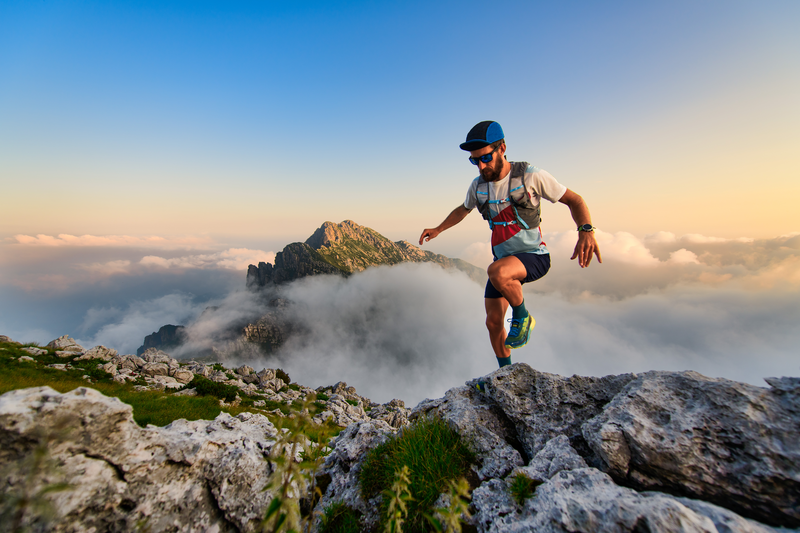
94% of researchers rate our articles as excellent or good
Learn more about the work of our research integrity team to safeguard the quality of each article we publish.
Find out more
ORIGINAL RESEARCH article
Front. Genet. , 31 October 2019
Sec. Livestock Genomics
Volume 10 - 2019 | https://doi.org/10.3389/fgene.2019.01070
This article is part of the Research Topic Integrative Genomics and Network Biology in Livestock and other Domestic Animals View all 33 articles
A better understanding of gene expression and metabolic pathways in response to a feeding system is critical for identifying key physiological processes and genes associated with polyunsaturated fatty acid (PUFA) content in lamb meat. The main objective of this study was to investigate transcriptional changes in L. thoracis (LT) muscle, liver, and subcutaneous fat (SF) of lambs that grazed alfalfa (ALF) and concentrate-fed (CON) slaughtered at 23 kg and using the Affymetrix Ovine Gene 1.1 ST whole-genome array. The study also evaluated the relationship between meat traits in LT muscle, including color, pigments and lipid oxidation during 7 days of display, α-tocopherol content, intramuscular fat (IMF) content and the fatty acid (FA) profile. Lambs that grazed on alfalfa had a greater α-tocopherol concentration in plasma than CON lambs (P < 0.05). The treatment did not affect the IMF content, meat color or pigments (P > 0.05). Grazing increased the α-tocopherol content (P < 0.001) and decreased lipid oxidation on day 7 of display (P < 0.05) in LT muscle. The ALF group contained a greater amount of conjugated linoleic acid (CLA), C18:3 n−3, C20:5 n−3, C22:5 n−3, and C22:6 n−3 than did the CON group (P < 0.05). We identified 41, 96 and four genes differentially expressed in LT muscle, liver, and subcutaneous fat, respectively. The most enriched biological processes in LT muscle were skeletal muscle tissue development, being the genes related to catabolic and lipid processes downregulated, except for CPT1B, which was upregulated in the ALF lambs. Animals grazing alfalfa had lower expression of desaturase enzymes in the liver (FADS1 and FADS2), which regulate unsaturation of fatty acids and are directly involved in the metabolism of n−3 PUFA series. The results found in the current study showed that ingesting diets richer in n−3 PUFA might have negative effects on the de novo synthesis of n−3 PUFA by downregulating the FADS1 and FADS2 expression. However, feeding diets poorer in n−3 PUFA can promote fatty acid desaturation, which makes these two genes attractive candidates for altering the content of PUFAs in meat.
Public health policies recommend an increase in the intake of the n−3 polyunsaturated fatty acid (PUFA) series due to the positive impact these molecules have on human health. In addition, a decrease in the consumption of trans-fatty acids and saturated fatty acids (SFAs) is recommended because they have been associated with increased cholesterol levels (Takeuchi and Sugano, 2017; Zhu et al., 2019). Other fatty acids, such as conjugated linoleic acids (CLAs), have also received increasing attention because of their possible beneficial effects on human health (Lehnen et al., 2015; Lee et al., 2018).
Currently, increasing feed efficiency and producing lean meat without reducing the nutritional value of the meat are major challenges of the meat industry. The nutritional value of meat can be influenced by dietary and genetic effects (Scollan et al., 2014). Grass feeding improves eicosapentaenoic acid (20:5n−3, EPA), docosapentaenoic acid (DPA, 22:5n−3), and docosahexaenoic acid (22:6n−3, DHA) contents in muscle (Fisher et al., 2000; Dervishi et al., 2010; Dervishi et al., 2011) as forage increases the content of alpha-linolenic acid (18:3n−3), the precursor for DHA and EPA production (Kitessa et al., 2010). Diet has been shown to have a major impact on the intramuscular FA profile of the muscle of light lambs (Dervishi et al., 2010; González-Calvo et al., 2015a); grazing increases the PUFA content of the n−3 series and conjugated fatty acids (CLAs) when compared to that with concentrate feeding. In semitendinosus muscle, genes related to adipogenesis are upregulated in concentrate-fed lambs, whereas CPT1B gene expression, related to the β-oxidation process, is upregulated in grazing lambs (Dervishi et al., 2011). However, the expression of genes implicated in lipid metabolism is not similar in the longissimus muscle of grazing and concentrate-fed lambs (González-Calvo et al., 2015a). These results demonstrate that the diet/feeding system has a differential effect on gene expression in different animal tissues. It has also been demonstrated that fiber type composition in skeletal muscle (the relative amounts of fast versus slow twitch fibers) affected the gene expression profiles among different muscle under the same environment (Terry et al., 2018). Therefore, a better understanding of the genes and metabolic pathways in response to the feeding system is critical for identifying key physiological processes and genes associated with lipid metabolism, especially for the n−3 PUFA series. A deeper understanding of the gene regulation of n−3 levels in lamb meat may help in designing new strategies for the production of healthier meat and satisfying consumers’ demand.
The combination of technologies such as fatty acids and gene profiling provides a powerful tool for discovering gene expression changes associated with meat quality traits and for discovering genes contributing to fatty acid content variation in meat. The main objective of this study was to investigate the fatty acid profile and transcriptional changes in the LT muscle, liver, and subcutaneous fat (SF) of lambs grazing on alfalfa pasture and receiving concentrate using the Affymetrix Ovine Gene 1.1 ST whole-genome array. Furthermore, we aimed to identify novel genes that may play important roles in the metabolism of PUFAs that may be associated with meat quality traits.
All experimental procedures, including the care of animals and euthanasia, were performed in accordance with the guidelines of the European Union and Spanish regulations for the use and care of animals in research and were approved by the Animal Welfare Committee of the Centro de Investigación y Tecnología Agroalimentaria (CITA) (protocol number 2009-01_MJT). In all cases, euthanasia was performed by penetrating captive bolt followed by immediate exsanguination.
Fourteen pairs of ewe-single reared male lambs of the Rasa Aragonesa breed grazed continuously during lactation in alfalfa pastures. The lambs had ad libitum access to a concentrate during lactation. Seven pairs of ewe-lambs were not weaned but remained grazing alfalfa with their mothers from birth until the slaughter of the lambs (23 ± 0.4 kg) (ALF group). The other seven lambs were weaned (48 ± 0.9 days of age) and then fed a basal concentrate for 24 (± 2.6) days until slaughter at 23 kg (CON group). These lambs were the same as those described in González-Calvo et al. (2017), and were reared alongside the ALF group. Lambs belonging to ALF treatment received dams’ milk, fresh alfalfa (grazing) and commercial concentrate, the same that was offered to CON treatment during the experimental period. The average concentrate intake of the CON and ALF groups during the experimental period was 24.3 and 7.4 kg per lamb, respectively. The weaning weight of CON treatment animals was 11.6 ± 1.91 kg BW and the weight of the alfalfa lambs at the same moment of the weaning of CON treatment was 12.8 ± 1.35 kg BW. The ingredients, chemical composition and FA composition of the feedstuffs are shown in Table 1. The experimental procedures, composition of diets, management of the animals and sample details for each group are described in detail in Ripoll et al. (2013). Blood samples were obtained weekly in test tubes containing heparin from the jugular vein. Samples were centrifuged at 3,500 rpm for 20 min, and plasma was stored at −80°C until α-tocopherol and triacylglycerols (TG), cholesterol, low density lipoprotein-cholesterol (LDL-cholesterol) and high density lipoprotein-cholesterol (HDL-cholesterol) analyses.
