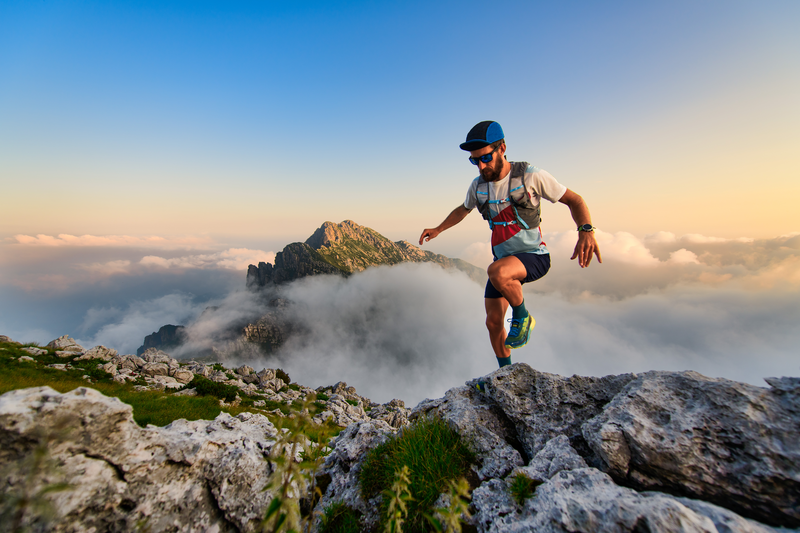
94% of researchers rate our articles as excellent or good
Learn more about the work of our research integrity team to safeguard the quality of each article we publish.
Find out more
ORIGINAL RESEARCH article
Front. Genet. , 30 April 2020
Sec. Evolutionary and Population Genetics
Volume 11 - 2020 | https://doi.org/10.3389/fgene.2020.00383
Human leukocyte antigen (HLA) genotyping displays the particular characteristics of HLA alleles and haplotype frequencies in each population. Although it is considered the current gold standard for HLA typing, high-resolution sequence-based HLA typing is currently unavailable in Kinh Vietnamese populations. In this study, high-resolution sequence-based HLA typing (3-field) was performed using an amplicon-based next-generation sequencing platform to identify the HLA-A, -B, -C, -DRB1, and -DQB1 alleles of 101 unrelated healthy Kinh Vietnamese individuals from southern Vietnam. A total of 28 HLA-A, 41 HLA-B, 21 HLA-C, 26 HLA-DRB1, and 25 HLA-DQB1 alleles were identified. The most frequently occurring HLA alleles were A∗11:01:01, B∗15:02:01, C∗07:02:01, DRB1∗12:02:01, and DQB1∗03:01:01. Haplotype calculation showed that A∗29:01:01∼B∗07:05:01, DRB1∗12:02:01∼DQB1∗3:01:01, A∗29:01:01∼C∗15:05:02∼B∗07:05:01, A∗33:03:01∼B∗58:01:01∼DRB1∗03:01:01, and A∗29:01:01∼C∗15:05:02∼B∗07:05:01∼DRB1∗10:01:01∼DQB1∗05:01:01 were the most common haplotypes in the southern Kinh Vietnamese population. Allele distribution and haplotype analyses demonstrated that the Vietnamese population shares HLA features with South-East Asians but retains unique characteristics. Data from this study will be potentially applicable in medicine and anthropology.
Human leukocyte antigen (HLA) genes, which encode major histocompatibility complex proteins in humans, are located in the short arm of chromosome 6 (Alper et al., 2006). These encoded HLA proteins are displayed on the cell surface and can be classified into two distinct classes. Class I HLA proteins (A, B, and C) present intracellular antigens originating from viruses or tumors to cytotoxic T lymphocytes. Class II HLA proteins (DR, DQ, and DP) present extracellular antigens to T-helper cells. HLA genes are highly polymorphic and play an important role in immune-mediated diseases, tumor-development processes, transplanted organ or tissue survival determination, and drug hypersensitivity (Dawson et al., 2001; Dhaliwal et al., 2003; Hung et al., 2005; Avila-Rios et al., 2009; Chen et al., 2015; Thao et al., 2018).
HLA genotyping is a complex procedure due to the extreme degree of polymorphism in the major histocompatibility complex family. The most polymorphic regions, known as the core exons, are exons 2 and 3 in HLA class I genes and exon 2 in HLA class II genes. The sequences of the core exons are the most popular targets for genotyping as they are believed to be essential determinants of antigen specificity, which is informative for transplantation. However, in population genetic and evolutionary studies, many polymorphisms in other exons, introns, and UTRs have been identified and contribute to creating HLA nomenclature (Marsh and WHO Nomenclature Committee for Factors of the Hla System, 2012). Currently, HLA typing is performed using DNA-based methods, including SSP- (sequence-specific primer), SSO- (sequence-specific oligonucleotide), and RFLP-PCR (restriction fragment length polymorphism polymerase chain reaction) and sequence-based typing (SBT) (Tait et al., 2009; Bontadini, 2012; Erlich, 2012). SBT was considered the gold-standard method for high-resolution HLA genotyping, although this technique may produce uncertain results due to insufficient sequencing and ambiguous haplotype phasing (Erlich, 2012). Recent advancements in next-generation sequencing (NGS) technologies have significantly impacted the HLA-typing process (Abbott et al., 2006; Bentley et al., 2009; Erlich et al., 2011; Erlich, 2012; Shiina et al., 2012; Hosomichi et al., 2013, 2015; Schöfl et al., 2017). These new approaches can overcome the usual phase ambiguity of HLA alleles and enable massive, parallel, high-resolution HLA-typing. Different NGS-based HLA-typing methods have been established, such as amplicon-based HLA sequencing (Boegel et al., 2012; Shiina et al., 2012; Hosomichi et al., 2013; Schöfl et al., 2017), target enrichment of HLA genes (Wittig et al., 2015), and whole exome or genome sequencing data-derived typing (Liu et al., 2012; Major et al., 2013).
