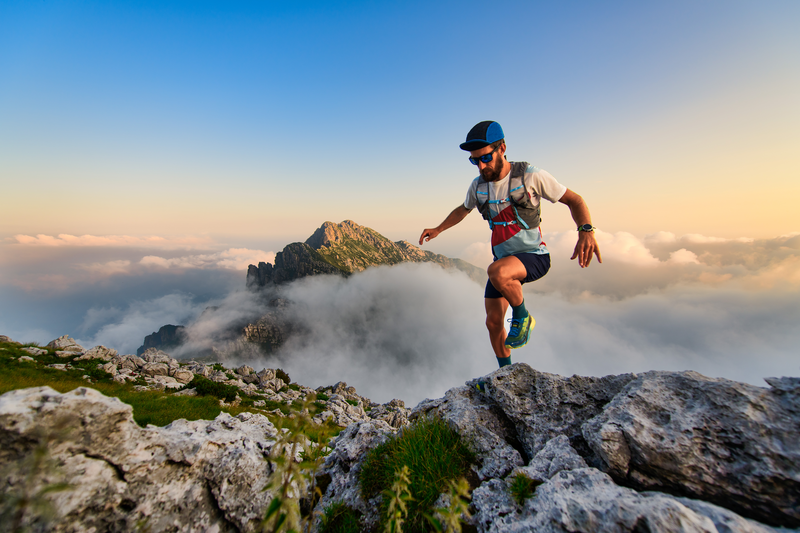
95% of researchers rate our articles as excellent or good
Learn more about the work of our research integrity team to safeguard the quality of each article we publish.
Find out more
ORIGINAL RESEARCH article
Front. Genet. , 18 December 2020
Sec. Genetics of Common and Rare Diseases
Volume 11 - 2020 | https://doi.org/10.3389/fgene.2020.591434
The clinical utility of genetic testing for epilepsy has been enhanced with the advancement of next-generation sequencing (NGS) technology along with the rapid updating of publicly available databases. The aim of this study was to evaluate the diagnostic yield of NGS and assess the value of reinterpreting genetic test results in children and adults with epilepsy. We performed genetic testing on 200 patients, including 82 children and 118 adults. The results were classified into three categories: positive, inconclusive, or negative. The reinterpretation of inconclusive results was conducted in April 2020. Overall, we identified disease-causing variants in 12% of the patients in the original analysis, and 14.5% at reinterpretation. The diagnostic yield for adults with epilepsy was similar to that for children (11 vs. 19.5%, p = 0.145). After reinterpretation, 9 of the 86 patients who initially had inconclusive results obtained a clinically significant change in diagnosis. Among these nine revised cases, five obtained positive diagnoses, representing a diagnosis rate of 5.8% (5/86). Manual searches for additional evidence of pathogenicity for candidate variants and updated patient clinical information were the main reasons for diagnostic reclassification. This study emphasizes the diagnostic potential of combining NGS and reinterpretation of inconclusive genetic test reports in children and adults with epilepsy.
Epilepsy is a common and highly heterogeneous neurological disease, with an estimated prevalence of 6 per 1,000 in the general population (Fiest et al., 2017). Genetic factors have long been recognized as playing an important role in the development of this disorder, a rationale supported by family studies and the identification of specific epilepsy syndrome-causing variants (Myers and Mefford, 2015; Perucca et al., 2020). Moreover, it is now thought that more than 70% of epilepsy cases have a genetic basis in both children and adults (Myers et al., 2019; Scala et al., 2020). Consequently, genomic testing of patients with epilepsy is becoming increasingly routine in clinical practice, given that detecting genetic causes may provide accurate prognoses and optimize the management and treatment options for some epileptic patients. However, the phenotypes associated with epilepsy syndromes are often variable and unspecific, involving several genes, while specific genetic variants are frequently associated with a wide phenotypic spectrum (Zhu et al., 2014). This considerable heterogeneity makes it challenging to precisely identify the underlying genetic cause of most epilepsies.
The emergence of next-generation sequencing (NGS) technologies, such as targeted gene panels, whole-exome sequencing (WES), and whole-genome sequencing (WGS), has revolutionized the application of genetic testing and made it technically practicable to evaluate hundreds of genes in a single test (Symonds and McTague, 2020). Over the past decades, NGS approaches have led to the identification of several epilepsy-related genes and expanded the knowledge of phenotypes associated with known genes (Dunn et al., 2018). The reported diagnostic yield of NGS for patients with epilepsy ranges from 10 to 40%, depending on the method of analysis used and the phenotypes among the studied cohorts (Butler et al., 2017; Mei et al., 2017; Perucca et al., 2017). However, although NGS has the potential to improve the detection of disease-causing variants, data interpretation remains the main challenge, especially for variants of uncertain significance, given our incomplete knowledge about the function of individual disease-causing variants and genes (Duzkale et al., 2013; Kassahn et al., 2014; Seaby and Ennis, 2020).