All the lambs were slaughtered when they reached 22–24 kg of slaughter weight (SW) according to the specifications of Ternasco de Aragón Protected Geographical Indication (Regulation (EC) No. 1107/96) that stipulates that lambs must be younger than 90 days old with a SW between 22 and 24 kg. The lambs were slaughtered using EU laws in the same commercial abattoir, and the carcasses were hung by the Achilles tendon and chilled for 24 h at 4°C in total darkness. The slaughter age, slaughter weight, and growth rate of the two management strategies are presented in Supplementary Table 1.
Just after slaughter, a sample of the LT muscle from the 12th thoracic vertebra, a sample of SF between the atlas and axis cervical vertebrae and a sample of the liver were excised, frozen in liquid nitrogen and stored at −80°C until RNA isolation.
The intramuscular fat content was quantified using the Ankom procedure (AOAC, 2000) with an Ankom extractor (model XT10, Ankom Technology, New York, USA).
Both muscle and feed fatty acids were determined as described in González-Calvo et al. (2015a). Feed samples were Soxhlet extracted (Sukhija and Palmquist, 1988), and muscle samples were determined according to Bligh and Dyer (1959) with the modifications described in González-Calvo et al. (2015a). The individual FA contents were expressed as weight percentages (g/100 g of FAME). The total amount of SFA, monounsaturated FA (MUFA), PUFA, n−6 PUFA and n−3 PUFA contents and their associated ratios (PUFA : SFA and n−6:n−3) were determined.
Alpha-tocopherol in plasma was determined by liquid extraction in duplicate as described in González-Calvo et al. (2015b). Triacylglycerols, cholesterol, LDL-cholesterol and HDL-cholesterol were determined using an automatic analyzer (Gernonstar, RAL, Barcelona, España). The reagent manufacturer was RAL (Técnica para el Laboratorio, S.A. Sant Joan Despí, Barcelona, Spain). The mean intra-assay coefficients of variation were 0.99–1.57%, 0.76–1.22%, 0.63–0.67%, and 0.8–1.06% for TG, cholesterol, LDL-cholesterol and HDL-cholesterol, respectively. The interassay coefficients of variation were 3.15–7.77%, 4.36–6.91%, 1.29–1.45% and 2.71–4.60% for the same metabolites.
After it was chilled, a piece of the LT muscle between the 4th and the 6th lumbar vertebrae was vacuum-packed and kept at −20°C in darkness until the α-tocopherol analysis. The α-tocopherol concentration was determined by liquid extraction as described in González-Calvo et al. (2015b). A portion of the loin between the 7th and the 13th thoracic vertebrae was used to measure the color (metmyoglobin content, MMb) and lipid oxidation analysis (thiobarbituric acid-reactive substance, TBARS), and were quantified at 7 days after being maintained in darkness at 4°C. The LT muscle color and LT intramuscular fat TBARS analysis were measured as described in González-Calvo et al. (2015b). Briefly, the relative content of metmyoglobin (MMb) was estimated by the K/S572/525 ratio (Hunt, 1980). This ratio decreases when the MMb content increases. The TBARS analysis was performed using the procedure reported by Pfalzgraf et al. (1995). The TBARS values are expressed as milligrams of malonaldehyde (MDA) kg−1 of muscle.
Total RNA was extracted from approximately 500 mg of LT muscle, SF, and liver using RNeasy Tissue mini kits (QIAGEN, Madrid, Spain) following the manufacturer’s protocol. Prior to microarray analysis, RNA integrity and quality were assessed by an RNA 6000 Nano LabChip on an Agilent 2100 Bioanalyzer and quantified using a nanophotometric spectrophotometer (Implen, Madrid, Spain). All RNA integrity number (RIN) values were above 8.
RNA samples (n = 14, seven samples from each treatment) were analyzed using the Ovine Gene 1.1 ST Array Strip (Affymetrix, High Wycombe, UK). Microarray hybridization and scanning were performed at the Functional Genomics Core Facility (Institute for Research in Biomedicine, IRB Barcelona, Spain) following the recommendations of the manufacturer. Scanned images (DAT files) were transformed into intensities (CEL files) by Affymetrix GeneChip Operating Software (GCOS). The overall array intensity was normalized between arrays to correct for systematic bias in the data and to remove the impact of nonbiological influences on biological data. The imported data were analyzed at the gene level, with exons summarized to genes, using the mean expression of all the exons of a gene. Normalization was carried out with the Robust Multi-Array Average (RMA) algorithm using quantile normalization, median polish probe summarization, and log2 probe transformation. The datasets supporting the results and discussed in this publication have been deposited in the NCBI Gene Expression Omnibus repository (Barrett et al., 2012) and are accessible through GEO Series accession numbers GSE63774 (LT muscle and SF) and GSE125661 (liver). The datasets for LT muscle and SF in CON group were previously presented in González-Calvo et al. (2017).
One microgram of RNA from each sample was treated with DNAse (Invitrogen, Carlsbad, CA, USA), and single-stranded cDNA was synthesized using the SuperScript®III Reverse Transcriptase kit (Invitrogen, Carlsbad, CA, USA), following the manufacturer’s recommendations. Specific exon-spanning primers for genes were generated and confirmed for specificity using BLAST (National Center for Biotechnology Information: http://www.ncbi.nlm.nih.gov/BLAST/). Before performing the real-time PCRs, a conventional PCR was performed for all genes to test the primers and to verify the amplified products. The PCR products were sequenced to confirm gene identity using an ABI Prism 3700 (Applied Biosystems, Madrid, Spain) with standard protocols. Homology searches were performed with BLAST to verify the identity of the amplified fragments. The real-time PCR was carried out in a 10 μl PCR total reaction mixture containing SYBR Green Master Mix: SYBR Premix Ex Taq II (Tli RNase H Plus, Takara, Sumalsa, Zaragoza, Spain). Reactions were run in triplicate on an ABI Prism 7500 platform (Applied Biosystem, Madrid, Spain) following the manufacturer’s cycling parameters. Standard curves for each gene were generated to calculate the amplification efficiency through a 4-fold serial dilution of cDNA pooled from LT muscle, liver and SF. The efficiency (E) of PCR amplification for each gene was calculated using the standard curve method (E = 10(−1/slope)). Two “connector samples” were replicated in all plates to remove technical variation from this source of variability. The annealing temperatures, primer concentrations, and primer sequences for GOIs (Genes of interest: CPT1B, MYOD1, MSTN, ABCC4, IGF1R and PLA2G16 for LT muscle; METTL1 for SF; and FADS1, FADS2, ACACA, SCD, SQLE, IER3, SLC19A1 and THRSP for liver tissue) and reference genes (GUSB and YWHAZ for LT muscle and SF; and RPL37, GUSB and RPL19 for liver) are described in Supplementary Table 2. These reference genes for LT muscle and SF were chosen because they were the most stable in these tissues in previous studies (González-Calvo et al., 2014). Five candidate reference genes (B2M, YWHAZ, RPL37, RPL19, and GUSB) were tested for liver tissue. Determinations of the gene expression stability of liver genes included in this study were calculated using NormFinder to select the best reference genes (Andersen et al., 2004).
Statistical analysis of the performance, the plasma metabolites and lipid oxidation of LT muscle (TBARS) was performed using the SAS statistical package v. 9.3 (SAS Institute, Cary NC, USA). The concentration of analytes in plasma, lipid oxidation levels and meat color and pigments were analyzed using mixed models for repeated measurements based on Kenward-Roger’s adjusted degrees of freedom solution for repeated measures including the management strategy (CON and ALF), the week/time of display and its interaction as fixed effects and the lamb as the random effect. A first-order autoregressive structure with heterogeneous variances for each date was used to model heterogeneous residual error.
The weight gain, age, weight at slaughter and IMF in LT muscle were analyzed using a general lineal model (GLM) with the treatment as a fixed factor. The content of α-tocopherol and the fatty acid profile of LT muscle were analyzed with a GLM with the treatment as a fixed factor and the slaughter age (SA) as a covariate. The results were expressed as least square means (LSM) ± the standard error (SE) values, and the differences were tested at a level of significance of 0.05 with the t statistic. The Tukey post hoc test was used to evaluate differences between treatments.
