- 1Respiratory Department, Chinese People's Liberation Army General Hospital, Beijing, China
- 2Respiratory Department, Tianjin Municipal Corps Hospital of CAPF, Tianjin, China
- 3No. 968 Hospital of Chinese People's Liberation Army, Jinzhou, China
Long non-coding RNA (lncRNA) is important in many diseases. Some studies have shown that lncRNA affects the pathogenesis of systemic inflammation of asthma. lncRNA regulates gene transcription, protein expression, and epigenetic regulation. However, lncRNAs associated with different airway phenotypes, such as eosinophilic (Eos) and neutrophilic (Neu) asthma have not been identified. The goal of this study was to determine the differences in circulating lncRNA signatures in Eos and Neu samples. Using RNA-sequencing (RNA-seq), lncRNA expression was evaluated in peripheral whole blood samples among Eos patients, Neu patients, and healthy individuals (Control). Bioinformatic analysis was used to predict relevant biological pathways. Quantitative PCR (qPCR) was used to measure gene expression in whole blood samples, Jurkat cells, and human CD4+ T cells. Finally, a novel lncRNA, LNC_000127, was inhibited by transfection of Jurkat cells with a lentiviral vector, and the effect was examined by Human Asthma RT2 Profiler™ PCR Array and western blotting. Compared to control samples, Eos samples contained 190 unique lncRNAs and Neu samples had 166 unique lncRNAs (difference ≥2-fold). KEGG pathway annotation data and GO terms revealed that different lncRNAs are involved in different mechanisms. LNC_000127, was highly expressed in Eos samples before treatment; its expression was increased in Jurkat cells and human CD4+ T cells following stimulation with PMA/CD28. Subsequent analyses revealed that LNC_000127 functions in the Th2 inflammation pathway. The results suggest that lncRNAs are involved in different phenotypes of asthma. Whether the different phenotypes of asthma can be recognized based on these lncRNAs (as biomarkers) requires further analysis. Targeting LNC_000127 may be effective for reducing Th2 inflammation in Eos asthma.
Introduction
Background
According to the proportion of granulocytes in induced sputum, airway inflammation in asthma can be divided into four inflammatory phenotypes: eosinophilic (Eos), neutrophilic (Neu), paucigranulocytic, and mixed Eos/Neu asthma (Simpson et al., 2006). CD4+ T-helper cells can mainly be divided into four subsets, Th1/Th2/Th17/Treg, and the Th2 subset exerts critical effects on Eos asthma (Muehling et al., 2017; Shinoda et al., 2017). The key cytokines in the Th2 response include interleukin (IL)-4, IL-5, and IL-13 (Wynn, 2015). Some scholars have also attributed the incidence of asthma to a Th2/Treg imbalance (Chen et al., 2017). The driving mechanism of Neu asthma is associated with Th1/Th17 cells (Li et al., 2016; Chang et al., 2017). Gene expression analysis of Neu sputum and blood samples confirmed high expression of genes related to neutrophilic protease and α-defensins (Baines et al., 2011). Severe asthma is defined as partial or total unresponsiveness to asthma treatments and is always accompanied by an increase in eosinophil or neutrophil granulocytes (Chung et al., 2014). To improve asthma management, studies are needed to evaluate disease heterogeneity and develop tools for phenotype recognition to guide individualized treatment methods.
Conceptually, lncRNAs are transcripts longer than 200 bases that do not have coding potential and thus do not produce functional proteins (Zhang et al., 2009; Song et al., 2012). The use of next-generation sequencing of RNA (RNA-Seq) has demonstrated that the number of lncRNAs is much greater than the number of genes that encode proteins. However, recent studies suggested that a few lncRNAs with short open reading frames code for peptides with biological functions (Anderson et al., 2015; Matsumoto et al., 2017). The human genome contains ~51,382 lncRNA genes (Volders et al., 2015). LncRNAs may interact with other molecules, such as RNA (Du et al., 2016; Hu et al., 2016), DNA (Beckedorff et al., 2013; Villegas and Zaphiropoulos, 2015), proteins (Kotake et al., 2011; Warburton and Boone, 2017), and metal ions (Nelson et al., 2016). LncRNAs show great diversity in the human genome (Quinn and Chang, 2016). According to their genomic locations and manner of expression, lncRNAs can be categorized as intergenic, intronic, bidirectional, enhancer, sense, or antisense (Han et al., 2014; Xie et al., 2014). Dysregulation of lncRNA can lead to numerous diseases, including cancer, cardiovascular disease, diabetes, and some other complex disorders (Reddy et al., 2014; Wang et al., 2015; Shan et al., 2017). Recent studies have highlighted the emerging role of lncRNA in asthma (Perry et al., 2014). lncRNAs show differential expression in T cell development and differentiation (Hu et al., 2013; Kanduri et al., 2015) and lncRNA functions in regulating the development of Treg cells and dendritic cells (Qiao et al., 2013; Wang et al., 2014) or activated CD4+ T-cells (Xia et al., 2014). It is unclear whether a relationship exists between lncRNA and different asthma phenotypes.
Objective
We evaluated the differences in circulating lncRNA signatures in Eos and Neu samples to determine whether lncRNAs are involved in Eos or Neu inflammation. Clustering analysis of differentially expressed genes (DEGs) was used for Eos vs. Neu samples. We characterized lncRNAs and predicted their functions by Gene Ontology (GO) analysis and Kyoto Encyclopedia of Genes and Genomes (KEGG) analysis using the mRNA-lncRNA co-expression network. In subsequent studies, we will examine the function of a novel lncRNA, LNC_000127, in the Th2 inflammation pathway.
Methods
Participants
Patients with onset Eos asthma (n = 12) and onset Neu asthma (n = 6) were selected according to the accepted standard (Eos asthma: induced sputum eosinophil count >3% and neutrophils <63%; Neu asthma: induced sputum eosinophil count <3% and neutrophils >63%) (Simpson et al., 2006). Exclusion criteria included recent (within the past weeks) respiratory tract infection, current smoking (or a history of smoking, within 6 months of cessation), and changes in maintenance therapy. All participants were selected from the People's Liberation Army General Hospital, and all participants provided informed consent before participating in the study. Healthy individuals were selected as control samples (n = 6). Clinical details for individual samples are shown in Table 1. The Ethics Committee of the People's Liberation Army General Hospital approved this study (Permit Number: S2016-089-01). Both informed and written consent was obtained from the participants.
Acquisition and Processing of Sputum Induction
Hypertonic saline (3% or 4.5%) was used for sputum induction. In the respiratory laboratory, each participant is subjected to a fixed sputum induction time (15 min). Selected sputum (sputum portion separated from saliva) was dispersed using dithiothreitol for inflammatory cell counts. The suspension was filtered, and then cell viability and the total cell count of leukocytes were determined.
