- 1Headquarter, Hospital Authority, Hong Kong, Hong Kong
- 2Department of Health Technology and Informatics, The Hong Kong Polytechnic University, Hong Kong, Hong Kong
Both tendon injuries and tendinopathies, particularly rotator cuff tears, increase with tendon aging. Tendon stem cells play important roles in promoting tendon growth, maintenance, and repair. Aged tendons show a decline in regenerative potential coupled with a loss of stem cell function. Recent studies draw attention to aging primarily a disorder of stem cells. The micro-environment (“niche”) where stem cells resided in vivo provides signals that direct them to metabolize, self-renew, differentiate, or remain quiescent. These signals include receptors and secreted soluble factors for cell-cell communication, extracellular matrix, oxidative stress, and vascularity. Both intrinsic cellular deficits and aged niche, coupled with age-associated systemic changes of hormonal and metabolic signals can inhibit or alter the functions of tendon stem cells, resulting in reduced fitness of these primitive cells and hence more frequent injuries and poor outcomes of tendon repair. This review aims to summarize the biological changes of aged tendons. The biological changes of tendon stem cells in aging are reviewed after a systematic search of the PubMed. Relevant factors of stem cell aging including cell-intrinsic factors, changes of microenvironment, and age-associated systemic changes of hormonal and metabolic signals are examined, with findings related to tendon stem cells highlighted when literature is available. Future research directions on the aging mechanisms of tendon stem cells are discussed. Better understanding of the molecular mechanisms underlying the functional decline of aged tendon stem cells would provide insight for the rational design of rejuvenating therapies.
Introduction - Biological Changes of Aged Tendons
Aging is known to reduce the regenerative capacity and increase the susceptibility of tissues and organs, including tendons, to injuries. Both tendon injuries and tendinopathies, particularly rotator cuff tears, increase with tendon aging (Teunis et al., 2014; Albers et al., 2016). In a systematic review including 30 studies with 6,112 shoulders, the prevalence of rotator cuff abnormalities increased with age, from 9.7% in patients aged 20 years and younger to 62% in patients aged 80 years and above (Teunis et al., 2014). Lower extremity tendinopathy was also reported to be more prevalent among older patients (Albers et al., 2016).
Structural Changes
Aged tendons exhibited structural, compositional and biomechanical changes. Aged tendons appeared yellowish (mucoid degeneration) (Zhang et al., 2016). Collagen fibers were less organized in aged mouse patellar tendons (Dunkman et al., 2013). Ultrastructural analysis of distribution of fibril diameters indicated an increase of a subpopulation of large fibrils in aged mouse patellar tendons (Dunkman et al., 2013) and Achilles tendons (Gehwolf et al., 2016). There were inconsistent findings regarding the change of vascularity and blood flow in tendon during aging, depending on the species, tendon types, and definition of aging. While there was no age-associated change in vascularity in equine superficial digital flexor tendons (aged 2 to 23 years) (Gillis et al., 1997), aged rat patellar tendons (20-month-old) were reported to be highly vascular (Zhang et al., 2016). An early human study reported a reduction of vascularity in the supraspinatus tendons with age (aged 20–70 years) (Brewer, 1979). While there was no change in the rate of blood flow in the flexor tendons with age in a rabbit study (Landi et al., 1983), there was a significant decrease in blood flow in the intratendinous region of rotator cuff of elderly subjects compared with younger subjects (Funakoshi et al., 2010). Rudzki et al. (2008) reported a significant decrease in blood flow in intact supraspinatus tendon in asymptomatic subjects older than 40 years compared with younger subjects after exercise. Non-tendinous tissues such as fatty and cartilaginous tissues as well as calcification were reported in aged tendons in both animal models and human (Iagnocco et al., 2013; Zhang and Wang, 2015; Gehwolf et al., 2016; Wood and Brooks, 2016; Zaseck et al., 2016). These non-tendinous tissues compromise tendon structure and reduce tendon’s biomechanical properties.
Compositional Changes
Tenocytes are the major cell types in tendons. The cell number decreased; tenocyte dedifferentiated and senescent; and their proliferation and metabolic activity reduced with aging (Ippolito et al., 1980; Josa and Kannus, 1997; Tsai et al., 2011; Dunkman et al., 2013; Yu et al., 2013).
The expression of extracellular matrix (ECM) genes and ECM remodeling gene were significantly reduced in aged Achilles tendons (Kostrominova and Brooks, 2013; Yu et al., 2013; Gehwolf et al., 2016; Wood and Brooks, 2016). The mRNA expression of collagen types I, III, and V, elastin and proteoglycan 4 (lubricin) in rat tendons decreased with age though immunostaining showed no apparent differences in protein levels of collagen type I and type V (Kostrominova and Brooks, 2013). The mRNA expression of collagen type I was reported to be similar in rat Achilles tenocytes in young (2 months) and aged (24 months) groups but the mRNA expression and enzymatic activities of MMP-2 and MMP-9 was significantly higher while the mRNA expression of tissue inhibitor of metalloproteinase-1 (TIMP-1) and TIMP-2 were significantly lower in the aged group compared to the young group in vitro (Yu et al., 2013). The expressions of collagen type I and type III genes were reduced in aged mouse Achilles tendons (Gehwolf et al., 2016). The collagen content of aged mouse Achilles tendon was similar to their young counterpart (Gehwolf et al., 2016) but the level decreased in canine patellar tendon with aging (Haut et al., 1992). In a small clinical study involving 7 old men and 10 young men, the collagen concentration in the patellar tendon biopsies was lower in the old men compared to that in the young men with a similar physical activity level, supporting the reduction in collagen during the aging process (Couppe et al., 2009).
Proteoglycans are important for regulating collagen fibril assembly, fiber size, and fiber sliding as well as cellular functions. The age-associated changes of proteoglycans were inconclusive. Thorpe et al. (2016) reported no change in the mRNA expression of collagens and proteoglycans as well as protein and mRNA levels of matrix remodeling enzymes in equine superficial digital flexor tendons with age (3.3 ± 0.6 years versus 19.0 ± 1.7 years). There was also no difference in the mRNA expression of biglycan, decorin, fibromodulin, and lumican in the patellar tendons of aged mice (Dunkman et al., 2013). While there was no change in the levels of major matrix components with age, there was a reduction in protein levels of several less abundant small leucine-rich proteoglycans (fibromodulin, mimecan, asporin) in aged equine superficial digital flexor tendons (Peffers et al., 2014). One study reported significant lower total glycosaminoglycan, chondroitin sulphate, and dermatan sulphate in healthy human supraspinatus tendons with age, although there was no change in the relative proportion of different glycosaminoglycan types (Riley et al., 1994). The concentration of nonenzymatic cross-links was higher in the patellar tendon biopsies of aged men compared to that in the young men in a small scale clinical study (Couppe et al., 2009). The advanced glycation end-products (AGEs) adduct level in tibialis anterior tendons was also higher in aged compared to adult mice (Wood and Brooks, 2016).
Biomechanical Changes
The biomechanical properties of aged tendon were reported to be inferior in animal and human studies. The viscoelastic properties and mechanical strength of aged equine and mouse tendons were reported to be lower than those of young tendons (Dudhia et al., 2007; Dunkman et al., 2013; Zaseck et al., 2016). The mechanical properties (maximum stress and modulus) of aged rat Achilles tendon decreased with increasing age (Pardes et al., 2017). While aging did not alter tendon mechanical properties during homeostasis, it impaired tendon healing and hence biomechanical properties of flexor tendon in mice (Ackerman et al., 2017). Aged flexor tendons showed similar mechanical strength (maximum load to failure and ultimate tensile stress) but was significantly stiffer (higher Young’s modulus and stiffness) compared to young tendons (Gehwolf et al., 2016). Aged human Achilles tendons were also stiffer compared to young tendons as shown by sonoelastography (Turan et al., 2015). Aged human patellar tendons had significantly lower elastic modulus and shear wave velocity compared to young tendons as indicated by shear wave elastography (Hsiao et al., 2015). In a systematic review of age-related changes of biomechanical properties of healthy Achilles tendon, its stiffness, and elastic modulus decreased in older compared to younger adults (Delabastita et al., 2018). The responses of human Achilles and patellar tendons to transverse strain was reduced by 2.5% for every 10 years of life (Dudhia et al., 2007). Table 1 summarizes the biological changes of aging tendons.
Consequently, the structure, composition, and biomechanical properties of aged tendons are compromised and aged tendons are prone to higher risk of tendon injuries, re-injuries, and tendinopathy as well as treatment failure (de Jonge et al., 2011; Teunis et al., 2014; Salini et al., 2015; Albers et al., 2016). The odds of a rotator cuff tear increased by 2.69-fold for every 10 years of age (Fehringer et al., 2008). After injury, aged tendons require very long time for rehabilitation. The current treatment modalities fail to completely restore tendon function. A summary of biological changes of aging tendons is showed in Table 1.
Tendon Aging as a Problem of Aging of Tendon Stem Cells and Their Niche
Recent studies draw attention to aging being primarily a disorder of stem cells with a decrease in the number and functional fitness of tissue-specific adult stem cells (Sharpless and DePinho, 2007; Fukada et al., 2014). Tendon stem cells have been recently identified in tendons of various species (Bi et al., 2007; Rui et al., 2010). Stem/progenitor cells isolated from tendon mid-substances of various species, called tendon-derived stem cells (TDSCs), showed standard mesenchymal stem cell (MSC) characteristics, with expression of typical MSC surface antigens, self-renewal, colony-forming, and tri-lineage differentiation potential (Rui et al., 2010; Lui and Chan, 2011; Lui, 2015). In addition, they are able to form tendon- and enthesis- like tissues when implanted in vivo. TDSC expressed higher Oct4 level, showed higher clonogenicity and proliferative, and differentiation capacity compared to BMSC (Tan et al., 2012). TDSCs expressed embryonic stem cell markers and are different from tenocytes which are terminally differentiated cells (Zhang and Wang, 2010a). Recent studies showed that the functions of TDSCs declined or skewed with age in vitro. An in-depth understanding of the molecular mechanisms controlling tendon stem cell aging is essential to inform the development of stem cell-based therapeutics that can slow, or even forestall age-associated degenerative changes as well as enhance repair of aged tendons.
Niche is a specialized dynamic micro-environment which regulates stem cells’ functions and fate. Aging of cellular and acellular components of the niche can alter stem cell functions. Numerous studies showed that exposure of stem cells to a young systemic environment restored the regenerative capacity of stem cells in various tissues in aged mice (Conboy et al., 2005; Ruckh et al., 2012). Transplantation of aged spermatogonial stem cells to the testes of young male mice was sufficient to maintain stem cell functions (Sato et al., 2011). These findings underscore a major role of aging of the niche in the functional decline of stem cells and suggest opportunities of reversing tissue aging by targeting the niche.
Scope of Review and Search Strategy
This review aims to summarize the biological changes of tendon stem cells in aging after a systematic search of PubMed. Relevant factors of stem cell aging including cell-intrinsic factors, changes of microenvironment, and systemic changes of hormonal and metabolic signals are reviewed, with findings on tendon stem cells highlighted when literature is available. Future research directions on the aging mechanisms of tendon stem cells are discussed.