The reinterpretation of genetic data has proven to be an effective means of revealing new disease-causing variants and can increase the diagnostic yield (Wenger et al., 2017). Indeed, the American College of Medical Genetics and Genomics (ACMG) has proposed that previously reported genetic variants be periodically reviewed (Richards et al., 2015; Deignan et al., 2019). Thus, owing to the rapid advances in publicly available databases and the ongoing updating of the clinical phenotypes of the patients, it is particularly important in epilepsy to periodically reinterpret genetic test reports (Epilepsy Genetics Initiative, 2019; Rochtus et al., 2020). Here, we aimed to evaluate the clinical utility and diagnostic potential of reinterpreting NGS results in a cohort of 200 pediatric and adult epileptic patients.
From December 2015 to February 2019, a total of 200 patients were recruited sequentially from the Department of neurology of the Comprehensive Epilepsy Center in Xijing Hospital, a tertiary academic hospital in Shaanxi Province, China. For an etiological diagnosis, 80 patients were tested using a commercially available gene panel and 120 patients were tested by WES. The demographic and clinical characteristics, seizure history, EEG findings, brain imaging reports, and antiepileptic drug (AED) medications used were collected and summarized. Epilepsy syndromes and types were classified according to the consensus proposed by the International League Against Epilepsy (ILAE) (Scheffer et al., 2017). Familial history was defined as a history of epilepsy in first-degree relatives. Informed consent was obtained from the patients and their parents/legal guardians. This study was approved by the ethics committee of Xijing Hospital and conducted in agreement with the relevant guidelines and regulations.
Genomic DNA was extracted from peripheral blood. The exome was captured using either the GenCap custom enrichment kit (including 153 epilepsy-related genes; MyGenostics Inc., Beijing, China, Supplementary Table 1) or SureSelect XTHuman All Exon v4 (Agilent Technologies, Santa Clara, CA, United States) following the manufacturers’ protocols. The enriched libraries were sequenced on the Illumina HiSeq X Ten sequencer (Illumina, San Diego, CA, United States) with a paired-end read length of 150 bp. For the two methods, the average on targeted sequencing depth was 334.50× with 95.71% regions were covered at greater than 20×, and was 160.11× with 97.41% regions were covered at greater than 20×, respectively (Supplementary Table 2). Raw image files were processed using Bcl2Fastq conversion software (Bcl2Fastq 2.18.0.12, Illumina) or cutadapt v1.16 for base calling and raw data generation. Clean reads were aligned to the reference human genome (hg19) using BWA v0.7.15 or v0.7.12-r1044. Duplicated reads were removed using the Picard program, and SNP and indel variants were detected using GATK v3.7 or v4.0. The identified variants were annotated using Exome-assistant or ANNOVAR1. MagicViewer or IGV (Integrative Genomics Viewer) v2.8.2 was used to view short-read alignments and confirm the candidate SNPs and indels.
In this study, we analyzed exome sequence data from 200 families: 193 non-trios (proband only; 80 were tested using a panel and 113 were tested by WES); 7 trios (proband and both parents). For the seven trios, paternity/maternity was checked by a freely available software package, KING2 using NGS data. For the 193 non-trios, paternity/maternity were detected by short tandem repeats (STR) analysis.
Variants were filtered and prioritized based on the following parameters: (i) Variant reads should be more than 5 and the mutation ratio should be no less than 30%; (ii) the minor allele frequency (MAF) should be <1% in several databases, such as dbSNP138, 1000 Genomes, and ESP6500; and should not be present in the InNormal database (MyGenostics); (iii) conservation using GERP++, and pathogenicity prediction from Mutation Taster, SIFT, and PolyPhen; (iv) if the variants were synonymous and they were reported in HGMD or ClinVar, left them; and (v) association(s) with human neurological disease (s) (for WES data). Variants were further filtered based on genotype and possible inheritance models. The filtered variants were reviewed according to the recommendation of the ACMG (Richards et al., 2015) and were considered pathogenic based on the phenotype and family history of the patients. All putative causative variants identified by NGS were confirmed by conventional Sanger sequencing. To detect the inheritance status, segregation analyses of variants with the phenotype were performed in the family members of the probands who were available for molecular screening.