Whole Genome Gene Expression Analysis (RNA Isolation, Library Preparation, Sequencing, and Data Analysis)
Four milliliters of peripheral whole blood were collected by venipuncture at asthma onset. Two milliliters were used for RNA-seq analysis. Total cellular RNA was isolated from peripheral blood samples with TRIzol reagent (Invitrogen, Carlsbad, CA, USA) and stored at −80°C. RNA was isolated using the RNeasy mini extraction kit (#A419, Qiagen, Hilden, Germany) according to the manufacturer's instructions. RNA purity was checked using the NanoPhotometer® spectrophotometer (Implen, München, Germany). RNA concentration was measured using a Qubit® RNA Assay Kit in a Qubit® 2.0 Fluorometer (Life Technologies, Carlsbad, CA, USA). RNA integrity was assessed using the RNA Nano 6000 Assay Kit of the Bioanalyzer 2100 system (Agilent Technologies, Santa Clara, CA, USA). A total of 3 μg of RNA per sample was used as input material for the RNA sample preparations. The Illumina Hiseq 2500 platform (San Diego, CA, USA) was used to measure gene expression (see the Supplementary Text 1 for specific methods of RNA-seq). We treated the peripheral blood with TRIzol reagent without removing red blood cells. Because the nucleus of red blood cells is degraded, it contains no RNA. Therefore, the RNA of peripheral blood mainly originates from leukocyte. To reduce statistical errors, we used the leukocyte counts to adjust for expression differences. The level of RNA does not reflect changes in cell proportions, but one of the purposes of this study was to identify biomarkers in different asthma phenotypes. Peripheral whole blood is easy to obtain and store and is excellent for biomarker development. So differentially expressed mRNAs and lncRNAs in leukocytes were compared between Eos and Neu samples, and cut-off points of 0.5-fold for downregulation and 2-fold for upregulation of lncRNA expression were used. Student's t-test was used for gene expression analysis and a p-value < 0.05 was considered as statistically significant.
GO and KEGG Enrichment Analysis
We conducted trans and cis analysis to evaluate the correlation between lncRNAs and mRNA expression. Cis role (co-location) of target gene prediction: The cis role is exerted when lncRNA acts on neighboring target genes. We searched for coding genes 10/100 k upstream and downstream of lncRNA and then analyzed their functions. Trans role (co-expression) of target gene prediction: The trans role occurs when lncRNAs identify each other based on expression levels. In terms of the modes of function, both cis-and trans-regulatory activities have been described previously (Mercer and Mattick, 2013). As cis-regulators, lncRNAs exert their functions on neighboring genes on the same allele from which they are transcribed, displaying an allele-specific correlation in expression and perturbation. GO and KEGG analysis is conducted using mRNA sequences. Through cis and trans studies, we identified lncRNAs suitable for analysis. In fact, both GO analysis and KEGG analysis can suggest the mechanism in which an RNA is involved.
To predict the ontology of differentially expressed lncRNA, GO enrichment analysis was conducted using the GOseq R package. GO terms were considered significantly enriched for DEGs if the corrected p-value was <0.05.
KEGG (http://www.genome.jp/kegg/) is a database resource for understanding processes in biological systems and high-level functions at the organism, cell, and ecosystem levels. By using molecular datasets generated by RNA-seq and the network between lncRNA and mRNA, we used KOBAS software to analyze the possible functions of differentially expressed lncRNA in the pathways.
Cell Isolation, Culture, and Stimulation
1. Primary human CD4+ T cells were isolated from buffy coats of healthy donors using MACS negative CD4+ purification technology (Miltenyi Biotech, Bergisch Gladbach, Germany), yielding an overall 95% pure CD4+ T cell population.
2. The Jurkat cell line was obtained from the respiratory laboratory of the People's Liberation Army General Hospital.
3. Human CD4+ T and Jurkat cells were cultured in RP1640 (Solarbio, Beijing, China, Cat: 31800-500) supplemented with 10% fetal bovine serum. Cells were cultured at concentrations of 1–2 × 105 cells/mL at 37°C/5% CO2.
4. Human CD4+ T and Jurkat cells were stimulated for 8–24 h with a combination of anti-CD3 (1 μg/mL, BioLegend, San Diego, CA, USA)/phorbol 12-myristate 13-acetate (PMA) (10 ng/mL, Lot #SLBT8132, Sigma, St. Louis, MO, USA) or anti-CD28 (1 μg/mL, pericluster CD28, Cat: 302902, BioLegend)/PMA.
Generation of Stable Jurkat Cell Lines With LNC_000127 Knockdown
To generate a construct expressing LNC_000127 short hairpin RNA (shRNA) and LNC_000127 shRNA, the targeting sequences were cloned into the pLKD-CMV-EGFP-2A-puro-U6-shRNA vector. The resulting construct was named as pLKD-CMV-G&PR-U6-shRNA. A Jurkat cell line with stably knocked down LNC_000127 was generated by transfecting the Jurkat cells with pLKD-CMV-G&PR-U6-shRNA, followed by selection culture in puromycin (2 μg/mL, Sigma) for 2 weeks (knockdown group, KD). As controls, the Jurkat cell line was transfected with the empty vector pLKD-CMV- EGFP-2A-puro-U6-shRNA and stable lines were established as described above (normal control group, NC/CV). The lentivirus was supported by Obio Technology (Shanghai) Corp., Ltd. (Shanghai, China) and the procedures were conducted using standard protocols.
Lentivirus Transduction
Jurkat cells were seeded into 48-well plates at 1 × 105/well in 250 μL medium and infected by adding the proper lentivirus according to the protocol. After 24 h of transduction, fresh medium was added, and the cells were cultured for another 2 days before treatment.
Quantitative Real-Time PCR
Confirmation of lncRNA Expression in Blood at Asthma Onset
By using TRIzol reagent (Invitrogen), total cellular RNA was isolated from peripheral whole blood samples. cDNA was synthesized with the Takara PrimeScript™ RT Master Mix Kit (Shiga, Japan). Using KAPA SYBR Fast Universal (Kapa Biosystems, Wilmington, MA, USA), we performed qPCR to evaluate lncRNA expression. The ABI Prism 7500 sequence detection system was used to perform qPCR (Applied Biosystems, Foster City, CA, USA). Briefly, the reactions were performed in a mixture (20 μL) containing 8 μL H2O, 10 μL 2X SYBR-Green PCRMix (Kapa), 1 μL cDNA template, and 0.5 μL each of sense and antisense primers. A total of 3 lncRNAs were tested. Primer sequences are listed in Table 2. We used GAPDH as a housekeeping gene for normalization. The relative changes in lncRNA expression were calculated using the 2−ΔΔCt method. When the Eos asthma patient was cured, another 2 mL of blood was collected for verification of specific genes by RT-PCR. Statistical analysis was conducted as described above.
Confirmation of Gene Expression in Jurkat Cells
Total RNA was isolated from Jurkat cells by using TRIzol reagent as described above. Before and after the stimulation with PMA/CD28, three lncRNAs were examined by qPCR. Statistical analysis was conducted as described above.
Confirmation of lncRNA Expression in Human CD4+ T Cells
This process was conducted as described above. Before and after stimulation with PMA/CD28 and PMA/CD3, LNC_000127, GATA3 and INF-r were examined by qPCR. Primer sequences are listed in Table 2. Statistical analysis was conducted as described above.
Human Asthma RT2 Profiler™ PCR Array
By using TRIzol reagent, total cellular RNA was isolated from Jurkat stable cell strains KD and NC/CV (see the Supplementary Text 2 for specific steps used in this section).
