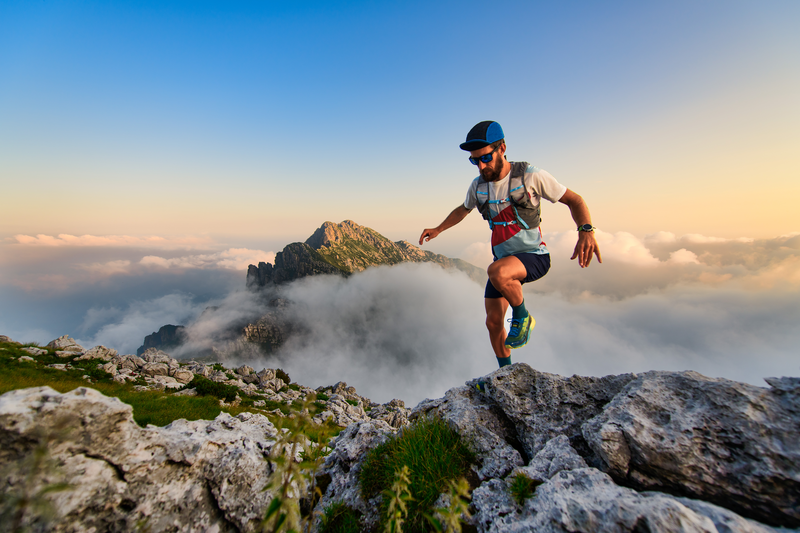
95% of researchers rate our articles as excellent or good
Learn more about the work of our research integrity team to safeguard the quality of each article we publish.
Find out more
MINI REVIEW article
Front. Genet. , 04 November 2014
Sec. Pharmacogenetics and Pharmacogenomics
Volume 5 - 2014 | https://doi.org/10.3389/fgene.2014.00383
This article is part of the Research Topic Improving cancer chemotherapy through pharmacogenomics View all 6 articles
NF-E2-related factor 2 (NRF2) is a transcription factor that controls the expression of a variety of antioxidant and detoxification genes. Accumulating evidence strongly suggests that NRF2 mediates cancer cell proliferation and drug resistance, as well. Single nucleotide polymorphism (SNP) -617C > A in the anti-oxidant response element-like loci of the human NRF2 gene play a pivotal role in the positive feedback loop of transcriptional activation of the NRF2 gene. Since the SNP (-617A) reportedly decreases the binding affinity to the transcription factors of NRF2/small multiple alignment format (MafK), the homozygous -617A/A allele may attenuate the positive feedback loop of transcriptional activation of the NRF2 gene and reduce the NRF2 protein level. As the consequence, cancer cells are considered to become more sensitive to therapy and less aggressive than cancer cells harboring the -617C (WT) allele. Indeed, Japanese lung cancer patients carrying SNP homozygous alleles (c. -617A/A) exhibited remarkable survival over 1,700 days after surgical operation (log-rank p = 0.021). The genetic polymorphism in the human NRF2 gene is considered as one of prognosis markers for cancer therapy.
In the field of cancer chemotherapy, it has been well documented that glutathione (GSH) plays a pivotal role in conferring cancer cells resistance to anti-tumor drugs, such as cisplatin and alkylating agents. Several lines of evidence suggest that certain multi-drug efflux pumps encoded by ATP-binding cassette (ABC) transporter genes are up-regulated by oxidative stress and/or chemotherapeutic agents to contribute to multi-drug resistance of cancer cells. About two decades ago, Ishikawa and Kuo first reported that many cytotoxic agents induced the expression of both γ-glutamylcysteine synthetase (γ-GCS) and ABCC1 (MRP1) genes (Ishikawa et al., 1996; Kuo et al., 1996, 1998; Gomi et al., 1997; Yamane et al., 1998). Coordinated up-regulation of both γ-GCS and ABCC1 genes was found in human malignant tissues. Among 32 cases of human colorectal cancer biopsies, 78% of the cases exhibited co-elevated expression of ABCC1 and γ-GCS genes in tumor samples as compared with their corresponding adjacent naïve normal samples (Kuo et al., 1996). At that time, it was speculated that a common transcriptional regulator might exist for the coordinated expression of both γ-GCS and ABCC1 genes (Ishikawa et al., 1996).