The genetic results were classified into three categories: (i) positive results, where a clinically significant variant (pathogenic or likely pathogenic) identified in a gene could completely explain the phenotypes of a case; (ii) negative results, where no variants were initially reported in the genetic test or where variants were classified as likely benign/benign after reinterpretation; (iii) inconclusive results [all other results not in categories i and ii, including genetic results reported variants of unknown significance (VUS)].
Repeat interpretation was conducted by removing cases with either a positive genetic diagnosis or a negative result from the overall cohort in April 2020. Reinterpretation was performed based on updated information in databases for population frequency (Exome Aggregation Consortium, 1000 Genomes) or clinical association (Human Gene Mutation Database, ClinVar), manually reviewing the literature, and additional phenotypes of the patients for which the reports were inconclusive.
Categorical variables were expressed as counts and percentages. Continuous variables were summarized using medians and interquartile ranges (IQRs). Chi-square tests with Bonferroni correction or Fisher’s exact test were used to evaluate the proportions of diagnostic results among categorical variables. Two-tailed p < 0.01 were considered statistically significant. Statistical analysis was performed using IBM SPSS for Windows v.26.0 (IBM, New York, United States).
Our cohort consisted of 200 patients with genetically unexplained epilepsy. Among them, 82 (41%) were children and 118 (59%) were adults, with 104 males (52%) and 96 females (48%) (sex ratio M:F = 1.1:1). The mean age was 15.5 years (SD = ±4.7, median = 15 years) and ranged from 2 to 50 years at testing. Generalized epilepsy was reported in 101 cases (50.5%), focal epilepsy in 49 patients (24.5%), combined generalized and focal epilepsy in 41 patients (20.5%), while unknown epilepsy type was reported for 9 patients (4.5%). The median age at seizure onset was 12 years (IQR, 6.3–15 years). Thirty-four (17%) of the participants had some degree of delay or cognitive impairment, including developmental delay, learning disability, speech delay, and/or behavioral issues (referred to as seizure plus). In total, 21.5% of the patients (43/200) had a family history of epilepsy.
Over the 52 months and after reinterpretation, 29 of the initial 200 patients (14.5%) obtained a positive molecular diagnosis, with 31 variants spanning 19 genes being identified (Supplementary Table 3). Testing in 77 patients (38.5%) yielded inconclusive findings, and 94 patients (47%) had negative results. Genes identified in more than one patient who had obtained a positive result were SCN1A (n = 4), GABRG2 (n = 3), LGI1 (n = 3), TSC2 (n = 3), and CHRNA4 (n = 2), accounting for 26.3% (5/19) of all mutated genes in our study (Figure 1A). The distribution of mode of inheritance and variant type is presented in Figure 1B. Among the 26 autosomal dominant cases, 16 (61.5%) were inherited, and 10 (38.5%) were linked to a de novo variant. Two cases were associated with X-linked dominant disorders, one carrying a de novo variant and the other an undetermined variant. And one patient diagnosed with autosomal recessive disorders was compound heterozygotes.
Figure 1. The distribution of mutated genes, mode of inheritance, and variant type for cases with a positive molecular diagnosis. (A) y-axis: the number of patients; x-axis: genes carrying presumed pathogenic variants. (B) AD, autosomal dominant; AR, autosomal recessive; XL, X-linked disorders.
The diagnostic yield varied significantly according to age at seizure onset and neurodevelopmental phenotype. The positive rate was highest in patients under than 6 years of age at seizure onset (14/50, 28%), followed by those aged 6–18 years (14/122, 11.5%), contributing 14% to the overall diagnostic yield in the cohort. Furthermore, in our study, patients with seizure plus were significantly more likely to have a positive result (35.3%, p = 0.000). However, there was no significant difference in yield between children and adults with epilepsy (19.5 vs. 11%, p = 0.145). Additionally, the test sensitivity for the detection of the relevant genetic information was not significantly different (p = 0.673) between probands tested with the epilepsy gene panel (13/80, 16.3%) and those tested using WES (16/120, 13.4%) (Table 1).
After the first analysis, 24/200 patients (12%) received a positive result, with 25 variants in 17 genes being detected and classified as pathogenic or likely pathogenic (Supplementary Table 3). Ninety patients (45%) had negative results and 86 (43%) obtained inconclusive yields. Additionally, 3 patients with no etiological diagnosis harbored a medically actionable secondary finding in one of the 59 ACMG genes (Supplementary Table 4).