Western Blot Analysis
In addition to NC/CV cells, two other stable cell strains, KD and NC/CV, were collected after stimulation with PMA/CD28 for 8 h. Total cellular proteins were extracted according to standard procedures and the protein concentration was determined using Bradford reagent (Bio-Rad, Hercules, CA, USA). The isolated proteins were separated by SDS-PAGE and electrotransferred to polyvinyl difluoride membranes. Non-specific binding was blocked by incubating the membrane in 0.1% of Tween-20 in phosphate-buffered saline and 5% of dried milk for 1 h at 20–25°C. First, antibodies against GATA3, CD40L, CCR8, STAT5A, and CRLF2 were added and incubation was carried out overnight at 4°C. Next, the appropriate peroxidase-conjugated secondary antibody was added and immunoreactive bands were detected by chemiluminescence. Band intensities were quantified with ImageQuant software. The relative abundance of the above genes was calculated as the ratio of the normalized densitometric values between three samples. Intergroup differences in densitometry data were calculated by the Mann-Whitney U test using SPSS version 15.0 software (SPSS, Inc., Chicago, IL, USA). A p-value <0.05 was considered to indicate a statistically significant difference.
Statistical Analysis
SPSS v11.6 was used for statistical analyses. All data are expressed as the mean ± SD. Differences between groups were assessed by one-way analysis of variance (ANOVA) and the Student-Newman-Keuls multiple comparison post-test or Student's t-test.
Results
The clinic characteristics of the study samples (Eos n = 12, Neu n = 6, and control n = 6) are presented in Table 1. Compared to control samples, both the Eos and Neu samples showed lower forced expiratory volumes in the first second (FEV1%) and forced expiratory volumes in the first second/forced vital capacity (FeV1/FVC%). Eos samples showed higher fractional exhaled nitric oxide (FeNO) and induced sputum and blood eosinophil numbers; particularly, heredity values were higher than in the control and Neu samples. Neu samples showed higher induced sputum and blood neutrophil numbers. The ratio of smoking history in Neu samples was higher than in Eos samples, although the difference was not significant.
DGE Analysis of mRNA and lncRNA
We analyzed the transcriptome of peripheral whole blood. The expression patterns differed significantly between asthma samples and control samples. Using 2-fold and 0.5-fold expression differences as cutoffs, a total of 295 lncRNA transcripts were specifically dysregulated (117 lncRNA transcripts upregulated and 178 lncRNA transcripts downregulated; each p < 0.05) in asthma samples compared to in control samples (Figures 1A,B). Additionally, a total of 3,192 mRNAs were specifically dysregulated, including 1,364 mRNAs upregulated and 1,828 mRNAs downregulated in asthma samples (Figures 1C,D).
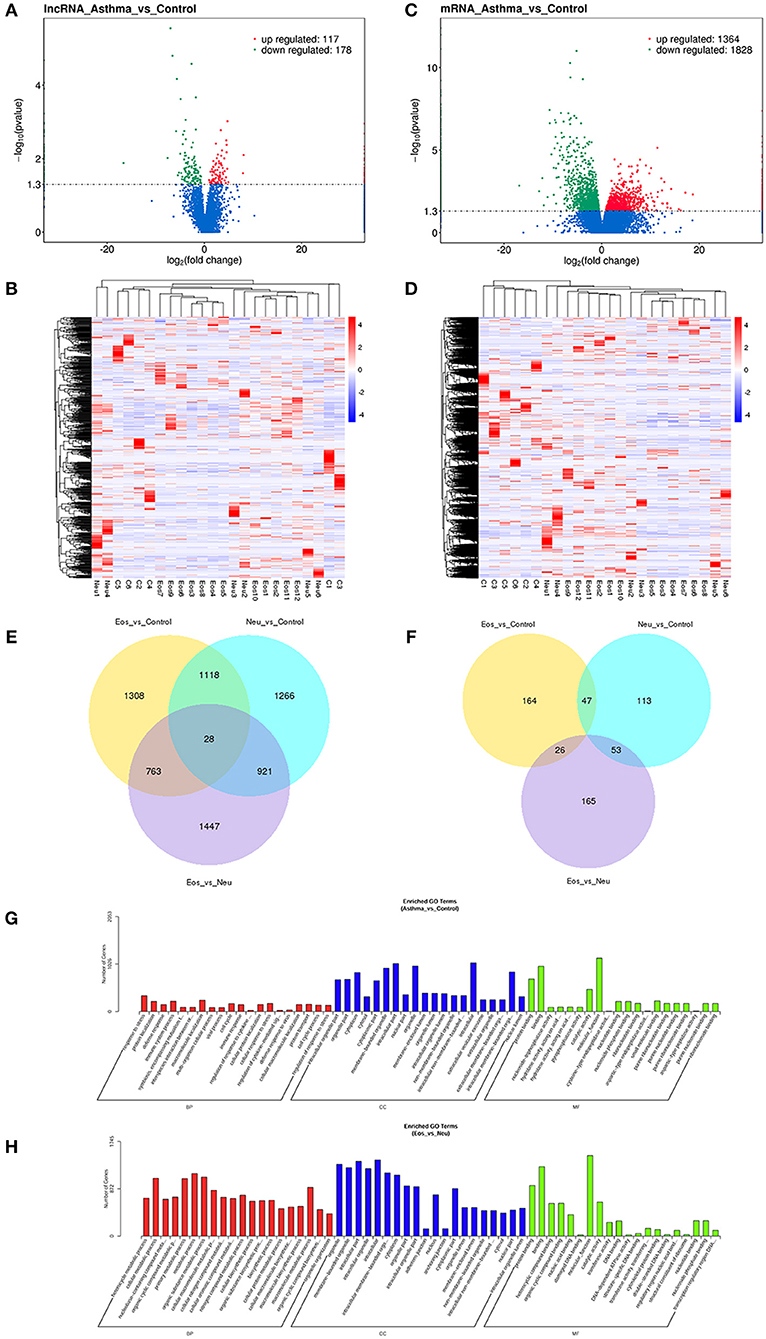
Figure 1. (A) Volcano plot assessment of lncRNA expression between asthma and control groups. Red spots indicate a >2.0-fold change in lncRNA expression between asthma and control groups. Green spots indicate a <0.5-fold change between asthma and control groups. (B) Heat map analysis of differentially expressed lncRNAs between asthma and control group. Blue indicates low lncRNA expression and red indicates high lncRNA expression. (C) Volcano plot assessment of mRNA expression between asthma and control groups. Red spots indicate a >2.0-fold change in mRNA expression between asthma and control groups. Green spots indicate a <0.5-fold change between asthma and control groups. (D) Heat map analysis of differentially expressed mRNAs between asthma and control group. Blue indicates low lncRNA expression and red indicates high lncRNA expression. (E) Venn diagram showing differential expression of mRNAs between asthma and control groups. (F) Venn diagram showing differential expression of lncRNA between asthma and control groups. (G) Gene Ontology (GO) analysis of differentially expressed lncRNAs between asthma and control groups. The most enriched GO terms targeted by dysregulated transcripts were involved in a variety of functions, such as immune response, immune system process, cellular response to stress, and response to stress. (H) Gene Ontology (GO) analysis of differentially expressed lncRNAs between Eos and Neu groups. The enriched GO term targeted by dysregulated transcripts was involved in multiple metabolic processes.
We then performed differential expression analysis for all pair-wise comparisons: Eos vs. control samples, Neu vs. control samples, and Eos vs. Neu samples. In addition to the differences observed in gene expression between asthma and control samples, the Eos and Neu samples showed distinct gene expression profiles and clustered separately.
With respect to mRNA, a total of 6,851 mRNAs were significantly differentially expressed (p-value ≤ 0.05). Venn diagrams illustrating the overlap between the two differential expression analyses results are shown in Figure 1E. Each two sample sets showed distinct mRNA expression profiling patterns (see Supplementary Tables 1–4). Eos asthma is known to be associated with the Th2 pathway and IL4, IL5, and IL13 are involved in “Th2-high” asthma. In the present study, as shown in Supplementary Table 4 (mRNA_Eos_vs_Neu), some genes, such as IL4R (receptor of IL4, IL13) and IL5R were significantly upregulated in Eos samples.