In this review, “TDSCs” refer to stem/progenitor cells isolated from tendon mid-substances in vitro while “tendon stem cells” refer to stem/progenitor cells residing in situ in tendon mid-substances. TDSCs are often used as in vitro model to understand the physiology of tendon stem cells residing in situ in tendon mid-substances. The properties of tendon stem cells may change due to cell isolation and in vitro culture conditions. “Tenocytes” refer to terminally differentiated cells in tendons.
For the studies on aged TDSCs, the PubMed database was searched with the keywords ((tendon[Title/Abstract]) AND (ageing[Title/Abstract] OR aging[Title/Abstract] OR age[Title/Abstract])) AND (stem cells[Title/Abstract] OR progenitor) on 25 Jan 2019 and updated on 22 Sep 2019 with no restriction in language and year of publication. The studies were selected after reviewing the titles and abstracts. Original studies investigating the biological changes of stem cells in tendons, either in vitro or in situ, in aging were included. A total of 74 articles was identified. Of these, 16 studies are eligible. Another two studies were identified by hand search. Therefore, a total of 18 studies were included in this review and all of them are on the biological changes of TDSCs of aged tendons. There were no studies on aging of tendon stem cells in situ. Table 2 summaries the studies on the biological changes of TDSCs in aging.
Characteristics of Aged TDSCs
Phenotypes of Aged TDSCs
Young TDSCs were reported as spindle or cobble-stone shape in previous studies (Rui et al., 2010; Zhang and Wang, 2015). However, TDSCs isolated from aged human, mouse and rat tendons were reported to be heterogeneous, much larger and show a round and flattened appearance (Wu et al., 2015; Zhang and Wang, 2015). The stiffness of aged rat TDSCs was higher than that of young TDSCs (Wu et al., 2015; Kiderlen et al., 2019). The increase in size and irregular shape of aged TDSCs might be associated with the dense cytoskeleton organization, which could lead to an increase in both stiffness and viscosity (Wu et al., 2015; Kiderlen et al., 2019). Indeed, the actin stress fiber content was higher and the turnover of actin filaments was slower in TDSCs isolated from aged/degenerated human Achilles tendon compared to TDSCs isolated from young/healthy tendons (Kohler et al., 2013). There was also an increase in focal adhesion kinase complex protein paxillin and profound rearrangement of actin cytoskeleton with an increase in actin in aged mouse Achilles TDSCs (Gehwolf et al., 2016).
Frequency, Proliferative, and Colony-Forming Ability of Aged TDSCs
Fewer TDSCs were isolated from aged tendons compared to young tendons (Zhou et al., 2010; Ruzzini et al., 2014). Aged TDSCs showed delayed cell cycle progression (Zhou et al., 2010), lower proliferation (Zhou et al., 2010; Klatte-Schulz et al., 2012; Kohler et al., 2013; Jiang et al., 2014; Zhang and Wang, 2015; Hu et al., 2017), lower proliferative response to fibroblast growth factor-2 (FGF-2), and transforming growth factor β 1 (TGF-β1) (Kohler et al., 2013) as well as lower colony-forming ability (Klatte-Schulz et al., 2012; Kohler et al., 2013) compared to their young counterparts.
Stem Cell Markers and ECM Expression and Migration Ability of Aged TDSCs
Aged TDSCs showed lower expression of stem cell markers (Oct-4, nucleostemin, Sca-1, and SSEA-1) (Zhang and Wang, 2015) and tenocyte-related markers (Zhou et al., 2010; Jiang et al., 2014) as well as lower collagen type I secretion (Klatte-Schulz et al., 2012; Kohler et al., 2013) and higher fibronectin protein expression (Kohler et al., 2013) compared to young TDSCs. However, Klatte-Schulz et al. (2012) reported no significant difference in the surface expression of MSC markers (CD29, CD44, CD73, CD90, and CD105) as well as mRNA expression of ECM proteins (collagen type I, collagen type III, osteocalcin, decorin), TGF-β1, TGF-β2, TGF-β3 between aged and young human tenocyte-like cells. Zhou et al. (2010) reported no difference in stem cell markers (nucleostemin, Oct-4, and SSEA-4) between young and aged rat TDSCs. The aged/degenerated TDSCs were deficient in cell adhesion, migration and actin dynamics (Kohler et al., 2013; Jiang et al., 2014). Aged mouse TDSCs showed lower collagen adhesion and contractility compared to their younger counterparts (Yin et al., 2019). This is consistent with change of transcriptome, especially in genes regulating cell adhesion, migration, and actin cytoskeleton, in aged human Achilles TDSCs (Kohler et al., 2013).
Differentiation Potential of Aged TDSCs
While two studies reported similar tri-lineage differentiation potential of young and aged human TDSCs (Kohler et al., 2013; Ruzzini et al., 2014), one study reported higher adipogenic differentiation potential of aged rat TDSCs (Zhou et al., 2010) and another study showed lower osteogenic differentiation of aged human tenocyte-like cells (Klatte-Schulz et al., 2012). Zhang and Wang (2015) reported reduced tri-lineage differentiation potential of aged TDSCs compared to their young counterparts. Han et al. (2017) showed that the tenogenic differentiation capacity, collagen type I concentration, and expression of tendon-related markers (Scx, Tnmd, Dcn, Bgn, Col1, and Col3) of human Achilles TDSCs decreased with advancing age. Treatment of human TDSCs with tarzarotene, a retinoic acid receptor (RAR) agonist, induced nuclear translation of scleraxis and inhibited spontaneous cell differentiation via histone methylation but not histone acetylation in RAR signaling, suggesting that histone methylation plays roles in the differentiation of tendon stem cells (Webb et al., 2016). Regulation of methylation status of aged TDSCs may help to restore the tenogenic differentiation potential of TDSCs and this requires further research.
Intrinsic Cellular Deficits
Cellular Senescence
Cellular senescence is a state in which a cell no longer has the ability to proliferate (Collado et al., 2007; Hoare et al., 2010). Senescent cells are irreversibly arrested at the G1 phase of the cell cycle and do not respond to various external stimuli, but they remain metabolically active (Collado et al., 2007; Hoare et al., 2010). Cellular senescence can be triggered by a number of cellular stresses, including oxidative stress, telomere dysfunction, non-telomeric DNA damage, epigenetic derepression of the INK4a/ARF locus, and oncogenic activation (Collado et al., 2007). Senescent cells are characterized by shortened telomeres, increased activity of senescence-associated β-galactosidase (SA-β-gal), increased expression of p16INK4a, p53, p15INK4B, and p21WAF1/Cip1, and histological changes (Collado et al., 2007; Hoare et al., 2010). Cellular senescence is a potent tumor suppression mechanism. It is seen in aged or damaged tissues, and is associated with reduced regenerative capacity with age. Similar to other tissue-specific stem cells, aged TDSCs displayed cellular senescence (Kohler et al., 2013; Jiang et al., 2014; Han et al., 2017; Hu et al., 2017). The expression of p21WAF1/Cip1 (Hu et al., 2017) and p16INK4a (Han et al., 2017) was higher in human aged/degenerated Achilles TDSCs. In another study, upregulation of p16INK4a gene and protein expression was observed, but the gene expression of p14, p21, and p53 were unchanged in age/degenerated human Achilles TDSCs (Kohler et al., 2013). Further studies are required to confirm this finding. p16INK4a is a critical effector of cellular senescence. Many studies showed that the increase in the expression of p16INK4a with aging was linked to the impairment of the number and/or function of adult stem cells (Janzen et al., 2006; Molofsky et al., 2006; Sousa-Victor et al., 2014) while abolishing p16INK4a function enhanced the regenerative potential of stem cells (Shibata et al., 2007; Sousa-Victor et al., 2014). Indeed, overexpression of p16INK4a inhibited tenogenic differentiation of young TDSCs (Han et al., 2017). Analysis of the mechanism revealed that the effect was mediated by miR-217 and its target EGR1 (Han et al., 2017). Silencing p16 in aged satellite cells restored their quiescence and regenerative potential (Sousa-Victor et al., 2014). miRNA are epigenetic regulators of multiple biological activities including stem cell differentiation. Microarray analysis suggested that dysregulation of the Rho-associated protein kinase (ROCK) pathway might be a key player in TDSC aging (Kohler et al., 2013). The ROCK1 and ROCK 2 mRNA and protein levels as well as activities increased in aged/degenerated TDSCs (Kohler et al., 2013). Inhibition of ROCK1 and ROCK2 activities in aged/degenerated human TDSCs with ROCK inhibitor restored the cell area, F-actin content, and dynamics as well as migration (Kohler et al., 2013). This is consistent with the findings of another study which treatment with ROCK inhibitor restored the morphology and stiffness of aged human TDSCs (Kiderlen et al., 2019). miR-135a which targets ROCK1, was significantly downregulated in aged compared to young TDSCs (Chen et al., 2015b). Inhibition of miR-135a was reported to increase senescence of TDSCs (Chen et al., 2015b). Overexpression of miR-135a in young TDSCs suppressed senescence, promoted proliferation, and induced migration and tenogenic differentiation in vitro (Chen et al., 2015b), suggesting that miR-135a is a key regulator of ROCK1-induced cellular senescence. The reduced expression of Tnmd in aged TDSCs (Zhou et al., 2010; Han et al., 2017) may affect cellular senescence of TDSCs as knockdown of Tnmd in mouse tail TDSC augmented cellular senescence, reduced the gene expression of cyclin D1, and increased the gene expression of p53 but has no effects on the gene expression of p16 and p21 (Alberton et al., 2015). However, it is important to note that the complete knockout of Tnmd was artificially created in the animal model which might be different from the reduced expression of Tnmd in aged TDSCs. miR124 suppressed collagen formation during tenogenic differentiation of human TDSCs via suppressing EGR1 expression while anti-miR124 promoted it, suggesting that miR124 might be a promising therapeutic target for TDSC and tendon aging (Wang et al., 2016).
Pin1 (peptidyl-prolyl cis-trans isomerase), an enzyme which regulates conformational changes of phosphoproteins, is an important enzyme necessary for healthy aging and prevention of age-related diseases. Deficiency of Pin1 in mice caused an early-aging phenotype (Driver and Lu, 2010). Overexpression of Pin1 was reported to delay the progression of cellular senescence, increase telomerase activity and decrease expression of p16INK4A while Pin1 knockdown promoted senescence of TDSCs in vitro, confirming that Pin1 is also an important regulator of tendon stem cell aging (Chen et al., 2015a). The Pin1-mediated anti-aging signaling mechanism might offer an attractive therapeutic target for protection against tendon aging.
Forkhead box P1 (FOXP1) plays a crucial role in tendon stem cell aging. FOXP1 directly repressed transcription of p16INK4A in BMSCs (Li et al., 2017). It controlled MSC fate and senescence during skeletal aging (Li et al., 2017). A recent study showed that the expression of FOXP1 mRNA and protein levels decreased markedly in aged TDSCs. Overexpression of FOXP1 attenuated TDSC aging with a decrease in SA-β-gal staining and p16INK4A expression (Xu and Liu, 2018). It also rescued decreased levels of E2F1, pRb, and cyclin D1 and restored self-renewal, migration and differentiation in aged TDSCs (Xu and Liu, 2018). Conversely, depletion of FOXP1 by siRNA promoted senescence of young TDSCs.