The reinterpretation was done between 14 and 52 months after the report issue date (median 42 months). The reinterpretation of these 86 inconclusive results led to 9 patients (9/86, 10.5%) having a clinically significant change in diagnosis (Table 2 and Figure 2). The second interpretation allowed the identification of five disease-causing variants in 5/86 patients, thereby leading to an additional diagnostic yield of 5.8% (Supplementary Table 3). Three diagnoses resulted from an internal analysis of new disease–gene associations, in which GABRG2 linked to sleep-related hypermotor epilepsy (SHE, we have performed in vitro experiments to investigate these GABRG2 variants’ function but the results have not yet been published). The result of the genetic testing report for patient P5 diagnosed with Rolandic epilepsy (RE), was upgraded to positive based on a recent publication: the author identified the same GRIN2A variant (c.1341T > A p.Asn447Lys) in a boy with RE by WES and demonstrated that Asn447Lys is a gain-of-function variant (Xu et al., 2018). Moreover, an incomplete penetrance of GRIN2A variants associated with RE has been extensively reported (Lemke et al., 2013). Therefore, we reclassified this variant as likely pathogenic based on manual adjustment of ACMG guidelines. For patient W85, who was diagnosed as having genetic epilepsy with febrile seizures plus (GEFS+), a missense variant was detected in SCN9A (c.5231A > G, p.Tyr1744Cys). This variant was inherited from the patient’s mother who suffered from febrile seizures between the ages of 4–7. Through follow-up, we identified the same variant in the patient’s younger sister who was also diagnosed with GEFS+. Moreover, several recent studies supported a causal role of this gene in epilepsy based on case-level data, particularly in GEFS+ (Mulley et al., 2013; Zhang et al., 2020). Therefore, we considered that this variant may be regarded as pathogenic variant in this pedigree and reclassified the result as positive (see Table 2 for additional details). However, given that SCN9A has limited disease-association per the clingen epilepsy working group3, the specific functional experiments considering this variant are needed to provide additional supports.
Table 2. Clinical and genetic characteristics of the patients who obtained a clinically significant change in diagnosis after reinterpretation.
The results of four patients were downgraded after reinterpretation (P20, P25, P45, and W53). In particular, patients P20 and P25, who presented different epilepsy types, were found to harbor the same inherited missense variant in RELN (c.3712A > C, p.Asn1238His), initially rendering the results inconclusive. In the second interpretation, we found that this variant had been submitted to the ClinVar database multiple times and interpreted as Benign as of December 2019. In addition, the MAF of this variant in genomeAD increased from 0.0024 to 0.0075, which was greater than expected for this disorder. The result for case P45 was downgraded also as a result of new information in ClinVar and population databases. For patient W53, an inherited missense variant was detected in SCN3A (c.1687C > T, p.Arg563Cys). Through follow-up, this variant was also detected in the patient’s healthy grandmother and younger sister. Moreover, as of January 2020, this variant had been submitted once to the ClinVar database and interpreted as Likely benign (Table 2).
Among the nine revised diagnoses, the most frequently reclassified variants for both upgraded and downgraded yields were missense (7/8, 87.5%), followed by nonsense (1/8, 12.5%). For the upgraded results, diagnostic reclassification was based on a manual analysis involving a literature review or analysis of cases with similar phenotypes (4/5, 80%), and updated information in medical records due to follow-up (1/8, 20%). Inconclusive results were downgraded mainly because of updated information in clinical and population databases (Figure 3).
Figure 3. Results reinterpreted in the study. (A) Number of reclassified variants; (B) evidence for change.
In the present study, we reported the NGS for 200 consecutive patients with suspected genetic epilepsy included over 3 years. The results highlight the clinical utility of using NGS and the importance of reinterpreting genetic test reports in epilepsy. We identified disease-causing variants in 14.5% of the patients after initial analysis and reinterpretation reports, which is comparable to previous NGS-related reports for epilepsy (Berg et al., 2017; Butler et al., 2017; Perucca et al., 2017). We also showed that NGS can be a valuable tool for detecting the etiology of adult epilepsy. Despite the recent advances in the molecular genetics of this condition, genetic evaluation is often overlooked in adults. In our study, a genetic diagnosis was made in 13 (11%) of 118 adults, which was similar to the diagnostic yield found in pediatric epilepsy (p = 0.145). However, our yield was slightly lower than that obtained in previous studies in adults, in which a genetic cause was identified in 22 or 23% of cases, respectively (Borlot et al., 2019; Johannesen et al., 2020). The higher yield in the other studies may have been due to most patients also presenting with ID and/or developmental delay. Although not statistically significant, we noticed that the diagnostic rate is higher in focal than generalized epilepsy (24.5 vs. 7.9%), which is different to what has been described in larger cohort studies (Epi4K consortium and Epilepsy Phenome/Genome Project, 2017; Al-Nabhani et al., 2018). Since our clinic is in a tertiary hospital, which is the largest medical care center in the northwestern China, quite many patients with focal epilepsy whose phenotypes are less severe might just go to local hospitals instead of coming all the way here for further diagnosis and treatment. This may be the reason why patients with focal epilepsy were more likely to accompany with ID than patients with generalized epilepsy in our study (9/49, 18.5% vs. 11/101, 10.9%). Evidence from our study and the studies of some others suggested that epilepsy patients with ID were significantly more likely to have a positive result (Borlot et al., 2019; Johannesen et al., 2020). Thus, at least in part, explain that the diagnostic rate is higher in focal vs. generalized epilepsy in our study.