Similarly, a total of 568 lncRNAs were differentially expressed (p-value ≤ 0.05). Venn diagrams illustrating the overlap between the two differential expression analyses results are shown in Figure 1F. Compared to control samples, Eos samples contained 190 unique lncRNAs and Neu samples had 166 unique lncRNAs (difference ≥2-fold). Each two sample sets showed distinct lncRNA expression profile patterns (see Supplementary Tables 5–8).
GO term and KEGG Pathway Analysis of lncRNAs Differing Between Asthma and Control Samples
A comparison of asthma with control samples using GO analysis showed that upregulated and downregulated transcripts were involved in immune response, immune system process, cellular response to stress, and response to stress (Figure 1G). The most significant molecular function enrichment scores are also shown in Figure 1G. KEGG pathway annotation indicated that the most enriched pathways were measles, JAK-STAT signaling pathway, TNF signaling pathway, cytokine-cytokine receptor interaction (see Supplementary Table 9). As one of the most important pathways, the T cell receptor signaling pathway is listed in Figure 2.
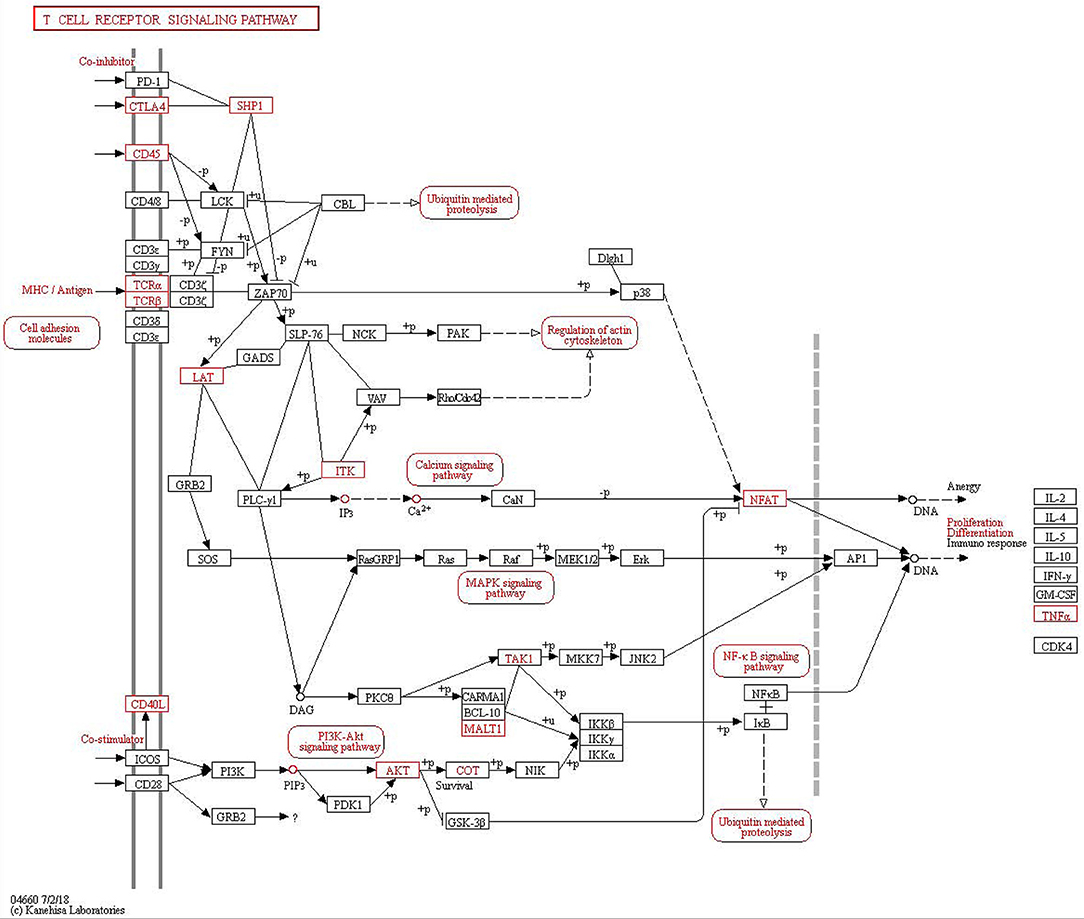
Figure 2. Kyoto Encyclopedia of Genes and Genomes (KEGG) pathway analysis of T cell receptor signaling pathway in asthma.
GO Term and KEGG Pathway Analysis of lncRNAs Differing Between Eos and Neu Samples
Comparison of Eos with Neu samples using GO analysis showed that upregulated and downregulated transcripts were involved in multiple metabolic processes (Figure 1H). KEGG pathway annotation indicated that the most enriched pathways were chemokine signaling pathway, B cell receptor signaling pathway, cytokine-cytokine receptor interaction, and tumor necrosis factor (TNF) signaling pathway (see Supplementary Table 10).
Prediction of lncRNA Target Genes Associated With Eos Asthma
To determine the association of lncRNAs and directly regulated expression of target mRNAs, we carried out lncRNA target predictions depending on the mRNA-lncRNA correlation network. Pearson's correlation analysis was performed using a coefficient ≤0.95 to construct the network. We choose several genes associated with Eos asthma, including Il5RA, GATA3, SATAT5, and SOCS, to identify co-expressed lncRNAs. Among the dysregulated lncRNAs, 3 lncRNAs (RP11-408H1.3, LNC_000127, OIP5-AS1) were found to be co-expressed with the above genes.
Confirmation of Dysregulated lncRNAs in Asthma vs. Control Samples
To confirm the sequencing gene data, we further analyzed the above 3 dysregulated lncRNAs by qPCR. One lncRNA, LNC_000127, was identified because its expression showed the highest conformance and stability, which agreed with the sequencing results (Figure 3A). LNC_000127 showed significant differences in expression between the three groups in vivo and was upregulated in the Eos group. When the Eos asthma patient was cured, 2 mL of peripheral whole blood was collected for LNC_000127 verification in vivo. LNC_000127 expression showed a decreasing trend (p < 0.05) after treatment (Figure 3B).