During the past two decades, evidence has accumulated to show that one transcription factor named NF-E2-related factor 2 (NRF2) is a common redox regulator to control cellular adaptation/protection to external stimuli by inducing antioxidant and detoxification genes (Motohashi and Yamamoto, 2004; Kobayashi and Yamamoto, 2006; Nguyen et al., 2009). In fact, NRF2 is a major player in the transcriptional upregulation of many target genes in phase II drug metabolizing enzymes and certain phase III ABC transporters (ABCC2, ABCC3, and ABCG2; Adachi et al., 2007). The 5′-flanking region of many of phase II xenobiotic detoxifying genes (e.g., γ-GCS) contains an antioxidant response element (ARE). NRF2 directly binds to the ARE sequence in those target genes (Shen and Kong, 2009; Singh et al., 2010). Furthermore, it has recently been reported NRF2 mediates cancer cell proliferation and drug resistance (Lau et al., 2008; Hayes and McMahon, 2009; Homma et al., 2009; Taguchi et al., 2011; Sporn and Liby, 2012; Yamadori et al., 2012; Shelton and Jaiswal, 2013).
NF-E2-related factor 2 is a “cap‘n’collar” basic region-leucine zipper (CNC-bZip) transcription factor involved in the induction of ARE-regulated genes (Moi et al., 1994; Motohashi and Yamamoto, 2004; Kobayashi and Yamamoto, 2006; Lau et al., 2008; Hayes and McMahon, 2009; Homma et al., 2009; Nguyen et al., 2009; Taguchi et al., 2011; Sporn and Liby, 2012; Yamadori et al., 2012). Under non-stressed conditions, NRF2 protein is associated with Kelch-like ECH associating protein 1 (KEAP1; Itoh et al., 1999) that negatively regulate NRF2 by retrieving the NRF2 protein in the cytoplasmic compartment. However, oxidative stress and/or electrophilic attack modifies the KEAP1 protein, which leads to dissociation of NRF2 from KEAP1. The NRF2 protein, thus released, is subsequently translocated into the nucleus. Coupling with small multiple alignment format (MAF) sequences, NRF2 binds to ARE sequences (Itoh et al., 1995). Many genes encoding detoxifying and antioxidant enzymes have been found to be regulated by the NRF2 protein in this manner (Itoh et al., 1995; Ishii et al., 2000; Ramos-Gomez et al., 2001; Motohashi and Yamamoto, 2004; Cho et al., 2005; Kobayashi and Yamamoto, 2006; Nguyen et al., 2009). Recent studies, on the other hand, have shown that NRF2 contributes to cancer cell proliferation, drug resistance, and metabolic re-programming, as well (Kwak et al., 2002; Lau et al., 2008; Hayes and McMahon, 2009; Homma et al., 2009; Taguchi et al., 2011; Mitsuishi et al., 2012; Sporn and Liby, 2012; Yamadori et al., 2012). In this context, the NRF2 gene is regarded as a “double-edged sword,” namely, protection of normal cells and progression of cancer malignancy.
Yamamoto et al. (2004) first reported three single nucleotide polymorphisms (SNPs; -653A > G, -651G > A, and -617C > A) and one triplet repeat polymorphism in the regulatory region of the human NRF2 gene. The physiological significance of these SNPs was not known at that time. Three years later, Marzec et al. (2007) reported the impact of those SNPs on the regulation of NRF2 gene expression. In fact, the -617C > A SNP significantly affected basal NRF2 protein levels in vitro (Marzec et al., 2007). Moreover, the SNP -617C > A was found to be associated with a higher risk of oxidant-induced acute lung injury in humans (Marzec et al., 2007). These findings suggest that the SNP (-617C > A) in the ARE-like loci of the human NRF2 gene is important for self-induction of the NRF2 gene (Okano et al., 2013); refer to schematic illustrations in Figure 1.
