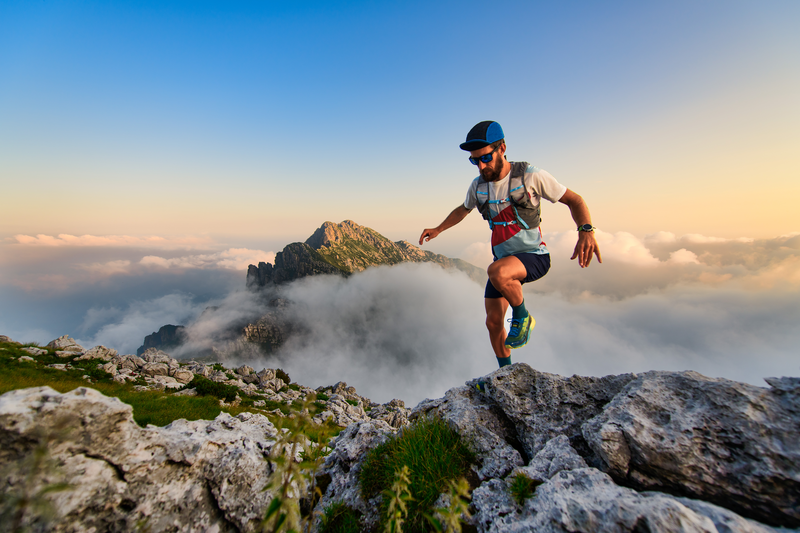
94% of researchers rate our articles as excellent or good
Learn more about the work of our research integrity team to safeguard the quality of each article we publish.
Find out more
ORIGINAL RESEARCH article
Front. Genet. , 14 March 2018
Sec. Evolutionary and Population Genetics
Volume 9 - 2018 | https://doi.org/10.3389/fgene.2018.00075
This article is part of the Research Topic Genetics, Evolution, and Conservation of Neotropical Fishes View all 34 articles
Some mammalian reference genes, which are widely used to normalize the qRT-PCR, could not be used for this purpose due to its high expression variation. The normalization with false reference genes leads to misinterpretation of results. The silversides (Odontesthes spp.) has been used as models for evolutionary, osmoregulatory and environmental pollution studies but, up to now, there are no studies about reference genes in any Odontesthes species. Furthermore, many studies on silversides have used reference genes without previous validations. Thus, present study aimed to was to clone and sequence potential reference genes, thereby identifying the best ones in Odontesthes humensis considering different tissues, ages and conditions. For this purpose, animals belonging to three ages (adults, juveniles, and immature) were exposed to control, Roundup®, and seawater treatments for 24 h. Blood samples were subjected to flow-cytometry and other collected tissues to RNA extraction; cDNA synthesis; molecular cloning; DNA sequencing; and qRT-PCR. The candidate genes tested included 18s, actb, ef1a, eif3g, gapdh, h3a, atp1a, and tuba. Gene expression results were analyzed using five algorithms that ranked the candidate genes. The flow-cytometry data showed that the environmental challenges could trigger a systemic response in the treated fish. Even during this systemic physiological disorder, the consensus analysis of gene expression revealed h3a to be the most stable gene expression when only the treatments were considered. On the other hand, tuba was the least stable gene in the control and gapdh was the least stable in both Roundup® and seawater groups. In conclusion, the consensus analyses of different tissues, ages, and treatments groups revealed that h3a is the most stable gene whereas gapdh and tuba are the least stable genes, even being considered two constitutive genes.
Northern-blotting, ribonuclease protection assay, microarray, RNA-Seq, semi-quantitative RT-PCR, and quantitative RT-PCR (qRT-PCR) are among the most currently used techniques for evaluation of gene expression levels. The latter stands out for fast readout, high throughput and high automation potential (Tang et al., 2012). In absolute quantification of qRT-PCR, the increasing level of fluorescent signal emitted by the amplified products is compared to a standard curve. The relative quantification describes the change in the expression pattern of genes of interest when subjected to certain controlled situations compared to the constant expression of reference genes (RGs), also known as housekeeping genes under same conditions. Therefore, it is extremely important to have a stable and constant expression pattern of RGs regardless of gender, age, organ or tissue, and even after environmental changes. Thus, it is necessary to validate diverse RGs for various species and their stages of development in different tissues, as well as for each type of controlled environmental condition (Livak and Schmittgen, 2001; Zheng and Sun, 2011).
However, most studies related to the quantification of gene expression in various species of teleosts are not based on RGs displaying unchanged expression. Most studies use RGs properly confirmed in mammalian species to normalize the relative quantification using qRT-PCR of non-mammalian species, such as fishes. The main RGs from mammals that have been empirically used to normalize experiments in teleost species are the GAPDH (Lin et al., 2004); ACTB (Choi and An, 2008; Nawata et al., 2010); EF1α (Scott et al., 2004; Nilsen et al., 2007; Patterson et al., 2012; Sinha et al., 2015); RPL (Hsu et al., 2014) and 18S (Sinha et al., 2015). Studies also report the distribution of RGs in the tissues; however, these genes are not objectively evaluated in relation to the maintenance of the constant expression during development stages of individuals, in both genders or in different controlled environmental conditions (Tomy et al., 2009; Hsu et al., 2014).
Numerous studies have reported that some mammalian RGs, which are widely used to normalize the qRT-PCR reactions of different species (i.e., ACTB and GAPDH), could not be used owing to their high variation in expression levels (Bustin, 2002; Dheda et al., 2004; Silver et al., 2006). This could lead to misinterpretation of results. It is impossible to find suitable RGs that exhibit constant expression pattern for all species and under all experimental conditions (Zheng and Sun, 2011; Tang et al., 2012). Due to these reasons, the identification of RGs for each species and each experimental condition is justified.
The silversides (Odontesthes spp.) comprise a genus of fish that are naturally endemic to the South American waters (Bemvenuti, 2006). This genus consists of the biggest number of species of the South American atherinopsids (Dyer, 2006). In nature, the silversides are usually found in southern Brazil, Uruguay, and Argentina (Bemvenuti, 2006). In these regions, some species have economic importance for production, flesh commercialization and sport fishing (Menone et al., 2000; Somoza et al., 2008). Furthermore, some silversides have been used as experimental models for evolutionary, osmoregulatory, and environmental pollution studies due to the radiation after continental waters invasion from ocean, euryhaline biology and inhabitation of environments contaminated by glyphosate coming from rice and soybean monocultures in fields near to the habitat of silversides (Menone et al., 2000; Carriquiriborde and Ronco, 2006; Tsuzuki et al., 2007; Piedras et al., 2009; Bloom et al., 2013; Hughes et al., 2017; Zebral et al., 2017). Moreover, the silversides have already been utilized to address various aspects of gene expression (Strobl-Mazzulla et al., 2005; Karube et al., 2007; Fernandino et al., 2008, 2011; Majhi et al., 2009; Miranda et al., 2009; Blasco et al., 2010; Gómez-Requeni et al., 2012; Pérez et al., 2012; González et al., 2015). The expression pattern of a gene can be used to assess physiological responses of fishes to environmental changes, such as those to which some silversides are exposed in their habitat (salinity variations and contamination by glyphosate) (Wang et al., 2014; Velasques et al., 2016).
Similarly, Odontesthes humensis de Buen, 1953, a native species to coastal lagoons from southern Brazil, Uruguay and Argentina, has similar potential to be used as a biological model. However, a previous basic knowledge about the RGs is necessary to employ modern techniques such as qRT-PCR to study the gene expression of different genes of interest, independent from the biological model. Up to now, no studies have focused on the sequence determination and validation of RGs in any species of Odontesthes spp. Due to this, the present study aimed to clone and sequence eight potential RGs, thereby identifying and validating the best RGs in O. humensis using different tissues, stages of development and a natural and an anthropized environmental conditions, to standardize the qRT-PCR technique.