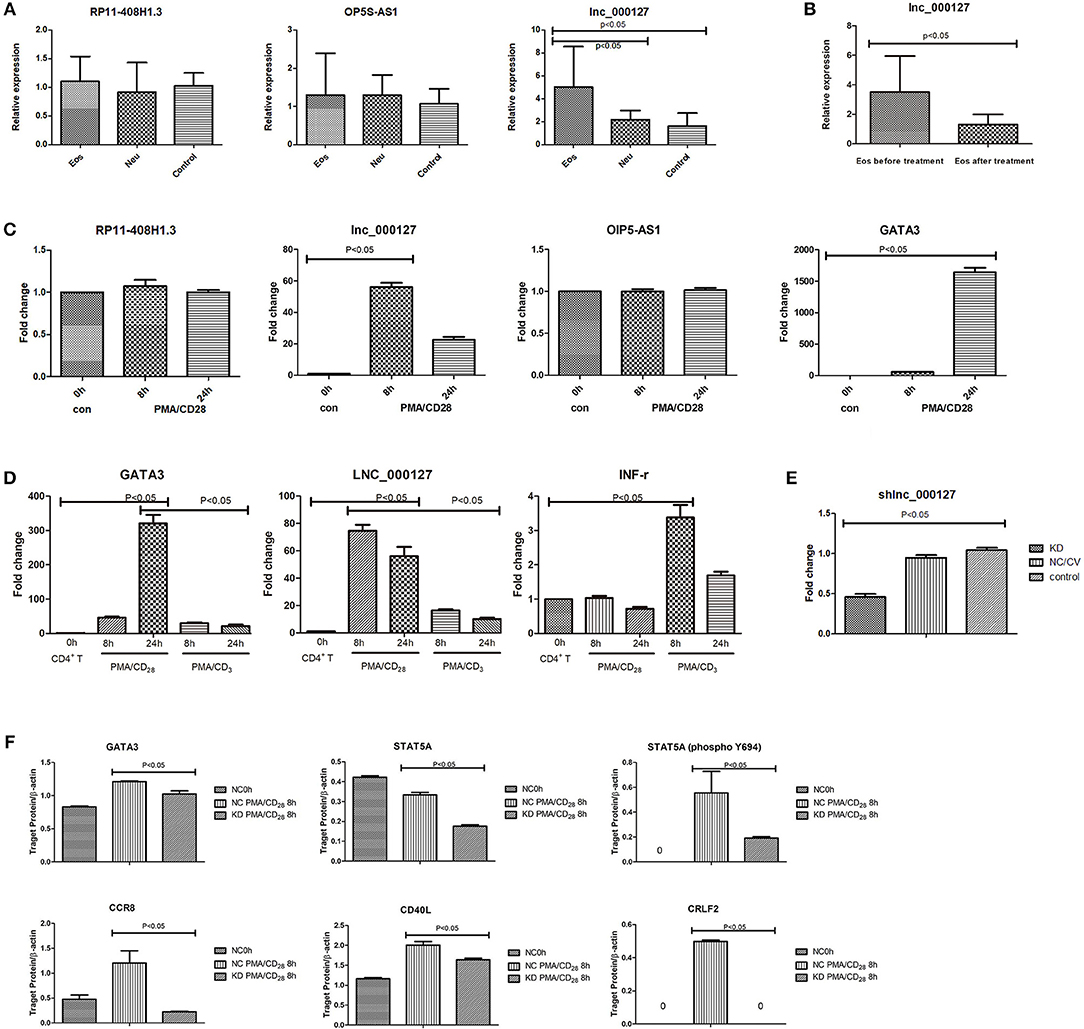
Figure 3. (A) Three selected lncRNA expression levels were validated in vivo. The expression of the selected three lncRNAs was validated by qPCR in three groups in vivo. LNC_000127 was upregulated in Eos samples (p < 0.05). (B) Before and after treatment, the expression of LNC_000127 was validated by qPCR in Eos samples in vivo. LNC_000127 was downregulated after treatment in Eos samples (p < 0.05). (C) lncRNA expression in response to PMA/CD28 stimulation. Jurkat cells were treated without (control) or with 10 ng/mL PMA, and 1 μg/mL CD28 for 8 and 24 h. Levels of selected lncRNAs and GATA3 were determined by qPCR, using the housekeeping gene GAPDH as a reference. Data are shown as the mean ± SD (n = 3) and are representative of one of three independent experiments. p < 0.05 compared to the control group, as analyzed by one-way ANOVA and post-hoc Bonferroni test. (D) Human CD4+ T cells were divided into three groups. One group was treated with 10 ng/mL PMA, and 1 μg/mL CD28 for 8 and 24 h. One group was treated with 10 ng/mL PMA, and 1 μg/mL CD3 for 8 and 24 h. The last group was used as a control. Gene expression results were validated by qPCR. Levels of selected genes (GATA3, LNC_000127, and INF-r) were determined by real-time PCR using the housekeeping gene GAPDH as a reference. Data are shown as the mean ± SD (n = 3) and are representative of one of three independent experiments. A p-value <0.05 compared to the control group, as analyzed by one-way ANOVA and post-hoc Bonferroni test. (E) Expression of LNC_000127 was validated by qPCR. Data are shown as the mean ± SD (n = 3) and are representative of one of three independent experiments. p-Value <0.05 compared to the control group, as analyzed by one-way ANOVA and post-hoc Bonferroni test. (F) Expression of the genes was validated by western blotting. Gene expression in response to PMA/CD28 stimulation. Jurkat cells were treated without (control) or with 10 ng/mL PMA, and 1 μg/mL CD28 for 8 h. β-Actin was used as a reference. The relative abundance of genes was calculated as the ratio of the normalized densitometric values between three samples. Intergroup differences (of the densitometry data) were calculated by the Mann-Whitney U test using SPSS version 11.6 software. p-Value <0.05 was considered to indicate a statistically significant difference.
LNC_000127 Showed Altered Expression in Response to PMA/CD28 Stimulation
Eos asthma is closely related to the Th2 pathway and Neu asthma is correlated with the Th1 pathway. Translation toward primary purified human CD4+ T cells revealed that PMA/CD3 stimulation induced a more pronounced Th1-specific Interferon Gamma(INF-γ), whereas PMA/CD28 induced Th2-specific GATA3 production (Smeets et al., 2012). Differential stimulation observed in the Jurkat assay is applicable in the primary human cellular assay and depends on the same proximal signaling hubs (Smeets et al., 2012). Thus, we used Jurkat cells in this study. Of the above 3 lncRNAs, LNC_000127 showed the greatest increase in PMA/CD28-treated Jurkat cells. LNC_000127 was upregulated at 8 h after PMA/CD28 stimulation. Twenty-four hours later, a decreasing trend was observed. Additionally, the expression of GATA3 was already increased at 8 h PMA/CD28 treatment (Figure 3C). Human CD4+ T cells were used to validate the effects of specific genes after stimulation by PMA/CD28 and PMA/CD3. PMA/CD3 appeared to be most powerful stimulus inducing IFN-r, whereas PMA/CD28 induced GATA3 and LNC_000127 production. These data suggest that LNC_000127 affects the Th2 pathway rather than the Th1 pathway (Figure 3D). Therefore, we further examined the function of LNC_000127.
Characterization of LNC_000127 Expression
1. Full-length LNC_000127 is ~1,561 nucleotides in length (sequence shown in Supplementary Text 3). LNC_000127 is located ~8,000 base pairs upstream of the known sequence of GBP6 (NCBI ENST00000370456) and is a novel lncRNA.
2. We determined the subcellular localization of LNC_000127. To do this, fluorescence in situ hybridization was conducted in Jurkat cells. We found that most LNC_000127 was present in the nuclei (Supplementary Image 1). These data suggest that LNC_000127 exerts its functions in the nucleus.
3. Through the experiments described above, we found that LNC_000127 expression was increased in the Th2 pathway in CD4+ T cells and Jurkat cells. Peripheral blood detection before and after treatment of Eos patients showed that LNC_000127 expression was decreased (p < 0.05). This suggests that LNC_000127 can be used as a biomarker for the onset and prognosis of patients. Studies with very large sample sizes are required to verify this result.
LNC_000127 Regulates PMA/CD28-Induced Inflammatory Response
To determine the role of LNC_000127 in the PMA/CD28-induced inflammatory response, we used a knockdown strategy in Jurkat cells using a lentiviral system (KD group). As controls, the Jurkat cell line was transfected with empty vector (NC/CV group). To exclude the possibility that transient transfection had non-specific effects on cellular responses, we generated a cell line with stable knockdown of LNC_000127 and cell line with empty vector (Supplementary Image 2). As shown in Figure 3E, the level of LNC_000127 was downregulated in the KD group, indicating that the knockdown strategy was successful.
