 2-OH PHE was the highest (0.092 μg/L), then followed by urinary 2-OH NAP (0.058 μg/L), and urinary 2-OH FLU (0.032 μg/L). While the median concentration of urinary 1-OH PYR was the lowest (0.015 μg/L).
Methylation Levels of Imprinting Genes in Sperm DNA
For the 302 participants, DNA methylation levels of H19, Peg3, and Meg3 measured in sperm samples are presented as mean ± standard deviation (Table 4). The average methylation levels of all analyzed CpG sites in H19, Peg3, and Meg3 were 87.55, 0.99, and 2.25%, respectively. To further determine potential correlations among individual CpG sites and between individual CpG sites and their average methylation levels for H19, Peg3, and Meg3, respectively, we performed the Pearson correlation analyses among seven CpG sites in H19, seven CpG sites in Peg3 and eight CpG sites in Meg3. DNA methylation levels in more than half of CpG sites within the H19 gene and all sites in Peg3 and Meg3 were positively correlated (r = 0.882–0.986, p < 0.05–0.01) (Table 5). Additionally, average methylation levels were also strongly correlated with relevant CpG sites (r = 0.217–0.994, p < 0.05–0.01). Thus, average methylation levels were employed to further assess associations.
Association Between Sperm DNA Methylation and OH-PAHs Concentrations
To examine potential relationship between DNA methylation levels of selected imprinting genes and urinary OH-PAHs concentrations, Spearman’s rank correlation analysis was carried out by using average methylation level of selected CpGs sites in each gene. The results indicated that there were associations between some individual CpG sites on H19, Peg3, and Meg3 DMR and OH-PAHs concentrations. In H19, we found multiple sites correlated with OH-PAHs concentrations. Namely, there were positive correlation found at CpG #1 between in the level of methylation and 1-OH PHY (rs = 0.243, p = 0.002); at CpG #5 between the level of methylation and 1-OH NAP (rs = 0.157, p = 0.05) and 2-OH PHE (rs = 0.186, p = 0.02); at CpG #6 between the level of methylation and 3-OH FLU (rs = 0.188, p = 0.019). While a negative correlation between the level of 1-OH PHE and methylation at CpG #3 (rs = −0.302, p < 0.001) and CpG #5 (rs = −0.279, p < 0.001). In Peg3, methylation levels at CpG #1 (rs = 0.175, p = 0.029), CpG #2 (rs = 0.194, p = 0.015), CpG #3 (rs = 0.160, p = 0.046), and CpG #5 (rs = 0.214, p = 0.007) of demonstrated a positive correlation with the levels of 2-OH FLU, respectively. And methylation levels at CpG #2 of Meg3 demonstrated a positive correlation with 1-OH PHE (rs = 0.301, p = 0.02) and 2-OHPHE (rs = 0.173, p = 0.03), respectively (Table 6). These results imply the associations between DNA methylation levels of selected imprinting genes and OH-PAHs concentrations.
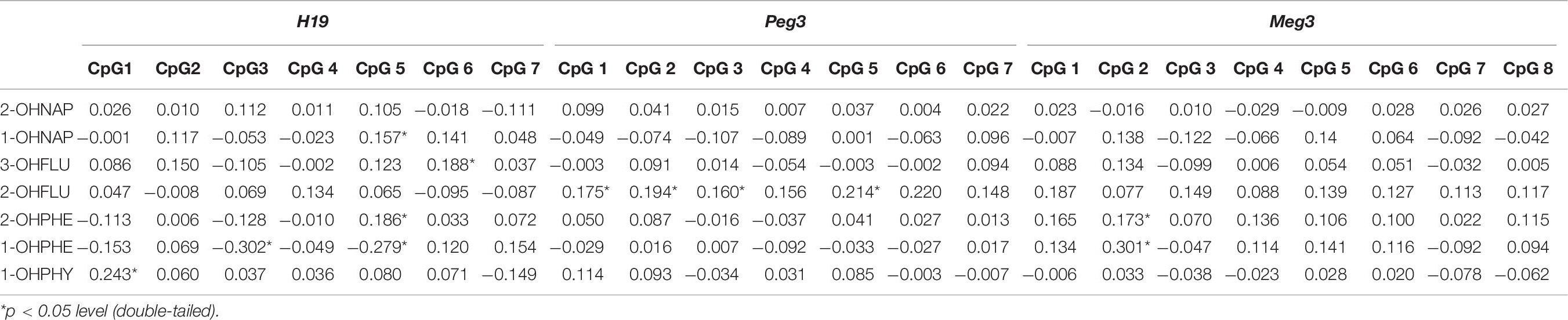
Table 6. Spearman correlation analysis between individual methylation levels CpGs of H19, Peg3, and Meg3 and OH-PAHs
Associations of Paternal Urine OH-PAHs Concentrations With Birth Outcomes in the Newborns
These 302 reproductive-aged men were then followed up for 1–3 years to record the development of their offspring. A total of 157 out of the 302 participants’ wives were pregnant and successively gave birth. The babies were born with the mean BW of 3,169.3 g (±643.8 g) and BL of 49.8 cm (±2.9 cm). The percentage of newborn girls was slightly lower compared with that of boys (42.7 and 57.3%, respectively). Three parameters of BW (t = −0.477, p = 0.634), BL (t = −0.044, p = 0.965), and PI (t = −0.951, p = 0.343) of the newborns at delivery were comparable in girls and boys.