CITED2 is a transcription factor implicated in the control of cell proliferation and cellular senescence. Aged human Achilles TDSCs were shown to display lower mRNA and protein expression of CITED2 as well as lower proliferation and higher cellular senescence compared to young TDSCs (Zhou et al., 2010; Hu et al., 2017). Moreover, TGF-β2 was reported to downregulate the expression of CITED2 gene and knockdown of CITED2 abolished the effect of TGF-β2–mediated TDSC senescence (Hu et al., 2017). The effects of TGF-β2 inhibitors and CITED2 activators in reversing the senescence of aged TDSCs and tendon aging await further studies.
Accumulation of Damaged DNA and Proteins
Aging is one of the major risk factors for DNA damage, protein mis-folding and accumulation. Age-dependent deficits of the autophagy-lysosomal and ubiquitin-proteasome systems, which remove the damaged proteins, were reported in recent studies (Rubinsztein et al., 2011; Tomaru et al., 2012). However, other studies argued that it was the increase in damage caused by metabolic stress, not the decrease in autophagy potential and proteasome activity, which overwhelmed the protective capacity of proteolytic systems in aged cells (Warr et al., 2013). Inhibition of autophagy has been shown to inhibit self-renewal and differentiation of stem cells, including TDSCs (Cheng et al., 2015; Chen et al., 2016; Sbrana et al., 2016). Tendon aging is dependent at least in part on the actions of mechanistic target of rapamycin (mTOR). Long-term dietary administration of rapamycin, an inhibitor of mTOR, was reported to attenuate aged-associated increase in stiffness; decrease in elastiscity, cellularity, and progression of spontaneous tendon calcification; as well as enhance collagen turnover in aged mouse tendons (Wilkinson et al., 2012; Zaseck et al., 2016). Autophagy plays an important role in regulating the self-renewal capacity and stemness of human TDSCs through suppressing reactive oxygen species (ROS) production. Treatment of human TDSCs isolated from supraspinatus tendon of adult donors (26–34 years old) with starvation or rapamycin prevented H2O2-induced loss of self-renewal capacity and stemness (Chen et al., 2016). The effects were mediated by increasing autophagy activity and suppressing ROS production in TDSCs (Chen et al., 2016). On the other hand, inhibition of autophagy reduced the protective effects of starvation and rapamycin on H2O2-treated cells (Chen et al., 2016).
Aged Niche
Cell-Cell Communication
Stem cells interact with each other or niche cells via direct interaction of membrane proteins or secretion of soluble factors. The changes of membrane proteins or secretome of stem cells or niche cells during aging therefore could impact stem cell fate and functions. Ephrin are receptor tyrosine kinases for mediating short-distance cell-cell communication. Specifically, ephrins were reported to regulate the attachment, spreading, migration, differentiation, and age-associated senescence of stem cells (Arthur et al., 2011; Goichberg et al., 2013; Li and Johnson, 2013). Dysregulated cell-cell communication and hence proliferation, motility, and actin turnover of aged TDSCs were reported to be mediated by down-regulation of ephrin receptors EphA4, EphB2, and EphB4 and ligands EFNB1 (Popov et al., 2016). Interestingly, activation of EphA4- or EphB2- dependent reverse signaling in aged TDSCs restored migratory ability and normalized actin turnover while activation of EphA4 reverse signaling increased proliferation of aged TDSCs (Popov et al., 2016).
Extracellular Matrix
The ECM of tendon consists primarily of collagens (mainly collagen type I), proteoglycans, and glycoproteins. ECM functions to provide mechanical strength to tendon. In addition, it also functions to retain resident cells in place, localize and create gradients of instructive signals that guide stem cells in their processes of self-renewal and differentiation.
The alterations in the composition, topography and organization of ECM of aged tendons therefore can contribute to the loss of functions of tendon stem cells. While tendon-derived decellularized matrix promoted tendinous phenotype and inhibited osteogenesis of TDSCs in the presence of osteogenic induction conditions, bone-derived decellularized matrix induced osteogenic differentiation of TDSCs (Yin et al., 2013). The accumulation of AGEs with age likely plays a significant role in stem cell dysfunction during tendon aging. AGEs are proteins or lipids that become nonenzymatically glycated and oxidized after exposure to carbohydrates. Proteins are usually glycated through the lysine resides. Collagen, which is rich in lysine, is frequently glycated. Non-enzymatic glycation of collagen type I diminished collagen-proteoglycan binding and weakened cell adhesion and migration (Reigle et al., 2008). The formation of AGEs also resulted in the denaturation and cross-linking of collagen and the AGEs were nearly irreversible once formed. The mechanical and biological functions of long-lived proteins like collagen are therefore affected in the aging process. AGEs also increased the activity of transglutaminase, a cross-linking enzyme of ECM proteins, in tenocytes in vitro, further promoting collagen cross-linking (Rosenthal et al., 2009). The collagen cross-links limited fiber sliding, reduced viscoelasticity of tendon, and hence increased its stiffness and brittleness (Reddy, 2004; Gautieri et al., 2017), consistent with the increase in tendon stiffness with age (Andreassen et al., 1981; Haut et al., 1992). Matrix stiffness is known to regulate proliferation and lineage commitment of MSCs (Engler et al., 2006; Nam et al., 2011; Lee et al., 2014; Navaro et al., 2015). Matrix with lower stiffness promoted chondrogenic differentiation, whereas matrix with high stiffness promoted osteogenic differentiation of stem cells (Nam et al., 2011; Navaro et al., 2015). The increase in matrix stiffness as a result of age-induced collagen cross-links might underlie higher non-tenogenic differentiation potential of aged TDSCs. Bone morphogenetic protein-2 (BMP-2) stimulated non-tenogenic differentiation and promote proteoglycan deposition of TDSCs in vitro (Rui et al., 2013). A recent study showed that AGEs increased BMP-2 expression and accelerated progression of atherosclerotic calcification in diabetes (Wang et al., 2012). AGEs were also reported to increase the mRNA expression of BMP2, BMP4, and osteogenic markers of human yellow ligament cells isolated from cervical spine (Yokosuka et al., 2007). There has been no study about the effects of AGEs on TDSCs and is an area worthy of investigation.
The ECM composition can affect tendon stem cell fate. A deficiency in proteoglycans (biglycan and fibromodulin) resulted in altered differentiation of TDSCs towards the osteogenic linage via modulating the BMP signaling (Bi et al., 2007). Secreted protein acidic and rich in cysteine (Sparc), also known as osteonectin, is a collagen-binding matricellular glycoprotein involved in collagen fibril assembly and procollagen processing. It regulates cell-ECM interactions impacting upon cell signaling, adhesion, proliferation, migration, and survival. Sparc also regulates adipogenesis by modulating cell adhesiveness and cytoarchitecture. A recent study reported that the expression of Sparc decreased in aged mouse Achilles tendons (Gehwolf et al., 2016). The decrease in Sparc expression led to a more contracted phenotype, an altered actin cytoskeleton, and an elevated expression of the adipogenic marker genes Pparg and Cebpa with a concomitant increase in lipid deposits in both aged and Sparc -/- mouse tendons, a phenomenon that is commonly seen in elderly (Gehwolf et al., 2016).
The cytoskeleton of a cell and cell-matrix interactions affect stem cell migration, proliferation, and differentiation. Cell migration is strongly dependent on fibronectin, actin cytoskeletal organization, and turnover rate of actin filament. Integrins bind stem cells to different ECM components and regulate downstream signaling which are important for stem cell self-renewal and proliferation (Piwko-Czuchra et al., 2009; Ellis and Tanentzapf, 2010). Aged/degenerated TDSCs showed lower actin turnover, gene expression of collagen type I, and collagen type I-binding integrins α1, α2, and α11 but higher gene expression of fibronectin, fibronectin-binding integrins αv, β3, and β5 compared to young/healthy TDSCs (Kohler et al., 2013). The changes therefore might cause poor cell adhesion, migration, and actin dynamics of aged/degenerated TDSCs (Kohler et al., 2013). Integrin receptors and their ECM ligands function upstream of RhoA/ROCK signaling. Both the expression and activities of ROCK1 and ROCK2, which mediate RhoA-induced actin stress fiber formation via phosphorylation of myosin light chain (Schmitz et al., 2000), increased in aged/degenerated TDSCs (Kohler et al., 2013). Inhibition of ROCK1 and ROCK2 activities in aged/degenerated TDSCs with ROCK inhibitor Y-27632 restored the cell area, F-actin content and dynamics as well as migration ability, with results comparable to young/healthy TDSCs (Kohler et al., 2013).
CD44 is a cell-surface glycoprotein involved in cell adhesion and migration. As the expression of CD44 is implicated in poor tendon healing (Favata et al., 2006; Ansorge et al.,2009), the increased expression of CD44 in aged rat TDSCs might contribute to reduced tendon repair capacity with age (Zhou et al., 2010). Overexpression and knockdown experiments of CD44 in aged TDSCs would shed light on the aging mechanisms.
Collagen fibers are poorly organized in aged tendons (Gehwolf et al., 2016). This is partly due to chronic-inflammation and alteration in the production and activities of MMPs in aged tendons (Yu et al., 2013). The change of organization of collagen fibers from an intact, tightly-packed parallel orientation to a more random arrangement in aged tendons could affect the fate of resident tendon stem cells. Human fetal TDSCs seeded onto randomly-oriented fibrous scaffold underwent osteogenesis with lower levels of integrin α1, α5, and β1 subunits compared to cells seeded onto aligned fibers (Yin et al., 2010).
Locally Produced Biological Signals
Aging causes changes in the local production of biological signals at the stem cell niche which can profoundly impact tissue stem cell functions. Growth factors such as FGF, insulin growth factor-1 (IGF-1), vascular endothelial growth factor (VEGF), TGF-β1, platelet-derived growth factor (PDGF) and BMPs, as well as proteins in the Wnt/β-catenin, notch, and angiopoietin-1 signaling pathways are important modulators of stem cell functions. The Wnt/β-catenin and BMP signaling pathways enhanced non-tenogenic differentiation of TDSCs in vitro (Lui et al., 2013; Rui et al., 2013). Dysregulation of Wnt/β-catenin signaling pathway was observed in different aged stem cell and animal models (Brack et al., 2007; Liu et al., 2007; Gehwolf et al., 2016). However, there was no differences in mRNA expression of connective tissue growth factor (CTGF), TGF-β1, or stromal cell-derived factor 1 in tendons of old and young rats (Kostrominova and Brooks, 2013). The mRNA and protein expression of TGF-β1 also did not change with age in rat Achilles tenocytes (Yu et al., 2013). There was disruption of circadian control in aged mouse tendon (Yeung et al., 2014). One of the circadian genes, Gremlin-2 (Grem2) oscillated antiphase to BMP signaling and inhibited BMP-2 induced osteogenic differentiation of human tenocytes (Yeung et al., 2014). The study reported reduced Grem2 expression, dysregulated BMP signaling, and spontaneous calcification in aged mouse tendon, suggesting that inhibition of Grem2 might be involved in the calcification of aged tendons (Yeung et al., 2014). The growth factor profile of aged tendons and their effects on functions of tendon stem cells should be examined. Modulation of these growth factor signaling pathways might shed light on strategies to prevent tendon aging and promote healing of aged tendons after injury.