FIGURE 1. Schematic illustrations showing the effect of NRF2 SNP -617C > A and MDM2 SNP c. 309 T > G on the p53-mediated suppression of cancer cell proliferation (A) and ABCG2-mediated drug resistance to gefitinib (B). Refer to Okano et al. (2013) for more details.
NF-E2-related factor 2 plays a pivotal role in protecting normal cells from external toxic challenges and oxidative stress, whereas it can modulate the cancer phenotype (Figure 1A) and also endow cancer cells resistance to anticancer drugs (Figure 1B). NRF2 activation appears to be associated with the emergence of cancer resistance to various anticancer drugs by transcriptionally activating a battery of self-defense genes. Indeed, NRF2 can induction the expression of γ-GCS and ABCC1 genes involved cancer cell resistance to cisplatin and alkylating agents (Ishikawa et al., 1996; Adachi et al., 2007). In addition, ABCG2 is known to mediate the efflux of gefitinib (Iressa) from cancer cells (Saito et al., 2006), and its expression is regulated by NRF2 (Singh et al., 2010) and the EGFR-tyrosine kinase cascade (Meyer zu Schwabedissen et al., 2006; Huang et al., 2011; Figure 1B).
Single nucleotide polymorphism -617C > A could affect the positive feedback loop of transcriptional activation of the NRF2 gene, and thereby it can regulate the NRF2 protein level. It is proposed that the homozygote -617A/A significantly attenuates the positive feedback loop of transcriptional activation of the NRF2 gene. Interestingly, Asians, including Japanese, have higher frequencies of the -617A allele as compared with African–Americans and Caucasians (Okano et al., 2013). As demonstrated in Figure 2A, Japanese lung cancer patients carrying SNP homozygous alleles (c.-617A/A) exhibited remarkable survival over 1,700 days after surgical operation (log-rank p = 0.021). This SNP is considered as a new biomarker for prognosis of lung cancer in Japanese population, and a hypothetical molecular mechanism has been proposed (Okano et al., 2013).
FIGURE 2. Kaplan-Meier plots showing the overall survival of patients harboring the WT homozygote (-617C/C), WT/SNP heterozygote (-617C/A), or SNP homozygote (-617A/A) in the NRF2 gene (A) and genotyping of NRF2 SNP -617C > A by the rapid SNP-detection method (B, upper panels) and DNA sequence analysis (B, lower panels). Data from Okano et al. (2013).
The genetic polymorphisms are the “intrinsic” mechanism, whereas the mutations are the “acquired” mechanism in cancer cells. Hitherto, several mutations in the NRF2 and KEAP1 genes have been reported in carcinomas of the lung (Sporn and Liby, 2012), liver (Yoo et al., 2012), stomach (Yoo et al., 2012), and breast (Sjöblom et al., 2006). Abnormalities in NRF2 activity were correlated with poor prognosis in terms of either recurrence-free or overall 5-year survival. Increased expression of NRF2 protein and decreased expression of KEAP1 protein were often observed as common abnormalities in non-small cell lung cancer (NSCLC), being associated with poor prognosis (Solis et al., 2010).
Identification and validation of biomarkers for personalized cancer therapy is one of the challenges in cancer management. To practically realize personalized medicine, development of cost-effective methods is required. Furthermore, genetic information in each patient’s record should be timely provided for individualized cancer treatment. In this regards, we have recently developed a rapid isothermal method to detect genetic polymorphisms in the NRF2 gene and correlated the genotyping data with the survival of patients who had primary lung cancer (Okano et al., 2013). By means of the new method (Ishikawa and Hayashizaki, 2013), we could detect the SNP -617C > A in the NRF2 gene within 30 to 45 min without DNA isolation and PCR amplification (Figure 2B). Such genotyping methods would provide a simple and practical tool for personalized cancer therapy and assessment of prognosis.