Only a few studies have been performed to analyze HLA allele and haplotype frequency in the Vietnamese population (Vu-Trieu et al., 1997; Busson et al., 2002; Hoa et al., 2008). Moreover, these studies failed to present detailed HLA information due to low-resolution or incomplete loci description. There is an urgent need for an HLA-typing procedure that can yield accurate and detailed HLA allele distribution. Previous studies have investigated HLA allele distribution among the Kinh population in northern Vietnam, but this study aimed to perform high-resolution HLA typing (3-field) via NGS and determine the frequency of specific alleles and haplotypes of HLA-A, -B, -C, -DRB1, and -DQB1 in southern Kinh Vietnamese populations.
A descriptive, cross-sectional study was conducted involving 101 unrelated healthy individuals. All subjects, who originated from Ho Chi Minh City and the surrounding Mekong delta provinces, were self-identified as Kinh Vietnamese and were recruited at the University of Medicine and Pharmacy, Ho Chi Minh City, Vietnam from August to October 2017. The study was approved by the Ethics Committee of the University of Medicine and Pharmacy at Ho Chi Minh City, Vietnam. All subjects were counseled and provided written informed consent for the study.
Venous blood (2 ml) was collected from each subject using an EDTA anticoagulant tube. Genomic DNA was extracted from peripheral blood leukocytes using the QIAamp DNA Mini Kit (Qiagen, Hilden, Germany) according to the manufacturer’s protocol, and samples were stored at −20°C until analysis.
Genomic DNA quality was assessed by measuring absorbance at 260 nm using a NanoDrop 2000 (Thermo Scientific, MA, United States), and the optical density (OD) ratio (260/280 nm) was calculated to evaluate sample purity. The recommended purified genomic DNA concentration (≥30 μg/μL) and OD ratio (≥1.8) for library preparation were ascertained.
The HLA TruSight kit (CareDx, Brisbane, CA, United States) was used for library preparation. Library construction began with a long-range PCR for full-length HLA-A, -B, -C, -DRB1, -DQB1 loci. All amplicons were normalized to prevent sequencing bias between samples by using magnetic beads consisting of carboxy-coated paramagnetic particles (Hawkins et al., 1994). The beads bound saturating amounts of DNA, and the DNA concentration was normalized to a similar concentration across samples after the washing and elution steps (Hosomichi et al., 2014). Subsequently, the DNA amplicons were fragmented into approximately 2-kb pieces, indexed, and pooled for sequencing on the MiniSeq platform (Illumina, San Diego, CA, United States). The pooled library was quantitated before loading on MiniSeq as the library concentration determines cluster density, which is an important parameter for data quality. As instructed in the Illumina protocol, a Qubit 3.0 fluorometer (Thermo Scientific, Waltham, MA, United States) was used for library quantitation. The pooled library was loaded unto the MiniSeq system when its concentration was ≥10 ng/μL.
Next-generation sequencing was performed via the MiniSeq system. Each sample was examined for average depth of coverage and Q30 quality scores, which were >200 and 85, respectively, for all five loci. The sequences were subsequently analyzed using an Assign TruSight HLA v2.0 (CareDx, Brisbane, CA, United States).
Qualified FASTQ files from the MiniSeq system were analyzed by Assign TruSight HLA v2.0 (CareDx, Brisbane, CA, United States). Results with 0 core exon mismatch and phasing ≤2 were accepted. Although full-length HLA loci were sequenced, the maximum resolution that the software Assign TruSight HLA v2.0 can provide is 3-field. Higher resolution (4-field) can be achieved if other analysis tools are applied to assign HLA alleles.
For single-locus analysis, allele frequencies were calculated by direct counting, deviation from Hardy–Weinberg (HW) proportions was calculated via chi-square test, and the Ewens–Watterson (EW) homozygosity test of neutrality was also performed via Monte-Carlo implementation of the exact test (Ewens, 1972; Watterson, 1978; Slatkin, 1996). The calculation was executed in PyPop: Python for Population Genomics (Lancaster et al., 2007). For multiple-locus analysis, haplotype frequencies were estimated using an expectation-maximization algorithm by Arlequin ver. 3.5 with default settings (Excoffier and Lischer, 2010); linkage disequilibrium (LD) between all HLA allele pairs was analyzed in PyPop, in which D′ and Wn of specific allele pairs were calculated (Lancaster et al., 2007). LD between all HLA loci pairs was further calculated and plotted using conditional asymmetric linkage disequilibrium (ALD) measures (Thomson and Single, 2014). The principal component analysis (PCA) of HLA-A, -B, and -DRB1 was performed using Excel 2010 to compare allele distribution between our data (n = 101) and HLA allele frequency data of the Vietnamese Hanoi Kinh population 2 (n = 170), Chinese Canton Han population (n = 264), Indonesian Sundanese and Javanese population (n = 201), Thai population (n = 142), Japanese population 3 (n = 1018), South Korean population 3 (n = 485), and Malaysian Peninsular Malay population (n = 951), which were retrieved from the Allele Frequencies Net Database (allelefrequencies.net) (González-Galarza et al., 2015). Due to the unavailability of 3-field HLA data in previous studies, we converted 3-field to 2-field data. For example, HLA-A∗24:02:01, A∗24:02:13, and A∗24:02:40 were converted to HLA-A∗24:02 with a frequency (0.13861) that was the sum of the three 3-field alleles (0.12871, 0.00495, and 0.00495, respectively). PCA results were plotted using BioVinci software (BioTuring Inc., San Diego, CA, United States).