The silversides Odontesthes humensis used in this study was procreated from the eggs previously collected in nature (Arroio Grande, Brazil: 32°14′15″S/53°05′13″W) and hatched in tanks. The silversides were kept within an experimental room in nine 1,000 L circular plastic tanks with water at a temperature of 13.3 ± 1.8°C, pH 7.0 ± 0.7, salinity 3.2 ± 0.67 ppt, dissolved oxygen 9.5 ± 0.5 mg/L, ammonia levels lower than 0.6 mg/L and a natural photoperiod of winter. The tank sides were opaque to reduce visual stress, and once a week 2/3 of the water in the tanks were renewed. Three tanks were occupied by 3-year-old fish (5 animals/tank), classified as adults considering the complete development of gonads. Other three tanks were occupied by 1.5-year-old fish (5 animals/tank), classified as juveniles considering the partial development of gonads. In addition, three tanks were occupied by 6-month-old silversides (5 animals/tank), classified as immature due to the undifferentiated gonads. The animals were fed thrice a day on a commercial diet (Supra, 38% crude protein) and zooplankton ad libitum. The acclimation period was 4 weeks under these conditions.
The experimental design consisted of three treatment groups: the “control group,” exposed to water in previously mentioned conditions; the “Roundup® group,” exposed to Roundup® Transorb (a glyphosate based herbicide) diluted in water at a concentration of 10 mg.L−1 (acid equivalent, a.e.) of glyphosate; and the “seawater group,” exposed to seawater at 30 ppt (dissolution of non-iodized sea salt in the medium). Three tanks were prepared for each treatment, the first one received the adults, the second one received the juveniles, and the third one received the immature individuals. After the acclimation period, the animals were exposed to treatments for 24 h. The water quality parameters did not differ statistically from those observed in the acclimatization period, except for the salinity of the group exposed to seawater. During the experimental period, the fish continued to be fed as during the acclimation period.
Each animal was hooked from the tank and anesthetized by submersion in benzocaine at 50 ppm. Three fish from each tank were collected, leading to a total of 27 animals. The animals were weighed, measured, and euthanized by cranial spinal section and excision of the brain. Adults, juveniles and immature silversides had mean lengths of 20.2 ± 2.3 cm, 15.2 ± 1.2 cm, and 11.7 ± 0.7 cm and mean weight of 55.2 ± 11.7 g, 24.6 ± 6.5 g, and 9.6 ± 1.9 g, respectively. Brain, hepatopancreas, gills, and kidney (the main organs affected by glyphosate and/or hyperosmotic environments in fishes; Tipsmark et al., 2004; Carriquiriborde and Ronco, 2006; Hiroi and McCormick, 2012; Harayashiki et al., 2013; Velasques et al., 2016) from adults, juveniles and immature silversides in the three treated groups were collected and preserved in liquid nitrogen (N2). Furthermore, a total of 20 μL of peripheral blood was collected by puncturing the caudal fin. Each blood sample was added to 1 mL of fetal bovine serum (FBS), stored at 4°C, and protected from the light until used for flow-cytometry analysis. The animal use and all handling practices were approved by the Ethics Committee on Animal Experimentation of the Federal University of Pelotas (process no. 23110.007018/2015-85). The Roundup® residual from experimental tanks was sent to university chemical waste facility to to be treated prior to disposal.
The flow-cytometry analysis was performed using the Attune® Acoustic Focusing Flow Cytometer (Applied Biosystems, USA) to evaluate the efficacy of treatments to affect the physiology of silversides. Each blood sample was exposed to 2 mM of Hoechst 33342 (H33342; Sigma-Aldrich, USA) for 5 min before each read, except in the DNA damage analysis. Events were detected by fluorochrome with violet laser (405 nm) and photomultiplier (PMT) VL1 (filter 450/40 nm). The green, orange, and red fluorescences were analyzed by blue laser (488 nm) and PMTs BL1 (filter 530/30 nm), BL2 (filter 575/24 nm), and BL3 (filter > 640 nm) filters, respectively. Cytometry fluorescence stability was tested daily using standard beads (Invitrogen, USA). The acquisition rate was 200 events/s totaling 20,000 events per sample. All assays were performed in triplicate. The results were analyzed using the Attune Cytometric Software v2.1. The non-cellular events were eliminated from the analysis by scatter plots of forward scatter (FSC) × side scatter (SSC) (Petrunkina et al., 2005) and negative fluorescence of H33342 events (debris).
For evaluation of reactive oxygen species (ROS) produced by the erythrocytes, 10 μL of blood sample previously collected and stored was added to 20 μL of saline solution with 2 μM of 2′,7′-dichlorofluorescein diacetate (DCFH-DA) and 5 μM of propidium iodide (PI) fluorescent probes (Sigma-Aldrich Co., USA). The samples were analyzed in triplicate after incubation for 60 min at 22°C in the dark. The ROS production was measured by the median of the intensity of the green fluorescence. Only intact cells (PI negative) were evaluated.
To evaluate DNA damage in the erythrocytes, 10 μL of blood sample previously collected and stored was added to 5 μL of TNE (0.01 M Tris-HCl. 0.15 M NaCl, 0.001 M EDTA, pH 7.2) and 30 s later to 10 μL of Triton 1X (Triton X-100, 1%, v/v). Then, 50 μL acridine orange dye (2 mg/mL, #A6014, Sigma-Aldrich, USA) was added to the sample, followed by incubation from 30 s up to 2 min before each reading. The samples were analyzed in triplicate. The DNA of the erythrocytes was classified as integrated (green fluorescence emission) and damaged (orange/red fluorescence emission). The percentage of DNA fragmentation index (DFI) was obtained by the median of the red fluorescence intensity/(median of the red + green fluorescence intensities) × 100.
The total RNA was extracted from tissue samples using the commercial kit RNeasy® Mini Kit (Qiagen, USA) following the manufacturer's instructions. The RNA was treated with DNA-free® Kit (Ambion, USA) to remove genomic DNA contamination. Subsequently, the RNA concentration and purity were measured using a spectrophotometer NanoVue™ Plus (GE Healthcare Life Science, USA), and only the samples presenting high purity (OD260/280 ≥ 2.0 nm) were used. In addition, RNA quality was analyzed on the 4,200 TapeStation (Agilent Technologies, USA) system using the TapeStation analysis application. The mean RIN number for each experimental group is listed in Supplementary Table 1. First-strand cDNA synthesis was performed with 2 μg of RNA using the commercial kit High Capacity cDNA Reverse Transcription (Applied Biosystems, USA) according to manufacturer's recommendation. The reactions were run in SimpliAmp™ thermal cycler (Applied Biosystems, USA). Finally, the cDNA was stored at −20°C until its use.
The tested candidates RGs were: 18S ribosomal RNA (18s), β-actin (actb), elongation factor 1 α (ef1a), eukaryotic translation initiation factor 3g (eif3g), glyceraldehyde-3-phosphate dehydrogenase (gapdh), histone h3a (h3a), Na+/K+-ATPase α (atp1a), and tubulin-α (tuba) (Table 1). The atp1a gene represented the control, which is a constitutive gene that is reported to be affected by glyphosate-based herbicides and salt treatments (Shiogiri et al., 2012; Armesto et al., 2014).
The gene fragments were amplified by PCR using primers designed in the PriFi online tool (https://services.birc.au.dk/prifi/) after alignments (Supplementary Table 2) of known sequences deposited in the GenBank (Table 2). The PCR was performed using the mix buffer GoTaq® G2 Flexi DNA Polymerase (Promega, USA) following manufacturer's instructions. The reactions were run in the SimpliAmp™ thermal cycler (Applied Biosystems, USA). The PCR parameters were: an initial denaturation step for 1 min at 94°C, followed by 35 cycles at 94°C for 30 s, 59.9 to 65.5°C (depending on the primer sequence, Table 2) for 30 s; and 72°C for 1 min, with a final extension of 5 min at 72°C. To confirm the amplification of the fragments, the reactions were analyzed using 1.5% agarose gel electrophoresis. The PCR products were inserted into the cloning vector pCR™4-TOPO® TA (Invitrogen, USA). The transformed vector was used to transform electrocompetent Escherichia coli DH5α.