To determine the mechanisms by which LNC_000127 regulates the PMA/CD28-induced inflammatory response, the Human Asthma RT2 Profiler™ PCR Array was used. A total of 84 gene related to asthma were compared between the KD and NC/CV groups. Thirty-four genes were differentially expressed between the KD and NC/CV groups by ≥2-fold. A total of 20 genes were upregulated and 14 were downregulated (see Supplementary Table 11). The main dysregulated genes were interleukins, chemokines, and T cell surface receptors.
The expression of some genes (GATA3, CD40L, CCR8, STAT5A, and CRLF2) after stimulation by PMA/CD28 was significantly decreased in the KD group according to our PCR array analysis. Decreased protein expression was confirmed by western blot analysis (Figure 3F and Supplementary Image 3). The results indicate that the average levels of genes (GATA3, CD40L, CCR8, STAT5A, and CRLF2) in the NC/CV group were significantly higher than those in the KD group (p < 0.05).
Discussion
lncRNAs can be categorized as intergenic, intronic, bidirectional, enhancer, sense, or antisense. Combining the epigenetic function with the variability, plasticity, and tissue specificity, lncRNAs are regarded as key regulators of disease. No studies have examined lncRNA expression in eosinophilic and neutrophilic asthma. In this study, compared to in control samples, Eos samples contained 190 unique lncRNAs and Neu samples contained 166 unique lncRNAs (difference ≥2-fold). Some of these differentially expressed lncRNAs may be involved in the chemokine signaling pathway, B cell receptor signaling pathway, cytokine-cytokine receptor interaction, and TNF signaling pathway. We demonstrated that LNC_000127 was upregulated in Eos samples by qPCR and was downregulated after treatment. LNC_000127, which is located upstream of the GBP6 transcript, may participate in and contribute to eosinophilic asthma.
In the present study, we found that the expression of LNC_000127 was increased in response to PMA/CD28 stimulation in CD4+ T cells and Jurkat cells. This suggests that LNC_000127 is involved in regulated the Th2 inflammatory response. To characterize the role of LNC_000127 in this process, we used a knockdown strategy in Jurkat cells using a lentiviral system. GATA3 and STAT5A can be induced by PMA/CD28, whereas knockdown of LNC_000127 resulted in significant inhibition of both GATA3 and STAT5A. In response to PMA/CD28, we found that knockdown of LNC_000127 decreased the expression of CCR8, CRLF2, and CD40L (Th2 inflammatory receptors). This suggests that LNC_000127 is a positive regulator of PMA/CD28-induced Th2 inflammation. Such findings in Jurkat cells are particularly important because a dysregulated immune response in T cell is closely associated with the development of Eos asthma.
lncRNAs have been reported to exhibit distinct profiles in immune processes. Airway smooth muscle cells contribute to asthma via airway remodeling. The lncRNA BCYRN1 similarly increased airway smooth muscle proliferation and migration in an asthma model (Zhang et al., 2016). Another study showed that expression of the lncRNA MEG3 was reduced in circulating CD8+ T cells in patients with severe asthma, in addition to 18 other lncRNAs (Tsitsiou et al., 2012). For glucocorticoid-resistant asthma, lncRNA GAS5 was found to function as a glucocorticoid receptor (Kino et al., 2010). Pro-inflammatory mediators up-regulate GAS5 levels in both smooth muscle cells and the airway epithelia (Keenan et al., 2015). An in vivo study supported the role of lncRNA in therapy-resistant asthma. The expression of NEAT1 and PINT (AC058791.2) is upregulated in children with therapy-resistant asthma (Persson et al., 2015). lncRNAs expressed from intergenic transcripts were also shown to have a supporting function in Th2 cell differentiation (Hu et al., 2013; Kanduri et al., 2015). A whole-genome sequencing approach identified differently lncRNAs to be expressed in human Th1, Th2, and Th17 cells (Spurlock et al., 2015). The Th2 locus control region (Th2-LCR) upregulated the expression of IL-5, IL-4, and IL-13. TH2-LCR showed Th2-specific demethylation after antigenic stimulation (Koh et al., 2010). Thus, TH2-LCR is critically important in Th2 responses in vivo and in vitro (Spurlock et al., 2015). A previous study showed that LINC00882, LINC00883, and PVT1 are differentially expressed in the primary airway smooth muscle cell phenotype (Perry et al., 2014). PVT1 is decreased in patients with corticosteroid-sensitive non-severe asthma and increased in patients with corticosteroid-insensitive severe asthma (Austin et al., 2017). Thus, PVT1 may be a therapeutic target for reducing airway remodeling (Yu et al., 2017). Our results revealed that LNC_000127 participates in PMA/CD28-induced cellular activation, suggesting that inflammation is regulated not only by small non-coding RNAs and protein modulators, but also by lncRNA. Particularly, the involvement of LNC_000127 appears to add another layer of complexity to regulation of the inflammatory response. Th2 differentiation occurs primarily via the JAK-STAT signaling pathway and STAT5A is a major STAT in the JAK-STAT pathway. Our results suggest that LNC_000127 plays roles in the TCR/STAT/GATA3 pathway in the Th2 inflammatory response.
Conclusion
Because Eos and Neu asthma have different inflammatory phenotypes, it is useful to analyze gene expression to explore their pathogenesis. Our results provide insight into the peripheral whole blood transcriptome lncRNA. Peripheral whole blood is easy to obtain and store and thus is an excellent source of biomarkers. Whether LNC_000127 can be used as a new therapeutic target and diagnostic biomarker for Eos asthma must be confirmed in further studies.
Data Availability
The sequencing data have been submitted to GEO and are accessible through the accession numbers GSE106230 and GSE117038.
Author Contributions
HH conceived and designed the study and revised the manuscript. YZ performed the RNA-related experiments and data analysis and drafted the manuscript. DM and WG participated in the experimental design and sample collection. GH conducted qPCR validation. All authors read and approved the final manuscript.
Conflict of Interest Statement
The authors declare that the research was conducted in the absence of any commercial or financial relationships that could be construed as a potential conflict of interest.
Acknowledgments
This work was supported by the National Key Scientific Instrument Project under Grant 2012YQ15009210, in part by the National Basic Research Program of China under Grant 11374370.
Supplementary Material
The Supplementary Material for this article can be found online at: https://www.frontiersin.org/articles/10.3389/fgene.2019.00141/full#supplementary-material
Supplementary Text 1. Text that presents detailed information on data analysis.
Supplementary Text 2. Method of PCR Assay SABio96.
Supplementary Text 3. Sequence of LNC_000127.
Supplementary Image 1. FISH experiment. Most lnc_000127 was detected in the nuclei. Green for the target gene, blue for DAPI, and third for composite image.
Supplementary Image 2. Three sets of white light and fluorescent photos. (A,B) KD group; (C,D) NC group; (E,F) Control group. Lentivirus transfection was successful in the KD and NC groups.
Supplementary Image 3. Western blotting. The decrease in protein (GATA3, CD40L, CCR8, STAT5A, and CRLF2) expression was confirmed by western blot analysis in the KD and NC/CV groups.
Supplementary Tables 1–4. showing significant mRNA annotation.