Normalized data were further analyzed using Babelomics (http://babelomics.bioinfo.cipf.es/graph.html) and MetaboAnalyst software (Xia et al., 2009). Genes showing a statistically significant value of the Limma test (P < 0.01) were screened out as differentially expressed between treatments. Significant genes were annotated based on similarity scores in blastn comparisons of Affymetrix transcript cluster sequences against ovine sequences in GenBank. A second method, significance analysis of microarray (SAM), was used to identify and reconfirm differentially expressed genes in ALF–CON comparisons. Details of the protocol are described in González-Calvo et al. (2017).
Multivariate and cluster analysis was performed using MetaboAnalyst according to Xia et al. (2009). Principal components analysis (PCA) was used to cluster the samples based on the selected gene expression profile for each tissue. Hierarchical clustering analysis for gene expression was performed using all genes and only the significant genes for each tissue. Details are described in González-Calvo et al. (2017).
The corresponding mRNA levels were measured and analyzed by their quantification cycle (Cq). The statistical methodology to analyze differences in the expression rate was carried out following the method proposed by Steibel et al. (2009). The mixed model fitted was as follows:
where yrigkm is the Cq value (transformed data taking into account E < 2) obtained from the thermocycler software for the gth gene (GOIs and reference genes) from the rth well (reactions were run in triplicate) in the kth plate corresponding to the mth animal and to the ith treatment (CON and ALF); TGgi is the fixed interaction among the ith treatment and the gth gene (T is the effect of the ith treatment, and G is the effect of the gth gene); Pk is the fixed effect of the kth plate; IMFm and SAm are the effects of intramuscular fat (only used in LT muscle tissue gene expression) and the slaughter age of the mth animal, respectively, included as covariates; Am is the random effect of the mth animal from where samples were collected (Am∼(0,σ2A)); and erigkm is the random residual. Gene-specific residual variance (heterogeneous residual) was fitted to the gene by treatment effect (erigkm∼N(0, σ2egi).
To test differences (diffGOI) in the expression rate of the target genes between treatments and to obtain fold change (FC) values from the estimated TG differences, the approach suggested in Steibel et al. (2009) was used. The significance of the diffGOI estimates was determined with the t statistic. Additionally, asymmetric 95% confidence intervals (upper and lower) were calculated for each FC value using the standard error (SE) of diffGOI.
The Database for Annotation, Visualization and Integrated Discovery (DAVID) v6.7b (Huang et al., 2008) was used to determine pathways and processes of major biological significance and importance through the Functional Annotation Cluster (FAC) tool based on the Gene Ontology (GO) annotation function. DAVID FAC analysis was performed with the gene lists obtained after SAM analysis. Medium stringency EASE score parameters were selected to indicate confident enrichment scores of functional significance and importance of the given pathways and processes investigated. An enrichment score of 1.3 was employed as the threshold for cluster significance. The ClueGO plug-in (Bindea et al. 2009) and Cytoscape program (Shannon et al., 2003) were used to group genes according to the similarity of the biological processes in which they are involved. The relationships between the n−3 PUFA series in muscle and gene expression in liver were visualized using the Metscape plugin (Karnovsky et al., 2012) in Cytoscape (Shanon et al., 2003). Thirty significant genes (out of 96) and nine (out of 13) significant compounds were mapped to KEGG IDs. The file containing the list of genes and metabolites, their fold change and P-values was loaded in Metscape to generate a compound-gene network.
No differences were found in the slaughter weight and age (SA) or average daily gain (ADG) from birth to slaughter between treatments (Supplementary Table 1). Type III tests of the fixed effects (treatment and day) on blood parameters are shown in Supplementary Table 3.
The concentrations of α-tocopherol, cholesterol, LDL-cholesterol and HDL-cholesterol in plasma were affected by the interaction between the treatment and the day (P < 0.05 to < 0.01). Meanwhile, the concentration of TG in plasma was affected only by the treatment (P < 0.01).
Grazing animals (ALF group) had a greater concentration of α-tocopherol (P < 0.0001) and similar cholesterol (P = 0.056) contents throughout the experimental period when compared to those in the CON group (Figures 1A, B). The HDL-cholesterol content was similar between treatments except on day 8 after weaning, and the ALF group had a greater content when compared to that in the CON group (0.58 ± 0.03 mmol/l vs. 0.34 ± 0.03 mmol/l; P < 0.05). Similarly, ALF lambs presented a greater concentration of TG on days 8 and 28 postweaning (0.58 ± 0.03 vs. 0.67 ± 0.05 and 0.25 ± 0.03 vs. 0.3073 ± 0.07 mmol/l; P < 0.05). The LDL-cholesterol content was greater in the ALF group only on day 0 (equivalent to the day of weaning in the CON group) when compared to that in the CON group (0.48 ± 0.05 vs. 0.18 ± 0.05 mmol/l; P < 0.05) (Figure 1).
Figure 1 Concentration of (A) α-tocopherol, (B) cholesterol, (C) HDL-cholesterol, (D) LDL-cholesterol and (E) TG during the entire experimental period compared with those in the CON group.
Intramuscular fat content, meat color and pigments were not different between treatments (Supplementary Table 4; P > 0.05); however, ALF lambs had a greater content of α-tocopherol in LT muscle when compared with that in the CON group (2.38 ± 0.17 vs. 0.48 ± 0.17; P < 0.05) (Supplementary Table 3). Lipid oxidation was affected by the interaction between the treatment and the day of display (P < 0.01). Lipid oxidation was similar in the first days of display (P > 0.05), but ALF lambs had lower oxidation than did CON lambs on day 7 of display (P < 0.05).
Regarding the fatty acid profile, LT muscle from the ALF group had a greater content of capric (C10:0) and arachidic (C20:0) acids (Table 2; P < 0.05) and tended to have a greater content of margaric acid (C17:0) and stearic acid (C18:0) (0.1 < P > 0.05). However, the total SFA content was not different between treatments (P > 0.05; Table 2).
Table 2 Effect of the treatment on the content of α-tocopherol and fatty acid (FA) composition of LT muscle in Rasa Aragonesa lambs.
The treatment did not affect palmitoleic acid (C16:1; P > 0.05), vaccenic acid (C18:1 n−7; P > 0.05) and eicosenoic acid (C20:1 n−9; P > 0.05) content but did affect the content of oleic acid (C18:1 n−9; P < 0.05) and total MUFAs, which was greater in CON than in ALF lambs (P < 0.05; Table 2). Regarding individual PUFA contents, the linoleic acid (C18:2 n−6), linolenic acid (C18:3 n−3), EPA (C20:5 n−3), docosapentaenoic acid (C22:5 n−3), and DHA (C22:6 n−3) contents were greater in the ALF group than in the CON group (P < 0.05). In addition, the LT muscle of ALF lambs had a greater n−3 PUFA content and a lower n−6:n−3 ratio (P < 0.001) when compared with those in CON lambs but did not affect the total PUFA content (P = 0.07).
Forty-one, four and 96 genes were differentially expressed in LT muscle, SF, and liver, respectively, after SAM analysis (Supplementary Figure 1). In LT muscle, 41 genes were differentially expressed with an FDR = 0.002 (Table 3), of which 32 were downregulated and nine genes were upregulated. In the liver, 96 genes were differentially expressed (Supplementary Table 5), among which four genes were upregulated and 92 genes were downregulated with ALF treatment (FDR = 0.002). The top 20 significant genes in the liver are shown in Table 4.
Table 3 Differentially expressed genes in Longissimus thoracis muscle and fold-change in ALF–CON1 contrast.
Regarding SF, when ALF treatment was compared with CON, only four genes were differentially expressed with an FDR = 0.051, and all of them were upregulated in the ALF group (Table 5).
Table 5 Significant differentially expressed genes in subcutaneous fat and fold change in ALF–CON1 contrast.
Principal component (PC) analysis of the complete set showed that the first two PCs covered 81.1% of the observed variance of the sample set in LT muscle (Figure 2A). The clusters corresponding to gene expression profiles from ALF and CON groups were clearly separated from each other. Very similar results were obtained in the liver (Figure 2B), but in SF, this separation was less clear (Figure 2C).
Figure 2 Principal components analysis (PCA) based on gene expression profile data for the (A) LT muscle (B) liver, and (C) subcutaneous fat. Principal components analysis scores plots discriminating between the LT muscle of lambs fed concentrate CON (+) and Alfalfa (triangle).