Inflamm-Aging
Aging is associated with reduced ability of the body to modulate inflammation, resulting in chronic low-grade inflammation termed “inflamm-aging” (Franceschi et al., 2000). Aged mice were reported to show higher serum levels of inflammatory cytokines such as interleukin-6 (IL-6) and tumor necrosis factor α (TNF-α) compared to young mice (Wahl et al., 2010). There were greater inflammatory changes with higher IL-1βand IL-6 levels in flexor digitorum tendons of aged rats performing high repetition low force handle-pulling task (HRLF) compared to tendons in young rats performing HRLF and aged rats not performing HRLF (Kietrys et al., 2012). More macrophages and CTGF-immunoreactive fibroblasts were observed in the peritendon of supraspinatus tendons in aged rats after HRLF (Kietrys et al., 2012). The greater chronic inflammation in aged rat tendons after upper extremity overuse was associated with a decrease in forelimb agility and lack of improvement of success in reaching task (Kietrys et al., 2012). Dakin et al. (2012) measured the level of prostaglandin E2 (PGE2) in injured equine flexor tendons and found that the level of PGE2 increased while the level of formyl peptide receptor 2 (FRP2)/ALX, a receptor responsible for suppressing inflammatory response, decreased during injury with increasing age of the horses. Uninjured tendon explants isolated from younger (<10 years) but not older horses (≥10 years) treated with IL-1β responded by increasing FRP2/ALX receptor (Dakin et al., 2012), suggesting that aged tendons have lower capacity to resolve inflammation. de Gonzalo-Calvo et al. (2010) measured the oxidative stress and inflammatory cytokines of three volunteer groups, consisting of normoxic middle-aged people, normoxic aged people older than 75 years, and hypoxic aged people older than 75 years. The results showed that the antioxidant defenses of older people with hypoxia was lower. Serum TNF-α was significantly increased in the aged population while IL-6 was highly elevated in the hypoxic elderly population (de Gonzalo-Calvo et al., 2010). Another recent clinical study on 831 subjects showed that circulating mitochondrial DNA (mtDNA) increased with age and significantly contributed to the maintenance of chronic, low-grade inflammation observed in the elderly subjects with an increase in serum TNF- α, IL-6, RANTES, and IL-1ra (Pinti et al., 2014). Moreover, mtDNA values of siblings were directly correlated, suggesting a role for familiar/genetic background in controlling the levels of circulating mtDNA (Pinti et al., 2014). In vitro stimulation of monocytes with mtDNA resulted in an increased production of TNF-α, supporting that mtDNA could modulate the production of proinflammatory cytokines (Pinti et al., 2014). These findings supported that aged individuals exhibited reduced capacity to resolve inflammation and aging might scramble tendon stem cell functions and promote the development of chronic tendinopathy through these pathways. Indeed, high concentration of PGE2 was reported to reduce the proliferation and enhance non-tenocyte differentiation of TDSCs (Zhang and Wang, 2014). IL-1β irreversibly inhibited tenogenic and adipogenic differentiation as well as reversibly inhibited chondrogenic and osteogenic differentiation of TDSCs isolated from injured mouse Achilles tendons (Zhang et al., 2015). IL-6 was reported to stimulate rat Achilles TDSCs’ proliferation and entry into cell cycle but inhibited the expression of tendon-related markers (Chen et al., 2018). Recent studies showed that senescent cells exhibited a robust increase in mRNA expression and secreted numerous bioactive factors, including degradative enzymes, inflammatory cytokines, and growth factors, which might drive stem cell dysfunction in aged stem cells through paracrine signaling (Coppe et al., 2010; Campisi et al., 2011). Whether this mechanism also accounts for age-related degenerative disorders of tendons and the factors secreted by senescent cells during tendon aging needs further research. The damage of DNA of stem cells and niche cells were hypothesized to be major contributors to inflamm-aging and the effect was propagated and amplified by macrophages (Bonafe et al., 2012). More researches are needed to verify this hypothesis in aged tendon stem cells.
Systemic Changes of Hormonal and Metabolic Signals
Given the remarkable ability of stem cells to sense and respond to the external stimuli, it is not surprising that the age-related systemic changes t can also impact stem cells and their niches. Chronic conditions such as hormone deficiency, diabetes mellitus (DM) and hypertension are common in elderly. The systemic changes of hormonal and metabolic signals as well as drug used in the treatment of these chronic conditions can alter niche through circulation, causing stem cells to lose or deviate from their original functions.
DM has been reported to increase the prevalence/incidence of tendinopathy (Ranger et al., 2016; Lui, 2017). A recent meta-analysis of clinical studies provide strong evidence that diabetes is associated with higher risk of tendinopathy (Ranger et al., 2016), despite that there were inconsistent findings regarding the effects of DM on structural and biomechanical properties of tendon in animal models (Lui, 2017). Potential mechanisms of DM in causing and exacerbating tendinopathy by modifying stem cell functions via AGE formation, hyperglycemia, insulin deficiency/resistance, and adipokine dysregulation have been discussed in another review and will not be repeated (Lui, 2017).
In women, estrogen can enhance tendon collagen synthesis. Estrogen deficiency was reported to negatively affect tendon metabolism and healing. Tenocytes isolated from ovariectomised rats were reported to show lower proliferation rate, collagen type I, aggrecan, elastin, fibronectin content in the supernatant, and migration rate but higher apoptosis, VEGF and MMP-13 compared to young tenocytes (Aydin et al., 2013; Torricelli et al., 2013). Collagen content was lower in Achilles tendons after ovariectomy compared to control rats (Ramos et al., 2012). The Achilles tendons from ovariectomised rabbits also showed lower gene expressions of proteoglycans aggrecan, biglycan, decorin, and versican compared to tendons from intact normal rabbits (Huisman et al., 2014). However, there was no changes in the biomechanical properties of patellar tendon of monkeys after ovariectomy (Wentorf et al., 2006). Age-associated estrogen deficiency therefore can alter matrix composition of tendons and may influence the functions of tendon stem cells. Whether TDSCs are sensitive to estrogen is a first step to understand the influence of estrogen deficiency in age-associated loss or dysfunction of tendon stem cells.
Potential Future Research Directions
Research on Treatments to Reverse the Intrinsic Cellular Deficits and Aged Niche of Tendon Stem Cells
As a new cell type identified in tendon, more studies are needed to fill the gaps of the aging mechanisms of tendon stem cells. Interventions that can combat protein and DNA damages, cellular senescence, oxidative stress, chronic inflammation; and inhibit AGE formation or promote its removal may rejuvenate aged tendon stem cells. These are attractive anti-aging options to explore. The growth factor and cytokine profile of aged tendons and their effects on functions of tendon stem cells should be examined. Notably, medium of young TDSCs under hypoxic culture condition was reported to enhance the migration, proliferation and mRNA expression of tendon-related markers as well as reduced senescence of aged TDSCs (Jiang et al., 2014). Exposure of aged rat patellar tendon TDSCs to young decellularized tendon ECM enhanced their proliferation, tendon-related marker expression (Scx, Tnmd), and stem cell marker expression (Oct-4, SSEA-1) as well as reduced SA-β-gal activity (Jiang et al., 2018).
Research on the Effects and Mechanisms of Common Aging Conditions on Tendon Stem Cells and Tendon
The effects of common aging conditions particularly diabetes, hypertension and hormonal deficiency and the drugs used on the functions of tendon stem cells and tendon should be examined. The sensitivity of TDSCs to estrogen has not been explored and should be examined. Similarly, studies on the effects of hyperglycemia, AGE, insulin deficiency/resistance, and adipokine dysregulation in diabetes on functions of tendon stem cells are needed.
Research on the Effects and Mechanisms of Exercise on Tendon Stem Cells and Tendon
TDSCs are sensitive to mechanical load. High uniaxial cyclic tensile loading (8%) induced osteogenic differentiation of TDSCs while low uniaxial cyclic tensile loading (4%) promoted tenogenic differentiation of TDSCs (Zhang and Wang, 2010b; Rui et al., 2011; Wang et al., 2018). Modulating the mechanical load experienced by tendon stem cells therefore may counteract the effect of aging on tendon. In this regard, moderate mechanical stretching (4%) of aged TDSCs in vitro significantly increased the protein expression of nucleostemin and mRNA expression of Nanog. It also increased the expression of tenocyte-related genes (Col1 and Tnmd) without increasing the expression of non-tenocyte-related genes (Lpl, Sox9 and Runx2) of aged TDSCs (Zhang and Wang, 2015).
In an in vivo study, moderate treadmill running of aged mice increased the proliferation rate of aged TDSCs in culture, decreased lipid deposition, proteoglycan accumulation and calcification, and increased the expression of nucleostemin-expressing TDSCs in the patellar tendons (Zhang and Wang, 2015). Moderate exercises can also mitigate the deleterious effects of aging on the niche of tendon stem cells by improving tendon microcirculation, matrix composition, and organization as well as reducing AGE level and matrix stiffness (Couppe et al., 2014; Wood and Brooks, 2016). With training, Col1a1 and Mmp8 expression in aged mouse Achilles tendons were restored to levels similar to adult controls (Wood and Brooks, 2016). Moderate treadmill running prior to an injury augmented tendon wound healing in an aged rat model with reduced vascularity, lipid-like structures, and senescent cells as well as increased production of more collagen fibrils and better fibril organization (Zhang et al., 2016). The effect was likely mediated by the improvement of metabolism and functional fitness of aged tendon stem cells because aged TDSCs isolated from exercised rats showed higher stem cell marker expression, proliferation, colony-forming ability, and tenocyte-related gene expression as well as lower non-tenocyte-related gene expression compared to aged cells isolated from cage control rats (Zhang et al., 2016). The beneficial effect of exercise on aged tendons could also be due to systemic effects in addition to direct loading as tail tendons from aged and exercised rats assumed the elastic characteristics of their younger counterparts after exercise (Lacroix et al., 2013). Stem cells expressed different channels that could sense membrane tension (Soria et al., 2013). One study showed that the stretch-mediated activation channel, cystic fibrosis transmembrane conductance regulator (CFTR), was up-regulated in TDSCs during tenogenic differentiation under mechanical stretching (Liu et al., 2017). Further studies to confirm the beneficial effects, optimal loading protocol, and mechanosensing mechanisms of excise on aged tendon stem cells and tendon should be done.