To evaluate potential associations of paternal urinary OH-PAHs levels with offspring’s birth outcomes parameters, we divided the newborns into two groups based on BW: Group 1, 2,500–4,000 g, and Group 2, <2,500 g or >4,000 g. Group 1 had 129 individuals and Group 2 had 28. In addition, we divided the newborns into two groups by BL: Group 1, 45.2–55.8 cm, and Group 2, <45.2 cm or >55.8 cm. Also, based on PI, the newborns were divided into: Group 1, 2.0–2.2, and Group 2, <2.0 or >2.2. With GEE regression analysis, we found that the total OH-PAHs concentration was negatively correlated with newborns’ BW (β = −0.081, p = 0.020) (Supplementary Table 1 and Figure 1) after adjusting for paternal education level, paternal BMI, paternal age, paternal status of smoking (yes or no), paternal status of drinking (yes or no), paternal status of eating bacon (yes or no), newborn gender, length of gestational weeks, and delivery method. Using Multivariate procedures in the above described co-variables to control potential confounding. However, no association between the total OH-PAHs concentration and BL and PI (Supplementary Tables 2, 3) was observed.
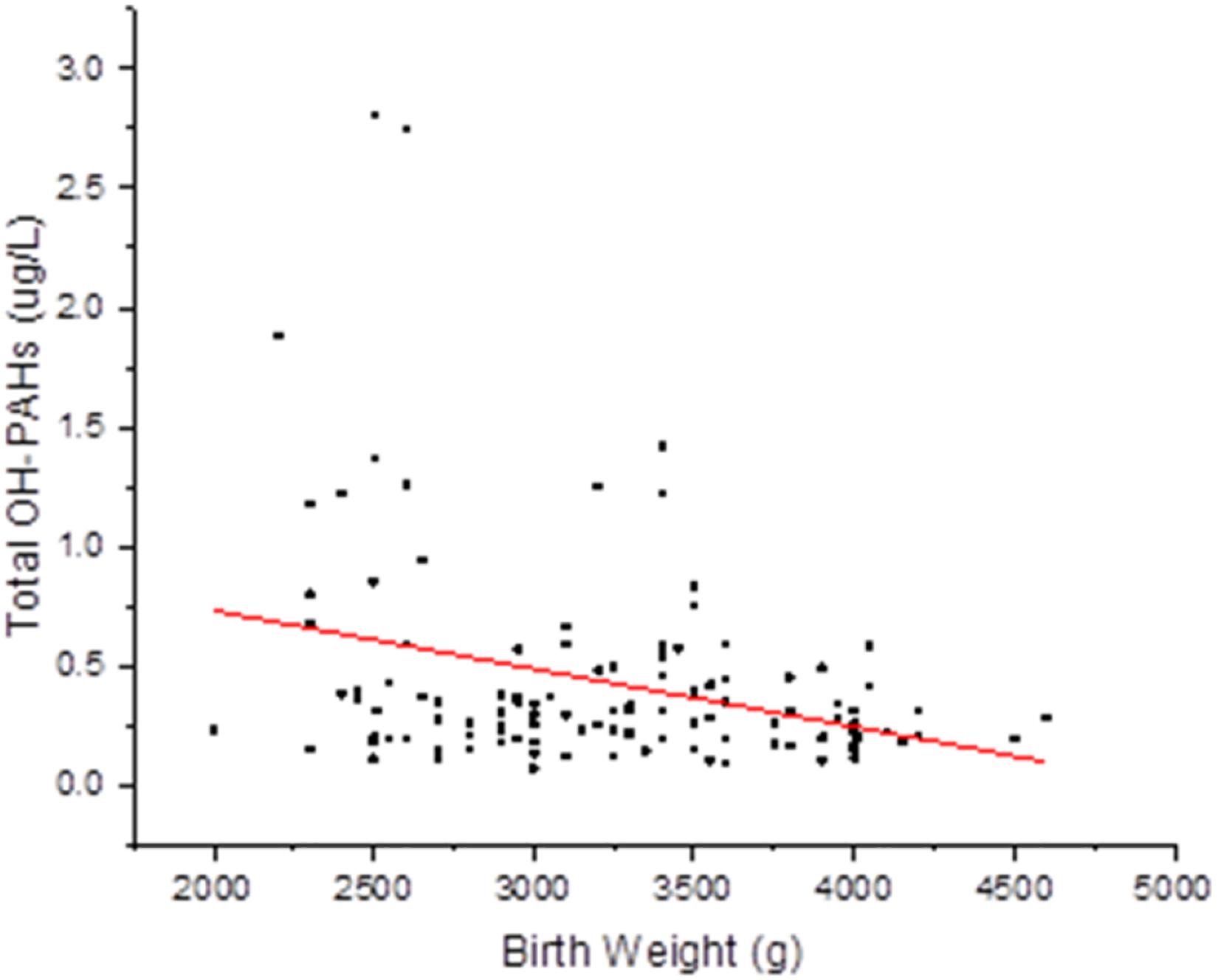
Figure 1. Correlation between total OH-PAHs levels and new born BW. Total OH-PAHs levels were negative correlated to new born BW in this study. Each point on the graph represents individual measurement and red line represents Liner fit trend.