A key challenge associated with the clinical utility of NGS is data interpretation, especially for variants of uncertain significance (Duzkale et al., 2013; Kassahn et al., 2014; Seaby and Ennis, 2020). Besides, with the rapid advances publicly available databases and the ongoing updating of clinical information of the patients over time, it appears manageable and worthwhile to reinterpret NGS reports for patients with inconclusive genetic test results (Nambot et al., 2018; Epi25 Collaborative, 2019; SoRelle et al., 2019). This strategy allowed us to obtain 9 (10.5%) clinically significant changes in diagnosis among the 86 initially inconclusive results, reinforcing the need for reinterpretation. Among the nine patients with revised cases, five obtained positive diagnoses. This represents a diagnosis rate of 5.8% (5/86) and a marked increase in diagnostic yield overall, which is similar to a recent study (Epilepsy Genetics Initiative) made 5.8% (8/139) new diagnoses during a systematic reanalysis of unresolved WES data (Epilepsy Genetics Initiative, 2019).
The major reason that led to our revised results after reinterpretation was the manual search for additional evidence of pathogenicity for candidate variants and the discovery of new gene-disease relationships. SHE is a focal epilepsy characterized by hypermotor seizures occurring predominantly in clusters during non-rapid eye movement sleep, which is a heterogeneous genetic syndrome, and the transmission pattern of the disorder is compatible with an autosomal dominant inheritance with reduced penetrance (Tinuper et al., 2016). Currently, variants in genes coding for subunits of the heteromeric neuronal nicotinic receptors (nAchR) are the most commonly identified pathogenic variants in SHE (Becchetti et al., 2015). In our SHE patients (P7, W48, and W58), we detected three variants (NM_198903:c.1070C > A, p.Thr357Asn, de novo; c.649C > T, p.Gln217X, inherited from unaffected father; c.269C > T, p.Thr90Met, inherited from unaffected mother) in GABRG2 at different time points (Table 2). Mutations in GABRG2 are associated with febrile seizures (FS) and various genetic epilepsy syndromes, which suggests a broad range of phenotypical spectrum associated with GABRG2 variants (Kang and Macdonald, 2016). And an incomplete penetrance has previously been noted for some GABRG2 mutation-positive families (Johnston et al., 2014). Additionally, by searching and reviewing the corresponding literature, we found that previously reported SHE variants are associated with large selective increases in nicotine-evoked GABAergic inhibition, a likely key factor in epileptogenesis, as the seizures in vivo can be blocked by subconvulsive doses of GABAA receptor antagonists (Klaassen et al., 2006). Besides, our experiments on HEK293T cells (unpublished data) have shown that these GABRG2 variants decrease GABA-evoked currents, and provided further evidence for the pathogenicity of the three GABRG2 variants in relation to SHE. Combined, these observations suggest that the variants in GABRG2 are associated with GABAergic disinhibition, thereby promoting SHE epileptogenesis. These variants were not initially reported as pathogenic because of the time lag between these genetic test reports. However, reinterpretation allowed us to re-evaluate these candidate variants over time, which not only benefited the patients, but also expanded the genetic spectrum for epilepsy.
Accurate and comprehensive clinical information was essential for correct and timely diagnosis. Follow-up and updated family segregation data, as in the case of patient W85, allowed us to assign a positive result after reinterpretation. Timely updated information in clinical and population databases also helped uncover new evidence that weakened the support for a particular diagnosis (Table 2: patients P20, P25, P45, and W53). This suggests that a clinician with experience in interpreting genetic diagnoses should take advantage of these resources and periodically perform a systematic review.
This study had several limitations. The patient sample size was relatively small, and there was a possible selection bias as we only enrolled patients who were referred to our Comprehensive Epilepsy Center. Additionally, patients with initially negative results were arbitrarily excluded at reinterpretation owing to unreported variants. This may have led to the absence of an analysis of benign variants that could have been upgraded to pathogenic or uncertain variants.