The transformed E. coli colonies were selected and cultured in 3 mL of Luria broth (LB) medium containing kanamycin antibiotic. The resistant bacteria were used to isolate the vector and the fragment was purified using the commercial kit Illustra plasmidPrep Mini Spin (GE Healthcare, USA) following the manufacturer's instructions. The purified samples of ten cloning fragments were submitted to sequencing by BigDye® Terminator v3.1 Cycle Sequencing (Applied Biosystems, USA) following the manufacturer's instructions. The reaction mixtures were incubated in a SimpliAmp™ thermal cycler (Applied Biosystems, USA) under the following parameters: 96°C for 1 min, 35 cycles of 96°C for 1 min, 50°C for 5 s, 60°C for 4 min.
One more purification was carried out using the BigDye® XTerminator™ Purification (Applied Biosystems, USA) according to the manufacturer's instructions. Following the purification, the samples were submitted to sequencing using an Applied Biosystems 3,500 Genetic Analyzer® automatic sequencer (Life Technologies, USA). The sequences were analyzed using the Vector NTI and deposited in GenBank®.
The primers used for qRT-PCR were designed using the previous sequence of the cloned fragments and Primer3 online tool (www.bioinfo.ut.ee/primer3-0.4.0/). The primers used in this study are presented in Table 2. The qRT-PCR was run in a Stratagene® Mx3005P™ Real-Time PCR System (Agilent Technologies, USA) using SYBR® Green PCR Master Mix (Applied Biosystems, USA). The amplification conditions were 95°C for 10 min, 40 cycles at 95°C for 15 s, and 60°C for 60 s followed by the conditions needed to calculate the melting curve. All the reactions were performed in triplicate.
The normality distribution of flow-cytometry data was evaluated by the Shapiro-Wilk test. None of the evaluated parameters presented normal distribution, thus, the data were submitted to the Kruskal-Wallis non-parametric test followed by Dunn's all-pairwise multiple comparisons, with a significance level of 5%. The results of ROS production and DFI were expressed as the mean fluorescent intensity and mean percentage, respectively, ± standard error of the means (SEM).
The cycle threshold (Ct) values were exported from the detection software MxPro™ v.4.1 (Stratagene, Waldbronn, Germany) to Excel files to facilitate the descriptive analysis and the values transformations required by some software. The specific analyses of stability of gene expression were performed using the comparative delta-Ct (dCt) method (Silver et al., 2006) and geNorm (Vandesompele et al., 2002), NormFinder (Andersen et al., 2004), BestKeeper (Pfaffl et al., 2004), and RefFinder (http://150.216.56.64/referencegene.php?type=reference) statistical approaches as previously reported (Yang et al., 2013; Taki et al., 2014). The results were expressed as average of standard deviation (SD) to dCt method; average expression stability value to geNorm and NormFinder; Pearson's correlation coefficient ([r]) to BestKeeper; and geometric mean of ranking values to RefFinder.
Except for atp1a gene, which was previously known (Silveira et al., 2018), all the other seven silverside O. humensis candidate RGs analyzed in this study were cloned and partially sequenced for the first time. Furthermore, the new sequences were deposited in the GenBank® (Table 1). The sequence length of 18s was 640 base pairs (bp). The sequence lengths of actb; ef1a; eif3g; gapdh; h3a; and tuba cloned fragments from O. humensis were 350, 638, 435, 325, 222, and 527 bp, respectively, that encoded 116, 212, 144, 108, 73, and 176 amino acids, respectively. The cloned fragments of tuba belongs to the open reading frame (ORF) +1, the fragments of actb and eif3g are part of the ORF +2, and the fragments of ef1a, gapdh, and h3a compose the ORF +3.
The exposure of silversides for 24 h to both Roundup® and seawater caused an increase in the ROS production in erythrocytes (P < 0.05) when compared to the control group (Figure 1A). Similarly, the Roundup® and seawater treatments increased (P < 0.05) the DFI of the erythrocytes in the exposed silversides (Figure 1B). The increase in ROS production and DNA damage in the treated fish confirmed that both Roundup® and seawater treatments negatively affected the physiology of silversides, thereby generating a systemic effect on fish.
Figure 1. Effects in erythrocytes of Odontesthes humensis of exposures to Roundup® and to seawater. Effects of exposures to Roundup® Transorb [10 mg.L−1 (acid equivalent) of glyphosate] and to seawater (30 ppt) for 24 h on ROS (Reactive Oxygen Species) production (A) and in DFI (DNA Fragmentation Index, %) (B). Mean ± SEM, n = 9. Different letters represents significant statistical difference between the measurements of the treatment groups (P < 0.05).
The comparative dCt method was used to select the most stable RG. A low average of SD value represented a low expression variance, or high stability. The analysis revealed the following order of stability of evaluated genes, from higher to lower, in water with controlled quality parameters (control group): h3a (2.57) > ef1a (2.82) = actb (2.82) > eif3g (3.00) > 18s (3.21) > gapdh (3.66) > tuba (3.77) > atp1a (4.98) (Figure 2A). The ranking order of stability of the evaluated genes upon exposure of silversides to Roundup® treatment was h3a (2.74) > actb (2.79) > ef1a (2.95) > eif3g (3.03) > 18s (3.51) > tuba (3.82) > gapdh (3.95) > atp1a (6.18) (Figure 2B). In saline condition, the seawater treated group presented the following stability order: h3a (2.62) > eif3g (2.91) > ef1a (3.00) > actb (3.19) > 18s (3.72) > tuba (3.84) > gapdh (3.90) > atp1a (5.05) (Figure 2C).
Figure 2. Stability analysis of the candidates reference genes in Odontesthes humensis calculated by dCt method. Gene expression stability in silversides exposed to water with quality parameters controlled (A); to Roundup® Transorb [10 mg.L−1 (acid equivalent) of glyphosate] (B); and to seawater (30 ppt) (C) across the different treatments for 24 h. The most stable genes are displayed on the left, and the least stable genes are displayed on the right of the x-axis.
The geNorm analysis was carried out for the result data set after transformation of the Ct values into relative quantities through the 2(minimum Ct value in a set sample−Ct value of a sample) formula and compare pairwise variation (SD values) for each gene pair. Then, the geometric mean of SD values was used to calculate the M-value. The generation of low average expression stability represents a low variance. The geNorm analysis revealed the following ranking order of stability of genes in the control group: h3a/actb (1.14) > eif3g (1.62) > ef1a (1.85) > 18s (2.19) > tuba (2.53) > gapdh (2.81) > atp1a (3.35) (Figure 3A). In the Roundup® group, the sequence of stability of the evaluated genes from the highest to the lowest was h3a/actb (1.19) > eif3g (1.43) > ef1a (1.61) > tuba (2.09) > 18s (2.44) > gapdh (2.77) > atp1a (3.62) (Figure 3B). When exposed to seawater, the silversides genes exhibited the following stability ranking order: h3a/eif3g (1.48) > actb (1.68) > ef1a (1.85) > tuba (2.36) > 18s (2.74) > gapdh (3.02) > atp1a (3.53) (Figure 3C).
Figure 3. Stability analysis of the candidates reference genes in Odontesthes humensis calculated by geNorm algorithm. Gene expression stability in silversides exposed to water with quality parameters controlled (A); to Roundup® Transorb [10 mg.L−1 (acid equivalent) of glyphosate] (B); and to seawater (30 ppt) (C) across the different treatments for 24 h. The most stable genes are displayed on the left, and the least stable genes are displayed on the right of the x-axis.