Advancements in NGS offer the ability to distinguish between a set of alleles that share two field names and differ in the third field, such as A∗24:02, C∗07:01, and DQB1∗05:02, in one sequencing batch. As the polymorphisms of A∗24:02:40, A∗24:02:13, C∗07:01:02, and DQB1∗05:02:02 are not in the core exons, several traditional PCR and sequencing reactions were required to determine these alleles before NGS methods became available.
The number of HLA-A, HLA-B, HLA-C, HLA-DRB1, and HLA-DQB1 alleles detected in this study were 28, 41, 21, 26, and 25, respectively. The frequencies of HLA class I and class II alleles are summarized in Table 1. HLA-A∗11:01:01, A∗24:02:01, and A∗33:03:01 (22.77, 12.87, and 10.89%) were the three most frequent HLA-A alleles, followed by A∗02:07:01, A∗29:01:01, and A∗02:03:01 (9.90, 8.42, and 7.43%, respectively). HLA-B∗15:02:01, B∗46:01:01, B∗58:01:01, B∗40:01:02, B∗38:02:01, and B∗07:05:01 (11.88, 9.41, 8.42, 7.92, 7.92, and 6.93%, respectively) were the most frequent HLA-B alleles. The most frequent alleles in locus C were HLA-C∗07:02:01, C∗01:02:01, and C∗08:01:01 (21.78, 13.37, and 12.87%). HLA-DRB1∗12:02:01 accounted for 22.28% of the HLA-DRB1 alleles. HLA-DRB1∗09:01:02 was the second most frequent allele (13.37%), followed by DRB1∗15:02:01, DRB1∗10:01:01, DRB1∗03:01:01, and DRB1∗04:05:01 (9.90, 7.92, 7.42, 6.44%, respectively). On the HLA-DQB1 locus, DQB1∗03:01:01 was the most frequent allele (28.71%), followed by DQB1∗03:03:02, DQB1∗05:01:01, and DQB1∗05:02:01 (12.87, 10.89, and 9.90%, respectively).
No tested loci showed any significant departure from the Hardy–Weinberg equilibrium; p-values for all homozygotes and all heterozygotes tests were 0.79 & 0.93, 0.73 & 0.93, 0.33 & 0.73, 0.68 & 0.89, and 0.40 & 0.74 for HLA- A, -B, -C, -DRB1, and -DQB1 loci, respectively. The results of the EW homozygosity test of neutrality are summarized in Table 2. p-values of F were 0.64, 0.37, 0.22, 0.44, and 0.76 for HLA- A, -B, -C, -DRB1, and -DQB1 loci, respectively.
Tables 3, 4, and 5 list the 20 most common two-locus, three-locus, and five-locus haplotypes. The most frequent haplotypes in the two-locus sets were A∗29:01:01∼B∗07:05:01 (6.93%), A∗33:03:01∼B∗58:01:01 (6.43%), A∗11:01:01∼B∗15:02:01 (5.87%), and DRB1∗12:02:01 ∼DQB1∗03:01:01 (21.28%), DRB1∗09:01:02∼DQB1∗03:03:02 (11.88%), DRB1∗10:01:01∼DQB1∗05:01:01 (7.42%). The two most frequent haplotypes in each three-locus set were A∗29:01:01 ∼C∗15:05:02∼B∗07:05:01 (6.93%) and A∗33:03:01∼B∗58:01:01 ∼DRB1∗03:01:01 (4.95%). The three most frequent five-locus haplotypes were A∗29:01:01∼C∗15:05:02∼B∗07:05:01∼DRB1∗ 10:01:01∼DQB1∗05:01:01 (4.46%), A∗33:03:01∼C∗03:02:02 ∼B∗58:01:01∼DRB1∗03:01:01∼DQB1∗02:01:01 (4.46%), and A∗11:01:01∼C∗08:01:01∼B∗15:02:01∼DRB1∗12:02:01∼DQB1∗ 03:01:01 (3.84%). The likelihood ratio test of linkage disequilibrium demonstrated that all two-, three- and five-locus associations were statistically significant (p < 0.001). Data on the full two-locus, three-locus, five-locus, and ten-locus haplotype frequencies are described in Supplementary Tables 1, 2, 3, and 4.
Pairwise LD estimates are given in Table 6 with D′ and Wn. The LD of allele pairs was always statistically significant with 1,000 permutations. LD plots based on ALD measures for HLA loci are shown in Figure 1. Generally, the associations between HLA loci within HLA classes were stronger than between HLA loci in different classes, except for the case of B & DRB1 loci. Both symmetric and asymmetric LD showed that the strongest genetic linkages were between C & B loci and DRB1 & DQB1 loci.
The PCA plot of eight Asian populations is shown in Figure 2. The percentage of variability represented by the first three principal components was 82.08%. The first, second, and third principal components demonstrated 47.29, 20.72, and 14.07% of the variances in allele frequencies between populations, respectively. The first principal component distinguished between the South-East Asian, Han Chinese, and East Asian (Japanese and South Korean) populations. The second principal component separated the Han Chinese, Kinh Vietnamese, and Thai from the Indonesian and Malaysian populations. The third principal component distinguished the Kinh Vietnamese from the Han Chinese and other South-East Asian populations. A homogeneous allele frequency distribution of HLA-A, -B, and -DRB1 was observed between the northern and southern Kinh Vietnamese (Hoa et al., 2008). Japanese and South Korean also presented a similar distribution of HLA alleles.
Figure 2. Principal component analysis (PCA) plot of eight populations based on HLA-A, -B, and -DRB1 allele frequencies. PC1, principal component 1; PC2, principal component 2; PC3, principal component 3.