Supplementary Tables 5–8. showing significant lncRNA annotation.
Supplementary Table 9. presents KEGG pathway analysis for lncRNAs that differed between asthma and control samples.
Supplementary Table 10. presents KEGG pathway analysis for lncRNAs that differed between Eos and Neu samples.
Supplementary Table 11. presents the results of PCR Assay SABio96s (KD vs. NC/CV).
Abbreviations
lncRNA, long non-coding RNA; mRNA, messenger RNA; Eos, eosinophilic asthma; Neu, neutrophilic asthma; GO, gene ontology; KEGG, Kyoto encyclopedia of genes and genomes; RT-PCR, real-time polymerase chain reaction; NC/CV, normal control group; KD, knockdown group; TNF, tumor necrosis factor; FISH, fluorescence in situ hybridization; GAPDH, glyceraldehyde-3-phosphate dehydrogenase; Th2, T-helper cell type 2; ILC2, innate lymphoid cell type 2; DEGs, differentially expressed genes; FEV1, forced expiratory volume in the first second; FeNO, higher fractional exhaled nitric oxide; MF, molecular function.
References
Anderson, D. M., Anderson, K. M., Chang, C. L., Makarewich, C. A., Nelson, B. R., McAnally, J. R., et al. (2015). A micropeptide encoded by a putative long noncoding RNA regulates muscle performance. Cell 160, 595–606. doi: 10.1016/j.cell.2015.01.009
Austin, P. J., Tsitsiou, E., Boardman, C., Jones, S. W., Lindsay, M. A., Adcock, I. M., et al. (2017). Transcriptional profiling identifies the long noncoding RNA plasmacytoma variant translocation (PVT1) as a novel regulator of the asthmatic phenotype in human airway smooth muscle. J. Allergy Clin. Immunol. 139, 780–789. doi: 10.1016/j.jaci.2016.06.014
Baines, K. J., Simpson, J. L., Wood, L. G., Scott, R. J., and Gibson, P. G. (2011). Systemic upregulation of neutrophil alpha-defensins and serine proteases in neutrophilic asthma. Thorax 66, 942–947. doi: 10.1136/thx.2010.157719
Beckedorff, F. C., Ayupe, A. C., Crocci-Souza, R., Amaral, M. S., Nakaya, H. I., Soltys, D. T., et al. (2013). The intronic long noncoding RNA ANRASSF1 recruits PRC2 to the RASSF1A promoter, reducing the expression of RASSF1A and increasing cell proliferation. PLoS Genet. 9:e1003705. doi: 10.1371/journal.pgen.1003705
Chang, H. S., Lee, T. H., Jun, J. A., Baek, A. R., Park, J. S., Koo, S. M., et al. (2017). Neutrophilic inflammation in asthma: mechanisms and therapeutic considerations. Exp. Rev. Respir. Med. 11, 29–40. doi: 10.1080/17476348.2017.1268919
Chen, X., Li, X. M., Gu, W., Wang, D., Chen, Y., and Guo, X. J. (2017). LAT alleviates Th2/Treg imbalance in an OVA-induced allergic asthma mouse model through LAT-PLC-gamma1 interaction. Int. Immunopharmacol. 44, 9–15. doi: 10.1016/j.intimp.2016.12.029
Chung, K. F., Wenzel, S. E., Brozek, J. L., Bush, A., Castro, M., Sterk, P. J., et al. (2014). International ERS/ATS guidelines on definition, evaluation and treatment of severe asthma. Eur. Respir. J. 43, 343–373. doi: 10.1183/09031936.00202013
Du, Z., Sun, T., Hacisuleyman, E., Fei, T., Wang, X., Brown, M., et al. (2016). Integrative analyses reveal a long noncoding RNA-mediated sponge regulatory network in prostate cancer. Nat. Commun. 7:10982. doi: 10.1038/ncomms10982
Han, P., Li, W., Lin, C. H., Yang, J., Shang, C., Nuernberg, S. T., et al. (2014). A long noncoding RNA protects the heart from pathological hypertrophy. Nature 514, 102–106. doi: 10.1038/nature13596
Hu, G., Tang, Q., Sharma, S., Yu, F., Escobar, T. M., Muljo, S. A., et al. (2013). Expression and regulation of intergenic long noncoding RNAs during T cell development and differentiation. Nat. Immunol. 14, 1190–1198. doi: 10.1038/ni.2712
Hu, S., Wang, X., and Shan, G. (2016). Insertion of an Alu element in a lncRNA leads to primate-specific modulation of alternative splicing. Nat. Struct. Mol. Biol. 23, 1011–1019. doi: 10.1038/nsmb.3302
Kanduri, K., Tripathi, S., Larjo, A., Mannerström, H., Ullah, U., Lund, R., et al. (2015). Identification of global regulators of T-helper cell lineage specification. Genome Med. 7:122. doi: 10.1186/s13073-015-0237-0
Keenan, C. R., Schuliga, M. J., and Stewart, A. G. (2015). Pro-inflammatory mediators increase levels of the noncoding RNA GAS5 in airway smooth muscle and epithelial cells. Can. J. Physiol. Pharmacol. 93, 203–206. doi: 10.1139/cjpp-2014-0391
Kino, T., Hurt, D. E., Ichijo, T., Nader, N., and Chrousos, G. (2010). Noncoding RNA gas5 is a growth arrest- and starvation-associated repressor of the glucocorticoid receptor. Sci. Signal 3:ra8. doi: 10.1126/scisignal.2000568
Koh, B. H., Hwang, S. S., Kim, J. Y., Lee, W., Kang, M. J., Lee, C. G., et al. (2010). Th2 LCR is essential for regulation of Th2 cytokine genes and for pathogenesis of allergic asthma. Proc. Natl. Acad. Sci. U.S.A. 107, 10614–10619. doi: 10.1073/pnas.1005383107
Kotake, Y., Nakagawa, T., Kitagawa, K., Suzuki, S., Liu, N., Kitagawa, M., et al. (2011). Long non-coding RNA ANRIL is required for the PRC2 recruitment to and silencing of p15(INK4B) tumor suppressor gene. Oncogene 30, 1956–1962. doi: 10.1038/onc.2010.568
Li, T., Ke, Y., and Cheng, H. (2016). Reasearch progress on the role of neutrophils in asthma. Zhejiang Da Xue Xue Bao Yi Xue Ban 45, 544–549. doi: 10.3785/j.issn.1008-9292.2016.09.15
Matsumoto, A., Pasut, A., Matsumoto, M., Yamashita, R., Fung, J., Monteleone, E., et al. (2017). mTORC1 and muscle regeneration are regulated by the LINC00961-encoded SPAR polypeptide. Nature 541, 228–232. doi: 10.1038/nature21034
Mercer, T. R., and Mattick, J. S. (2013). Structure and function of long noncoding RNAs in epigenetic regulation. Nat. Struct. Mol. Biol. 20, 300–307. doi: 10.1038/nsmb.2480
Muehling, L. M., Lawrence, M. G., and Woodfolk, J. A. (2017). Pathogenic CD4(+) T cells in patients with asthma. J. Allergy Clin. Immunol. 140, 1523–1540. doi: 10.1016/j.jaci.2017.02.025
Nelson, B. R., Makarewich, C. A., Anderson, D. M., Winders, B. R., Troupes, C. D., Wu, F., et al. (2016). A peptide encoded by a transcript annotated as long noncoding RNA enhances SERCA activity in muscle. Science 351, 271–275. doi: 10.1126/science.aad4076