Hierarchical clustering analysis for gene expression was performed using all genes and only the significant genes for each tissue. Because only four genes were significant in SF, the results of cluster analysis are not included. The results of HCA using only the significant genes for LT muscle and liver are presented in Figure 3. The expression profile of these genes was able to cluster and correctly classify the samples within their corresponding group. The heatmap shows the presence of two different clusters in both tissues. These two clusters clearly distinguished the ALF group from the CON group, as both groups showed very different gene expression patterns. For example, in LT muscle, the genes BOLA, HSF2, CHP1, DNAJB11, CDC5L, TP53INP2, CPT1B, C8ORF4, and NMT1 were upregulated in the ALF group. Furthermore, a second cluster including the rest of the genes was found to be downregulated in the ALF group (Figure 3A). In the liver, the genes BHMT, LOC105614373 and SLC19A1 were upregulated in the ALF group, and a second cluster, including the remaining genes, was downregulated in the ALF group (Figure 3B).
Figure 3 Hierarchical clustering analysis of significant gene expression in the (A) LT muscle and (B) liver of CON lambs (1) and ALF lambs (2). Cells are colored based on the measured signal intensity. Dark brown represents high gene expression levels, blue indicates low signal intensity, and gray cells represent the intermediate level.
To gain insight into the biological processes that are regulated differentially between dietary treatments, we performed enrichment analyses using DAVID and ClueGo. The results of DAVID functional annotation clustering (FAC) revealed that the most enriched functional clusters were associated with “lipid and catabolic processes” (CPT1B, PLA2G16, SPSB1, LRTOMT, PLCD4, FBXO9, CNBP and CYP27A1) and 14 genes related to “muscle development” (ALDH2, ANK3, CPT1B, FZD7, HSF2, IGF1R, LRTOMT, MSTN, MYLK2, MYOD1, MYOZ1, NMT1, PRDM1 and RSC1) (Supplementary Table 5). All these genes were downregulated in the ALF group except CPT1B, HSF2 and NMT1, although the confident enrichment scores were less than 1.3 in both cases. The biological roles of downregulated genes in LT muscle were also visualized with ClueGO (Figure 4). The size of the nodes reflects the statistical significance of the term. The most enriched biological process was that of “skeletal muscle tissue development” with four genes, MSTN, MYLK2, MYOD1 and BCL9L.
Figure 4 The biological role of up- (green) and downregulated (red) genes in the LT muscle of ALF animals visualized with ClueGO. The size of the nodes reflects the statistical significance of the term.
The results of DAVID revealed two major gene clusters associated with “sterol biosynthesis” (EBP, MVD, HMGCR, CYP51A1, HMGCS1, NR0B2, C14ORF1, FDFT1, SQLE, DHCR7, SC5DL, DHCR24, and NSDHL), “lipid biosynthetic process” (ACACA, CYP51A1, FADS1, FADS2, SCD and SC5DL), and “cholesterol metabolic process” (EBP, MVD, HMGCR, CYP51A1, SQLE, DHCR7, HMGCS1, NR0B2, DHCR24, FDFT1, and NSDHL) (Supplementary Table 6). Similar results were obtained with ClueGo, where the most enriched biological processes were “fatty acid biosynthetic process,” “sterol metabolic process,” “cofactor metabolic processes” and “coenzyme metabolic processes” (Figure 5). These genes were all downregulated in ALF treatment.
Figure 5 The biological role of up- (green) and downregulated (red) genes in ALF animals visualized with ClueGO in the liver. The size of the nodes reflects the statistical significance of the term.
Only 4 genes were significant in SF, and no cluster was found with DAVID FAC.
The gene set selected to validate the microarray results by qPCR included the following 15 genes: CPT1B, MYOD1, MSTN, ABCC4, IGF1R and PLA2G16 for LT muscle; FADS1, FADS2, ACACA, SCD, SQLE, IER3, SLC19A1 and THRSP for liver; and METTL1 for SF. The genes were selected because they were significantly differentially expressed between groups. The expression of these genes using microarray technology and qPCR is shown in Table 6. The housekeeping genes GUSB and YWHAZ were used to normalize the results for LT muscle and SF. In the liver, five candidate reference genes were tested, and the most stable genes exhibiting the lowest expression stability value (M) were RPL37 (M = 0.182), GUSB (M = 0.226), and RPL19 (M = 0.296). The three reference genes were more stable than the GOIs. The magnitude of the fold change obtained by microarray and qPCR was slightly different in some instances, but the qPCR results demonstrated a similar trend compared with the microarray results of these genes (Table 6).
Table 6 Real-time PCR confirmation of the microarray results. Gene expression changes in LT muscle, liver and subcutaneous fat in ALF vs CON comparison, and the fold change (FC) obtained with microarray and qPCR data.
In this study, we investigated the fatty acid profile and gene expression using a microarray in the LT muscle, liver and SF of lambs fed concentrate or alfalfa. As expected, ALF animals contained greater CLAs and a greater proportion of n−3 PUFAs in muscle, such as linolenic acid (C18:3 n−3), EPA (C20:5 n−3), docosapentaenoic acid (C22:5 n−3), and DHA (C22:6 n−3), when compared with levels in the CON group. Many studies have reported the impact of grazing on the fatty acid profile in meat lambs, particularly the fatty acids of the n−3 series (Fisher et al., 2000; Dervishi et al., 2010; Vasta et al., 2012). Zhang et al. (2017) suggested that specific compounds in the diet can be transferred to the meat. In our experiment, the fatty acid composition, especially that of the n−3 series, and α-tocopherol are probably a reflection of diet composition. Suckling lambs are functionally non-ruminants, and their meat FA profile should reflect the FA profile of the suckled milk (Napolitano et al., 2002; Valvo et al., 2005). Thus, grazing dams is an advisable alternative to increase PUFAs in the suckling lamb meat because fresh pasture has a high concentration of linolenic acid (C18:3n−3), which increases the contents of vaccenic acid (C18:1t-11), conjugated linoleic acid isomers (CLA), and n−3 PUFA in milk compared with diets comprising concentrate or preserved forage (Nudda et al., 2005; Joy et al., 2012). The high value of C18:3n−3 in ALF lamb meat could be due to the C18:3n−3 provided by pasture that, as they are not yet fully weaned, is not bio-hydrogenated by rumen microbiota. Moreover, the relatively low effectiveness of milk in affecting meat fatty acid composition, could explain the slightly difference between CON and ALF in CLA and VA. Therefore, lambs that were allowed to graze resulted in a meat fatty acid profile that is richer in fatty acids of the n−3 series, mainly due to the dam’s milk that were grazing continuously during lactation in alfalfa pastures. According to Álvarez-Rodriguez et al. (2018) dietary alfalfa but not milk supply improved CLA, and n−3 PUFAs contents in lamb meat. The FA composition of ALF lambs was more related to ewe’s milk than to fresh forage (Dervishi et al., 2010). Previous studies have shown that grazing increases the PUFA content in milk, particularly linolenic acid (C18:3n−3), while concentrates modify rumen retention time of the feed, increase linoleic acid (C18:2n−6) intake, and alter biohydrogenation pathways toward lower n−3 PUFA and CLA contents, leading to lower contents of these compounds in the milk (Elgersma, 2015). In addition, these animals had greater α-tocopherol in muscle and plasma. Vitamin E is a powerful fat-soluble antioxidant that plays important roles in scavenging free radicals and neurologic function (Wang and Quinn, 2000; Traber and Atkinson, 2007). In this study, we found that lipid oxidation was lower in ALF lambs on day 7 of display when compared with the levels in CON lambs. These results are in concordance with previous studies in which we reported that the addition of vitamin E to the diet increased the α-tocopherol muscle content and drastically diminished the lipid oxidation of meat (Kasapidou et al., 2012; González-Calvo et al., 2015b; Ponnampalam et al., 2017).