The NormFinder was applied to analyze the most stable evaluated genes. A low average of expression stability represents a low variance. The NormFinder analysis revealed the ranking order of stability in the control group to be h3a (0.79) > ef1a (1.26) > actb (1.63) > eif3g (1.95) > 18s (2.06) > gapdh (2.79) > tuba (3.05) > atp1a (4.55) (Figure 4A). For the Roundup® group, the ranking of expression stability was h3a (0.89) > actb (1.23) > ef1a (1.43) > eif3g (1.79) > 18s (2.15) > tuba (2.89) > gapdh (3.01) > atp1a (5.83) (Figure 4B). When exposed to the seawater, the ranking order of RGs was h3a (0.74) > ef1a (1.53) > eif3g (1.61) > actb (2.19) > 18s (2.70) > tuba (2.97) > gapdh (3.04) > atp1a (4.54) (Figure 4C). In addition, the best combination of genes for the experimental conditions was evaluated. A comparison of the gene expression in silversides of the control and the Roundup® groups, and of the control and the seawater groups revealed the best combination of genes to be h3a and ef1a with combined stability values of 0.28 and 0.29, respectively. The best suitable combination of two genes for all three tested conditions was also h3a and ef1a with a combined stability value of 0.26.
Figure 4. Stability analysis of the candidates reference genes in Odontesthes humensis calculated by NormFinder algorithm. Gene expression stability in silversides exposed to water with quality parameters controlled (A); to Roundup® Transorb [10 mg.L−1 (acid equivalent) of glyphosate] (B); and to seawater (30 ppt) (C) across the different treatments for 24 h. The most stable genes are displayed on the left, and the least stable genes are displayed on the right of the x-axis.
The BestKeeper analysis provided two-interpretation-ways to rank the gene stability: one based on the samples SD values of Ct and other based on the Pearson's correlation of expression among the genes. Thus, the genes with low SDs and high correlation with the BestKeeper index (indicating high similarity among the expression levels of the RGs) are ranked as the most stable genes. To construct the consensus comprehensive ranking, the SD values were used, which is a more conservative approach. However, to construct the rankings of the most stable genes with BestKeeper, [r] and P-values of Pearson's correlation were employed. This way is more sophisticated and statistically robust, as it results in the rankings more similar to the ones obtained using other algorithms.
The analyses based on Pearson's correlation revealed the following ranking order of stability in the control group: h3a (0.93) > actb (0.90) > ef1a (0.80) > eif3g (0.79) > tuba (0.76) > 18s (0.62) > gapdh (0.46) > atp1a (0.24) (Figure 5A). The genes presented a positive correlation with the BestKeeper index with P = 0.001 except atp1a with P = 0.05. In the Roundup® group the ranking of the best RG was h3a (0.83) = actb (0.83) > ef1a (0.79) > eif3g (0.78) > tuba (0.70) > gapdh (0.49) > 18s (0.41) > atp1a (0.26) (Figure 5B). All correlation analysis presented P = 0.001 except atp1a with P = 0.08. In the seawater group the ranking order from the most to the least stable genes was h3a (0.91) > ef1a (0.89) > actb (0.87) > eif3g (0.83) > tuba (0.76) > gapdh (0.49) > 18s (0.23) > atp1a (0.22) (Figure 5C). The genes atp1a and 18s exhibited P = 0.1 and P = 0.08, respectively. All the other genes displayed positive correlations with P = 0.001.
Figure 5. Stability analysis of the candidates reference genes in Odontesthes humensis calculated by BestKeeper algorithm. Gene expression stability in silversides exposed to water with quality parameters controlled (A); to Roundup® Transorb [10 mg.L−1 (acid equivalent) of glyphosate] (B); and to seawater (30 ppt) (C) across the different treatments for 24 h. The most stable genes are displayed on the left, and the least stable genes are displayed on the right of the x-axis.
The RefFinder is an online tool used to construct a consensus comprehensive ranking of stability of RGs among all the other methods using the calculation of geometric mean for the ranks calculated by each of other algorithms. Candidate genes with the lowest geometric mean are most stable.
Besides the graphical rankings of RefFinder, it presented the consensus results discriminated by treatment group (control, Roundup®, and seawater) by developmental stages (adults, juveniles, and immature) and tissue types (brain, gills, hepatopancreas, and kidney).
In the control group, the most stable gene across the different ages and tissues was h3a. The only exception was the brain samples, where the most stable gene was tuba. The least stable gene across the developmental stages and tissues was atp1a (Table 3).
Table 3. Consensus stability ranking by RefFinder of the candidate reference genes in Odontesthes humensis under normal conditions.
In the Roundup® group the most stable gene across different ages was h3a in adults and immature and ef1a for juveniles. In different tissues, the most stable gene was also h3a in gills and hepatopancreas, but actb was most stable in brain and kidney. As expected, the gene with the lowest stability was atp1a across different ages and tissues, except in hepatopancreas, where tuba exhibited the lowest stability (Table 4).
Table 4. Consensus stability ranking by RefFinder of the candidate reference genes in Odontesthes humensis exposed to Roundup®.
The h3a gene displayed the highest stability in different developmental stages in the seawater group. It was also the most stably expressed gene in the gills and hepatopancreas, while actb was the most stable in brain and kidney. The gene with the lowest stability across the ages and tissues was atp1a, except in hepatopancreas, where tuba exhibited the lowest stability in expression (Table 5).
Table 5. Consensus stability ranking by RefFinder of the candidate reference genes in Odontesthes humensis exposed to seawater.
In the control group, the consensus comprehensive ranking constructed by RefFinder was h3a (1.32) > ef1a (2.38) > actb (2.59) > 18s (3.34) > eif3g (4.12) > gapdh (5.63) > tuba (6.96) > atp1a (7.74) (Figure 6A). In the Roundup® group, the ranking order of stability was h3a (1.19) > actb (1.86) > 18s (3.50) > ef1a (3.66) > eif3g (3.72) > tuba (5.96) > gapdh (6.74) > atp1a (8.00) (Figure 6B). The consensus ranking of stability constructed based on the expression data of the seawater group was: h3a (1.19) > eif3g (2.06) > ef1a (3.13) > 18s (3.50) > actb (4.12) > tuba (5.96) > gapdh (6.44) > atp1a (8.00) (Figure 6C). The final consensus stability analysis, considering all methods and grouping the different tissues, ages, and treatments groups, revealed the following ranking order: h3a (1.19) > ef1a (2.63) > actb (2.99) > eif3g (3.22) > 18s (3.34) > gapdh (6.48) > tuba (6.48) > atp1a (8.00) (Figure 6D).
Figure 6. Consensus stability analysis of the candidates reference genes in Odontesthes humensis calculated by RefFinder algorithm. Gene expression stability in silversides exposed to water with quality parameters controlled (A); to Roundup® Transorb [10 mg.L−1 (acid equivalent) of glyphosate] (B); and to seawater (30 ppt) (C) across the different treatments for 24 h; and final consensus stability analysis between all methods and grouping the three treatments groups (D). The most stable genes are displayed on the left, and the least stable genes are displayed on the right of the x-axis.
In the present study, it was used individual methods (comparative dCt, geNorm, NormFinder, and BestKeeper) to obtain independent rankings of gene expression stability of candidate RGs in O. humensis and an algorithm that deduces a consensus ranking among all methods (RefFinder) such as presented in recent studies about RGs (Taki et al., 2014; Xu et al., 2014; Huang et al., 2015). Different algorithms can give different results depending of the set of analyzed genes and experimental variables (Yang et al., 2013; Zhang et al., 2013; Liu et al., 2014; Purohit et al., 2016) and no one statistical approach can cover all variables (Taki et al., 2014). For these reasons, the use of more than one algorithm is indicated. The different results presented by each algorithm are natural and expected due to their distinct statistical approach to construct the rankings (Liu et al., 2014). For example, the dCt method compares the relative expression of gene pairs with their SD values to get the most stable. GeNorm goes beyond, it calculates the M-value from the SD values and the geometric mean, and compares all gene pairs results. BestKeeper also starts from the SD values of gene expression and a geometric mean of the genes with the most stable Ct value are used to calculate the BestKeeper index. Then, it is calculated the Pearson's correlation coefficient ([r]) and the P-value to determine the similarity between the candidate RGs expression. The NormFinder is based in analysis of variance (ANOVA) and it is the unique of the used algorithms that evaluates the intragroup variation (i.e., replicates of a same treatment group) besides the intergroup variation (i.e., control vs. Roundup® or seawater groups), which also used by the other pairwise comparisons approaches, and combine both variations into a stability value. In this study, some differences were obtained in the ranking orders occupied by the genes in result of each algorithm analysis mainly from the second to the penultimate positions (Figures 2–5). Due to this, the use of the analysis by the ReFinder, which calculates the geometric mean for the previously obtained ranks, was important to a consensual unification of the discrepant results.