Associations of Sperm DNA Methylation With Newborn Birth Weight
Persistent epigenetic changes are considered a mechanism underlying the effects of environmental risk factors on infants (Gluckman et al., 2009; El Hajj et al., 2014). Since methylation is subjected to many factors, co-variates, such as paternal age, status of smoking, status of drinking, education level, BMI, newborn gender, gestational weeks, and delivery method, the interactions of total OH-PAHs concentration with average methylation levels of H19, Peg3, and Meg3 and were evaluated, respectively, to understand potential mechanism underlying the associations between OH-PAHs concentration and BW. Logistic regression analysis was carried out with the above co-variates to rule out potential confounders of BW. Potential confounding factors (paternal age, paternal status of smoking, paternal status of drinking, paternal education, paternal BMI, newborn gender, gestational weeks, delivery method, interaction of total PAHs concentration with mean of H19 methylation levels, interaction of total PAHs concentration with mean of Peg3 methylation levels, and interaction of total PAHs concentration with mean Meg3 methylation level) were included in the models. We found a significant negative correlation between mean H19 methylation and BW (β = −0.135, p = 0.019). Namely each unit percent (%) elevation in H19 methylation was significantly associated with 0.135 g reduction of BW (β = −0.135; 95% CI 0.781–0.978) (Table 7 and Figure 2). However, such an association was not observed for Meg3 or Peg3 in this study.
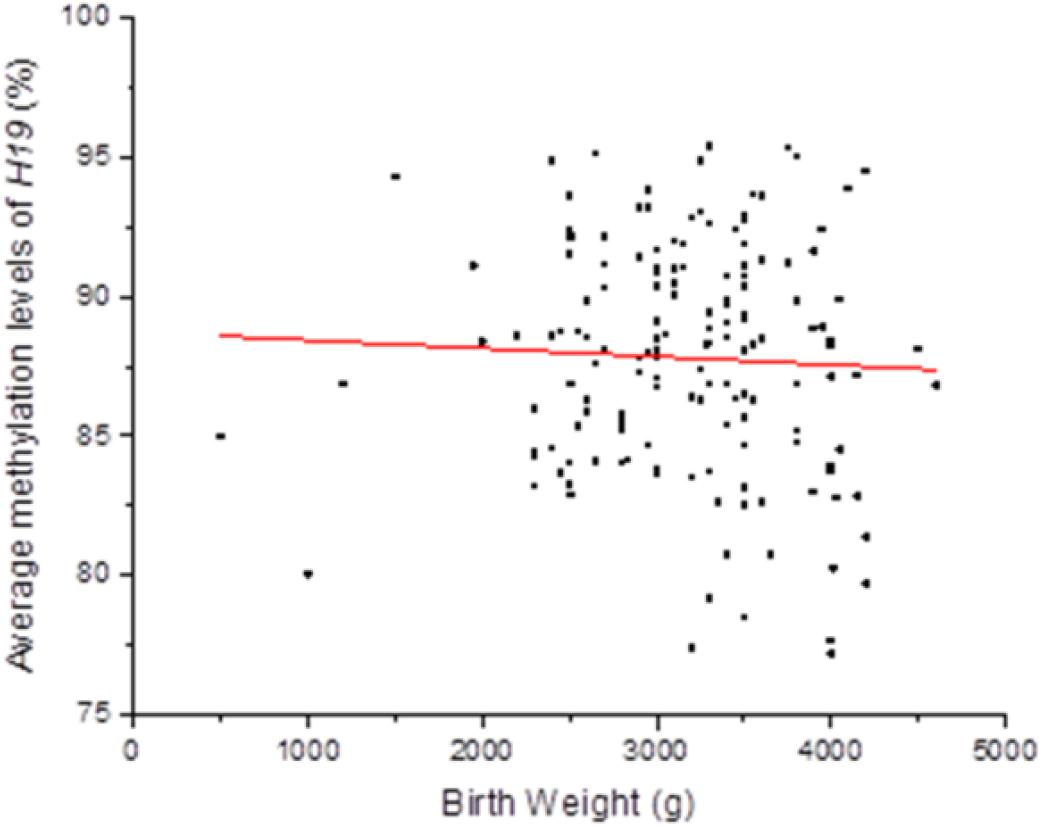
Figure 2. Correlation between methylation levels of imprinting gene H19 in Sperm DNA and new born BW. Methylation levels of imprinting gene H19 in Sperm DNA were negative correlated to new born BW in this study. Each point on the graph represents individual measurement and red line represents Liner fit trend.