Moreover, we investigated how the feeding system impacted gene expression in LT muscle, liver and SF in both treatment groups. Indeed, we found that both groups differed in their gene expression profile, mainly in LT muscle and liver, with the greatest impact in liver. It has been reported that dietary intervention can lead to major changes in gene expression in muscle and liver (Dervishi et al., 2011; Cui et al, 2018). In the ALF group, the most enriched biological processes in LT muscle were skeletal muscle tissue development (MYOD1, MYLK2 and MSTN) (Figure 4 and Supplementary Table 5). These genes were downregulated in the ALF group, with MYOD1 and MSTN being the most downregulated genes (the lowest FC). The yield of saleable meat and meat quality, and therefore the profitability for livestock operations, are greatly influenced by growth during the postnatal period. Therefore, the identification of genes that play a role in muscle growth in sheep is an important step for improving sheep meat production by selection. In this regard, in livestock species, MYOD1 and MSTN are considered candidate genes for meat quality and carcass traits (Ibeagha-Awemu et al., 2008; Bhuiyan et al., 2009). MYOD1 regulates muscle cell differentiation, growth, and development and is also involved in muscle regeneration (Kitzmann et al., 1998). For example, polymorphisms of MYOD1 have been associated with weight, several muscle fiber characteristics, the loin eye area and lightness in yak populations, pork and cattle (Chu et al., 2012; Lee et al., 2012; Du et al., 2013). In addition, low MYOD1 expression levels were related to low Warner–Bratzler shear force measured in the longissimus dorsi muscle of beef (Tizioto et al., 2014) and thus with greater tenderness. In sheep, a positive correlation between MYOD1 expression and cold carcass yield was found (Lôbo et al., 2012). The authors proposed that animals with a higher expression of MYOD1 were more efficient during postnatal growth and had a greater longissimus dorsi weight and a better cold and hot carcass yield. In our study, we did not observe differences in slaughter weight or average daily gain between the ALF and CON groups. These discrepancies may be due to different slaughter ages among both studies. We sacrificed our animals at 67–72 days, and other studies compared heavy lambs (at an average of 200 days) fed either concentrate or limited grazing (Lôbo et al., 2012), whereas in the present study, grazing lambs had free access to forage, concentrate, and dam’s milk. Despite the different results, further investigation into the role of MYOD1 in sheep carcass and meat quality traits in sheep is necessary for effective marker assisted selection. Another gene that was downregulated in the ALF group was MSTN. Myostatin is an extracellular cytokine that is mostly expressed in skeletal muscles and is known to play a crucial role in the negative regulation of muscle mass (Elkina et al., 2011). This effect is due to an increase in both muscle fiber number (hyperplasia) and mass (hypertrophy). For instance, Ji et al. (1998) demonstrated that myostatin expression in skeletal muscle peaks prenatally and that greater expression during the prenatal period is associated with low birth weight in pigs. Mutations in the myostatin gene with functional inactivation in beef cattle increase the muscle mass in the double-muscled phenotype and lead to smaller adipocytes and fewer fat islands in muscle (Cassar-Malek et al., 2007). In addition, in different cattle breeds, mutations in MSTN have been associated with significant reductions in the shear force and a decrease in total collagen content (Ngapo et al., 2002; Lines et al., 2009). Moreover, mutations in MSTN in sheep were associated with muscling and reduced intramuscular fat (Kijas et al., 2007) and an increased percentage of fast glycolytic myofibers (Laville et al., 2004). In our experiment, the ALF group showed downregulated MYOD1 and MSTN genes, which may be beneficial for increasing meat tenderness and cold carcass yield in heavier animals. However, the simultaneous downregulation of the MYOD1 and MSTN genes in ALF group might determine an opposite effect about animal’s performance, thus justifying the lack in different performance between two groups.
The results of the functional analysis showed that genes related to catabolic and lipid processes in LT muscle were downregulated (PLA2G16, SPSB1, LRTOMT, PLCD4, FBXO9, CNBP and CYP27A1), except for CPT1B, which was upregulated in the ALF group (Supplementary Table 5). Carnitine palmitoyl transferase I (M-CPT 1), codified by the CPT1B gene, is part of the mitochondrial transport system and is a key enzyme in the control of long-chain fatty acid oxidation (Bartelds et al., 2004). These results are in agreement with those previously obtained by Dervishi et al. (2011, 2012) in which grazing systems promoted higher levels of CPT1B gene expression in the semitendinosus muscle and mammary gland. As reported by Dervishi et al. (2010), concentrate feeding promotes the upregulation of genes related to adipogenesis, whereas the grazing system promotes higher levels of genes implicated in fatty acid oxidation.
The impact of feeding system was more pronounced on liver gene expression, where 96 genes were significantly changed compared to that in LT muscle (41 genes) and SF (4 genes). The major sites of fatty acid synthesis are adipose tissue and the liver. However, the results for gene expression in these three different tissues suggest that in young lambs, the major site of lipid metabolism is the liver rather than subcutaneous fat.
We attempted to link the significant fatty acids in muscle and metabolites in plasma with the results of gene expression to obtain a better understanding of the underlying metabolic processes associated with different feeding systems. The relationship between n−3 FAs in muscle and gene expression in liver mapped 30 significant genes (out of 92) and 9 significant compounds (out of 13) to KEGG IDs. A compound-gene network was generated (Figure 6 and Supplementary Figure 2). In addition, this approach helped us to identify genes related to enriched biological processes and certain desired outcomes, for example, n−3 PUFA series that are desirable regarding human health (Simopoulos, 2008; Cabo et al., 2012; Liu and Ma, 2014). Indeed, the animals grazing alfalfa had a greater content of fatty acids of the n−3 series such as linolenic acid (C18:3 n−3), EPA (C20:5 n−3), docosapentaenoic acid (C22:5 n−3), and DHA (C22:6 n−3) in LT muscle and lower expression of FADS1 and FADS2 in liver (Figure 6). It is worth mentioning that FADS1 and FADS2 in the “fatty acid biosynthetic process” cluster are key genes in the metabolism of n−3 PUFA series. The proteins encoded by these genes (FADS1 and FADS2) are members of the fatty acid desaturase (FADS) gene family. Desaturase enzymes regulate the unsaturation of fatty acids through the introduction of double bonds between defined carbons of the fatty acyl chain (Nakamura and Nara, 2004). In addition, these animals were characterized by a decrease in the expression of genes related to cholesterol metabolism (DHCR7, SC5DL, EBP, NSDHL, MTHFD1L, and CYP51A1; Supplementary Figure 2).
Figure 6 The network of metabolites and genes involved in omega-3 fatty acid metabolism in ALF lambs. Significant metabolites with experimental data are shown in green squares, and significant genes with experimental data are shown in purple circles with green borders. The size of the nodes represents the direction of the change. A small purple circle with a green border indicates downregulated genes, and large green square nodes point to upregulated compounds.
Nutrition is an important strategy to alter gene expression and the fatty acid profile of meat. It has been widely reported that grazing animals have a greater content of the n−3 PUFA series in the serum, liver and muscle and a lower n−6:n−3 ratio, in agreement with the present study. Interestingly, we also found that the expression of two genes related to n−3 PUFA metabolism was downregulated in the livers of ALF animals. Fatty acid desaturase 1 (FADS1) and 2 (FADS2) genes encode delta-5 and delta-6 desaturases, respectively, which are rate-limiting enzymes in the synthesis of polyunsaturated omega-3 and omega-6 FAs. Dietary FAs have been shown to regulate desaturase activity (Nakamura and Nara, 2004). Gene expression of both FADS1 and FADS2 is reduced by PUFAs in several hepatic models (Reardon et al., 2013; Cho et al., 1999a; Cho et al., 1999b). Furthermore, FADS1 and FADS2 gene expression was reduced by EPA and AA in 3T3‐L1 adipocytes (Ralston et al., 2015). In our study we found that ALF lambs have greater amount of EPA in their muscle mainly because of their diet. ALF lambs ingested diets rich in PUFAs (fresh alfalfa, and mainly dams’ milk), which in turn might have down-regulated FADS1 and FADS2 gene expression in liver. In support to our speculation da Costa et al. (2014) found that high levels of n−3 PUFA in cattle liver down-regulated the expression of the genes FADS1 and FADS2.
The results found in the current study showed that ingesting diets richer in n−3 PUFA might have negative effects on the de novo synthesis of n−3 PUFA by the FADS1 and FADS2 enzymes. However, feeding diets poorer in n−3 PUFA can promote fatty acid desaturation, which makes these two genes attractive candidates for altering the content of PUFAs in meat, by looking for polymorphisms that may affect the functionality and efficiency of these enzymes and alter the fatty acid profile in lamb meat. Functional SNPs can provide an additional resource as a potential genetic markers in breeding programs. In this respect, in humans, numerous studies have consistently replicated the associations between polymorphisms in the FADS1 and FADS2 genes and the PUFA concentration (Corella and Ordovas, 2012). In porcine, a polymorphism in exon 3 of the pig FADS2 has been associated with C20:4 and intramuscular fat (IMF) content (Renaville et al., 2013; Gol et al., 2018). In dairy cows, Ibeagha-Awemu et al. (2014) demonstrated positive associations between three SNPs within FADS1 and FADS2 with three milk PUFAs. Meanwhile, contradictory results have been reported in sheep. For example, a SNP in FADS2 was significantly associated with intramuscular levels of EPA (C20:5n−3) and DHA (C22:6n−3) (Malau-Aduli et al., 2011), but in a different report, no SNP within the FADS1 and FADS2 gene regions was associated with lamb muscle n−3 levels (Knight et al., 2012). Our study further points to the importance of nutritional modulation of FADS1 and FADS2 gene expression and the fatty acid profile in sheep.