In recent years, various HLA-typing methods using different NGS approaches have been performed. NGS-based HLA typing can provide high-resolution, unambiguous, phase-defined HLA alleles, avoiding several limitations compared to traditional sequence-based typing methods (Carapito et al., 2016). Our study showed the distribution of HLA-A, -B, -C, -DRB1, and -DQB1 alleles and haplotypes among the southern Kinh Vietnamese population using high-resolution NGS typing (reported at 3-field resolution, which remains ambiguous in many cases). Highly polymorphic sequences at both HLA class I and class II loci resulted in 28 alleles for HLA-A, 41 alleles for HLA-B, 21 alleles for HLA-C, 26 alleles for HLA-DRB1, and 25 alleles for HLA-DQB1.
The most frequent HLA-A alleles found in this study were A∗11:01:01 and A∗24:02:01. The high frequency of HLA-A∗11:01 and A∗24:02:01 is consistent with previous typing results of northern Kinh Vietnamese and other Asian populations, such as the Chinese, Thai, Indonesian, Korean, and Japanese (Lee et al., 2005; Hoa et al., 2008; Yuliwulandari et al., 2009; Shen et al., 2014; Ikeda et al., 2015; Nakkam et al., 2018). Among HLA-C alleles identified in this study, C∗07:02:01 was found to be widely distributed globally, while C∗01:02:01 was common in Asians (Lee et al., 2005; Shen et al., 2014; Ikeda et al., 2015; Nakkam et al., 2018). The predominance of HLA-B∗15 alleles is a major distinguishing characteristic of the Kinh population from the Thai and Chinese groups (Shen et al., 2014; Nakkam et al., 2018). However, this predominance is similar in the Indonesian population (Yuliwulandari et al., 2009). Detailed comparison of B∗15 alleles among the Vietnamese and Indonesians showed similar popularity of B∗15:02, while the second most-frequent B∗15 alleles were B∗15:25:01 and B∗15:13, respectively. HLA-B∗07:05:01, the only B∗07 allele found in Kinh Vietnamese, was the sixth most-frequent HLA-B allele, whereas it is a minor allele in other Asian groups (Whang et al., 2001).
At the HLA-DRB1 locus, the most frequent allele was HLA-DRB1∗12:02:01 (22.28%), which is common among South-East Asian populations (Busson et al., 2002; Hoa et al., 2008; Yuliwulandari et al., 2009; Nakkam et al., 2018) but infrequent among Northern East Asian groups, including Japanese and Koreans (Lee et al., 2005; Ikeda et al., 2015). Another similarity observed between the Kinh Vietnamese, Muong Vietnamese, and other South-East Asians is the predominance of HLA-DRB1∗15:02:01 over HLA-DRB1∗15:01:01, in contrast to what was observed among Northern East Asian populations. The first and second-most predominance of HLA-DQB1∗03:01:01 (28.71%) and DQB1∗03:03:02 (12.38%) in Kinh Vietnamese is similar among East Asian populations, including Taiwanese, Chinese, Korean, and Japanese (Saito et al., 2000; Lee et al., 2005; Yang and Chen, 2017), while the third-most predominance of HLA-DQB1∗05:02:01 (9.90%) is closer to the characteristics of the Thai population (Romphruk et al., 1999). In Kinh Vietnamese, the predominance of DQB1∗05:01 over DQB1∗05:02 in our data was consistent with data from a previous study (Hoa et al., 2008). However, Muong Vietnamese showed a contrary distribution (48%) of DQB1∗05:02 (Busson et al., 2002).
Based on the haplotype calculation, most two-, three-, and five-locus HLA haplotypes with predominant frequencies were consistent with a previous report on northern Kinh Vietnamese (Hoa et al., 2008). Despite being the sixth most common HLA-B allele, B∗07:05:01 was strongly associated with A∗29:01:01 and lead to the common signature haplotypes of the Kinh population, including A∗29:01:01∼B∗07:05:01, A∗29:01:01∼C∗15:05:02∼B∗07:05:01, and A∗29:01:01∼B∗07:05:01∼DRB1∗10:01:01. Interestingly, A∗29:01:01∼C∗15:05:02∼B∗07:05:01∼DRB1∗10:01:01∼DQB1∗ 05:01:01 was the most common five-locus haplotype (4.45%). The predominance of these haplotypes might be a unique feature of the Kinh Vietnamese. The strong association of DRB1∗12:02:01 and DQB1∗03:01:01 in HLA class II found in our study is also well-described in Thai, Indonesian, and surrounding populations (Gao et al., 1992; Romphruk et al., 1999; Mack et al., 2000).
The strong associations between all pairs of HLA loci in southern Kinh Vietnamese indicate a low probability of recombination between alleles from these loci; therefore, individuals who carry allele haplotypes in LD are more likely to find a donor with matching haplotypes. The strong LD between class I HLA loci has also been well-described in Asian populations (Shen et al., 2014; Ikeda et al., 2015), while the nearly complete LD of DRB1 and DQB1 loci has been observed in Han Chinese (Trachtenberg et al., 2007). PCA showed a homogeneous HLA-A, -B, and -DRB1 allele distribution of northern and southern Kinh Vietnamese. The allele distribution also demonstrated a closer relationship between Kinh Vietnamese and other South-East Asian groups than with the Han Chinese group. The Japanese were closely grouped with South Koreans, reflecting the similarity in HLA distribution among East Asian populations.