In the current study, the most stable gene across the treatment groups, independent of the method of analysis, including the final consensus RefFinder analysis, was h3a. This gene was also the most stable across different ages, except in the juveniles from the Roundup® group. However, in the consensus analysis of the tissues samples revealed h3a to be not as stable as in the age analysis. For example, the results of brain analysis demonstrated this gene not to be the most stable in all treatments. Moreover, h3a did not exhibit the highest stability in the kidney of Roundup® group. The h3a gene has already been evaluated in studies for validation of RGs showing high stability (Taylor et al., 2007; Koenigstein et al., 2013) and can be used to normalize qRT-PCR reactions (Ramachandra et al., 2008). However, up to now, no study has reported this gene as the candidate RG in a fish species. In the final consensus ranking, the second most stable gene was ef1a. All performed analysis revealed this gene to have high stability, occupying at least the third position of the constructed rankings, except in consensus ranking of the Roundup® group constructed by RefFinder, where it occupied the fourth position. A deviation in the ranking order of stability is natural owing to the use of different algorithms. Studies on stability of candidates RGs in different experimental protocols have revealed that ef1a is usually the most stable gene of fishes (Jorgensen et al., 2006; Tang et al., 2007, 2012; Infante et al., 2008; Liman et al., 2013; Hu et al., 2014).
The studies that evaluated the gene expression in the South American silversides have used actb as the internal control for qRT-PCR (Strobl-Mazzulla et al., 2005; Karube et al., 2007; Fernandino et al., 2008, 2011; Majhi et al., 2009; Miranda et al., 2009; Blasco et al., 2010; Gómez-Requeni et al., 2012; Pérez et al., 2012; González et al., 2015). In the present study, RefFinder analysis focused on the individual tissues revealing actb to be the most stable gene in brain and kidney from the Roundup® group and in the brain from the seawater group. Only the geNorm analysis of stability, based on control and Roundup® groups, revealed actb as the most stable gene. However, our results showed that actb was not the most stable gene. The RefFinder final consensus comprehensive ranking pointed this gene as the third most stable in O. humensis. There is still much debate on the use of actb to normalize qRT-PCR reactions in fishes. Some authors have reported that this gene is the least stable in some fish species (Jorgensen et al., 2006; Filby and Tyler, 2007; Yang et al., 2013; Hu et al., 2014; Chapman and Waldenström, 2015). However, the studies that present actb as a good RG have also reported that its high stability in fishes is tissue dependent within a species (Zheng and Sun, 2011; Zhang et al., 2013; Sun and Hu, 2015), such as in O. humensis. This does not mean that the studies using actb as the RG should not be considered. However, future studies should pay more attention to the choice of RG.
The 18s gene has been a subject of discussions on its use as the RG in fish models. This gene has been shown as the most or to be among the most stable candidates in some fish species (Jorgensen et al., 2006; Filby and Tyler, 2007; Tang et al., 2007; Small et al., 2008; Yang et al., 2013; Liu et al., 2014). However, in other species, this gene is considered unsuitable for use as an internal control for qRT-PCR (Infante et al., 2008; Hu et al., 2014; Purohit et al., 2016). In O. humensis from control, Roundup®, and seawater groups, and across different ages and tissues, 18s generally held the intermediary position in the rankings, attracting little attention for its use in normalization methods. The gapdh gene, as well as actb and 18s, has presented discrepant results in the literature that reports its expression stability. The gapdh has already been reported as one of the most stable genes in half-smooth tongue sole (Liu et al., 2014). Furthermore, its expression is usually stable depending on the evaluated tissue, being able to occupy both extremities of rankings of stability (Yang et al., 2013; Zhang et al., 2013). So, generally, gapdh is refuted as a suitable gene for an efficient normalization (Jorgensen et al., 2006; Filby and Tyler, 2007; Tang et al., 2007, 2012; Infante et al., 2008; Small et al., 2008; Ahi et al., 2013; Liman et al., 2013; Hu et al., 2014; Chapman and Waldenström, 2015). In the present study, gapdh appeared to be the second most unstable gene, only after atp1a gene.
The tuba gene usually presents high stability in studies on validation of candidates RGs. This gene was the second most stable after analysis by dCt and NormFinder in Nile tilapia under normal conditions (Yang et al., 2013). This gene also exhibited high stability in intestine and liver from Japanese flounder at 24 and 72 h after viral infection, respectively, as well as in muscle of turbot after 72 h post-infection (Zhang et al., 2013). Even with tuba gene presenting higher stability in the brain of silversides from the control group, this gene did not maintain this expression pattern. In the brain of silversides from other treatment groups, tuba had low stability levels. Across other tissues and different developmental stages, the tuba gene frequently occupied the last places of the final ranking, even behind atp1a, as well as across treatment groups and different algorithms used. The final consensus comprehensive ranking constructed by RefFinder presented tuba as the most unstable gene in O. humensis, disregarding atp1a. Finally, the control gene atp1a, despite being a constitutive gene, was the most unstable, as expected. It occupied the last position in the rankings of stability constructed by all algorithms used to compare the three treatment groups. This gene also exhibited lower stability across different ages and tissues from silversides from all treatments, except in the hepatopancreas of the Roundup® and seawater groups.
In the present study, a set of candidates of RGs was analyzed for the first time in O. humensis. The genes atp1a, tuba, gapdh, and 18s were the least stable and highly unsuitable to be used for qRT-PCR normalization in studies in silverside O. humensis. The three most stable genes were h3a, ef1a, and actb. The discordance observed among the results of analyses of the candidate RGs in different fish species, tissues, developmental stages, and experimental conditions, it is evident the importance of identification of efficient RGs for each experimental design instead of the indiscriminate use of traditional genes. Furthermore, the gene h3a exhibited a greater potential to be used as a RG in O. humensis in comparison with other traditional genes, such as actb and ef1a. Perhaps the h3a gene had the same potential to be used as the best RG in other fish species, since the stability of this gene has never been evaluated in other fishes. Therefore, future studies warrant the evaluation of h3a as candidate RG in other fish species, besides O. humensis or silversides.
TS and VC are responsible for experimental design, data analysis, manuscript writing. TS, MR, and RR were responsible for fish acclimation and maintenance. TS, BB, IL, LS, MR, RR, and WD were responsible for the biological collections. TS, BB, IL, LS, WD, and VC were responsible for the molecular biology, from RNA extraction to sequencing and qRT-PCR analysis. TC and FS were also responsible for qRT-PCR analysis. AV, CC, and DM were responsible for the flow-cytometry analysis.
This study was supported by the Ministério da Ciência, Tecnologia e Inovação/Conselho Nacional de Desenvolvimento Científico e Tecnológico (Edital Universal #472210/2013-0 and #422292/2016-8) and Coordenação de Aperfeiçoamento de Pessoal de Nível Superior (AUXPE 2900/2014). TS, WD, MR, and DM are individually supported by Coordenação de Aperfeiçoamento de Pessoal de Nível Superior. AV, CC, TC, FS, RR, and VC are also individually supported by Conselho Nacional de Desenvolvimento Científico e Tecnológico.
The authors declare that the research was conducted in the absence of any commercial or financial relationships that could be construed as a potential conflict of interest.