Grazing lambs presented a higher content of CLA and n−3 PUFA series and showed a lower n−6/n−3 ratio, which is favorable with regard to current human health. The feeding system is the main factor affecting the fatty acid composition and gene expression in LT muscle and liver. The gene expression results in the three different tissues suggest that the major site of lipid metabolism is the liver rather than subcutaneous fat in young lambs of the Rasa Aragonesa breed. Gene expression of FADS1 and FADS2 plays an important role in the synthesis of n−3 PUFA series, which in turn makes these two genes attractive candidates to alter the content of PUFAs in meat. More studies will be necessary to elucidate the effects of the feeding system on FADS1 and FADS2 expression in other tissues of interest or to search for mutations or functional SNPs that may be used in the future as a tool to improve the fatty acid profile in lamb meat.
The datasets generated for this study can be found in NCBI Gene Expression Omnibus repository, GSE63774 (LT muscle and SF) and GSE125661 (liver).
All Experimental Procedures, Including the Care of Animals and Euthanasia, Were Performed in Accordance With the Guidelines of the European Union and Spanish Regulations for the Use and Care of Animals in Research and Were Approved by the Animal Welfare Committee of the Centro De Investigación Y Tecnología Agroalimentaria (CITA) (Protocol Number 2009-01_MJT). in All Cases, Euthanasia Was Performed by Penetrating Captive Bolt Followed by Immediate Exsanguination.
LG-C, PS, and MB performed the experiments. MJ, MS, and JC designed the research and obtained funding for this research. ED, MS, MB, and JC wrote the paper. ED, LG-C, MS, MB, MJ, RM-H, JO, and JC analyzed the data. MJ provided animals. JC had primary responsibility for the final content. All of the authors contributed to the manuscript discussion. All of the authors read and approved the final manuscript.
This study was supported by the Ministry of Economy and Competitiveness of Spain, the European Union Regional Development Funds (INIA RTA2012-080-00, INIA RZP2017-00001-00) and the Research Group Funds of Aragón Government (A14_17R).
The authors declare that the research was conducted in the absence of any commercial or financial relationships that could be construed as a potential conflict of interest.
Appreciation is expressed to the staff of CITA de Aragón for their help in data collection. Special thanks are extended to F. Molino and G. Ripoll for their laboratory assistance
The Supplementary Material for this article can be found online at: https://www.frontiersin.org/articles/10.3389/fgene.2019.01070/full#supplementary-material
Álvarez-Rodríguez, J., Ripoll, G., Lobón, S., Sanz, A., Blanco, M., Joy, M. (2018). Alfalfa but not milk in lamb’s diet improves meat fatty acid profile and α-tocopherol content. Food Res. Int. 107, 708–716. doi: 10.1016/j.foodres.2018.03.007
Andersen, C. L., Jensen, J. L., Ørntoft, T. F. (2004). Normalization of real-time quantitative reverse transcription-PCR data: a model-based variance estimation approach to identify genes suited for normalization, applied to bladder and colon cancer data sets. Cancer Res. 64, 5245–5250. doi: 10.1158/0008-5472.CAN-04-0496
Barrett, T., Wilhite, S. E., Ledoux, P., Evangelista, C., Kim, I. F., Tomashevsky, M., et al. (2012). NCBI GEO: archive for functional genomics data sets update. Nucleic Acids Res. 41, D991–D995. doi: 10.1093/nar/gks1193
Bartelds, B., Takens, J., Smid, G. B., Zammit, V. A., Prip-Buus, C., Kuipers, J. R. G., et al. (2004). Myocardial carnitine palmitoyltransferase I expression and long-chain fatty acid oxidation in fetal and newborn lambs. Am. J. Physiol. Circ. Physiol. 286, H2243–H2248. doi: 10.1152/ajpheart.00864.2003
Bhuiyan, M. S. A., Kim, N. K., Cho, Y. M., Yoon, D., Kim, K. S., Jeon, J. T., et al. (2009). Identification of SNPs in MYOD gene family and their associations with carcass traits in cattle. Livest. Sci. 126, 292–297. doi: 10.1016/J.LIVSCI.2009.05.019
Bindea, G., Mlecnik, B., Hackl, H., Charoentong, P., Tosolini, M., Kirilovsky, A., et al. (2009). ClueGO: a Cytoscape plug-in to decipher functionally grouped gene ontology and pathway annotation networks. Bioinformatics 25 (8), 1091–1093. doi: 10.1093/bioinformatics/btp101
Bligh, E. G., Dyer, W. J. (1959). A rapid method of total lipid extraction and purification. Can. J. Biochem. Physiol. 37, 911–917. doi: 10.1139/o59-099
Cassar-Malek, I., Passelaigue, F., Bernard, C., Léger, J., Hocquette, J.-F. (2007). Target genes of myostatin loss-of-function in muscles of late bovine fetuses. BMC Genomics 8, 63. doi: 10.1186/1471-2164-8-63
Cabo, J., Alonso, R., Mata, P. (2012). Omega-3 fatty acids and blood pressure. Br. J. Nutr. 107, S195–S200. doi: 10.1017/S0007114512001584
Cho, H. P., Nakamura, M., Clarke, S. D. (1999a). Cloning, expression, and fatty acid regulation of the human delta-5 desaturase. J. Biol. Chem. 274, 37335–37339. doi: 10.1074/jbc.274.52.37335
Cho, H. P., Nakamura, M. T., Clarke, S. D. (1999b). Cloning, expression, and nutritional regulation of the mammalian Delta-6 desaturase. J. Biol. Chem. 274, 471–477. doi: 10.1074/jbc.274.1.471
Chu, M., Yan, P., Liang, C. N., Guo, X., Pei, J., Ding, X. Z., et al. (2012). Association between polymorphism of MyoD1 gene and growth traits in Yak. China Anim. Husb. Vet. Med 29, 111–113.
Corella, D., Ordovás, J. M. (2012). Interactions between dietary n-3 fatty acids and genetic variants and risk of disease. Br. J. Nutr. 107 Suppl 2(0 2), S271–S283. doi: 10.1017/S0007114512001651
Cui, K., Wang, B., Ma, T., Si, B. W., Zhang, N. F., Tu, Y., et al. (2018). Effects of dietary protein restriction followed by realimentation on growth performance and liver transcriptome alterations of lamb. Sci. Reports. 8, 15185. doi: 10.1038/s41598-018-33407-w
da Costa, A. S., Bessa, R. J., Pires, V. M., Rolo, E. A., Pinto, R. M., Andrade Fontes, C. M., et al. (2014). Is hepatic lipid metabolism of beef cattle influenced by breed and dietary silage level? BMC Vet. Res. 10, 65. doi: 10.1186/1746-6148-10-65
Dervishi, E., Joy, M., Alvarez-Rodriguez, J., Serrano, M., Calvo, J. H. (2012). The forage type (grazing versus hay pasture) fed to ewes and the lamb sex affect fatty acid profile and lipogenic gene expression in the longissimus muscle of suckling lambs1. J. Anim. Sci. 90, 54–66. doi: 10.2527/jas.2011-4057
Dervishi, E., Serrano, C., Joy, M., Serrano, M., Rodellar, C., Calvo, J. H. (2010). Effect of the feeding system on the fatty acid composition, expression of the Δ9-desaturase, peroxisome proliferator-activated receptor alpha, gamma, and sterol regulatory element binding protein 1 genes in the semitendinous muscle of light lambs of the R. BMC Vet. Res. 6, 40. doi: 10.1186/1746-6148-6-40
Dervishi, E., Serrano, C., Joy, M., Serrano, M., Rodellar, C., Calvo, J. H. (2011). The effect of feeding system in the expression of genes related with fat metabolism in semitendinous muscle in sheep. Meat Sci. 89, 91–97. doi: 10.1016/j.meatsci.2011.04.003
Du, X. H., Gan, Q. F., Yuan, Z. R., Gao, X., Zhang, L. P., Gao, H. J., et al. (2013). Polymorphism of MyoD1 and Myf6 genes and associations with carcass and meat quality traits in beef cattle. Genet. Mol. Res. 12, 6708–6717. doi: 10.4238/2013.December.13.4
Elgersma, A. (2015). Grazing increases the unsaturated fatty acid concentration of milk from grass-fed cows: a review of the contributing factors, challenges and future perspectives. Eur. J. Lipid Sci. Technol. 117, 1345–1369. doi: 10.1002/ejlt.201400469
Elkina, Y., von Haehling, S., Anker, S. D., Springer, J. (2011). The role of myostatin in muscle wasting: an overview. J. Cachexia. Sarcopenia Muscle 2, 143–151. doi: 10.1007/s13539-011-0035-5
Fisher, A. V., Enser, M., Richardson, R. I., Wood, J. D., Nute, G. R., Kurt, E., et al. (2000). Fatty acid composition and eating quality of lamb types derived from four diverse breed × production systems. Meat Sci. 55, 141–147.