Conclusion and Future Perspectives
In summary, the composition, structure, and mechanical properties of tendons deteriorate with aging and are driven at least in part by an age-associated decline in the metabolism and functional fitness of tendon stem cells. Unlike their young and healthy counterparts, aged TDSCs were shown to be larger and flatten. The number and stemness of TDSCs isolated from aged tendons were lower. They exhibited altered multi-lineage differentiation potential, deficiency in cell adhesion, and migration as well as early cellular senescence. The intrinsic cellular deficits, aged niche, and systemic hormonal and metabolic changes associated with aging can drive the aging process of stem cells, some of which have been demonstrated in TDSCs recently. There is no evidence about the factor(s) triggering the aging of tendon stem cells. The authors speculate that they may work together in inducing functional deficits of tendon stem cells as aging is a continued process. Figure 1 summarizes the key biological characteristics of aged tendon, and potential factors influencing the fate and functions of TDSCs during tendon aging. Uncovering the mechanisms underpinning the regenerative decline of metabolism and functions of aged tendon stem cells would have profound implications for the development of stem cell-based therapeutics to reset the aging clock of tendons.
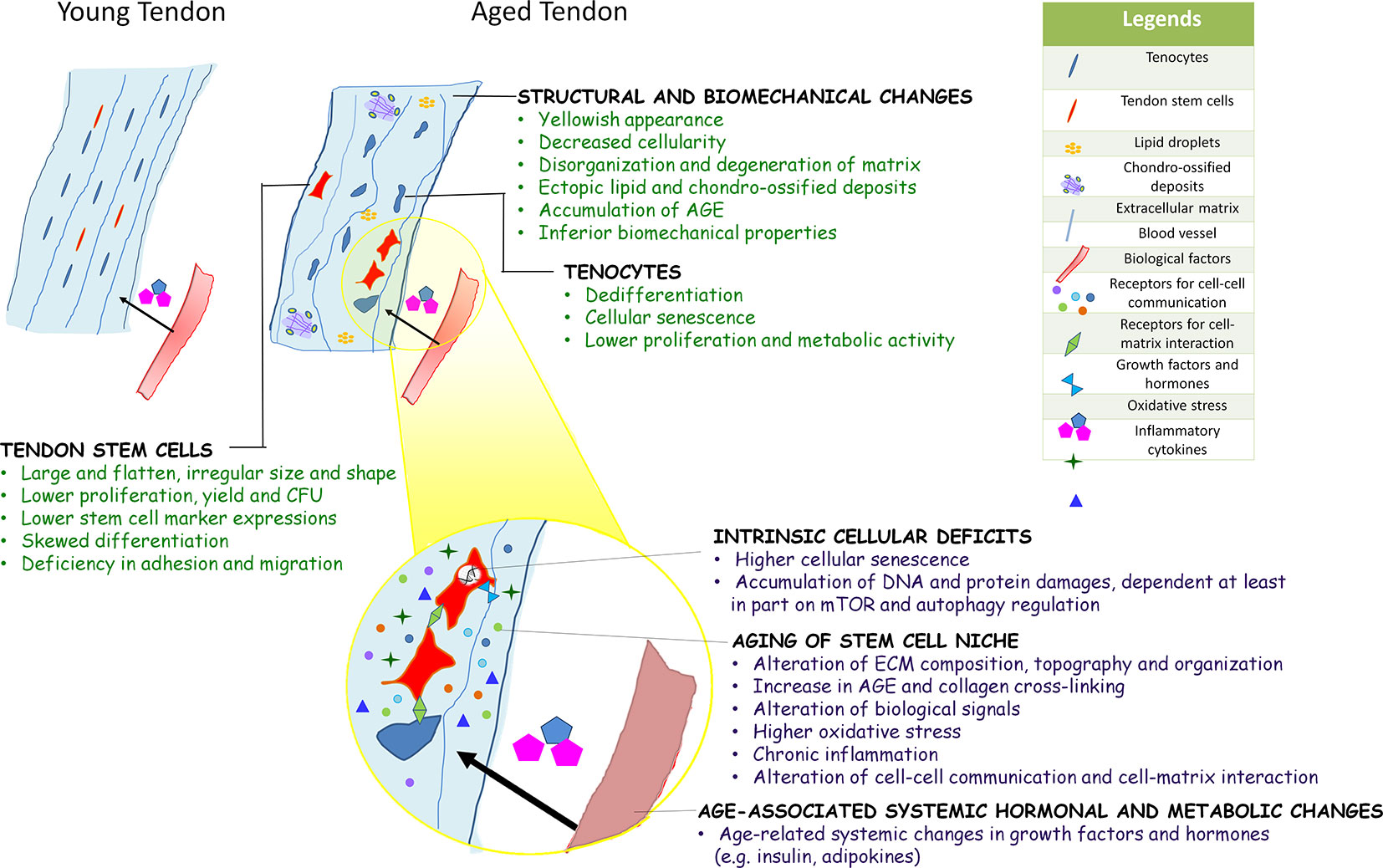
Figure 1 Diagram summarizing the key biological characteristics of aged tendon, and potential factors influencing the fate and functions of TDSCs during tendon aging. Current evidences on aging of other adult stem cells and limited data on tendon-derived stem cells (TDSCs) suggested that aging of tendon stem cell likely occurs via intrinsic cellular deficits, aging of stem cell niche, and age-associated systemic hormonal and metabolic changes. These factors interact with each other to affect stem cell function at multiple levels.
Author Contributions
PL conceived the idea, wrote, and proof-read the manuscript. CW wrote and proof-read the manuscript.
Funding
This study was supported by the one-line budget of The Hong Kong Polytechnic University (SF1920-CM).
Conflict of Interest
The authors declare that the research was conducted in the absence of any commercial or financial relationships that could be construed as a potential conflict of interest.
References
Ackerman, J. E., Bah, I., Jonason, J. H., Buckley, M. R., Loiselle, A. E. (2017). Aging does not alter tendon mechanical properties during homeostasis, but does impair flexor tendon healing. J. Orthop. Res. 35 (12), 2716–2724. doi: 10.1002/jor.23580
Albers, I. S., Zwerver, J., Diercks, R. L., Dekker, J. H., Van den Akker-Scheek, I. (2016). Incidence and prevalence of lower extremity tendinopathy in a Dutch general practice population: a cross-sectional study. BMC Musculoskelet Disord. 17, 16. doi: 10.1186/s12891-016-0885-2
Alberton, P., Dex, S., Popov, C., Shukunami, C., Schieker, M., Docheva, D. (2015). Loss of tenomodulin results in reduced self-renewal and augmented senescence of tendon stem/progenitor cells. Stem Cells Dev. 24 (5), 597–609. doi: 10.1089/scd.20140314
Andreassen, T. T., Seyerhansen, K., Bailey, A. J. (1981). Thermal-stability, mechanical-properties and reducible cross-links of rat tail tendon in experimental diabetes. Biochim. Biophys. Acta 677 (2), 313–317. doi: 10.1016/0304-4165(81)90101-X
Ansorge, H. L., Beredjiklian, P. K., Soslowsky, L. J. (2009). CD44 deficiency improves healing tendon mechanics and increases matrix and cytokine expression in a mouse patellar tendon injury model. J. Orthop. Res. 27 (10), 1386–1391. doi: 10.1002/jor.20891
Arthur, A., Zannettino, A., Panagopoulos, R., Koblar, S. A., Sims, N. A., Stylianou, C., et al. (2011). EphB/ephrin-B interactions mediate human MSC attachment, migration and osteochondral differentiation. Bone 48 (3), 533–542. doi: 10.1016/j.bone.2010.10.180
Aydin, A., Kenar, H., Atmaca, H., Alici, T., Gacar, G., Muezzinoglu, U. S., et al. (2013). The short- and long- term effects of estrogen deficiency on apoptosis in musculoskeletal tissues: an experimental animal model study. Arch. Iran Med. 16 (5), 271–276. doi: 013165/AIM.006
Bi, Y., Ehirchiou, D., Kilts, T. M., Inkson, C. A., Embree, M. C., Sonoyama, W., et al. (2007). Identification of tendon stem/progenitor cells and the role of the extracellular matrix in their niche. Nat. Med. 13 (10), 1219–1227. doi: 10.1038/nm1630
Bonafe, M., Storci, G., Franceschi, C. (2012). Inflamm-aging of the stem cell niche: breast cancer as a paradigmatic example: breakdown of the multi-shell cytokine network fuels cancer in aged people. Bioessays 34 (1), 40–49. doi: 10.1002/bies.201100104
Brack, A. S., Conboy, M. J., Roy, S., Lee, M., Kuo, C. J., Keller, C., et al. (2007). Increased Wnt signaling during aging alters muscle stem cell fate and increases fibrosis. Science 317 (5839), 807–810. doi: 10.1126/science.1144090
Brewer, B. J. (1979). Aging of the rotator cuff. Am. J. Sports Med. 7 (2), 102–110. doi: 10.1177/036354657900700206
Campisi, J., Andersen, J. K., Kapahi, P., Melov, S. (2011). Cellular senescence: a link between cancer and age-related degenerative disease? Seminar Cancer Biol. 21 (6), 354–359. doi: 10.1016/j.semcancer.2011.09.001
Chen, L., Liu, J., Tao, Z., Wang, G., Wang, Q., Liu, X. (2015a). The role of Pin1 protein in aging of human tendon stem/progenitor cells. Biochem. Biophys. Res. Commun. 464 (2), 487–492. doi: 10.1016/j.bbrc.2015.06.163
Chen, L., Wang, G. D., Liu, J. P., Wang, H. S., Liu, X. M., Wang, Q., et al. (2015b). miR-135a modulates tendon stem/progenitor cell senescence via suppressing ROCK1. Bone 71, 210–216. doi: 10.1016/j.bone.2014.11.001
Chen, H., Ge, H. A., Wu, G. B., Cheng, B., Lu, Y., Jiang, C. (2016). Autophagy prevents oxidative stress-induced loss of self-renewal capacity and stemness in human tendon stem cells by reducing ROS accumulation. Cell Physiol. Biochem. 39 (6), 2227–2238. doi: 10.1159/000447916
Chen, S., Deng, G., Li, K., Zheng, H., Wang, G., Yu, B., et al. (2018). Interleukin-6 promotes proliferation but inhibits tenogenic differentiation via the Janus Kinase/Signal transducers and activators of transcription 3 (JAK/STAT3) pathway in tendon-derived stem cells. Med. Sci. Monit. 24, 1567–1573. doi: 10.12659/MSM.908802
Cheng, Y., Wang, B., Zhou, H., Dang, S., Jin, M., Shi, Y., et al. (2015). Autophagy is required for the maintenance of liver progenitor cell functionality. Cell Physiol. Biochem. 36 (3), 1163–1174. doi: 10.1159/000430287
Collado, M., Blasco, M. A., Serrano, M. (2007). Cellular senescence in cancer and aging. Cell 130 (2), 223–233. doi: 10.1016/j.cell.2007.07.003
Conboy, I. M., Conboy, M. J., Wagers, A. J., Girma, E. R., Weissman, I. L., Rando, T. A. (2005). Rejuvenation of aged progenitor cells by exposure to a young systemic environment. Nature 433 (7027), 760–764. doi: 10.1038/nature03260
Coppe, J. P., Desprez, P. Y., Krtolica, A., Campisi, J. (2010). The senescence-associated secretory phenotype: the dark side of tumor suppression. Annu. Rev. Pathol. 5, 99–118. doi: 10.1146/annurev-pathol-121808-102144
Couppe, C., Hansen, P., Kongsgaard, M., Kovanen, V., Suetta, C., Aagaard, P., et al. (2009). Mechanical properties and collagen cross-linking of the patellar tendon in old and young men. J. Appl. Physiol. (1985), 880–886. doi: 10.1152/japplphysiol.002912009