Discussion
Recently, there has been emerging evidence that the profound paternal adverse exposure, such as obesity (Ost et al., 2014), ethanol exposure (Knezovich and Ramsay, 2012) lead an influence on the fetus growth. The results from our previous animal study indicated that paternal exposure to BaP, an important component of PAHs, induced changes in methylation levels of the four imprinting genes in sperm DNA and these alterations pasted to their F1 and F2 male offspring (Zhang et al., 2019). In the present study, we sought to provide evidence in human population by studying the impact of paternal natural exposure to environmental insult, PAHs on birth outcomes and address its underlying epigenetic mechanism. We found that a positive correlation between paternal urinary OH-PAHs level and some CpG sites in H19, Peg3, and Meg3. By following up 1–3 years, for the first time, we showed that both paternal urinary OH-PAHs and methylation levels of imprinting gene H19 negatively correlation with body weight of newborns. These results support that paternal-PAHs exposure impacts on the fetus growth, which may be mediated by potential epigenetic mechanism.
Environmental PAHs exposure induces alterations in imprinting genes in sperm DNA.
Direct environmental exposures have different impacts on exposed individuals in terms of somatic cell development. However, only changes in the germ line (sperm or egg) may explain the transgenerational effects of paternal experiences on offspring development and fitness. Increasing amounts of evidence are showing that environmental exposure to harmful agents including PAHs has adverse effects on reproduction and development in animal and human fetuses’ studies (Vidal et al., 2014; Montoya-Williams et al., 2017). For example, epidemiological studies provided evidence that PAHs greatly affect multiple parameters of fetal development, including BW (Jedrychowski et al., 2017). Low BW results in elevated mortality and morbidity from the neonatal period to adult age, making it a main deleterious perinatal outcome (Risnes et al., 2011). Although environmental pollutants affect fetal development, the underpinning mechanisms remain undefined (Pedersen et al., 2013). In this study, paternal imprinting genes H19 and Meg3, and the maternal imprinted gene Peg3 were selected to investigate their sensitivity to PAHs exposure. By analysis of urine and semen samples, we found that a certain type of PAHs showed a correlation with some CpG sites of imprinting gene H19, Peg3, and Meg3, respectively. For instance, the methylation levels of H19 showed at sites CpG#1, CpG #5, and #6 have positive correlations with 1-OH PHY, 1-OH NAP, 2-OH PHE, and 3-OH FLU, suggesting that the higher PAHs exposure may have the higher DNA methylation level. On the other hand, a negative correlation between the level of 1-OH PHE and methylation at CpG #3. So far, there is no direct evidence to explain which CpG sites should response for the effect of a specific parameter of fetal growth. Therefore, the present results demonstrate environmental PAHs exposure induces alterations in imprinting genes in sperm DNA. Therefore, they have potential to be biomarkers for the risk assessment of environmental exposure-induced embryonic developmental disorders. Paternal epigenetic mechanisms may mediate PAHs effects on birth outcomes in their offspring.
In mammalians, imprinting genes play roles in growth-related functions. Genomic imprinting represents an epigenetic event preferentially silencing a copy of an autosomal gene, allowing the expression of the other copy (Hutter et al., 2010). Epigenetic regulatory heavily contribute to imprinted genes expression, such as DNA methylation in DMRs (Iglesias-Platas et al., 2014). Meanwhile, DNA methylation is the most mature research mechanism among epigenetic markers of development, and highly vulnerable to environmental exposures. Recently, it was proposed that dynamic imprinted genes might have high susceptibility to environmental changes, and play an essential role in regulating plastic development and controlling cell functions via epigenetic modifications (Radford et al., 2011). In this study, we analyzed the association between paternal methylation level of the three imprinting genes and offspring BW; and between the level of paternal urinary OH-PAHs and offspring BW. The results indicated that only methylation of H19 in sperm DNA was significantly negatively correlated with offspring BW, although some CpG sites in Peg3 and Meg3 was also observed to correlate with the level of paternal urinary OH-PAHs. This result supports that imprinting gene regulation exists tissue-specific (Lee, 2001) and implies H19 DNA’s DMR as a potential biomarker of paternal environmental exposure to PAHs on fetal development and BW. Although sperm DNA methylation in H19 correlated with offspring weight in this work, the timing and levels of such methylation alterations are still undefined. The detailed mechanism in regulating fetal growth need to be revealed in the further study.