We are greatly thankful to Mr. Nilton Link and Mr. Vítor Colvara for the help in the maintenance of animals.
The Supplementary Material for this article can be found online at: https://www.frontiersin.org/articles/10.3389/fgene.2018.00075/full#supplementary-material
RG, reference gene.
Ahi, E. P., Guðbrandsson, J., Kapralova, K. H., Franzdóttir, S. R., Snorrason, S. S., Maier, V. H., et al. (2013). Validation of reference genes for expression studies during craniofacial development in arctic charr. PLoS ONE 8:e66389. doi: 10.1371/journal.pone.0066389
Andersen, C. L., Jensen, J. L., and Ørntoft, T. F. (2004). Normalization of real-time quantitative reverse transcription-PCR data: a model-based variance estimation approach to identify genes suited for normalization, applied to bladder and colon cancer data sets. Cancer Res. 64, 5245–5250. doi: 10.1158/0008-5472.CAN-04-0496
Armesto, P., Campinho, M. A., Rodríguez-Rúa, A., Cousin, X., Power, D. M., Manchado, M., et al. (2014). Molecular characterization and transcriptional regulation of the Na+/K+ ATPase α subunit isoforms during development and salinity challenge in a teleost fish, the Senegalese sole (Solea senegalensis). Comp. Biochem. Physiol. B Biochem. Mol. Biol. 175, 23–38. doi: 10.1016/j.cbpb.2014.06.004
Bemvenuti, M. A. (2006). Silversides in South Brazil: morphological and ecological aspects. Biocell 30, 111–118.
Blasco, M., Fernandino, J. I., Guilgur, L. G., Strüssmann, C. A., Somoza, G. M., and Vizziano-Cantonnet, D. (2010). Molecular characterization of cyp11a1 and cyp11b1 and their gene expression profile in pejerrey (Odontesthes bonariensis) during early gonadal development. Comp. Biochem. Physiol. A Mol. Integr. Physiol. 156, 110–118. doi: 10.1016/j.cbpa.2010.01.006
Bloom, D. D., Weir, J. T., Piller, K. R., and Lovejoy, N. R. (2013). Do freshwater fishes diversify faster than marine fishes? A test using state-dependent diversification analyses and molecular phylogenetics of New World silversides (Atherinopsidae). Evolution 67, 2040–2057. doi: 10.1111/evo.12074
Bustin, S. A. (2002). Quantification of mRNA using real-time reverse transcription PCR (RT-PCR): trends and problems. J. Mol. Endocrinol. 29, 23–39. doi: 10.1677/jme.0.0290023
Carriquiriborde, P., and Ronco, A. (2006). Ecotoxicological studies on the pejerrey (Odontesthes bonariensis, Pisces Atherinopsidae). Biocell 30, 97–109.
Chapman, J. R., and Waldenström, J. (2015). With reference to reference genes: a systematic review of endogenous controls in gene expression studies. PLoS ONE 10:e0141853. doi: 10.1371/journal.pone.0141853
Choi, C. Y., and An, K. W. (2008). Cloning and expression of Na+/K+-ATPase and osmotic stress transcription factor 1 mRNA in black porgy, Acanthopagrus schlegeli during osmotic stress. Comp. Biochem. Physiol. B Biochem. Mol. Biol. 149, 91–100. doi: 10.1016/j.cbpb.2007.08.009
Dheda, K., Huggett, J. F., Bustin, S. A., Johnson, M. A., Rook, G., and Zumla, A. (2004). Validation of housekeeping genes for normalizing RNA expression in real-time PCR. Biotechniques 37, 112–119.
Dyer, B. S. (2006). Systematic revision of the South American silversides (Teleostei, Atheriniformes). Biocell 30, 69–88.
Fernandino, J. I., Hattori, R. S., Kimura, H., Strüssmann, C. A., and Somoza, G. M. (2008). Expression profile and estrogenic regulation of anti-müllerian hormone during gonadal development in pejerrey Odontesthes bonariensis, a teleost fish with strong temperature-dependent sex determination. Dev. Dyn. 237, 3192–3199. doi: 10.1002/dvdy.21731
Fernandino, J. I., Popesku, J. T., Paul-Prasanth, B., Xiong, H., Hattori, R. S., Oura, M., et al. (2011). Analysis of sexually dimorphic expression of genes at early gonadogenesis of pejerrey Odontesthes bonariensis using a heterologous microarray. Sex Dev. 5, 89–101. doi: 10.1159/000324423
Filby, A. L., and Tyler, C. R. (2007). Appropriate “housekeeping” genes for use in expression profiling the effects of environmental estrogens in fish. BMC Mol. Biol. 8:10. doi: 10.1186/1471-2199-8-10
Gómez-Requeni, P., Kraemer, M. N., and Canosa, L. F. (2012). Regulation of somatic growth and gene expression of the GH-IGF system and PRP-PACAP by dietary lipid level in early juveniles of a teleost fish, the pejerrey (Odontesthes bonariensis). J. Comp. Physiol. B Biochem. Syst. Environ. Physiol. 182, 517–530. doi: 10.1007/s00360-011-0640-9
González, A., Fernandino, J. I., and Somoza, G. M. (2015). Effects of 5α-dihydrotestosterone on expression of genes related to steroidogenesis and spermatogenesis during the sex determination and differentiation periods of the pejerrey, Odontesthes bonariensis. Comp. Biochem. Physiol. A Mol. Integr. Physiol. 182, 1–7. doi: 10.1016/j.cbpa.2014.12.003
Harayashiki, C. A., Varela Junior, A. S., Machado, A. A., Cabrera, L. C., Primel, E. G., Bianchini, A., et al. (2013). Toxic effects of the herbicide Roundup in the guppy Poecilia vivipara acclimated to fresh water. Aquat. Toxicol. 142–143, 176–184. doi: 10.1016/j.aquatox.2013.08.006
Hiroi, J., and McCormick, S. D. (2012). New insights into gill ionocyte and ion transporter function in euryhaline and diadromous fish. Respir. Physiol. Neurobiol. 184, 257–268. doi: 10.1016/j.resp.2012.07.019
Hsu, H. H., Lin, L. Y., Tseng, Y. C., Horng, J. L., and Hwang, P. P. (2014). A new model for fish ion regulation: identification of ionocytes in freshwater- and seawater-acclimated medaka (Oryzias latipes). Cell Tissue Res. 357, 225–243. doi: 10.1007/s00441-014-1883-z
Hu, Q., Guo, W., Gao, Y., Tang, R., and Li, D. (2014). Reference gene selection for real-time RT-PCR normalization in rice field eel (Monopterus albus) during gonad development. Fish Physiol. Biochem. 40, 1721–1730. doi: 10.1007/s10695-014-9962-3
Huang, X., Gao, Y., Jiang, B., Zhou, Z., and Zhan, A. (2015). Reference gene selection for quantitative gene expression studies during biological invasions: a test on multiple genes and tissues in a model ascidian Ciona savignyi. Gene 576, 79–87. doi: 10.1016/j.gene.2015.09.066
Hughes, L. C., Somoza, G. M., Nguyen, B. N., Bernot, J. P., González-Castro, M., Díaz de Astarloa, J. M., et al. (2017). Transcriptomic differentiation underlying marine-to-freshwater transitions in the South American silversides Odontesthes argentinensis and O. bonariensis (Atheriniformes). Ecol. Evol. 7, 1–11. doi: 10.1002/ece3.3133
Infante, C., Matsuoka, M. P., Asensio, E., Cañavate, J. P., Reith, M., and Manchado, M. (2008). Selection of housekeeping genes for gene expression studies in larvae from flatfish using real-time PCR. BMC Mol. Biol. 9:28. doi: 10.1186/1471-2199-9-28
Jorgensen, S. M., Kleveland, E. J., Grimholt, U., and Gjoen, T. (2006). Validation of reference genes for real-time polymerase chain reaction studies in Atlantic salmon. Mar. Biotechnol. 8, 398–408. doi: 10.1007/s10126-005-5164-4