Perry, M. M., Tsitsiou, E., Austin, P. J., Lindsay, M. A., Gibeon, D. S., Adcock, I. M., et al. (2014). Role of non-coding RNAs in maintaining primary airway smooth muscle cells. Respir. Res. 15:58. doi: 10.1186/1465-9921-15-58
Persson, H., Kwon, A. T., Ramilowski, J. A., Silberberg, G., Söderhäll, C., Orsmark-Pietras, C., et al. (2015). Transcriptome analysis of controlled and therapy-resistant childhood asthma reveals distinct gene expression profiles. J. Allergy Clin. Immunol. 136, 638–648. doi: 10.1016/j.jaci.2015.02.026
Qiao, Y. Q., Huang, M. L., Xu, A. T., Zhao, D., Ran, Z. H., and Shen, J. (2013). LncRNA DQ786243 affects Treg related CREB and Foxp3 expression in Crohn's disease. J. Biomed. Sci. 20:87. doi: 10.1186/1423-0127-20-87
Quinn, J. J., and Chang, H. Y. (2016). Unique features of long non-coding RNA biogenesis and function. Nat. Rev. Genet. 17, 47–62. doi: 10.1038/nrg.2015.10
Reddy, M. A., Chen, Z., Park, J. T., Wang, M., Lanting, L., Zhang, Q., et al. (2014). Regulation of inflammatory phenotype in macrophages by a diabetes-induced long noncoding RNA. Diabetes 63, 4249–4261. doi: 10.2337/db14-0298
Shan, K., Liu, C., Liu, B. H., Chen, X., Dong, R., Liu, X., et al. (2017). Circular noncoding RNA HIPK3 mediates retinal vascular dysfunction in diabetes mellitus. Circulation 136, 1629–1642. doi: 10.1161/CIRCULATIONAHA.117.029004
Shinoda, K., Hirahara, K., and Nakayama, T. (2017). Maintenance of pathogenic Th2 cells in allergic disorders. Allergol. Int. 66, 369–376. doi: 10.1016/j.alit.2017.03.005
Simpson, J. L., Scott, R., Boyle, M. J., and Gibson, P. G. (2006). Inflammatory subtypes in asthma: assessment and identification using induced sputum. Respirology 11, 54–61. doi: 10.1111/j.1440-1843.2006.00784.x
Smeets, R. L., Fleuren, W. W., He, X., Vink, P. M., Wijnands, F., Gorecka, M., et al. (2012). Molecular pathway profiling of T lymphocyte signal transduction pathways; Th1 and Th2 genomic fingerprints are defined by TCR and CD28-mediated signaling. BMC Immunol. 13:12. doi: 10.1186/1471-2172-13-12
Song, X., Wang, X., Arai, S., and Kurokawa, R. (2012). Promoter-associated noncoding RNA from the CCND1 promoter. Methods Mol. Biol. 809, 609–622. doi: 10.1007/978-1-61779-376-9_39
Spurlock, C. F., Tossberg, J. T., Guo, Y., Collier, S. P., Crooke, P. S., and Aune, T. M. (2015). Expression and functions of long noncoding RNAs during human T helper cell differentiation. Nat. Commun. 6:6932. doi: 10.1038/ncomms7932
Tsitsiou, E., Williams, A. E., Moschos, S. A., Patel, K., Rossios, C., Jiang, X., et al. (2012). Transcriptome analysis shows activation of circulating CD8+ T cells in patients with severe asthma. J. Allergy Clin. Immunol. 129, 95–103. doi: 10.1016/j.jaci.2011.08.011
Villegas, V. E., and Zaphiropoulos, P. G. (2015). Neighboring gene regulation by antisense long non-coding RNAs. Int. J. Mol. Sci. 16, 3251–3266. doi: 10.3390/ijms16023251
Volders, P. J., Verheggen, K., Menschaert, G., Vandepoele, K., Martens, L., Vandesompele, J., et al. (2015). An update on LNCipedia: a database for annotated human lncRNA sequences. Nucl. Acids Res. 43, 4363–4364. doi: 10.1093/nar/gkv295
Wang, P., Xue, Y., Han, Y., Lin, L., Wu, C., Xu, S., et al. (2014). The STAT3-binding long noncoding RNA lnc-DC controls human dendritic cell differentiation. Science 344, 310–313. doi: 10.1126/science.1251456
Wang, Y., Qian, C. Y., Li, X. P., Zhang, Y., He, H., Wang, J., et al. (2015). Genome-scale long noncoding RNA expression pattern in squamous cell lung cancer. Sci. Rep. 5:11671. doi: 10.1038/srep11671
Warburton, A. J., and Boone, D. N. (2017). Insights from global analyses of long noncoding RNAs in breast cancer. Curr. Pathobiol. Rep. 5, 23–34. doi: 10.1007/s40139-017-0122-1
Wynn, T. A. (2015). Type 2 cytokines: mechanisms and therapeutic strategies. Nat. Rev. Immunol. 15, 271–282. doi: 10.1038/nri3831
Xia, F., Dong, F., Yang, Y., Huang, A., Chen, S., Sun, D., et al. (2014). Dynamic transcription of long non-coding RNA genes during CD4+ T cell development and activation. PLoS ONE 9:e101588. doi: 10.1371/journal.pone.0101588
Xie, C., Yuan, J., Li, H., Li, M., Zhao, G., Bu, D., et al. (2014). NONCODEv4: exploring the world of long non-coding RNA genes. Nucl. Acids Res. 42, D98–103. doi: 10.1093/nar/gkt1222
Yu, X., Zhe, Z., Tang, B., Li, S., Tang, L., Wu, Y., et al. (2017). alpha-Asarone suppresses the proliferation and migration of ASMCs through targeting the lncRNA-PVT1/miR-203a/E2F3 signal pathway in RSV-infected rats. Acta Biochim. Biophys. Sin. 49, 598–608. doi: 10.1093/abbs/gmx048
Zhang, X., Lian, Z., Padden, C., Gerstein, M. B., Rozowsky, J., Snyder, M., et al. (2009). A myelopoiesis-associated regulatory intergenic noncoding RNA transcript within the human HOXA cluster. Blood 113, 2526–2534. doi: 10.1182/blood-2008-06-162164
Keywords: long non-coding RNA, eosinophilic asthma, neutrophilic asthma, peripheral whole blood, RNA-sequencing
Citation: Zhu Y, Mao D, Gao W, Han G and Hu H (2019) Analysis of lncRNA Expression in Patients With Eosinophilic and Neutrophilic Asthma Focusing on LNC_000127. Front. Genet. 10:141. doi: 10.3389/fgene.2019.00141
Received: 04 September 2018; Accepted: 11 February 2019;
Published: 19 March 2019.
Edited by:
Rui Henrique, Portuguese Oncology Institute, PortugalReviewed by:
Mark Andrew Lindsay, University of Bath, United KingdomEzgi Hacisuleyman, Rockefeller University, United States
Copyright © 2019 Zhu, Mao, Gao, Han and Hu. This is an open-access article distributed under the terms of the Creative Commons Attribution License (CC BY). The use, distribution or reproduction in other forums is permitted, provided the original author(s) and the copyright owner(s) are credited and that the original publication in this journal is cited, in accordance with accepted academic practice. No use, distribution or reproduction is permitted which does not comply with these terms.
*Correspondence: Hong Hu, aHVob25nX19fZHJAYWxpeXVuLmNvbQ==