Overall, the results implicated that sperm DNA responding to environmental elevated PAHs exposure lead an increase in methylation level of H19 sperm DNA; which may mediate the occurrence of lower BW. Therefore, methylation alteration of H19 of sperm DNA could represent a “signature” for the environmental insults.
Limitation of the Present Study
Due to the reason of viability and cost, sample size was relatively small. In addition, birth outcomes data is less too. Therefore, our analysis could not go to deep insight. Further investigation is required by using more samples and more parameters.
Data Availability Statement
The raw data supporting the conclusions of this article will be made available by the authors, without undue reservation.
Ethics Statement
The studies involving human participants were reviewed and approved by the Research Ethics Committee of Shanxi Medical University. The patients/participants provided their written informed consent to participate in this study.
Author Contributions
JY, LW, and MQ: conceptualization. JY and ZXL: methodology. JY: formal analysis and writing– original draft preparation. JY and ZCL: investigation. LW and MQ: writing– review and editing. MQ: supervision. MQ and JY: funding acquisition. All authors contributed to the article and approved the submitted version.
Funding
This research was funded by the National Natural Science Foundation of China (Grant Number: 81673185 for MQ) and Science and Technology Council of Shanxi (Grant Number: 201801D121315 for LW), Ph.D. Start-up Fund Project of Shanxi Medical University (BS201728 for LW), and Graduate Innovation Fund of Shanxi Provincial Education Department (Grant Number: 2019BY080 for JY). The funders had no role in study design, data collection and analysis, decision to publish, or preparation of the manuscript.
Conflict of Interest
The authors declare that the research was conducted in the absence of any commercial or financial relationships that could be construed as a potential conflict of interest.
Supplementary Material
The Supplementary Material for this article can be found online at: https://www.frontiersin.org/articles/10.3389/fgene.2020.611276/full#supplementary-material
References
Abbasi, J. (2017). The paternal epigenome makes its mark. JAMA 317, 2049–2051. doi: 10.1001/jama.2017.1566
Aquilina, N. J., Delgado-Saborit, J. M., Meddings, C., Baker, S., Harrison, R. M., Jacob, P. III, et al. (2010). Environmental and biological monitoring of exposures to PAHs and ETS in the general population. Environ. Int. 36, 763–771. doi: 10.1016/j.envint.2010.05.015
Choi, H., Jedrychowski, W., Spengler, J., Camann, D. E., Whyatt, R. M., Rauh, V., et al. (2006). International studies of prenatal exposure to polycyclic aromatic hydrocarbons and fetal growth. Environ. Health Perspect. 114, 1744–1750. doi: 10.1289/ehp.8982
Choi, H., Rauh, V., Garfinkel, R., Tu, Y., and Perera, F. P. (2008). Prenatal exposure to airborne polycyclic aromatic hydrocarbons and risk of intrauterine growth restriction. Environ. Health Perspect. 116, 658–665. doi: 10.1289/ehp.10958
Dejmek, J., Solanský, I., Benes, I., Lenícek, J., and Srám, R. J. (2000). The impact of polycyclic aromatic hydrocarbons and fine particles on pregnancy outcome. Environ. Health Perspect. 108, 1159–1164. doi: 10.1289/ehp.001081159
Detmar, J., Rennie, M. Y., Whiteley, K. J., Qu, D., Taniuchi, Y., Shang, X., et al. (2008). Fetal growth restriction triggered by polycyclic aromatic hydrocarbons is associated with altered placental vasculature and AhR-dependent changes in cell death. Am. J. Physiol. Endocrinol. Metab. 295, E519–E530. doi: 10.1152/ajpendo.90436.2008