The author declares that the research was conducted in the absence of any commercial or financial relationships that could be construed as a potential conflict of interest.
The author’s study was supported by a research fund of Japan Science and Technology Agency (JST) project named “Development of the world’s fastest SNP detection system.” This means, except for the following governmental research fund, the author had no financial relationships with third parties.
Adachi, T., Nakagawa, H., Chung, I., Hagiya, Y., Hoshijima, K., Noguchi, N., et al. (2007). Nrf2-dependent and -independent induction of ABC transporters ABCC1, ABCC2, and ABCG2 in HepG2 cells under oxidative stress. J. Exp. Ther. Oncol. 6, 335–648.
Cho, H. Y., Reddy, S. P., DeBiase, A., Yamamoto, M., and Kleeberger, S. R. (2005). Gene expression profiling of NRF2-mediated protection against oxidative injury. Free Radic. Biol. Med. 38, 325–343. doi: 10.1016/j.freeradbiomed.2004.10.013
Pubmed Abstract | Pubmed Full Text | CrossRef Full Text | Google Scholar
Gomi, A., Shinoda, S., Masuzawa, T., Ishikawa, T., and Kuo, M. T. (1997). Transient induction of the MRP/GS-X pump and γ-glutamylcysteine synthetase by 1-(4-amino-2-methyl-5-pyrimidinyl)methyl-3-(2-chloroethyl)-3-nitrosourea in human glioma cells. Cancer Res. 57, 5292–5299.
Hayes, J. D., and McMahon, M. (2009). NRF2 and KEAP1 mutations: permanent activation of an adaptive response in cancer. Trends Biochem. Sci. 34, 176–188. doi: 10.1016/j.tibs.2008.12.008
Pubmed Abstract | Pubmed Full Text | CrossRef Full Text | Google Scholar
Homma, S., Ishii, Y., Morishima, Y., Yamadori, T., Matsuno, Y., Haraguchi, N., et al. (2009). Nrf2 enhances cell proliferation and resistance to anticancer drugs in human lung cancer. Clin. Cancer Res. 15, 3423–3432. doi: 10.1158/1078-0432.CCR-08-2822
Pubmed Abstract | Pubmed Full Text | CrossRef Full Text | Google Scholar
Huang, W. C., Chen, Y. J., Li, L. Y., Wei, Y. L., Hsu, S. C., Tsai, S. L., et al. (2011). Nuclear translocation of epidermal growth factor receptor by Akt-dependent phosphorylation enhances breast cancer-resistant protein expression in gefitinib-resistant cells. J. Biol. Chem. 286, 20558–20568. doi: 10.1074/jbc.M111.240796
Pubmed Abstract | Pubmed Full Text | CrossRef Full Text | Google Scholar
Ishii, T., Itoh, K., Takahashi, S., Sato, H., Yanagawa, T., Katoh, Y., et al. (2000). Transcription factor Nrf2 coordinately regulates a group of oxidative stress-inducible genes in macrophages. J. Biol. Chem. 275, 16023–16029. doi: 10.1074/jbc.275.21.16023
Pubmed Abstract | Pubmed Full Text | CrossRef Full Text | Google Scholar
Ishikawa, T., Bao, J. J., Yamane, Y., Akimaru, K., Frindrich, K., Wright, C. D., et al. (1996). Coordinated induction of MRP/GS-X pump and γ-glutamylcysteine synthetase by heavy metals in human leukemia cells. J. Biol. Chem. 271, 14981–14988. doi: 10.1074/jbc.271.25.14981
Ishikawa, T., and Hayashizaki, Y. (2013). Clinical SNP detection by SmartAmp method. Methods Mol. Biol. 1015, 55–69. doi: 10.1007/978-1-62703-435-7_3
Pubmed Abstract | Pubmed Full Text | CrossRef Full Text | Google Scholar
Itoh, K., Igarashi, K., Hayashi, N., Nishizawa, M., and Yamamoto, M. (1995). Cloning and characterization of a novel erythroid cell-derived CNC family transcription factor heterodimerizing with the small Maf family proteins. Mol. Cell. Biol. 15, 4184–4193.