Previously, HLA typing of Asian populations were mainly based on SSO-PCR (Lee et al., 2005; Yuliwulandari et al., 2009; Shen et al., 2014; Ikeda et al., 2015; Nakkam et al., 2018). Due to the finite amounts of probes designed to recognize the polymorphisms in the core exons, this technique only allows certain allele typing with 2-field resolution. Alleles were then assigned by software based on SSO-PCR patterns. Hence, the number of alleles determined by SSO-PCR is limited. With full-length HLA sequences provided by NGS, HLA-typing software programs align sequence reads to the entire IMGT/HLA Database to find the best-matching alleles. NGS-based typing, therefore, can provide diversified HLA assignments. In our study, the number of identified alleles (141 alleles) in 101 subjects was higher compared to the previous study in northern Kinh Vietnamese (115 identified alleles in 170 subjects) (Hoa et al., 2008). Similar results were obtained in the Thai population, in which the number of HLA alleles determined by NGS and SSO-PCR were 156 and 144, respectively (Geretz et al., 2018; Nakkam et al., 2018).
Recently, it has been shown that both high-resolution HLA typing and haplotyping are important in hematopoietic stem cell transplantation for both unrelated and related donors in reducing post-transplantation adverse outcomes (Agarwal et al., 2017; Buhler et al., 2019); a single high-resolution HLA mismatch may lead to a similar negative effect on outcomes as a low-resolution one (Fuji et al., 2015; Armstrong et al., 2017). Therefore, it has been suggested that high-resolution HLA typing can reduce the likelihood of missing a clinically significant mismatch compared to traditional low-resolution typing, especially in developing countries where high-resolution HLA typing methods are not widely available (Agarwal et al., 2017). With a 3-field resolution, our typing process can distinguish between HLA-A∗24:02:01, HLA-A∗24:02:13, and HLA-A∗24:02:40 and between HLA-C∗07:01:01 and HLA-C∗07:01:02, which are considered high-resolution mismatches. Although traditional SBT can separate these alleles, it is time and resource-consuming.
Our study had several limitations that should be considered in interpreting the results. First of all, the absence of other class II HLA descriptions (HLA-DQA1, -DPA1, and -DPB1) makes the study less informative, especially for population genetic purposes. Second, the study sample size was relatively small. This may increase the risk of missing rare HLA alleles in Kinh Vietnamese and reduce the significance of statistical analysis. These limitations will necessitate further studies with comprehensive allele descriptions and larger sample sizes.
It is now also well-recognized that HLA molecules are strongly associated with the pathophysiology of adverse drug reactions, including severe cutaneous adverse reaction (SCAR), agranulocytosis, and liver injury. High prevalence of HLA-B∗15:02, B∗58:01, B∗38:02, DRB1∗08:03, and C∗03:02 suggests that the Kinh Vietnamese population is at a high risk of developing carbamazepine-induced SCAR, allopurinol-induced SCAR, methimazole-induced agranulocytosis, and methimazole-induced liver injury, respectively (Hung et al., 2005; Chen et al., 2015; Thao et al., 2018; Li et al., 2019), while the risk of developing dapsone or abacavir-induced hypersensitivity is low due to the low prevalence of HLA-B∗13:01 and B∗57:01 (Mallal et al., 2008; Sousa-Pinto et al., 2015; Tempark et al., 2017). Therefore, HLA information is important to clinicians for treatment modality adoption and to healthcare policymakers for constructing personalized medicine strategies.
To our knowledge, this is the first report of high-resolution HLA-A, -B, -C, -DRB1, and -DQB1 allele and haplotype frequencies in southern Kinh Vietnamese individuals. These data display the homogenous distribution of HLA between the northern and southern Kinh population in Vietnam. Although the characteristics of HLA class I and II alleles and haplotypes in the Kinh Vietnamese are similar to those in the Thai, Malaysian, and Indonesian populations, they still retain unique characteristics. Data from this study will be useful in anthropology, immune-mediated diseases, transplantation therapy, and drug hypersensitivity.
Raw data supporting the conclusions of this article are available on NCBI SRA with accession PRJNA609593. The data on HLA allele frequencies and haplotypes presented in this study are available on allelefrequencies.net with accession Vietnam Kinh (n = 101).
The studies involving human participants were reviewed and approved by The Ethics committee of University of Medicine and Pharmacy at Ho Chi Minh City, Vietnam. The patients/participants provided their written informed consent to participate in this study.
TM and MD designed the study, wrote the manuscript. MD, LL, and VN performed the experiments. TD, HV, NN, MD, and TM analyzed the data.
The study was supported by the Department of Science and Technology, Ho Chi Minh City, Vietnam (Grant Number 101/2017/HD-SKHCN).
The authors declare that the research was conducted in the absence of any commercial or financial relationships that could be construed as a potential conflict of interest.