Karube, M., Fernandino, J. I., Strobl-Mazzulla, P., Strüssmann, C. A., Yoshzaki, G., Somoza, G. M., et al. (2007). Characterization and expression profile of the ovarian cytochrome P-450 aromatase (cyp19A1) gene during thermolabile sex determination in pejerrey, Odontesthes bonariensis. J. Exp. Zool. A Ecol. Genet. Physiol. 307, 625–636. doi: 10.1002/jez.416
Koenigstein, S., Pöhlmann, K., Held, C., and Abele, D. (2013). Ecological comparison of cellular stress responses among populations – normalizing RT-qPCR values to investigate differential environmental adaptations. BMC Ecol. 13:21. doi: 10.1186/1472-6785-13-21
Liman, M., Wenji, W., Conghui, L., Haiyang, Y., Zhigang, W., Xubo, W., et al. (2013). Selection of reference genes for reverse transcription quantitative real-time PCR normalization in black rockfish (Sebastes schlegeli). Mar. Genomics 11, 67–73. doi: 10.1016/j.margen.2013.08.002
Lin, C. H., Huang, C. L., Yang, C. H., Lee, T. H., and Hwang, P. P. (2004). Time-course changes in the expression of Na, K-ATPase and the morphometry of mitochondrion-rich cells in gills of euryhaline tilapia (Oreochromis mossambicus) during freshwater acclimation. J. Exp. Zool. A Comp. Exp. Biol. 301, 85–96. doi: 10.1002/jez.a.20007
Liu, C., Xin, N., Zhai, Y., Jiang, L., Zhai, J., Zhang, Q., et al. (2014). Reference gene selection for quantitative real-time RT-PCR normalization in the half-smooth tongue sole (Cynoglossus semilaevis) at different developmental stages, in various tissue types and on exposure to chemicals. PLoS ONE 9:e91715. doi: 10.1371/journal.pone.0091715
Livak, K. J., and Schmittgen, T. D. (2001). Analysis of relative gene expression data using real-time quantitative PCR and the 2−ΔΔCT method. Methods 25, 402–408. doi: 10.1006/meth.2001.1262
Majhi, S. K., Hattori, R. S., Rahman, S. M., Suzuki, T., and Strüssmann, C. A. (2009). Experimentally induced depletion of germ cells in sub-adult Patagonian pejerrey (Odontesthes hatcheri). Theriogenology 71, 1162–1172. doi: 10.1016/j.theriogenology.2008.12.008
Menone, M. L., Aizpún de Moreno, J. E., Moreno, V. J., Lanfranchi, A. L., Metcalfe, T. L., and Metcalfe, C. D. (2000). Environmental contamination and toxicology PCBs and organochlorines in tissues of silverside (Odontesthes bonariensis) from a coastal lagoon in Argentina. Arch. Environ. Contam. Toxicol. 38, 202–208. doi: 10.1007/s002449910027
Miranda, L. A., Strüssmann, C. A., and Somoza, G. M. (2009). Effects of light and temperature conditions on the expression of GnRH and GtH genes and levels of plasma steroids in Odontesthes bonariensis females. Fish Physiol. Biochem. 35, 101–108. doi: 10.1007/s10695-008-9232-3
Nawata, C. M., Hirose, S., Nakada, T., Wood, C. M., and Kato, A. (2010). Rh glycoprotein expression is modulated in pufferfish (Takifugu rubripes) during high environmental ammonia exposure. J. Exp. Biol. 213, 3150–3160. doi: 10.1242/jeb.044719
Nilsen, T. O., Ebbesson, L. O., Madsen, S. S., McCormick, S. D., Andersson, E., Björnsson, B. T., et al. (2007). Differential expression of gill Na+, K+-ATPase alpha- and beta-subunits, Na+, K+, 2Cl− cotransporter and CFTR anion channel in juvenile anadromous and landlocked Atlantic salmon Salmo salar. J. Exp. Biol. 210, 2885–2896. doi: 10.1242/jeb.002873
Patterson, J., Bodinier, C., and Green, C. (2012). Effects of low salinity media on growth, condition, and gill ion transporter expression in juvenile Gulf killifish, Fundulus grandis. Comp. Biochem. Physiol. A Mol. Integr. Physiol. 161, 415–421. doi: 10.1016/j.cbpa.2011.12.019
Pérez, M. R., Fernandino, J. I., Carriquiriborde, P., and Somoza, G. M. (2012). Feminization and altered gonadal gene expression profile by ethinylestradiol exposure to pejerrey, Odontesthes bonariensis, a South American teleost fish. Environ. Toxicol. Chem. 31, 941–946. doi: 10.1002/etc.1789
Petrunkina, A. M., Volker, G., Weitze, K. F., Beyerbach, M., Töpfer-Petersen, E., and Waberski, D. (2005). Detection of cooling-induced membrane changes in the response of boar sperm to capacitating conditions. Theriogenology 63, 2278–2299. doi: 10.1016/j.theriogenology.2004.10.008
Pfaffl, M. W., Tichopad, A., Prgomet, C., and Neuvians, T. P. (2004). Determination of stable housekeeping genes, differentially regulated target genes and sample integrity: bestKeeper – excel-based tool using pair-wise correlations. Biotechnol. Lett. 26, 509–515. doi: 10.1023/B:BILE.0000019559.84305.47
Piedras, S. R. N., Fernandes, J. L. O., Motoyama, I. S., and Martins, G. B. (2009). Efeito de diferentes concentrações de salinas (NaCl) na sobrevivência de embriões de peixe – rei Odontesthes bonariensis e Odontesthes humensis. Biotemas 22, 235–238. doi: 10.5007/2175-7925.2009v22n3p235
Purohit, G. K., Mahanty, A., Mohanty, B. P., and Mohanty, S. (2016). Evaluation of housekeeping genes as references for quantitative real-time PCR analysis of gene expression in the murrel Channa striatus under high-temperature stress. Fish Physiol. Biochem. 42, 125–135. doi: 10.1007/s10695-015-0123-0
Ramachandra, R. K., Salem, M., Gahr, S., Rexroad, C. E. III., and Yao, J. (2008). Cloning and characterization of microRNAs from rainbow trout (Oncorhynchus mykiss): Their expression during early embryonic development. BMC Dev. Biol. 8:41. doi: 10.1186/1471-213X-8-41
Scott, G. R., Richards, J. G., Forbush, B., Isenring, P., and Schulte, P. M. (2004). Changes in gene expression in gills of the euryhaline killifish Fundulus heteroclitus after abrupt salinity transfer. Am. J. Cell Physiol. 287, C300–C309. doi: 10.1152/ajpcell.00054.2004
Shiogiri, N. S., Paulino, M. G., Carraschi, S. P., Baraldi, F. G., da Cruz, C., and Fernandes, M. N. (2012). Acute exposure of a glyphosate-based herbicide affects the gills and liver of the Neotropical fish, Piaractus mesopotamicus. Environ. Toxicol. Pharmacol. 34, 388–396. doi: 10.1016/j.etap.2012.05.007
Silver, N., Best, S., Jiang, J., and Thein, S. L. (2006). Selection of housekeeping genes for gene expression studies in human reticulocytes using real-time PCR. BMC Mol. Biol. 7:33. doi: 10.1186/1471-2199-7-33
Sinha, A. K., Dasan, A. F., Rasoloniriana, R., Pipralia, N., Blust, R., and De Boeck, G. (2015). Hypo-osmotic stress-induced physiological and ion-osmoregulatory responses in European sea bass (Dicentrarchus labrax) are modulated differentially by nutritional status. Comp. Biochem. Physiol. A Mol. Integr. Physiol. 181, 87–99. doi: 10.1016/j.cbpa.2014.11.024
Silveira, T. L. R., Martins, G. B., Domingues, W. B., Remião, M. H., Barreto, B. F., Lessa, I. M., et al. (2018). Gene and blood analysis reveal that transfer from brackish water to freshwater is less stressful to the silverside Odontesthes humensis. Front. Genet. 9:28. doi: 10.3389/fgene.2018.00028