Couppe, C., Svensson, R. B., Grosset, J. F., Kovanen, V., Nielsen, R. H., Olsen, M. R., et al. (2014). Life-long endurance running is associated with reduced glycation and mechanical stress in connective tissue. Age (Dordr) 36 (4), 9665. doi: 10.1007/s11357-014-9665-9
Dakin, S. G., Dudhia, J., Werling, N. J., Werling, D., Abayasekara, D. R., Smith, R. K. (2012). Inflamm-aging and arachidonic acid metabolite difference with stage of tendon disease. PloS One 7 (11), e48978. doi: 10.1371/journal.pone.0048978
de Gonzalo-Calvo, D., Neitzert, K., Fernandez, M., Vega-Naredo, I., Caballero, B., Garcia-Macia, M., et al. (2010). Differential inflammatory responses in aging and disease: TNF-alpha and IL-6 as possible biomarkers. Free Radic. Biol. Med. 49 (5), 733–737. doi: 10.1016/j.freeradbiomed.2010.05.019
de Jonge, S., van den Berg, C., de Vos, R. J., van der Heide, H. J., Weir, A., Verhaar, J. A., et al. (2011). Incidence of midportion Achilles tendinopathy in the general population. Br. J. Sports Med. 45 (13), 1026–1028. doi: 10.1136/bjsports-2011-090342
Delabastita, T., Bogaerts, S., Vanwanseele, B. (2018). Age-related changes in Achilles tendon stiffness and impact of functional activities: a systematic review and meta-analysis. J. Aging Phys. Act. 27 (1), 116–127. doi: 10.1123/japa.2017-0359
Driver, J. A., Lu, K. P. (2010). Pin1: a new genetic link between Alzheimer’s disease, cancer and aging. Curr. Aging Sci. 3 (3), 158–165. doi: 10.2174/1874609811003030158
Dudhia, J., Scott, C. M., Draper, E. R., Heinegard, D., Pitsillides, A. A., Smith, R. K. (2007). Aging enhances a mechanically-induced reduction in tendon strength by an active process involving matrix metalloproteinase activity. Aging Cell. 6 (4), 547–556. doi: 10.1111/j.1474-9726.2007.00307.x
Dunkman, A. A., Buckley, M. R., Mienaltowski, M. J., Adams, S. M., Thomas, S. J., Satchell, L., et al. (2013). Decorin expression is important for age-related changes in tendon structure and mechanical properties. Matrix Biol. 32 (1), 3–13. doi: 10.1016/j.matbio.2012.11.005
Ellis, S. J., Tanentzapf, G. (2010). Integrin-mediated adhesion and stem-cell-niche interactions. Cell Tissue Res. 339 (1), 121–130. doi: 10.1007/s00441-009-0828-4
Engler, A. J., Sen, S., Sweeney, H. L., Sischer, D. E. (2006). Matrix elasticity directs stem cell lineage specification. Cell 126 (4), 677–689. doi: 10.1016/j.cell.2006.06.044
Favata, M., Beredjiklian, P. K., Zgonis, M. H., Beason, D. P., Crombleholme, T. M., Jawad, A. F., et al. (2006). Regenerative properties of fetal sheep tendon are not adversely affected by transplantation into an adult environment. J. Orthop. Res. 24 (11), 2124–2132. doi: 10.1002/jor.20271
Fehringer, E. V., Sun, J., VanOeveren, L. S., Keller, B. K., Matsen, F. A. (2008). Full-thickness rotator cuff tear prevalence and correlation with function and co-morbidities in patients sixty-five years and older. J. Shoulder Elbow Surg. 17 (6), 881–885. doi: 10.1016/j.jse.2008.05.039
Franceschi, C., Bonafè, M., Valensin, S., Olivieri, F., De Luca, M., Ottaviani, E., et al. (2000). Inflamm-aging: an evolutionary perspective on immunosenescence. Ann. N. Y. Acad. Sci. 908, 244–254. doi: 10.1111/j.1749-6632.2000.tb06651.x
Fukada, S., Ma, Y., Uezumi, A. (2014). Adult stem cell and mesenchymal progenitor theories of aging. Front. Cell. Dev. Biol. 2, 10. doi: 10.3389/fcell.2014.00010
Funakoshi, T., Iwasaki, N., Kamishima, T., Nishida, M., Ito, Y., Kondo, M., et al. (2010). In vivo visualization of vascular patterns of rotator cuff tears using contrast-enhanced ultrasound. Am. J. Sports Med. 38 (12), 2464–2471. doi: 10.1177/0363546510375536
Gautieri, A., Passini, F. S., Silvan, U., Guizar-Sicairos, M., Carimati, G., Volpi, P., et al. (2017). Advanced glycation end-products: mechanics of aged collagen from molecule to tissue. Matrix Biol. 59, 95–108. doi: 10.1016/j.matbio.2016.09.001
Gehwolf, R., Wagner, A., Lehner, C., Bradshaw, A. D., Scharler, C., Niestrawska, J. A., et al. (2016). Pleiotropic roles of the matricellular protein Sparc in tendon maturation and ageing. Sci. Rep. 6, 32635. doi: 10.1038/srep32635
Gillis, C., Pool, R. R., Meagher, D. M., Stover, S. M., Reiser, K., Willits, N. (1997). Effect of maturation and aging on the histomorphometric and biochemical characteristics of equine superficial digital flexor tendon. Am. J. Vet. Res. 58 (4), 425–430.
Goichberg, P., Kannappan, R., Cimini, M., Bai, Y., Sanada, F., Sorrentino, A., et al. (2013). Age-associated defects in EphA2 signaling impair the migration of human cardiac progenitor cells. Circulation 128 (20), 2211–2223. doi: 10.1161/CIRCULATIONAHA.113.004698
Han, W., Wang, B., Liu, J., Chen, L. (2017). The p16/miR-217/EGR1 pathway modulates age-related tenogenic differentiation in tendon stem/progenitor cells. Acta Biochim. Biophys. Sin. (Shanghai). 49 (11), 1015–1021. doi: 10.1093/abbs/gmx104
Haut, R. C., Lancaster, R. L., DeCamp, C. E. (1992). Mechanical properties of the canine patellar tendon: some correlations with age and the content of collagen. J. Biomech. 25 (2), 163–173. doi: 10.1016/0021-9290(92)90273-4
Hoare, M., Das, T., Alexander, G. (2010). Ageing, telomeres, senescence, and liver injury. J. Hepatol. 53 (5), 950–961. doi: 10.1016/j.jhep.2010.06.009
Hsiao, M. Y., Chen, Y. C., Lin, C. Y., Chen, W. S., Wang, T. G. (2015). Reduced patellar tendon elasticity with aging: In vivo assessment by shear wave elastography. Ultrasound Med. Biol. 41 (11), 2899–2905. doi: 10.1016/j.ultrasmedbio.2015.07.008
Hu, C., Zhang, Y., Tang, K., Luo, Y., Liu, Y., Chen, W. (2017). Downregulation of CITED2 contributes to TGFβ-mediated senescence of tendon-derived stem cells. Cell Tissue Res. 368 (1), 93–104. doi: 10.1007/s00441-016-2552-1
Huisman, E. S., Andersson, G., Scott, A., Reno, C. R., Hart, D. A., Thomton, G. M. (2014). Regional molecular and cellular differences in the female rabbit Achilles tendon complex: potential implications for understanding responses to loading. J. Anat. 224 (5), 528–547. doi: 10.1111/joa.12169
Iagnocco, A., Filippucci, E., Sakellariou, G., Ceccarelli, F., Di Geso, L., Carili, L., et al. (2013). Ultrasound imaging for the rheumatologist XLIV. Ultrasound of the shoulder in healthy individuals. Clin. Exp. Rheumatol. 31 (2), 165–171.
Ippolito, E., Natali, P. G., Postacchini, F., Accinni, L., De Martino, C. (1980). Morphological, immunochemical and biochemical study of rabbit Achilles tendon at various ages. JBJS 62 (4), 583–598.
Janzen, V., Forkert, R., Fleming, H. E., Saito, Y., Waring, M. T., Dombkowski, D. M., et al. (2006). Stem-cell ageing modified by the cyclin-dependent kinase inhibitor p16INK4a. Nature 443 (7110), 421–426. doi: 10.1038/nature05159
Jiang, D., Jiang, Z., Zhang, Y., Wang, S., Yang, S., Xu, B., et al. (2014). Effect of young extrinsic environment stimulated by hypoxia on the function of aged tendon stem cell. Cell Biochem. Biophys. 70 (2), 967–973. doi: 10.1007/s12013-014-0004-7
Jiang, D., Xu, B., Gao, P. (2018). Effects of young extracellular matrix on the biological characteristics of aged tendon stem cells. Adv. Clin. Exp. Med. 27 (12), 625–1630. doi: 10.17219/acem/75503
Josa, L. G., Kannus, P. (1997). Human Tendons: Anatomy, Physiology and Pathology (Champaign, IL: Human Kinetics Publishers).