Gol, S., Pena, R. N., Rothschild, M. F., Tor, M., Estany, J. (2018). A polymorphism in the fatty acid desaturase-2 gene is associated with the arachidonic acid metabolism in pigs. Sci. Rep. 8. doi: 10.1038/s41598-018-32710-w
González-Calvo, L., Dervishi, E., Joy, M., Sarto, P., Martin-Hernandez, R., Serrano, M., et al. (2017). Genome-wide expression profiling in muscle and subcutaneous fat of lambs in response to the intake of concentrate supplemented with vitamin E. BMC Genomics 18, 92. doi: 10.1186/s12864-016-3405-8
González-Calvo, L., Joy, M., Alberti, C., Ripoll, G., Molino, F., Serrano, M., et al. (2014). Effect of finishing period length with α-tocopherol supplementation on the expression of vitamin E-related genes in the muscle and subcutaneous fat of light lambs. Gene 552, 225–233. doi: 10.1016/j.gene.2014.09.037
González-Calvo, L., Joy, M., Blanco, M., Dervishi, E., Molino, F., Sarto, P., et al. (2015a). Effect of vitamin E supplementation or alfalfa grazing on fatty acid composition and expression of genes related to lipid metabolism in lambs1. J. Anim. Sci. 93, 3044–3054. doi: 10.2527/jas.2014-8758
González-Calvo, L., Ripoll, G., Molino, F., Calvo, J. H., Joy, M. (2015b). The relationship between muscle α -tocopherol concentration and meat oxidation in light lambs fed vitamin E supplements prior to slaughter. J. Sci. Food Agric. 95, 103–110. doi: 10.1002/jsfa.6688
Huang, D. W., Sherman, B. T., Lempicki, R. A. (2009). Systematic and integrative analysis of large gene lists using DAVID bioinformatics resources. Nat. Protoc. 4, 44–57. doi: 10.1038/nprot.2008.211
Hunt, M. (1980). Meat color measurements. Proceedings of 33th Reciprocal Meat Conference (1980). Reciprocal Meat Conf. Proc. 33, 41–46. Avalaible at: https://meatscience.org/docs/default-source/publications-resources/rmc/1980/meat-color-measurements.pdf?sfvrsn=ae05bbb3_2. [Accessed October 18, 2019].
Ibeagha-Awemu, E. M., Akwanji, K. A., Beaudoin, F., Zhao, X. (2014). Associations between variants of FADS genes and omega-3 and omega-6 milk fatty acids of Canadian Holstein cows. BMC Genet. 15, 25. doi: 10.1186/1471-2156-15-25
Ibeagha-Awemu, E. M., Kgwatalala, P., Zhao, X. (2008). A critical analysis of production-associated DNA polymorphisms in the genes of cattle, goat, sheep, and pig. Mamm. Genome 19, 591–617. doi: 10.1007/s00335-008-9141-x
Joy, M., Ripoll, G., Molino, F., Dervishi, E., Álvarez-Rodriguez, J. (2012). Influence of the type of forage supplied to ewes in pre- and post-partum periods on the meat fatty acids of suckling lambs. Meat Sci. 90, 775–782. doi: 10.1016/J.MEATSCI.2011.11.013
Ji, S., Losinski, R. L., Cornelius, S. G., Frank, G. R., Willis, G. M., Gerrard, D. E., et al. (1998). Myostatin expression in porcine tissues: tissue specificity and developmental and postnatal regulation. Am. J. Physiol. Integr. Comp. Physiol. 275, R1265–R1273. doi: 10.1152/ajpregu.1998.275.4.R1265
Karnovsky, A., Weymouth, T., Hull, T., Tarcea, V. G., Scardoni, G., Laudanna, C., et al. (2012). Metscape 2 bioinformatics tool for the analysis and visualization of metabolomics and gene expression data. Bioinformatics 28, 373–380. doi: 10.1093/bioinformatics/btr661
Kasapidou, E., Wood, J. D., Richardson, R. I., Sinclair, L. A., Wilkinson, R. G., Enser, M. (2012). Effect of vitamin E supplementation and diet on fatty acid composition and on meat colour and lipid oxidation of lamb leg steaks displayed in modified atmosphere packs. Meat Sci. 90, 908–916. doi: 10.1016/j.meatsci.2011.11.031
Kijas, J. W., McCulloch, R., Edwards, J. E. H., Oddy, V. H., Lee, S. H., van der Werf, J. (2007). Evidence for multiple alleles effecting muscling and fatness at the Ovine GDF8 locus. BMC Genet. 8, 80. doi: 10.1186/1471-2156-8-80
Kitessa, S., Liu, S., Briegel, J., Pethick, D., Gardner, G., Ferguson, M., et al. (2010). Effects of intensive or pasture finishing in spring and linseed supplementation in autumn on the omega-3 content of lamb meat and its carcass distribution. Anim. Prod. Sci. 50, 130. doi: 10.1071/AN09095
Kitzmann, M., Carnac, G., Vandromme, M., Primig, M., Lamb, N. J., Fernandez, A. (1998). The muscle regulatory factors MyoD and myf-5 undergo distinct cell cycle-specific expression in muscle cells. J. Cell Biol. 142, 1447–1459.
Knight, M. I., Daetwyler, H. D., Hayes, B. J., Hayden, M. J., Ball, A. J., Pethick, D. W., et al. (2012). Discovery and trait association of single nucleotide polymorphisms from gene regions of influence on meat tenderness and long-chain omega-3 fatty acid content in Australian lamb. Anim. Prod. Sci. 52, 591. doi: 10.1071/AN11229
Laville, E., Bouix, J., Sayd, T., Elsen, J. M., Larzul, C., et al. (2004). Effects of a quantitative trait locus for muscle hypertrophy from Belgian Texel sheep on carcass conformation and muscularity. J. Anim. Sci. 82, 3128–3137. doi: 10.2527/2004.82113128x
Lee, E. A., Kim, J. M., Lim, K. S., Ryu, Y. C., Jeon, W. M., Hong, K. C. (2012). Effects of variation in porcine MYOD1 gene on muscle fiber characteristics, lean meat production, and meat quality traits. Meat Sci. 92, 36–43. doi: 10.1016/J.MEATSCI.2012.03.018
Lehnen, T. E., da Silva, M. R., Camacho, A., Marcadenti, A., Lehnen, A. M. (2015). A review on effects of conjugated linoleic fatty acid (CLA) upon body composition and energetic metabolism. J. Int. Soc. Sports Nutr. 12, 36. doi: 10.1186/s12970-015-0097-4
Li, K., Sinclair, A. J., Zhao, F., Li, D. (2018). Uncommon fatty acids and cardiometabolic health. Nutrients 10 (10), 1559. doi: 10.3390/nu10101559
Lines, D. S., Pitchford, W. S., Kruk, Z. A., Bottema, C. D. K. (2009). Limousin myostatin F94L variant affects semitendinosus tenderness. Meat Sci. 81, 126–131. doi: 10.1016/j.meatsci.2008.07.007
Liu, J., Ma, D. (2014). The role of n-3 polyunsaturated fatty acids in the prevention and treatment of breast cancer. Nutrients 6, 5184–5223. doi: 10.3390/nu6115184
Lôbo, A. M. B. O., Guimarães, S. E. F., Paiva, S. R., Cardoso, F. F., Silva, F. F., Júnior, G. A. F., et al. (2012). Differentially transcribed genes in skeletal muscle of lambs. Livest. Sci. 150, 31–41. doi: 10.1016/J.LIVSCI.2012.07.027
Malau-Aduli, A. E. O., Bignell, C. W., McCulloch, R., Nichols, P. D., Kijas, J. W., (2011). Genetic association of delta-six fatty acid desaturase single nucleotide polymorphic molecular marker and muscle long chain omega-3 fatty acids in Australian lamb. Proceedings of the 57th International Congress of Meat Science and Technology. pp. 141–144. Ghent, Belgium. Available at: https://isgcdata.agresearch.co.nz/ [Accessed January 25, 2019].