The Supplementary Material for this article can be found online at: https://www.frontiersin.org/articles/10.3389/fgene.2020.00383/full#supplementary-material
Abbott, W. G. H., Tukuitonga, C. F., Ofanoa, M., Munn, S. R., and Gane, E. J. (2006). Low-cost, simultaneous, single-sequence genotyping of the HLA-A, HLA-B and HLA-C loci. Tissue Antigens 68, 28–37. doi: 10.1111/j.1399-0039.2006.00620.x
Agarwal, R. K., Kumari, A., Sedai, A., Parmar, L., Dhanya, R., and Faulkner, L. (2017). The case for high resolution extended 6-Loci HLA typing for identifying related donors in the indian subcontinent. Biol. Blood Marrow Transpl. J. Am. Soc. Blood Marrow Transpl. 23, 1592–1596. doi: 10.1016/j.bbmt.2017.05.030
Alper, C. A., Larsen, C. E., Dubey, D. P., Awdeh, Z. L., Fici, D. A., and Yunis, E. J. (2006). The haplotype structure of the human major histocompatibility complex. Hum. Immunol. 67, 73–84. doi: 10.1016/j.humimm.2005.11.006
Armstrong, A., Smyth, E., Helenowski, I., Tse, W., Duerst, R., Schneiderman, J., et al. (2017). The impact of high-resolution HLA-A, HLA-B, HLA-C, and HLA-DRB1 on transplant-related outcomes in single-unit umbilical cord blood transplantation in pediatric patients. J. Pediatr. Hematol. Oncol. 39, 26–32. doi: 10.1097/mph.0000000000000690
Avila-Rios, S., Ormsby, C. E., Carlson, J. M., Valenzuela-Ponce, H., Blanco-Heredia, J., Garrido-Rodriguez, D., et al. (2009). Unique features of HLA-mediated HIV evolution in a Mexican cohort: a comparative study. Retrovirology 6:72. doi: 10.1186/1742-4690-6-72
Bentley, G., Higuchi, R., Hoglund, B., Goodridge, D., Sayer, D., Trachtenberg, E. A., et al. (2009). High-resolution, high-throughput HLA genotyping by next-generation sequencing. Tissue Antigens 74, 393–403. doi: 10.1111/j.1399-0039.2009.01345.x
Boegel, S., Löwer, M., Schäfer, M., Bukur, T., de Graaf, J., Boisguérin, V., et al. (2012). HLA typing from RNA-Seq sequence reads. Genome Med. 4:102. doi: 10.1186/gm403
Bontadini, A. (2012). HLA techniques: typing and antibody detection in the laboratory of immunogenetics. Methods San Diego Calif. 56, 471–476. doi: 10.1016/j.ymeth.2012.03.025
Buhler, S., Baldomero, H., Ferrari-Lacraz, S., Nunes, J. M., Sanchez-Mazas, A., Massouridi-Levrat, S., et al. (2019). High-resolution HLA phased haplotype frequencies to predict the success of unrelated donor searches and clinical outcome following hematopoietic stem cell transplantation. Bone Marrow Transplant. 54, 1701–1709. doi: 10.1038/s41409-019-0520-6
Busson, M., Vu Trieu, A., Labelle, P., Pham-Van, K., Ho-Quang, H., Bouteiller, A. M., et al. (2002). HLA-DRB1 and DQB1 allele distribution in the Muong population exposed to malaria in Vietnam. Tissue Antigens 59, 470–474. doi: 10.1034/j.1399-0039.2002.590603.x
Carapito, R., Radosavljevic, M., and Bahram, S. (2016). Next-generation sequencing of the HLA locus: methods and impacts on HLA typing, population genetics and disease association studies. Hum. Immunol. 77, 1016–1023. doi: 10.1016/j.humimm.2016.04.002
Chen, P.-L., Shih, S.-R., Wang, P.-W., Lin, Y.-C., Chu, C.-C., Lin, J.-H., et al. (2015). Genetic determinants of antithyroid drug-induced agranulocytosis by human leukocyte antigen genotyping and genome-wide association study. Nat. Commun. 6:7633. doi: 10.1038/ncomms8633
Dawson, D. V., Ozgur, M., Sari, K., Ghanayem, M., and Kostyu, D. D. (2001). Ramifications of HLA class I polymorphism and population genetics for vaccine development. Genet. Epidemiol. 20, 87–106. doi: 10.1002/1098-2272(200101)20:1<87::aid-gepi8>3.0.co;2-r
Dhaliwal, J. S., Too, C. L., Lisut, M., Lee, Y. Y., and Murad, S. (2003). HLA-B27 polymorphism in the Malays. Tissue Antigens 62, 330–332. doi: 10.1034/j.1399-0039.2003.00107.x
Erlich, H. (2012). HLA DNA typing: past, present, and future. Tissue Antigens 80, 1–11. doi: 10.1111/j.1399-0039.2012.01881.x
Erlich, R. L., Jia, X., Anderson, S., Banks, E., Gao, X., Carrington, M., et al. (2011). Next-generation sequencing for HLA typing of class I loci. BMC Genomics 12:42. doi: 10.1186/1471-2164-12-42
Ewens, W. J. (1972). The sampling theory of selectively neutral alleles. Theor. Popul. Biol. 3, 87–112. doi: 10.1016/0040-5809(72)90035-4
Excoffier, L., and Lischer, H. E. L. (2010). Arlequin suite ver 3.5: a new series of programs to perform population genetics analyses under Linux and Windows. Mol. Ecol. Resour. 10, 564–567. doi: 10.1111/j.1755-0998.2010.02847.x
Fuji, S., Kanda, J., Kato, S., Ikegame, K., Morishima, S., Miyamoto, T., et al. (2015). A single high-resolution HLA mismatch has a similar adverse impact on the outcome of related hematopoietic stem cell transplantation as a single low-resolution HLA mismatch. Am. J. Hematol. 90, 618–623. doi: 10.1002/ajh.24028
Gao, X., Zimmet, P., and Serjeantson, S. W. (1992). HLA-DR,DQ sequence polymorphisms in polynesians, micronesians, and Javanese. Hum. Immunol. 34, 153–161. doi: 10.1016/0198-8859(92)90107-x
Geretz, A., Ehrenberg, P. K., Bouckenooghe, A., Fernández Viña, M. A., Michael, N. L., Chansinghakule, D., et al. (2018). Full-length next-generation sequencing of HLA class I and II genes in a cohort from Thailand. Hum. Immunol. 79, 773–780. doi: 10.1016/j.humimm.2018.09.005
González-Galarza, F. F., Takeshita, L. Y. C., Santos, E. J. M., Kempson, F., Maia, M. H. T., da Silva, A. L. S., et al. (2015). Allele frequency net update: new features for HLA epitopes, KIR and disease and HLA adverse drug reaction associations. Nucleic Acids Res. 43, D784–D788.