Kiderlen, S., Polzer, C., Radler, J. O., Docheva, D., Clausen-Schaumann, H., Sudhop, S. (2019). Age related changes in cell stiffness of tendon stem/progenitor cells an rejuvenating effect of ROCK-inhibition. Biochem. Biophys. Res. Commun. 509 (3), 839–844. doi: 10.1016/j.bbrc.2019.01.027
Kietrys, D. M., Barr-Gillespie, A. E., Amin, M., Wade, C. K., Popoff, S. N., Barbe, M. F. (2012). Aging contributes to inflammation in upper extremity tendons and declines in forelimb agility in a rat model of upper extremity overuse. PloS One 7 (10), e46954. doi: 10.1371/journal.pone.0046954
Klatte-Schulz, F., Pauly, S., Scheibel, M., Greiner, S., Gerhardt, C., Schmidmaier, G., et al. (2012). Influence of age on the cell biological characteristics and the stimulation potential of male human tenocyte-like cells. Eur. Cell Mater 24, 74–89. doi: 10.22203/eCM.v024a06
Kohler, J., Popov, C., Klotz, B., Alberton, P., Prall, W. C., Haasters, F., et al. (2013). Uncovering the cellular and molecular changes in tendon stem/progenitor cells attributed to tendon aging and degeneration. Aging Cell 12 (6), 988–999. doi: 10.1111/acel.12124
Kostrominova, T., Brooks, S. (2013). Age related changes in structure and extracellular matrix protein expression levels in rat tendons. Age 35 (6), 2203–2214. doi: 10.1007/s11357-013-9514-2
Lacroix, A. S., Duenwald-Kuehl, S. E., Brickson, S., Akins, T. L., Diffee, G., Aiken, J., et al. (2013). Effect of age and exercise on the viscoelastic properties of rat tail tendon. Ann. Biomed. Eng. 41 (6), 1120–1128. doi: 10.1007/s10439-013-0796-4
Landi, A., Elves, M., Piaggi, W. (1983). The blood flow of rabbits’ tendons. Acta Orthop. Scand. 54 (6), 832–835. doi: 10.3109/17453678308992917
Lee, J., Abdeen, A. A., Huang, T. H., Kilian, K. A. (2014). Controlling cell geometry on substrates of variables stiffness can tune the degree of osteogenesis in human mesenchymal stem cells. J. Mech. Behav. Biomed. Mater. 38, 209–218. doi: 10.1016/j.jmbbm.2014.01.009
Li, J., Johnson, S. E. (2013). Ephrin-A5 promotes bovine muscle progenitor cell migration before mitotic activation. J. Anim. Sci. 91 (3), 1086–1093. doi: 10.2527/jas.2012-5728
Li, H., Liu, P., Xu, S., Li, Y., Dekker, J. D., Li, B., et al. (2017). FOXP1 controls mesenchymal stem cell commitment and senescence during skeletal aging. J. Clin. Invest. 127 (4), 1241–1253. doi: 10.1172/JCI89511
Liu, H., Fergusson, M. M., Castilho, R. M., Liu, J., Cao, L., Chen, J., et al. (2007). Augmented Wnt signaling in a mammalian model of accelerated aging. Science 317 (5839), 803–806. doi: 10.1126/science.1143578
Liu, Y., Xu, J., Xu, L., Wu, T., Sun, Y., Lee, Y. W., et al. (2017). Cystic fibrosis transmembrane conductance regulator mediates tenogenic differentiation of tendon-derived stem cells and tendon repair: accelerating tendon injury healing by intervening in its downstream signaling. FASEB J. 31 (9), 3800–3815. doi: 10.1096/fj.201601181R
Lui, P. P., Chan, K. M. (2011). Tendon-derived stem cells (TDSC): from basic science to potential roles in tendon pathology and tissue engineering applications. Stem Cell Rev. 7 (4), 883–897. doi: 10.1007/s12015-011-9276-0
Lui, P. P., Lee, Y. W., Wong, Y. M., Zhang, X., Dai, K., Rolf, C. G. (2013). Expression of Wnt pathway mediators in metaplasic tissue in animal model and clinical samples of tendinopathy. Rheumatol. (Oxford) 52 (9), 1609–1618. doi: 10.1093/rheumatology/ket214
Lui, P. P. (2015). Markers for the identification of tendon-derived stem cells in vitro and tendon stem cells in situ – update and future development. Stem Cell Res. Ther. 6, 106. doi: 10.1186/s13287-015-0097-y
Lui, P. P. (2017). Tendinopathy in diabetes patients – epidemiology, pathogenesis and management. Scand. J. Med. Sci. Sports 27 (8), 776–787. doi: 10.1111/sms.12824
Molofsky, A. V., Slutsky, S. G., Joseph, N. M., He, S., Pardal, R., Krishnamurthy, J., et al. (2006). Increasing p16INK4a expression decreases forebrain progenitors and neurogenesis during ageing. Nature 443 (7110), 448–452. doi: 10.1038/nature05091
Nam, J., Johnson, J., Lannutti, J. J., Agarwal, S. (2011). Modulation of embryonic mesenchymal progenitor cell differentiation via control over pure mechanical modulus in electrospun nanofibers. Acta Biomater. 7 (4), 1516–1524. doi: 10.1016/j.actbio.2010.11.022
Navaro, Y., Bleich-Kimelman, N., Hazanov, L., Mironi-Harpaz, I., Shachaf, Y., Garty, S., et al. (2015). Matrix stiffness determines the fate of nucleus pulposus-derived stem cells. Biomaterials 49, 68–76. doi: 10.1016/j.biomaterials.2015.01.021
Pardes, A. M., Beach, Z. M., Raja, H., Rodriguez, A. B., Freedman, B. R., Soslowsky, L. J. (2017). Aging leads to inferior Achilles tendon mechanics and altered ankle function in rodents. J. Biomech. 60, 30–38. doi: 10.1016/j.jbiomech.2017.06.008
Peffers, M. J., Thorpe, C. T., Collins, J. A., Eong, R., Wei, T. K. J., Screen, H. R. C., et al. (2014). Proteomic analysis reveals age-related changes in tendon matrix composition, with age- and injury- specific matrix fragmentation. J. Biol. Chem. 289 (37), 25867–25878. doi: 10.1074/jbc.M114.566554
Pinti, M., Cevenini, E., Nasi, M., De Biasi, S., Salvioli, S., Monti, D., et al. (2014). Circulating mitochondrial DNA increases with age and is a familiar trait: Implications for inflamm-aging. Eur. J. Immunol. 44 (5), 1552–1562. doi: 10.1002/eji.201343921
Piwko-Czuchra, A., Koegel, H., Meyer, H., Bauer, M., Werner, S., Brakebusch, C., et al. (2009). B1 integrin-mediated adhesion signaling is essential for epidermal progenitor cell expansion. PloS One 4 (5), e5488. doi: 10.1371/journal.pone.0005488
Popov, C., Kohler, J., Docheva, D. (2016). Activation of EphA4 and EphB2 reverse signaling restores the age-associated reduction of self-renewal, migration, and actin turnover in human tendon stem/progenitor cells. Front. Aging Neurosci. 7, 246. doi: 10.3389/fnagi.2015.00246
Ramos, J. E., Al-Nakkash, L., Peterson, A., Gump, B. S., Janjulia, T., Moore, M. S., et al. (2012). The soy isoflavone genistein inhibits the reduction in Achilles tendon collagen content induced by ovariectomy in rats. Scand. J. Med. Sci. Sports 22 (5), e108–e114. doi: 10.1111/j.1600-0838.2012.01516.x
Ranger, T. A., Wong, A. M., Cook, J. L., Gaida, J. E. (2016). Is there an association between tendinopathy and diabetes mellitus? systematic Rev. meta-analysis. Br. J. Sports Med. 50 (16), 982–989. doi: 10.1136/bjsports-2015-094735
Reddy, G. K. (2004). Cross-linking in collagen by nonenzymatic glycation increases the matrix stiffness in rabbit achilles tendon. Exp. Diabetes Res. 5 (2), 143–153. doi: 10.1080/15438600490277860
Reigle, K. L., Di Lullo, G., Turner, K. R., Last, J. A., Chervoneva, I., Birk, D. E., et al. (2008). Non-enzymatic glycation of type I collagen diminishes collagen-proteoglycan binding and weakens cell adhesion. J. Cell Biochem. 104 (5), 1684–1698. doi: 10.1002/jcb.21735
Riley, G. P., Harrall, R. L., Constnt, C. R., Chard, M. D., Cawston, T. E., Hazleman, B. L. (1994). Glycosaminoglycans of human rotator cuff tendons: changes with age and in chronic rotator cuff tendinitis. Ann. Rheumatol. Dis. 53 (6), 367–376. doi: 10.1136/ard.53.6.367
Rosenthal, A. K., Gohr, C. M., Mitton, E., Monnier, V., Burner, T. (2009). Advanced glycation end products increase transglutaminase activity in primary porcine tenocytes. J. Investig. Med. 57 (2), 460–466. doi: 10.2310/JIM.0b013e3181954ac6
Rubinsztein, D. C., Marino, G., Kroemer, G. (2011). Autophagy and aging. Cell 146 (5), 682–695. doi: 10.1016/j.cell.2011.07.030
Ruckh, J. M., Zhao, J. W., Shadrach, J. L., van Wijngaarden, P., Rao, T. N., Wagers, A. J., et al. (2012). Rejuvenation of regeneration in the aging central nervous system. Cell Stem Cell. 10 (1), 96–103. doi: 10.1016/j.stem.2011.11.019
Rudzki, J. R., Adler, R. S., Warren, R. F., Kadrmas, W. R., Verma, N., Pearle, A. D., et al. (2008). Contrast-enhanced ultrasound characterization of the vascularity of the rotator cuff tendon: age- and activity-related changes in the intact asymptomatic rotator cuff. J. Shoulder Elbow Surg. 17 (1 suppl), 96S–100S. doi: 10.1016/j.jse.2007.07.004
Rui, Y. F., Lui, P. P., Li, G., Fu, S. C., Lee, Y. W., Chan, K. M. (2010). Isolation and characterization of multipotent rat tendon-derived stem cells. Tissue Eng. Part A. 16 (5), 1549–1558. doi: 10.1089/ten.TEA.20090529
Rui, Y. F., Lui, P. P., Ni, M., Chan, L. S., Lee, Y. W., Chan, K. M. (2011). Mechanical loading increased BMP-2 expression which promoted osteogenic differentiation of tendon-derived stem cells. J. Orthop. Res. 29 (3), 390–396. doi: 10.1002/jor.21218
Rui, Y. F., Lui, P. P., Wong, Y. M., Tan, Q., Chan, K. M. (2013). BMP-2 stimulated non-tenogenic differentiation and promoted proteoglycan deposition of tendon-derived stem cells (TDSCs) in vitro. J. Orthop. Res. 31 (5), 746–753. doi: 10.1002/jor.22290
Ruzzini, L., Abbruzzese, F., Rainer, A., Longo, U., Trombetta, M., Maffulli, N., et al. (2014). Characterization of age-related changes of tendon stem cells from adult human tendons. Knee Surg. Sports Traumatol. Arthrosc. 22 (11), 2856–2866. doi: 10.1007/s00167-013-2457-4
Salini, V., Vanni, D., Pantalone, A., Abate, M. (2015). Platelet rich plasma therapy in non-insertional achilles tendinopathy: the efficacy is reduced in 60-years old people compared to young and middle-age individuals. Front. Aging Neurosci. 7, 228. doi: 10.3389/fnagi.2015.00228
Sato, T., Katagiri, K., Yokonishi, T., Kubota, Y., Inoue, K., Ogonuki, N., et al. (2011). In vitro production of fertile sperm from murine spermatogonial stem cell lines. Nat. Commun. 2, 472. doi: 10.1038/ncomms1478