Duarte-Salles, T., Mendez, M. A., Meltzer, H. M., Alexander, J., and Haugen, M. (2013). Dietary benzo(a)pyrene intake during pregnancy and birth weight: associations modified by vitamin C intakes in the Norwegian Mother and Child Cohort Study (MoBa). Environ. Int. 60, 217–223. doi: 10.1016/j.envint.2013.08.016
Edwards, T. M., Moore, B. C., and Guillette, L. J. Jr. (2006). Reproductive dysgenesis in wildlife: a comparative view. Int. J. Androl. 29, 109–121. doi: 10.1111/j.1365-2605.2005.00631.x
El Hajj, N., Schneider, E., Lehnen, H., and Haaf, T. (2014). Epigenetics and life-long consequences of an adverse nutritional and diabetic intrauterine environment. Reproduction 148, R111–R120. doi: 10.1530/REP-14-0334
Gluckman, P. D., Hanson, M. A., Buklijas, T., Low, F. M., and Beedle, A. S. (2009). Epigenetic mechanisms that underpin metabolic and cardiovascular diseases. Nat. Rev. Endocrinol. 5, 401–408. doi: 10.1038/nrendo.2009.102
Guo, Y., Huo, X., Wu, K., Liu, J., Zhang, Y., and Xu, X. (2012). Carcinogenic polycyclic aromatic hydrocarbons in umbilical cord blood of human neonates from Guiyu, China. Sci. Total Environ. 427-428, 35–40. doi: 10.1016/j.scitotenv.2012.04.007
Hutter, B., Bieg, M., Helms, V., and Paulsen, M. (2010). Imprinted genes show unique patterns of sequence conservation. BMC Genomics 11:649. doi: 10.1186/1471-2164-11-649
Iglesias-Platas, I., Martin-Trujillo, A., Petazzi, P., Guillaumet-Adkins, A., Esteller, M., and Monk, D. (2014). Altered expression of the imprinted transcription factor PLAGL1 deregulates a network of genes in the human IUGR placenta. Hum. Mol. Genet. 23, 6275–6285. doi: 10.1093/hmg/ddu347
Jedrychowski, W., Perera, F. P., Tang, D., Stigter, L., Mroz, E., Flak, E., et al. (2012). Impact of barbecued meat consumed in pregnancy on birth outcomes accounting for personal prenatal exposure to airborne polycyclic aromatic hydrocarbons: Birth cohort study in Poland. Nutrition 28, 372–377. doi: 10.1016/j.nut.2011.07.020
Jedrychowski, W. A., Majewska, R., Spengler, J. D., Camann, D., Roen, E. L., and Perera, F. P. (2017). Prenatal exposure to fine particles and polycyclic aromatic hydrocarbons and birth outcomes: a two-pollutant approach. Int. Arch. Occupat. Environ. Health 90, 255–264. doi: 10.1007/s00420-016-1192-9
Joomyeong, K., Wesley, D. F., Hongzhi, H., Hana, K., Muhammad, B. E., Arundhati, B., et al. (2013). Peg3 mutational effects on reproduction and placenta-specific gene families. PLoS One 8:e83359.
Knezovich, J. G., and Ramsay, M. (2012). The effect of preconception paternal alcohol exposure on epigenetic remodeling of the h19 and rasgrf1 imprinting control regions in mouse offspring. Front. Genet. 3:10. doi: 10.3389/fgene.2012.00010
Lamichhane, D. K., Leem, J. H., Kim, H. C., Lee, J. Y., Park, M. S., Jung, D. Y., et al. (2016). Impact of prenatal exposure to polycyclic aromatic hydrocarbons from maternal diet on birth outcomes: a birth cohort study in Korea. Public Health Nutr. 19, 2562–2571. doi: 10.1017/S1368980016000550
Langlois, P. H., Hoyt, A. T., Desrosiers, T. A., Lupo, P. J., Lawson, C. C., Waters, M. A., et al. (2014). Maternal occupational exposure to polycyclic aromatic hydrocarbons and small for gestational age offspring. Occupat. Environ. Med. 71, 529–535. doi: 10.1136/oemed-2013-101833
Lankova, D., Urbancova, K., Sram, R. J., Hajslova, J., and Pulkrabova, J. (2016). A novel strategy for the determination of polycyclic aromatic hydrocarbon monohydroxylated metabolites in urine using ultra-high-performance liquid chromatography with tandem mass spectrometry. Anal. Bioanal. Chem. 408, 2515–2525. doi: 10.1007/s00216-016-9350-1
Lee, S. W. (2001). The role of tissue-specific imprinting as a source of phenotypic heterogeneity in human disease. Bio. Biol. Psychiatry 50, 927–931.