Itoh, K., Wakabayashi, N., Katoh, Y., Ishii, Y., Igarashi, K., Engel, J. D., et al. (1999). Keap1 represses nuclear activation of antioxidant responsive elements by Nrf2 through binding to the amino-terminal Neh2 domain. Genes Dev. 13, 76–86. doi: 10.1101/gad.13.1.76
Pubmed Abstract | Pubmed Full Text | CrossRef Full Text | Google Scholar
Kobayashi, M., and Yamamoto, M. (2006). Nrf2-Keap1 regulation of cellular defense mechanisms against electrophiles and reactive oxygen species. Adv. Enzyme Regul. 46, 113–140. doi: 10.1016/j.advenzreg.2006.01.007
Pubmed Abstract | Pubmed Full Text | CrossRef Full Text | Google Scholar
Kuo, M. T., Bao, J. J., Curley, S. A., Ikeguchi, M., Johnston, D. A., and Ishikawa, T. (1996). Frequent coordinated over0expression of the MRP/GS-X pump and γ-glutamylcysteine synthetase genes in human colorectal cancers. Cancer Res. 56, 3642–3644.
Kuo, M. T., Bao, J., Furuichi, M., Yamane, Y., Gomi, A., Savaraj, N., et al. (1998). Frequent coexpression of MRP/GS-X pump and gamma-glutamylcysteine synthetase mRNA in drug-resistant cells, untreated tumor cells, and normal mouse tissues. Biochem. Pharmacol. 55, 605–615. doi: 10.1016/S0006-2952(97)00494-2
Pubmed Abstract | Pubmed Full Text | CrossRef Full Text | Google Scholar
Kwak, M. K., Itoh, K., Yamamoto, M., and Kensler, T. W. (2002). Enhanced expression of the transcription factor Nrf2 by cancer chemopreventive agents: role of antioxidant response element-like sequences in the nrf2 promoter. Mol. Cell. Biol. 22, 2883–2892. doi: 10.1128/MCB.22.9.2883-2892.2002
Pubmed Abstract | Pubmed Full Text | CrossRef Full Text | Google Scholar
Lau, A., Villeneuve, N. F., Sun, Z., Wong, P. K., and Zhang, D. D. (2008). Dual roles of Nrf2 in cancer. Pharmacol. Res. 58, 262–270. doi: 10.1016/j.phrs.2008.09.003
Pubmed Abstract | Pubmed Full Text | CrossRef Full Text | Google Scholar
Marzec, J. M., Christie, J. D., Reddy, S. P., Jedlica, A. E., Vuong, H., Lanken, P. N., et al. (2007). Functional polymorphisms in the transcription factor NRF2 in humans increase the risk of acute lung injury. FASEB J. 21, 2237–2246. doi: 10.1096/fj.06-7759com
Pubmed Abstract | Pubmed Full Text | CrossRef Full Text | Google Scholar
Meyer zu Schwabedissen, H. E., Grube, M., Dreisbach, A., Jedlitschky, G., Meissner, K., Linnemann, K., et al. (2006). Epidermal growth factor-mediated activation of the map kinase cascade results in altered expression and function of ABCG2 (BCRP). Drug Metab. Dispos. 34, 524–533. doi: 10.1124/dmd.105.007591
Pubmed Abstract | Pubmed Full Text | CrossRef Full Text | Google Scholar
Mitsuishi, Y., Taguchi, K., Kawatani, Y., Shibata, T., Nukiwa, T., Aburatani, H., et al. (2012). Nrf2 redirects glucose and glutamine into anabolic pathways in metabolic reprogramming. Cancer Cell 22, 66–79. doi: 10.1016/j.ccr.2012.05.016
Pubmed Abstract | Pubmed Full Text | CrossRef Full Text | Google Scholar