Nakamura, M. T., Nara, T. Y. (2004). Structure, function, and dietary regulation of Δ6, Δ5, and Δ9 desaturases. Annu. Rev. Nutr. 24, 345–376. doi: 10.1146/annurev.nutr.24.121803.063211
Napolitano, F., Cifuni, G. F., Pacelli, C., Riviezzi, A. M., Girolami, A. (2002). Effect of artificial rearing on lamb welfare and meat quality. Meat Sci. 60, 307–315.
Ngapo, T. M., Berge, P., Culioli, J., Dransfield, E., De Smet, S., Claeys, E. (2002). Perimysial collagen crosslinking and meat tenderness in Belgian Blue double-muscled cattle. Meat Sci. 61, 91–102.
Nudda, A., McGuire, M. A., Battacone, G., Pulina, G. (2005). Seasonal variation in conjugated linoleic acid and vaccenic acid in milk fat of sheep and its transfer to cheese and ricotta. J. Dairy Sci. 88, 1311–1319. doi: 10.3168/jds.S0022-0302(05)72797-1
Pfalzgraf, A., Frigg, M., Steinhart, H. (1995). Alpha-tocopherol contents and lipid oxidation in pork muscle and adipose tissue during storage. J. Agric. Food Chem. 43, 1339–1342. doi: 10.1021/jf00053a039
Ponnampalam, E. N., Plozza, T., Kerr, M. G., Linden, N., Mitchell, M., Bekhit, A. E.-D. A., et al. (2017). Interaction of diet and long ageing period on lipid oxidation and colour stability of lamb meat. Meat Sci. 129, 43–49. doi: 10.1016/j.meatsci.2017.02.008
Ralston, J. C., Matravadia, S., Gaudio, N., Holloway, G. P., Mutch, D. M. (2015). Polyunsaturated fatty acid regulation of adipocyte FADS1 and FADS2 expression and function. Obesity (Silver Spring). 23, 725–728. doi: 10.1002/oby.21035
Reardon, H. T., Hsieh, A. T., Park, W. J., Kothapalli, K. S. D., Anthony, J. C., Nathanielsz, P. W., et al. (2013). Dietary long-chain polyunsaturated fatty acids upregulate expression of FADS3 transcripts. Prostaglandins. Leukot. Essent. Fatty Acids 88, 15–19. doi: 10.1016/j.plefa.2012.02.003
Renaville, B., Prandi, A., Fan, B., Sepulcri, A., Rothschild, M. F., Piasentier, E. (2013). Candidate gene marker associations with fatty acid profiles in heavy pigs. Meat Sci. 93, 495–500. doi: 10.1016/J.MEATSCI.2012.11.019
Ripoll, G., González-Calvo, L., Molino, F., Calvo, J. H., Joy, M. (2013). Effects of finishing period length with vitamin E supplementation and alfalfa grazing on carcass color and the evolution of meat color and the lipid oxidation of light lambs. Meat Sci. 93, 906–913. doi: 10.1016/j.meatsci.2012.09.017
Scollan, N. D., Dannenberger, D., Nuernberg, K., Richardson, I., MacKintosh, S., Hocquette, J.-F., et al. (2014). Enhancing the nutritional and health value of beef lipids and their relationship with meat quality. Meat Sci. 97, 384–394. doi: 10.1016/j.meatsci.2014.02.015
Shannon, P., Markiel, A., Ozier, O., Baliga, N. S., Wang, J. T., Ramage, D., et al.. (2003). Cytoscape: a software environment for integrated models of biomolecular interaction networks. Genome Res. 13, 2498–2504. doi: 10.1101/gr.1239303
Simopoulos, A. P. (2008). The importance of the omega-6/omega-3 fatty acid ratio in cardiovascular disease and other chronic diseases. Exp. Biol. Med. 233, 674–688. doi: 10.3181/0711-MR-311
Steibel, J. P., Poletto, R., Coussens, P. M., Rosa, G. J. M. (2009). A powerful and flexible linear mixed model framework for the analysis of relative quantification RT-PCR data. Genomics 94, 146–152. doi: 10.1016/j.ygeno.2009.04.008
Sukhija, P. S., Palmquist, D. L. (1988). Rapid method for determination of total fatty acid content and composition of feedstuffs and feces. J. Agric. Food Chem. 36, 1202–1206. doi: 10.1021/jf00084a019
Takeuchi, H., Sugano, M. (2017). Industrial trans fatty acid and serum cholesterol: the allowable dietary level. J. Lipids 2017, 9751756. doi: 10.1155/2017/9751756
Terry, E. E., Zhang, X., Hoffmann, C., Hughes, L. D., Lewis, S. A., Li, J., et al. (2018). Transcriptional profiling reveals extraordinary diversity among skeletal muscle tissues. Elife 7. eLife. 7, e34613. doi: 10.7554/eLife.34613
Tizioto, P., Gasparin, G., Coutinho, L., Mourao, G., Mudadu, M., Souza, M., et al. (2014). MyoD1 expression levels affect meat tenderness in Nellore beef cattle. Proceedings of 10th World Congress of Genetics Applied to Livestock Production. pp. 601. Vancouver. Canada. Avalaible at: http://www.alice.cnptia.embrapa.br/alice/handle/doc/998063 [Accessed October 18, 2019].
Traber, M. G., Atkinson, J. (2007). Vitamin E, antioxidant and nothing more. Free Radic. Biol. Med. 43, 4–15. doi: 10.1016/j.freeradbiomed.2007.03.024
Vasta, V., Pagano, R. I., Luciano, G., Scerra, M., Caparra, P., Foti, F., et al. (2012). Effect of morning vs. afternoon grazing on intramuscular fatty acid composition in lamb. Meat Sci. 90, 93–98. doi: 10.1016/j.meatsci.2011.06.009
Valvo, M. A., Lanza, M., Bella, M., Fasone, V., Scerra, M., Biondi, L., et al. (2005). Effect of ewe feeding system (grass v. concentrate) on intramuscular fatty acids of lambs raised exclusively on maternal milk. Anim. Sci. 81, 431–436. doi: 10.1079/ASC50480431
Wang, X., Quinn, P. J. (2000). The location and function of vitamin E in membranes (review). Mol. Membr. Biol. 17, 143–156. doi: 10.1080/09687680010000311
Xia, J., Psychogios, N., Young, N., Wishart, D. S. (2009). MetaboAnalyst: a web server for metabolomic data analysis and interpretation. Nucleic Acids Res. 37, W652–W660. doi: 10.1093/nar/gkp356
Zhang, X. Q., Jin, Y. M., Badgery, W. B., Tana, (2017). Diet selection and n-3 polyunsaturated fatty acid deposition in lambs as affected by restricted time at pasture. Sci. Rep. 7, 15641. doi: 10.1038/s41598-017-15875-8
Keywords: concentrate, alfalfa, microarray, ovine, muscle, subcutaneous fat, liver, meat quality
Citation: Dervishi E, González-Calvo L, Blanco M, Joy M, Sarto P, Martin-Hernandez R, Ordovás JM, Serrano M and Calvo JH (2019) Gene Expression and Fatty Acid Profiling in Longissimus thoracis Muscle, Subcutaneous Fat, and Liver of Light Lambs in Response to Concentrate or Alfalfa Grazing. Front. Genet. 10:1070. doi: 10.3389/fgene.2019.01070
Received: 09 April 2019; Accepted: 04 October 2019;
Published: 31 October 2019.
Edited by:
Robert J. Schaefer, University of Minnesota Twin Cities, United StatesReviewed by:
Andrea Serra, University of Pisa, ItalyCopyright © 2019 Dervishi, González-Calvo, Blanco, Joy, Sarto, Martin-Hernandez, Ordovás, Serrano and Calvo. This is an open-access article distributed under the terms of the Creative Commons Attribution License (CC BY). The use, distribution or reproduction in other forums is permitted, provided the original author(s) and the copyright owner(s) are credited and that the original publication in this journal is cited, in accordance with accepted academic practice. No use, distribution or reproduction is permitted which does not comply with these terms.
*Correspondence: Jorge H. Calvo, amhjYWx2b0BhcmFnb24uZXM=
Disclaimer: All claims expressed in this article are solely those of the authors and do not necessarily represent those of their affiliated organizations, or those of the publisher, the editors and the reviewers. Any product that may be evaluated in this article or claim that may be made by its manufacturer is not guaranteed or endorsed by the publisher.
Research integrity at Frontiers
Learn more about the work of our research integrity team to safeguard the quality of each article we publish.