Hawkins, T. L., O’Connor-Morin, T., Roy, A., and Santillan, C. (1994). DNA purification and isolation using a solid-phase. Nucleic Acids Res. 22, 4543–4544. doi: 10.1093/nar/22.21.4543
Hoa, B. K., Hang, N. T. L., Kashiwase, K., Ohashi, J., Lien, L. T., Horie, T., et al. (2008). HLA-A, -B, -C, -DRB1 and -DQB1 alleles and haplotypes in the Kinh population in Vietnam. Tissue Antigens 71, 127–134. doi: 10.1111/j.1399-0039.2007.00982.x
Hosomichi, K., Jinam, T. A., Mitsunaga, S., Nakaoka, H., and Inoue, I. (2013). Phase-defined complete sequencing of the HLA genes by next-generation sequencing. BMC Genomics 14:355. doi: 10.1186/1471-2164-14-355
Hosomichi, K., Mitsunaga, S., Nagasaki, H., and Inoue, I. (2014). A bead-based normalization for uniform sequencing depth (BeNUS) protocol for multi-samples sequencing exemplified by HLA-B. BMC Genomics 15:645. doi: 10.1186/1471-2164-15-645
Hosomichi, K., Shiina, T., Tajima, A., and Inoue, I. (2015). The impact of next-generation sequencing technologies on HLA research. J. Hum. Genet. 60, 665–673. doi: 10.1038/jhg.2015.102
Hung, S.-I., Chung, W.-H., Liou, L.-B., Chu, C.-C., Lin, M., Huang, H.-P., et al. (2005). HLA-B∗5801 allele as a genetic marker for severe cutaneous adverse reactions caused by allopurinol. Proc. Natl. Acad. Sci. U.S.A. 102, 4134–4139. doi: 10.1073/pnas.0409500102
Ikeda, N., Kojima, H., Nishikawa, M., Hayashi, K., Futagami, T., Tsujino, T., et al. (2015). Determination of HLA-A, -C, -B, -DRB1 allele and haplotype frequency in Japanese population based on family study. Tissue Antigens 85, 252–259. doi: 10.1111/tan.12536
Lancaster, A. K., Single, R. M., Solberg, O. D., Nelson, M. P., and Thomson, G. (2007). PyPop update–a software pipeline for large-scale multilocus population genomics. Tissue Antigens 69(Suppl. 1), 192–197. doi: 10.1111/j.1399-0039.2006.00769.x
Lee, K. W., Oh, D. H., Lee, C., and Yang, S. Y. (2005). Allelic and haplotypic diversity of HLA-A, -B, -C, -DRB1, and -DQB1 genes in the Korean population. Tissue Antigens 65, 437–447. doi: 10.1111/j.1399-0039.2005.00386.x
Li, X., Jin, S., Fan, Y., Fan, X., Tang, Z., Cai, W., et al. (2019). Association of HLA-C∗03:02 with methimazole-induced liver injury in Graves’ disease patients. Biomed. Pharmacother. 117:109095. doi: 10.1016/j.biopha.2019.109095
Liu, C., Xiao, Y., Duffy, B., Zody, M., Tycksen, E., Shrivastava, S., et al. (2012). High resolution HLA typing by next generation exome sequencing. Blood 120:4166.
Mack, S. J., Bugawan, T. L., Moonsamy, P. V., Erlich, J. A., Trachtenberg, E. A., Paik, Y. K., et al. (2000). Evolution of Pacific/Asian populations inferred from HLA class II allele frequency distributions. Tissue Antigens 55, 383–400. doi: 10.1034/j.1399-0039.2000.550501.x
Major, E., Rigó, K., Hague, T., Bérces, A., and Juhos, S. (2013). HLA typing from 1000 genomes whole genome and whole exome illumina data. PLoS One 8:e78410. doi: 10.1371/journal.pone.0078410
Mallal, S., Phillips, E., Carosi, G., Molina, J.-M., Workman, C., Tomazic, J., et al. (2008). HLA-B∗5701 screening for hypersensitivity to abacavir. N. Engl. J. Med. 358, 568–579. doi: 10.1056/NEJMoa0706135
Marsh, S. G. E. WHO Nomenclature Committee for Factors of the Hla System (2012). Nomenclature for factors of the HLA system, update January. Tissue Antigens 79, 393–397.
Nakkam, N., Konyoung, P., Kanjanawart, S., Saksit, N., Kongpan, T., Khaeso, K., et al. (2018). HLA pharmacogenetic markers of drug hypersensitivity in a thai population. Front. Genet. 9:277. doi: 10.3389/fgene.2018.00277
Romphruk, A. V., Puapairoj, C., Romphruk, A., Barasrux, S., Urwijitaroon, Y., and Leelayuwat, C. (1999). Distributions of HLA-DRB1/DQB1 alleles and haplotypes in the north-eastern Thai population: indicative of a distinct Thai population with Chinese admixtures in the central Thais. Eur. J. Immunogenet. Off. J. Br. Soc. Histocompat. Immunogenet. 26, 129–133. doi: 10.1046/j.1365-2370.1999.00133.x
Saito, S., Ota, S., Yamada, E., Inoko, H., and Ota, M. (2000). Allele frequencies and haplotypic associations defined by allelic DNA typing at HLA class I and class II loci in the Japanese population. Tissue Antigens 56, 522–529. doi: 10.1034/j.1399-0039.2000.560606.x
Schöfl, G., Lang, K., Quenzel, P., Böhme, I., Sauter, J., Hofmann, J. A., et al. (2017). 2.7 million samples genotyped for HLA by next generation sequencing: lessons learned. BMC Genomics 18:161. doi: 10.1186/s12864-017-3575-z
Shen, Y., Cao, D., Li, Y., Kulski, J. K., Shi, L., Jiang, H., et al. (2014). Distribution of HLA-A, -B, and -C Alleles and HLA/KIR combinations in han population in China. J. Immunol. Res. 2014:565296.