Small, B. C., Murdock, C. A., Bilodeau-Bourgeois, A. L., Peterson, B. C., and Waldbieser, G. C. (2008). Stability of reference genes for real-time PCR analyses in channel catfish (Ictalurus punctatus) tissues under varying physiological conditions. Comp. Biochem. Physiol. B Biochem. Mol. Biol. 151, 296–304. doi: 10.1016/j.cbpb.2008.07.010
Somoza, G. M., Miranda, L. A., Berasain, G. E., Colautti, D., Remes Lenicov, M., and Strüssmann, C. A. (2008). Historical aspects, current status and prospects of pejerrey aquaculture in South America. Aquac. Res. 39, 784–793. doi: 10.1111/j.1365-2109.2008.01930.x
Strobl-Mazzulla, P. H., Moncaut, N. P., López, G. C., Miranda, L. A., Canario, A. V., and Somoza, G. M. (2005). Brain aromatase from pejerrey fish (Odontesthes bonariensis): cDNA cloning, tissue expression, and immunohistochemical localization. Gen. Comp. Endocrinol. 143, 21–32. doi: 10.1016/j.ygcen.2005.02.026
Sun, B. G., and Hu, Y. H. (2015). Evaluation of potential internal references for quantitative real-time RT-PCR normalization of gene expression in red drum (Sciaenops ocellatus). Fish Physiol. Biochem. 41, 695–704. doi: 10.1007/s10695-015-0039-8
Taki, F. A., Abdel-Rahman, A. A., and Zhang, B. (2014). A comprehensive approach to identify reliable reference gene candidates to investigate the link between alcoholism and endocrinology in Sprague-Dawley rats. PLoS ONE 9:e94311. doi: 10.1371/journal.pone.0094311
Tang, R., Dodd, A., Lai, D., McNabb, W. C., and Love, D. R. (2007). Validation of zebrafish (Danio rerio) reference genes for quantitative real-time RT-PCR normalization. Acta Biochim. Biophys. Sin. (Shanghai) 39, 384–390. doi: 10.1111/j.1745-7270.2007.00283.x
Tang, Y. K., Yu, J. H., Xu, P., Li, J. L., Li, H. X., and Ren, H. T. (2012). Identification of housekeeping genes suitable for gene expression analysis in Jian carp (Cyprinus carpio var. jian). Fish Shellfish Immunol. 33, 775–779. doi: 10.1016/j.fsi.2012.06.027
Taylor, D. L., Thomson, P. C., de Silva, K., and Whittington, R. J. (2007). Validation of endogenous reference genes for expression profiling of RAW264.7 cells infected with Mycobacterium avium subsp. paratuberculosis by quantitative PCR. Vet. Immunol. Immunopathol. 115, 43–55. doi: 10.1016/j.vetimm.2006.10.007
Tipsmark, C. K., Madsen, S. S., and Borski, R. J. (2004). Effect of salinity on expression of branchial ion transporters in striped bass (Morone saxatilis). J. Exp. Zool. A Comp. Exp. Biol. 301, 979–991. doi: 10.1002/jez.a.119
Tomy, S., Chang, Y. M., Chen, Y. H., Cao, J. C., Wang, T. P., and Chang, C. F. (2009). Salinity effects on the expression of osmoregulatory genes in the euryhaline black porgy Acanthopagrus schlegeli. Gen. Comp. Endocrinol. 161, 123–132. doi: 10.1016/j.ygcen.2008.12.003
Tsuzuki, M. Y., Ogawa, K., Strüssmann, C. A., Maita, M., Takashima, F., and Melo, C. M. R. (2007). The significance of cortisol on acclimation to salinity in pejerrey Odontesthes bonariensis. Arq. Bras. Med. Vet. Zootec. 59, 1301–1307. doi: 10.1590/S0102-09352007000500030
Vandesompele, J., De Preter, K., Pattyn, F., Poppe, B., Van Roy, N., De Paepe, A., et al. (2002). Accurate normalization of real-time quantitative RT -PCR data by geometric averaging of multiple internal control genes. Genome Biol. 3, 1–12. doi: 10.1186/gb-2002-3-7-research0034
Velasques, R. R., Sandrini, J. Z., and da Rosa, C. E. (2016). Roundup in zebrafish: effects on oxidative status and gene expression. Zebrafish 13, 432–441. doi: 10.1089/zeb.2016.1259
Wang, G., Yang, E., Smith, K. J., Zeng, Y., Ji, G., Connon, R., et al. (2014). Gene expression responses of threespine stickleback to salinity: implications for salt-sensitive hypertension. Front. Genet. 5:312. doi: 10.3389/fgene.2014.00312
Xu, X. Y., Shen, Y. B., Fu, J. J., Lu, L. U., and Li, J. L. (2014). Determination of reference microRNAs for relative quantification in grass carp (Ctenopharyngodon idella). Fish Shellfish Immunol. 36, 374–382. doi: 10.1016/j.fsi.2013.12.007
Yang, C. G., Wang, X. L., Tian, J., Liu, W., Wu, F., Jiang, M., et al. (2013). Evaluation of reference genes for quantitative real-time RT-PCR analysis of gene expression in Nile tilapia (Oreochromis niloticus). Gene 527, 183–192. doi: 10.1016/j.gene.2013.06.013
Zebral, Y. D., Costa, P. G., de Castro Knopp, B., Lansini, L. R., Zafalon-Silva, B., Bianchini, A., et al. (2017). Effects of a glyphosate-based herbicide in pejerrey Odontesthes humensis embryonic development. Chemosphere 185, 860–867. doi: 10.1016/j.chemosphere.2017.07.069
Zhang, J., Hu, Y., Sun, B., Xiao, Z., and Sun, L. (2013). Selection of normalization factors for quantitative real time RT-PCR studies in Japanese flounder (Paralichthys olivaceus) and turbot (Scophthalmus maximus) under conditions of viral infection. Vet. Immunol. Immunopathol. 152, 303–316. doi: 10.1016/j.vetimm.2012.12.018
Keywords: algorithm, expression, fish, gene, normalization, real time PCR, sequencing, validation
Citation: Silveira TLR, Domingues WB, Remião MH, Santos L, Barreto B, Lessa IM, Varela Junior AS, Martins Pires D, Corcini C, Collares T, Seixas FK, Robaldo RB and Campos VF (2018) Evaluation of Reference Genes to Analyze Gene Expression in Silverside Odontesthes humensis Under Different Environmental Conditions. Front. Genet. 9:75. doi: 10.3389/fgene.2018.00075
Received: 23 October 2017; Accepted: 19 February 2018;
Published: 14 March 2018.
Edited by:
Rodrigo A. Torres, Universidade Federal de Pernambuco, BrazilReviewed by:
Bruno Cavalheiro Araújo, University of Mogi das Cruzes, BrazilCopyright © 2018 Silveira, Domingues, Remião, Santos, Barreto, Lessa, Varela Junior, Martins Pires, Corcini, Collares, Seixas, Robaldo and Campos. This is an open-access article distributed under the terms of the Creative Commons Attribution License (CC BY). The use, distribution or reproduction in other forums is permitted, provided the original author(s) and the copyright owner are credited and that the original publication in this journal is cited, in accordance with accepted academic practice. No use, distribution or reproduction is permitted which does not comply with these terms.
*Correspondence: Vinicius F. Campos, ZmFyaWFzY2FtcG9zQGdtYWlsLmNvbQ==
Disclaimer: All claims expressed in this article are solely those of the authors and do not necessarily represent those of their affiliated organizations, or those of the publisher, the editors and the reviewers. Any product that may be evaluated in this article or claim that may be made by its manufacturer is not guaranteed or endorsed by the publisher.
Research integrity at Frontiers
Learn more about the work of our research integrity team to safeguard the quality of each article we publish.