Leitner, Y., Fattal-Valevski, A., Geva, R., Eshel, R., Toledano-Alhadef, H., Rotstein, M., et al. (2007). Neurodevelopmental outcome of children with intrauterine growth retardation: a longitudinal, 10-year prospective study. J. Child Neurol. 22, 580–587. doi: 10.1177/0883073807302605
Lewtas, J. (2007). Air pollution combustion emissions: characterization of causative agents and mechanisms associated with cancer, reproductive, and cardiovascular effects. Mutat. Res. 636, 95–133. doi: 10.1016/j.mrrev.2007.08.003
Lu, Z., Ma, Y., Gao, L., Li, Y., Li, Q., and Qiang, M. (2018). Urine mercury levels correlate with DNA methylation of imprinting gene H19 in the sperm of reproductive-aged men. PLoS One 13:e0196314. doi: 10.1371/journal.pone.0196314
Ma, Y., Lu, Z., Wang, L., and Qiang, M. (2019). Correlation of internal exposure levels of polycyclic aromatic hydrocarbons to methylation of imprinting genes of sperm DNA. Int. J. Environ. Res. Public Health 16:2606. doi: 10.3390/ijerph16142606
Marques, C. J., Carvalho, F., Sousa, M., and Barros, A. (2004). Genomic imprinting in disruptive spermatogenesis. Lancet 363, 1700–1702. doi: 10.1016/S0140-6736(04)16256-9
McMinn, J., Wei, M., Sadovsky, Y., Thaker, H. M., and Tycko, B. (2006). Imprinting of PEG1/MEST isoform 2 in human placenta. Placenta 27, 119–126.
Montoya-Williams, D., Quinlan, J., Clukay, C., Rodney, N. C., Kertes, D. A., and Mulligan, C. J. (2017). Associations between maternal prenatal stress, methylation changes in IGF1 and IGF2, and birth weight. J. Dev. Orig. Health Dis. 9, 215–222. doi: 10.1017/S2040174417000800
Mumtaz, M., and George, J. (1995). Toxicological Profile for Polycyclic Aromatic Hydrocarbons. Atlanta, GA: US Department of Health and Human Services.
Nassar, N., Abeywardana, P., Barker, A., and Bower, C. (2010). Parental occupational exposure to potential endocrine disrupting chemicals and risk of hypospadias in infants. Occupat. Environ. Med. 67, 585–589. doi: 10.1136/oem.2009.048272
Ost, A., Lempradl, A., Casas, E., Weigert, M., Tiko, T., Deniz, M., et al. (2014). Paternal diet defines offspring chromatin state and intergenerational obesity. Cell 159, 1352–1364. doi: 10.1016/j.cell.2014.11.005
Padula, A. M., Noth, E. M., Hammond, S. K., Lurmann, F. W., Yang, W., Tager, I. B., et al. (2014). Exposure to airborne polycyclic aromatic hydrocarbons during pregnancy and risk of preterm birth. Environ. Res. 135, 221–226. doi: 10.1016/j.envres.2014.09.014
Pedersen, M., Schoket, B., Godschalk, R. W., Wright, J., von Stedingk, H., Tornqvist, M., et al. (2013). Bulky dna adducts in cord blood, maternal fruit-and-vegetable consumption, and birth weight in a European mother-child study (NewGeneris). Environ. Health Perspect. 121, 1200–1206. doi: 10.1289/ehp.1206333
Perera, F. P., Rauh, V., Whyatt, R. M., Tang, D., Tsai, W. Y., Bernert, J. T., et al. (2005). A summary of recent findings on birth outcomes and developmental effects of prenatal ETS, PAH, and pesticide exposures. NeuroToxicol. 26, 573–587. doi: 10.1016/j.neuro.2004.07.007
Polanska, K., Dettbarn, G., Jurewicz, J., Sobala, W., Magnus, P., Seidel, A., et al. (2014). Effect of prenatal polycyclic aromatic hydrocarbons exposure on birth outcomes: the Polish mother and child cohort study. BioMed Res. Int. 2014, 1–10.