In conclusion, we have highlighted the clinical utility of NGS and the importance of reinterpreting inconclusive genetic test reports in epileptic patients. Our results demonstrated the importance of keeping up with expanding knowledge, and stress that patients should be informed that medicine is constantly evolving and genetic test results may change over time. Additionally, our results indicated that routine diagnostic genetic testing should also be considered for adults with epilepsy.
The datasets for this article are not publicly available due to concerns regarding participant/patient anonymity. Requests to access the datasets should be directed to the WJ, ZHJqaWFuZ3dlbkBob3RtYWlsLmNvbQ==.
The studies involving human participants were reviewed and approved by the ethics committee of the Xijing Hospital. Written informed consent to participate in this study was provided by the participants’ legal guardian/next of kin. Written informed consent was obtained from the individual(s), and minor(s)’ legal guardian/next of kin, for the publication of any potentially identifiable images or data included in this article.
YJ: study concept and design, drafting of the manuscript, critical revision, statistical analysis, and study supervision. CS and YW: genetic analysis and study supervision. JZ, FY, QG, XL, and YM: data acquisition, analysis, and interpretation. WJ: study concept and design, critical revision, and obtained funding. All authors contributed to the article and approved the submitted version.
This study was supported by the National Natural Science Foundation of China (Grant No. 81974204).
The authors declare that the research was conducted in the absence of any commercial or financial relationships that could be construed as a potential conflict of interest.
We thank the patients for their participation in this study.
The Supplementary Material for this article can be found online at: https://www.frontiersin.org/articles/10.3389/fgene.2020.591434/full#supplementary-material
Al-Nabhani, M., Al-Rashdi, S., Al-Murshedi, F., Al-Kindi, A., Al-Thihli, K., Al-Saegh, A., et al. (2018). Reanalysis of exome sequencing data of intellectual disability samples: yields and benefits. Clin. Genet. 94, 495–501. doi: 10.1111/cge.13438
Becchetti, A., Aracri, P., Meneghini, S., Brusco, S., and Amadeo, A. (2015). The role of nicotinic acetylcholine receptors in autosomal dominant nocturnal frontal lobe Epilepsy. Front. Physiol. 6:22. doi: 10.3389/fphys.2015.00022
Berg, A. T., Coryell, J., Saneto, R. P., Grinspan, Z. M., Alexander, J. J., Kekis, M., et al. (2017). Early-life epilepsies and the emerging role of genetic testing. JAMA Pediatr. 171, 863–871. doi: 10.1001/jamapediatrics.2017.1743
Borlot, F., de Almeida, B. I., Combe, S. L., Andrade, D. M., Filloux, F. M., and Myers, K. A. (2019). Clinical utility of multigene panel testing in adults with Epilepsy and intellectual disability. Epilepsia 60, 1661–1669. doi: 10.1111/epi.16273
Butler, K. M., Da, S. C., Alexander, J. J., Hegde, M., and Escayg, A. (2017). Diagnostic yield from 339 Epilepsy patients screened on a clinical gene panel. Pediatr. Neurol. 77, 61–66. doi: 10.1016/j.pediatrneurol.2017.09.003
Deignan, J. L., Chung, W. K., Kearney, H. M., Monaghan, K. G., Rehder, C. W., and Chao, E. C. (2019). Points to consider in the reevaluation and reanalysis of genomic test results: a statement of the American College of Medical Genetics and Genomics (ACMG). Genet. Med. 21, 1267–1270. doi: 10.1038/s41436-019-0478-1
Dunn, P., Albury, C. L., Maksemous, N., Benton, M. C., Sutherland, H. G., Smith, R. A., et al. (2018). Next generation sequencing methods for diagnosis of Epilepsy syndromes. Front. Genet. 9:20. doi: 10.3389/fgene.2018.00020
Duzkale, H., Shen, J., McLaughlin, H., Alfares, A., Kelly, M. A., Pugh, T. J., et al. (2013). A systematic approach to assessing the clinical significance of genetic variants. Clin. Genet. 84, 453–463. doi: 10.1111/cge.12257