Sbrana, F. V., Cortini, M., Avnet, S., Perut, F., Columbaro, M., De Milito, A., et al. (2016). The role of autophagy in the maintenance of stemness and differentiation of mesenchymal stem cells. Stem Cell Rev. 12 (6), 621–633. doi: 10.1007/s12015-016-9690-4
Schmitz, A. A., Govek, E. E., Bottner, B., Van Aelst, L. (2000). Rho GTPases: signaling, migration, and invasion. Exp. Cell Res. 261 (1), 1–12. doi: 10.1006/excr.20005049
Sharpless, N. E., DePinho, R. A. (2007). How stem cells age and why this makes us grow old. Nat. Rev. Mol. Cell. Biol. 8 (9), 703–713. doi: 10.1038/nrm2241
Shibata, K. R., Aoyama, T., Shima, Y., Fukiage, K., Otsuka, S., Furu, M., et al. (2007). Expression of the p16INK4A gene is associated closely with senescence of human mesenchymal stem cells and is potentially silenced by DNA methylation during in vitro expansion. Stem Cells 25 (9), 2371–2382. doi: 10.1634/stemcells.2007-0225
Soria, B., Navas, S., Hmadcha, A., Hamill, O. P. (2013). Single mechanosensitive and Ca2+- sensitive channel currents recorded from mouse and human embryonic stem cells. J. Membr. Biol. 246 (3), 215–230. doi: 10.1007/s00232-012-9523-6
Sousa-Victor, P., Gutarra, S., Garcia-Prat, L., Rodriguez-Ubreva, J., Ortet, L., Ruiz-Bonilla, V., et al. (2014). Geriatric muscle stem cells switch reversible quiescence into senescence. Nature 506 (7488), 316–321. doi: 10.1038/nature13013
Tan, Q., Lui, P. P., Rui, Y. F., Wong, Y. M. (2012). Comparison of potentials of stem cells isolated from tendon and bone marrow for musculoskeletal tissue engineering. Tissue Eng. Part A. 18 (7-8), 840–851. doi: 10.1089/ten.TEA.2011.0362
Teunis, T., Lubberts, B., Reilly, B. T., Rin, D. (2014). A systematic review and pooled analysis of the prevalence of rotator cuff disease with increasing age. J. Shoulder Elbow Surg. 23 (12), 1913–1921. doi: 10.1016/j.jse.2014.08.001
Thorpe, C. T., McDermott, B. T., Goodship, A. E., Clegg, P. D., Birch, H. L. (2016). Ageing does not result in a decline in cell synthetic activity in an injury prone tendon. Scand. J. Med. Sci. Sports. 26 (6), 684–693. doi: 10.1111/sms.12500
Tomaru, U., Takahashi, S., Ishizu, A., Miyatake, Y., Gohda, A., Suzuki, S., et al. (2012). Decreased proteasomal activity causes age-related phenotypes and promotes the development of metabolic abnormalities. Am. J. Pathol. 180 (3), 963–972. doi: 10.1016/j.ajpath.2011.11.012
Torricelli, P., Veronesi, F., Pagani, S., Maffulli, N., Masiero, S., Frizziero, A., et al. (2013). In vitro tenocyte metabolism in aging and oestrogen deficiency. Age (Dordr) 35 (6), 2125–2136. doi: 10.1007/s11357-012-9500-0
Tsai, W. C., Chang, H. N., Yu, T. Y., Chien, C. H., Fu, L. F., Liang, F. C., et al. (2011). Decreased proliferation of aging tenocytes is associated with down-regulation of cellular senescence-inhibited gene and up-regulation of p27. J. Orthop. Res. 29 (10), 1598–1603. doi: 10.1002/jor.21418
Turan, A., Teber, M. A., Yakut, Z. I., Unlu, H. A., Hekimoglu, B. (2015). Sonoelastographic assessment of the age-related changes of the Achilles tendon. Med. Ultrason. 17 (1), 58–61. doi: 10.11152/mu.2013.2066.171.ayt
Wahl, E. C., Aronson, J., Liu, L., Fowlkes, J. L., Thrailkill, K. M., Bunn, R. C., et al. (2010). Restoration of regenerative osteoblastogenesis in aged mice: modulation of TNF. J. Bone Miner. Res. 5 (1), 114–123. doi: 10.1359/jbmr.090708
Wang, Z., Jiang, Y., Liu, N., Ren, L., Zhu, Y., An, Y., et al. (2012). Advanced glycation end-product Nϵ-carboxymethyl-Lysine accelerates progression of artherosclerotic calcification in diabetes. Atherosclerosis 221 (2), 387–396. doi: 10.1016/j.atherosclerosis.2012.01.019
Wang, B., Guo, J., Feng, L., Suen, C. W., Fu, W. M., Zhang, J. F., et al. (2016). miR124 suppresses collagen formation of human tendon derived stem cells through targeting egr1. Exp. Cell Res. 347 (2), 360–366. doi: 10.1016/j.yexcr.2016.08.018
Wang, T., Thien, C., Wang, C., Ni, M., Gao, J., Wang, A., et al. (2018). 3D uniaxial mechanical stimulation induces tenogenic differentiation of tendon-derived stem cells through a PI3K/AKT signaling pathway. FASEB J. 32 (9), 4804–4814. doi: 10.1096/fj.201701384R
Warr, M. R., Binnewies, M., Flach, J., Reynaud, D., Garg, T., Malhotra, R., et al. (2013). FOXO3A directs a protective autophagy program in haematopoietic stem cells. Nature 494 (7437), 323–327. doi: 10.1038/nature11895
Webb, S., Gabrelow, C., Pierce, J., Gibb, E., Elliott, J. (2016). Retinoic acid receptor signaling preserves tendon stem cell characteristics and prevents spontaneous differentiation in vitro. Stem Cell Res Ther 7, 45. doi: 10.1186/s13287-016-0306-3
Wentorf, F. A., Sudoh, K., Moses, C., Arendt, E. A., Carlson, C. S. (2006). The effects of estrogen on material and mechanical properties of the intra- and extra- articular knee structures. Am. J. Sports Med. 34 (12), 1948–1952. doi: 10.1177/0363546506290060
Wilkinson, J. E., Burmeister, L., Brooks, S. V., Chan, C. C., Friedline, S., Harrison, D. E., et al. (2012). Rapamycin slows aging in mice. Aging Cell 11 (4), 675–682. doi: 10.1111/j.1474-9726.2012.00832.x
Wood, L. K., Brooks, S. V. (2016). Ten weeks of treadmill running decreases stiffness and increases collagen turnover in tendons of old mice. J. Orthop. Res. 34 (2), 346–353. doi: 10.1002/jor.22824
Wu, H., Zhao, G., Zu, H., Wang, J. H., Wang, Q. M. (2015). Aging-related viscoelasticity variation of tendon stem cells (TSCs) characterized by quartz thickness shear mode (TSM) resonators. Sens. Actuators (Warrendale Pa). 210, 369–380. doi: 10.1016%2Fj.snb.2014.12.117
Xu, H., Liu, F. (2018). Downregulation of FOXP1 correlates with tendon stem/progenitor cells aging. Biochem. Biophys. Res. Commun. 504 (1), 96–102. doi: 10.1016/j.bbrc.2018.08.136
Yeung, C. Y., Gossan, N., Lu, Y., Hughes, A., Hensman, J. J., Bayer, M. L., et al. (2014). Gremlin-2 is a BMP antagonist that is regulated by the circadian clock. Sci. Rep. 4, 5183. doi: 10.1038/srep05183
Yin, Z., Chen, X., Chen, J. L., Shen, W. L., Hieu Nguyen, T. M., Gao, L., et al. (2010). The regulation of tendon stem cell differentiation by the alignment of nanofibers. Biomaterials 31 (8), 2163–2175. doi: 10.1016/j.biomaterials.2009.11.083
Yin, Z., Chen, X., Zhu, T., Hu, J. J., Song, H. X., Shen, W. L., et al. (2013). The effect of decellularized matrices on human tendon stem/progenitor cell differentiation and tendon repair. Acta Biomater. 9 (12), 9317–9329. doi: 10.1016/j.actbio.2013.07.022
Yin, H., Caceres, M. D., Yan, Z., Schieker, M., Nerlich, M., Docheva, D. (2019). Tenomodulin regulates matrix remodeling of mouse tendon stem/progenitor cells in an ex vivo collagen I gel model. Biochem. Biophys. Res. Commun. 512 (4), 691–697. doi: 10.1016/j.bbrc.2019.03.063
Yokosuka, K., Park, J. S., Jimbo, K., Yoshida, T., Yamada, K., Sato, K., et al. (2007). Immunohistochemical demonstration of advanced glycation end products and the effects of advanced glycation end products in ossified ligament tissues in vitro. Spine (Phila Pa 1976) 32 (11), E337–E339. doi: 10.1097/01.brs.0000263417.17526.35
Yu, T. Y., Pang, J. H., Wu, K. P., Chen, M. J., Chen, C. H., Tsai, W. C. (2013). Aging is associated with increased activities of matrix metalloproteinase-2 and -9 in tenocytes. BMC Musculoskelet. Disord. 14, 2. doi: 10.1186/1471-2474-14-2
Zaseck, L. W., Miller, R. A., Brooks, S. V. (2016). Rapamycin attenuates age-associated changes in tibialis anterior tendon viscoelastic properties. J. Gerontol. A Biol. Sci. Med. Sci. 71 (7), 858–865. doi: 10.1093/gerona/glv307
Zhang, J., Wang, J. H. (2010a). Characterization of differential properties of rabbit tendon stem cells and tenocytes. BMC Musculoskelet Disord. 11, 10. doi: 10.1186/1471-2474-11-10
Zhang, J., Wang, J. H. (2010b). Mechanobiological response of tendon stem cells: implications of tendon homeostasis and pathogenesis of tendinopathy. J. Orthop. Res. 28 (5), 639–643. doi: 10.1002/jor.21046
Zhang, J., Wang, J. H. (2014). Prostaglandin E2 (PGE2) exerts biphasic effects on human tendon stem cells. PloS One 9 (2), e87706. doi: 10.1371/journal.pone.0087706
Zhang, J., Wang, J. H. (2015). Moderate exercise mitigates the detrimental effects of aging on tendon stem cells. PloS One 10 (6), e0130454. doi: 10.1371/journal.pone.0130454
Zhang, K., Asai, S., Yu, B. (2015). Enomoto-Iwamoto M. IL-1 β irreversibly inhibits tenogenic differentiation and alters metabolism in injured tendon-derived progenitor cells in vitro. Biochem. Biophys. Res. Commun. 463 (4), 667–672. doi: 10.1016/j.bbrc.2015.05.122
Zhang, J., Yuan, T., Wang, J. H. (2016). Moderate treadmill running exercise prior to tendon injury enhances wound healing in aging rats. Oncotarget 7 (8), 8498–8512. doi: 10.18632/oncotarget7381
Keywords: tendon-derived stem cells, tendon stem cells, stem cell niche, tendon aging, stem cell aging
Citation: Lui PPY and Wong CM (2020) Biology of Tendon Stem Cells and Tendon in Aging. Front. Genet. 10:1338. doi: 10.3389/fgene.2019.01338
Received: 01 March 2019; Accepted: 09 December 2019;
Published: 16 January 2020.
Edited by:
Erdal Karaoz, Istinye University, TurkeyReviewed by:
Atsushi Asakura, University of Minnesota Twin Cities, United StatesHakan Darici, Istinye University, Turkey
Copyright © 2020 Lui and Wong. This is an open-access article distributed under the terms of the Creative Commons Attribution License (CC BY). The use, distribution or reproduction in other forums is permitted, provided the original author(s) and the copyright owner(s) are credited and that the original publication in this journal is cited, in accordance with accepted academic practice. No use, distribution or reproduction is permitted which does not comply with these terms.
*Correspondence: Pauline Po Yee Lui, paulinelui00@gmaiil.com