Shiina, T., Suzuki, S., Ozaki, Y., Taira, H., Kikkawa, E., Shigenari, A., et al. (2012). Super high resolution for single molecule-sequence-based typing of classical HLA loci at the 8-digit level using next generation sequencers. Tissue Antigens 80, 305–316. doi: 10.1111/j.1399-0039.2012.01941.x
Slatkin, M. (1996). A correction to the exact test based on the Ewens sampling distribution. Genet Res. 68, 259–260. doi: 10.1017/s0016672300034236
Sousa-Pinto, B., Pinto-Ramos, J., Correia, C., Gonçalves-Costa, G., Gomes, L., Gil-Mata, S., et al. (2015). Pharmacogenetics of abacavir hypersensitivity: a systematic review and meta-analysis of the association with HLA-B∗57:01. J. Allergy Clin. Immunol. 136, 1092.e3–1094.e3.
Tait, B. D., Hudson, F., Cantwell, L., Brewin, G., Holdsworth, R., Bennett, G., et al. (2009). Review article: luminex technology for HLA antibody detection in organ transplantation. Nephrol. Carlton Vic. 14, 247–254. doi: 10.1111/j.1440-1797.2008.01074.x
Tempark, T., Satapornpong, P., Rerknimitr, P., Nakkam, N., Saksit, N., Wattanakrai, P., et al. (2017). Dapsone-induced severe cutaneous adverse drug reactions are strongly linked with HLA-B∗13: 01 allele in the Thai population. Pharmacogenet. Genomics 27, 429–437. doi: 10.1097/FPC.0000000000000306
Thao, M. P., Tuan, P. V. A., Linh, L. G. H., Van Hoang, L., Hen, P. H., Hoa, L. T., et al. (2018). Association of HLA-B∗38:02 with antithyroid drug-induced agranulocytosis in kinh vietnamese patients. Int. J. Endocrinol. 2018:7965346.
Thomson, G., and Single, R. M. (2014). Conditional asymmetric linkage disequilibrium (ALD): extending the biallelic r2 measure. Genetics 198, 321–331. doi: 10.1534/genetics.114.165266
Trachtenberg, E., Vinson, M., Hayes, E., Hsu, Y.-M., Houtchens, K., Erlich, H., et al. (2007). HLA class I (A, B, C) and class II (DRB1, DQA1, DQB1, DPB1) alleles and haplotypes in the Han from southern China. Tissue Antigens 70, 455–463. doi: 10.1111/j.1399-0039.2007.00932.x
Vu-Trieu, A., Djoulah, S., Tran-Thi, C., Ngyuyen-Thanh, T., Le Monnier De Gouville, I., Hors, J., et al. (1997). HLA-DR and -DQB1 DNA polymorphisms in a Vietnamese Kinh population from Hanoi. Eur. J. Immunogenet. Off. J. Br. Soc. Histocompat. Immunogenet. 24, 345–356. doi: 10.1046/j.1365-2370.1997.d01-107.x
Whang, D. H., Kang, S. J., Han, K. S., and Park, M. H. (2001). HLA-B∗07 allele frequencies and haplotypic associations in Koreans. Tissue Antigens 57, 76–79. doi: 10.1034/j.1399-0039.2001.057001076.x
Wittig, M., Anmarkrud, J. A., Kässens, J. C., Koch, S., Forster, M., Ellinghaus, E., et al. (2015). Development of a high-resolution NGS-based HLA-typing and analysis pipeline. Nucleic Acids Res. 43:e70. doi: 10.1093/nar/gkv184
Yang, K.-L., and Chen, H.-B. (2017). Using high-resolution human leukocyte antigen typing of 11,423 randomized unrelated individuals to determine allelic varieties, deduce probable human leukocyte antigen haplotypes, and observe linkage disequilibria between human leukocyte antigen-B and-C and human leukocyte antigen-DRB1 and-DQB1 alleles in the Taiwanese Chinese population. Tzu Chi Med. J. 29, 84–90. doi: 10.4103/tcmj.tcmj_35_17
Keywords: high-resolution, HLA typing, allele frequency, haplotype frequency, Kinh Vietnamese, next-generation sequencing
Citation: Do MD, Le LGH, Nguyen VT, Dang TN, Nguyen NH, Vu HA and Mai TP (2020) High-Resolution HLA Typing of HLA-A, -B, -C, -DRB1, and -DQB1 in Kinh Vietnamese by Using Next-Generation Sequencing. Front. Genet. 11:383. doi: 10.3389/fgene.2020.00383
Received: 07 November 2019; Accepted: 27 March 2020;
Published: 30 April 2020.
Edited by:
Denis Baurain, University of Liège, BelgiumReviewed by:
Steven J. Mack, University of California, San Francisco, United StatesCopyright © 2020 Do, Le, Nguyen, Dang, Nguyen, Vu and Mai. This is an open-access article distributed under the terms of the Creative Commons Attribution License (CC BY). The use, distribution or reproduction in other forums is permitted, provided the original author(s) and the copyright owner(s) are credited and that the original publication in this journal is cited, in accordance with accepted academic practice. No use, distribution or reproduction is permitted which does not comply with these terms.
*Correspondence: Thao Phuong Mai, ZHJtYWl0aGFvQHVtcC5lZHUudm4=
Disclaimer: All claims expressed in this article are solely those of the authors and do not necessarily represent those of their affiliated organizations, or those of the publisher, the editors and the reviewers. Any product that may be evaluated in this article or claim that may be made by its manufacturer is not guaranteed or endorsed by the publisher.
Research integrity at Frontiers
Learn more about the work of our research integrity team to safeguard the quality of each article we publish.