Epi25 Collaborative (2019). Electronic address:cy5iZXJrb3ZpY0B1bmltZWxiLmVkdS5hdQ==; Epi25 collaborative. ultra-rare genetic variation in the epilepsies: a whole-exome sequencing study of 17,606 Individuals. Am. J. Hum. Genet. 105, 267–282. doi: 10.1016/j.ajhg.2019.05.020
Epi4K consortium and Epilepsy Phenome/Genome Project (2017). Ultra-rare genetic variation in common epilepsies: a case-control sequencing study. Lancet Neurol. 16, 135–143. doi: 10.1016/S1474-4422(16)30359-3
Epilepsy Genetics Initiative (2019). The Epilepsy genetics initiative: systematic reanalysis of diagnostic exomes increases yield. Epilepsia 60, 797–806. doi: 10.1111/epi.14698
Fiest, K. M., Sauro, K. M., Wiebe, S., Patten, S. B., Kwon, C. S., Dykeman, J., et al. (2017). Prevalence and incidence of Epilepsy: a systematic review and meta-analysis of international studies. Neurology 88, 296–303. doi: 10.1212/WNL.0000000000003509
Johannesen, K. M., Nikanorova, N., Marjanovic, D., Pavbro, A., Larsen, L. H. G., Rubboli, G., et al. (2020). Utility of genetic testing for therapeutic decision-making in adults with Epilepsy. Epilepsia 61, 1234–1239. doi: 10.1111/epi.16533
Johnston, A. J., Kang, J. Q., Shen, W., Pickrell, W. O., Cushion, T. D., Davies, J. S., et al. (2014). A novel GABRG2 mutation, p.R136∗, in a family with GEFS+ and extended phenotypes. Neurobiol. Dis. 64, 131–141. doi: 10.1016/j.nbd.2013.12.013
Kang, J. Q., and Macdonald, R. L. (2016). Molecular pathogenic basis for GABRG2 mutations associated with a spectrum of Epilepsy syndromes, from generalized absence Epilepsy to dravet syndrome. JAMA Neurol 73, 1009–1016. doi: 10.1001/jamaneurol.2016.0449
Kassahn, K. S., Scott, H. S., and Caramins, M. C. (2014). Integrating massively parallel sequencing into diagnostic workflows and managing the annotation and clinical interpretation challenge. Hum. Mutat. 35, 413–423. doi: 10.1002/humu.22525
Klaassen, A., Glykys, J., Maguire, J., Labarca, C., Mody, I., and Boulter, J. (2006). Seizures and enhanced cortical GABAergic inhibition in two mouse models of human autosomal dominant nocturnal frontal lobe Epilepsy. Proc. Natl. Acad. Sci. U.S.A. 103, 19152–19157. doi: 10.1073/pnas.0608215103
Lemke, J. R., Lal, D., Reinthaler, E. M., Steiner, I., Nothnagel, M., Alber, M., et al. (2013). Mutations in GRIN2A cause idiopathic focal Epilepsy with rolandic spikes. Nat. Genet. 45, 1067–1072. doi: 10.1038/ng.2728
Mei, D., Parrini, E., Marini, C., and Guerrini, R. (2017). The impact of next-generation sequencing on the diagnosis and treatment of Epilepsy in paediatric patients. Mol. Diagn. Ther. 21, 357–373. doi: 10.1007/s40291-017-0257-0
Mulley, J. C., Hodgson, B., McMahon, J. M., Iona, X., Bellows, S., Mullen, S. A., et al. (2013). Role of the sodium channel SCN9A in genetic Epilepsy with febrile seizures plus and Dravet syndrome. Epilepsy 54, e122–e126. doi: 10.1111/epi.12323
Myers, C. T., and Mefford, H. C. (2015). Advancing Epilepsy genetics in the genomic era. Genome Med. 7, 91–101. doi: 10.1186/s13073-015-0214-7
Myers, K. A., Johnstone, D. L., and Dyment, D. A. (2019). Epilepsy genetics: current knowledge, applications, and future directions. Clin. Genet. 95, 95–111. doi: 10.1111/cge.13414
Nambot, S., Thevenon, J., Kuentz, P., Duffourd, Y., Tisserant, E., Bruel, A. L., et al. (2018). Clinical whole-exome sequencing for the diagnosis of rare disorders with congenital anomalies and/or intellectual disability: substantial interest of prospective annual reanalysis. Genet. Med. 20, 645–654. doi: 10.1038/gim.2017.162
Perucca, P., Bahlo, M., and Berkovic, S. F. (2020). The genetics of Epilepsy. Annu. Rev. Genomics Hum. Genet. 21, 205–230. doi: 10.1146/annurev-genom-120219-074937
Perucca, P., Scheffer, I. E., Harvey, A. S., James, P. A., Lunke, S., Thorne, N., et al. (2017). Real-world utility of whole exome sequencing with targeted gene analysis for focal Epilepsy. Epilepsy Res. 131, 1–8. doi: 10.1016/j.eplepsyres.2017.02.001
Richards, S., Aziz, N., Bale, S., Bick, D., Das, S., Gastier-Foster, J., et al. (2015). Standards and guidelines for the interpretation of sequence variants: a joint consensus recommendation of the American College of Medical Genetics and genomics and the association for molecular pathology. Genet. Med. 17, 405–424. doi: 10.1038/gim.2015.30