Radford, E. J., Ferrón, S. R., and Ferguson-Smith, A. C. (2011). Genomic imprinting as an adaptative model of developmental plasticity. FEBS Lett. 585, 2059–2066. doi: 10.1016/j.febslet.2011.05.063
Ramesh, A., Walker, S. A., Hood, D. B., Guillen, M. D., Schneider, K., and Weyand, E. H. (2004). Bioavailability and risk assessment of orally ingested polycyclic aromatic hydrocarbons. Int. J. Toxicol. 23, 301–333. doi: 10.1080/10915810490517063
Rengarajan, T., Rajendran, P., Nandakumar, N., Lokeshkumar, B., Rajendran, P., and Nishigaki, I. (2015). Exposure to polycyclic aromatic hydrocarbons with special focus on cancer. Asian Pac. J. Trop. Biomed. 5, 182–189. doi: 10.1016/S2221-1691(15)30003-4
Risnes, K. R., Vatten, L. J., Baker, J. L., Jameson, K., Sovio, U., Kajantie, E., et al. (2011). Birthweight and mortality in adulthood: a systematic review and meta-analysis. Int. J. Epidemiol. 40, 647–661. doi: 10.1093/ije/dyq267
Shirangi, A., Nieuwenhuijsen, M., Vienneau, D., and Holman, C. D. (2011). Living near agricultural pesticide applications and the risk of adverse reproductive outcomes: a review of the literature. Paediatr Perinat Epidemiol. 25, 172–191. doi: 10.1111/j.1365-3016.2010.01165.x
Singh, V. K., Singh, J., Anand, M., Kumar, P., Patel, D. K., Krishna Reddy, M. M., et al. (2008). Comparison of polycyclic aromatic hydrocarbon levels in placental tissues of Indian women with full- and preterm deliveries. Int. J. Hygiene Environ. Health 211, 639–647. doi: 10.1016/j.ijheh.2007.11.004
Tang, D., Li, T. Y., Liu, J. J., Chen, Y. H., Qu, L., and Perera, F. (2006). PAH-DNA adducts in cord blood and fetal and child development in a Chinese cohort. Environ. Health Perspect. 114, 1297–1300. doi: 10.1289/ehp.8939
Tunster, S. J., Jensen, A. B., and John, R. M. (2013). Imprinted genes in mouse placental development and the regulation of fetal energy stores. Reproduction 145, R117–R137.
Urbancova, K., Lankova, D., Rossner, P., Rossnerova, A., Svecova, V., Tomaniova, M., et al. (2017). Evaluation of 11 polycyclic aromatic hydrocarbon metabolites in urine of Czech mothers and newborns. Sci. Total Environ. 577, 212–219. doi: 10.1016/j.scitotenv.2016.10.165
Us Department of Health and Human Services (1995). Agency for Toxic Substances and Disease Registry. Toxicological Profile for Polycyclic Aromatic Hydrocarbons (PAHs),(update) PB/95/264370. Atlanta, GA: US Department of Health and Human Services.
Vidal, A. C., Neelon, S. E. B., Liu, Y., Tuli, A. M., Fuemmeler, B. F., Hoyo, C., et al. (2014). Maternal Stress, preterm birth, and DNA methylation at imprint regulatory sequences in humans. Genet. Epigen. 6:S18067. doi: 10.4137/geg.s18067
Wilhelm, M., Ghosh, J. K., Su, J., Cockburn, M., Jerrett, M., and Ritz, B. (2011). Traffic-related air toxics and preterm birth: a population-based case-control study in Los Angeles County, California. Environ. Health 10:89. doi: 10.1186/1476-069X-10-89
Yuan, H. -f., Kuete, M., Su, L., Yang, F., Hu, Z. -y., Tian, B. -z., et al. (2015). Comparison of three different techniques of human sperm DNA isolation for methylation assay. J. Huazhong Univ. Sci. Technol. [Med. Sci.] 35, 938–942. doi: 10.1007/s11596-015-1532-0
Zhang, W., Yang, J., Lv, Y., Li, S., and Qiang, M. (2019). Paternal benzo[a]pyrene exposure alters the sperm DNA methylation levels of imprinting genes in F0 generation mice and their unexposed F1-2 male offspring. Chemosphere 228, 586–594. doi: 10.1016/j.chemosphere.2019.04.092
Keywords: polycyclic aromatic hydrocarbons, birth outcomes, imprinted genes, methylation, sperm
Citation: Yang J, Lu Z, Liu Z, Wang L and Qiang M (2021) Methylation of Imprinted Genes in Sperm DNA Correlated to Urinary Polycyclic Aromatic Hydrocarbons (PAHs) Exposure Levels in Reproductive-Aged Men and the Birth Outcomes of the Offspring. Front. Genet. 11:611276. doi: 10.3389/fgene.2020.611276
Received: 28 September 2020; Accepted: 03 December 2020;
Published: 11 January 2021.
Edited by:
Hehuang Xie, Virginia Tech, United StatesReviewed by:
Apiwat Mutirangura, Chulalongkorn University, ThailandNaoko Hattori, National Cancer Center Research Institute, Japan
Copyright © 2021 Yang, Lu, Liu, Wang and Qiang. This is an open-access article distributed under the terms of the Creative Commons Attribution License (CC BY). The use, distribution or reproduction in other forums is permitted, provided the original author(s) and the copyright owner(s) are credited and that the original publication in this journal is cited, in accordance with accepted academic practice. No use, distribution or reproduction is permitted which does not comply with these terms.
*Correspondence: Li Wang, wangli_1@sxmu.edu.cn; Mei Qiang, qiang@uthscsa.edu
†Present address: Zhaoxu Lu, Capital Institute of Pediatrics, Graduate Schools of Peking Union Medical College, Beijing, China