Rochtus, A., Olson, H. E., Smith, L., Keith, L. G., El Achkar, C., Taylor, A., et al. (2020). Genetic diagnoses in Epilepsy: the impact of dynamic exome analysis in a pediatric cohort. Epilepsia 61, 249–258. doi: 10.1111/epi.16427
Scala, M., Bianchi, A., Bisulli, F., Coppola, A., Elia, M., Trivisano, M., et al. (2020). Advances in genetic testing and optimization of clinical management in children and adults with Epilepsy. Expert Rev. Neurother. 20, 251–269. doi: 10.1080/14737175.2020.1713101
Scheffer, I. E., Berkovic, S., Capovilla, G., Connolly, M. B., French, J., Guilhoto, L., et al. (2017). ILAE classification of the epilepsies: position paper of the ILAE commission for classification and terminology. Epilepsia 58, 512–521. doi: 10.1111/epi.13709
Seaby, E. G., and Ennis, S. (2020). Challenges in the diagnosis and discovery of rare genetic disorders using contemporary sequencing technologies. Brief Funct. Genomics 19, 243–258. doi: 10.1093/bfgp/elaa009
SoRelle, J. A., Thodeson, D. M., Arnold, S., Gotway, G., and Park, J. Y. (2019). Clinical utility of reinterpreting previously reported genomic Epilepsy test results for pediatric patients. JAMA Pediatr. 173:e182302. doi: 10.1001/jamapediatrics.2018.2302
Symonds, J. D., and McTague, A. (2020). Epilepsy and developmental disorders: next generation sequencing in the clinic. Eur. J. Paediatr. Neurol. 24, 15–23. doi: 10.1016/j.ejpn.2019.12.008
Tinuper, P., Bisulli, F., Cross, J. H., Hesdorffer, D., Kahane, P., Nobili, L., et al. (2016). Definition and diagnostic criteria of sleep-related hypermotor Epilepsy. Neurology 86, 1834–1842. doi: 10.1212/WNL.0000000000002666
Wenger, A. M., Guturu, H., Bernstein, J. A., and Bejerano, G. (2017). Systematic reanalysis of clinical exome data yields additional diagnoses: implications for providers. Genet. Med. 19, 209–214. doi: 10.1038/gim.2016.88
Xu, X. X., Liu, X. R., Fan, C. Y., Lai, J. X., Shi, Y. W., Yang, W., et al. (2018). Functional investigation of a GRIN2A variant associated with rolandic Epilepsy. Neurosci. Bull. 34, 237–246. doi: 10.1007/s12264-017-0182-6
Zhang, T., Chen, M., Zhu, A., Zhang, X., and Fang, T. (2020). Novel mutation of SCN9A gene causing generalized Epilepsy with febrile seizures plus in a Chinese family. Neurol. Sci. 41, 1913–1917. doi: 10.1007/s10072-020-04284-x
Keywords: genetic testing, next-generation sequencing, reinterpretation, epilepsy, adults
Citation: Jiang YL, Song CG, Wang YY, Zhao JJ, Yang F, Gao Q, Leng XX, Man YL and Jiang W (2020) Clinical Utility of Exome Sequencing and Reinterpreting Genetic Test Results in Children and Adults With Epilepsy. Front. Genet. 11:591434. doi: 10.3389/fgene.2020.591434
Received: 04 August 2020; Accepted: 30 November 2020;
Published: 18 December 2020.
Edited by:
Andrew Landstrom, Duke University, United StatesReviewed by:
Gemma Louise Carvill, Northwestern University, United StatesCopyright © 2020 Jiang, Song, Wang, Zhao, Yang, Gao, Leng, Man and Jiang. This is an open-access article distributed under the terms of the Creative Commons Attribution License (CC BY). The use, distribution or reproduction in other forums is permitted, provided the original author(s) and the copyright owner(s) are credited and that the original publication in this journal is cited, in accordance with accepted academic practice. No use, distribution or reproduction is permitted which does not comply with these terms.
*Correspondence: Wen Jiang, ZHJqaWFuZ3dlbkBob3RtYWlsLmNvbQ==
Disclaimer: All claims expressed in this article are solely those of the authors and do not necessarily represent those of their affiliated organizations, or those of the publisher, the editors and the reviewers. Any product that may be evaluated in this article or claim that may be made by its manufacturer is not guaranteed or endorsed by the publisher.
Research integrity at Frontiers
Learn more about the work of our research integrity team to safeguard the quality of each article we publish.