Moi, P., Chan, K., Asunis, I., Cao, A., and Kan, Y. W. (1994). Isolation of NF-E2-related factor 2 (Nrf2), a NF-E2-like basic leucine zipper transcriptional activator that binds to the tandem NF-E2/AP1 repeat of the β-globin locus control region. Proc. Natl. Acad. Sci. U.S.A. 91, 9926–9930. doi: 10.1073/pnas.91.21.9926
Pubmed Abstract | Pubmed Full Text | CrossRef Full Text | Google Scholar
Motohashi, H., and Yamamoto, M. (2004). Nrf2-Keap1 defines a physiologically important stress response mechanism. Trends Mol. Med. 10, 549–557. doi: 10.1016/j.molmed.2004.09.003
Pubmed Abstract | Pubmed Full Text | CrossRef Full Text | Google Scholar
Nguyen, T., Nioi, P., and Pickett, C. B. (2009). The Nrf2-antioxidant response element signaling pathway and its activation by oxidative stress. J Biol. Chem. 284, 13291–13295. doi: 10.1074/jbc.R900010200
Pubmed Abstract | Pubmed Full Text | CrossRef Full Text | Google Scholar
Okano, Y., Nezu, U., Enokida, Y., Lee, M. T. M., Kinoshita, H., Lezhava, A., et al. (2013). SNP (-617C > A) in ARE-like loci of the NRF2 gene: a new biomarker for prognosis of lung adenocarcinoma in Japanese non-smoking women. PLoS ONE 8:e73794. doi: 10.1371/journal.pone.0073794
Pubmed Abstract | Pubmed Full Text | CrossRef Full Text | Google Scholar
Ramos-Gomez, M., Kwak, M. K., Dolan, P. M., Itoh, K., Yamamoto, M., Talalay, P., et al. (2001). Sensitivity to carcinogenesis is increased and chemoprotective efficacy of enzyme inducers is lost in nrf2 transcription factor-deficient mice. Proc. Natl. Acad. Sci. U.S.A. 98, 3410–3415. doi: 10.1073/pnas.051618798
Pubmed Abstract | Pubmed Full Text | CrossRef Full Text | Google Scholar
Saito, H., Hirano, H., Nakagawa, H., Fukami, T., Oosumi, K., Murakami, K., et al. (2006). A new strategy of high-speed screening and quantitative structure-activity relationship analysis to evaluate human ATP-binding cassette transporter ABCG2-drug interactions. J. Pharmacol. Exp. Ther. 317, 1114–1124. doi: 10.1124/jpet.105.099036
Pubmed Abstract | Pubmed Full Text | CrossRef Full Text | Google Scholar
Shelton, P., and Jaiswal, A. K. (2013). The transcriptional factor NF-E2-related factor 2 (Nrf2): a protooncogene? FASEB J. 27, 414–423. doi: 10.1096/fj.12-217257
Pubmed Abstract | Pubmed Full Text | CrossRef Full Text | Google Scholar
Shen, G., and Kong, A. N. (2009). Nrf2 plays an important role in coordinated regulation of phase II drug metabolism enzymes and phase III drug transporters. Biopharm. Drug Dispos. 30, 345–355. doi: 10.1002/bdd.680
Pubmed Abstract | Pubmed Full Text | CrossRef Full Text | Google Scholar
Singh, A., Wu, H., Zhang, P., Happel, C., Ma, J., and Biswal, S. (2010). Expression of ABCG2 (BCRP) is regulated by Nrf2 in cancer cells that confers side population and chemoresistance phenotype. Mol. Cancer Ther. 9, 2365–2376. doi: 10.1158/1535-7163.MCT-10-0108
Pubmed Abstract | Pubmed Full Text | CrossRef Full Text | Google Scholar
Sjöblom, T., Jones, S., Wood, L. D., Parsons, D. W., Lin, J., Barber, T. D., et al. (2006). The consensus coding sequences of human breast and colorectal cancers. Science 314, 268–274. doi: 10.1126/science.1133427
Pubmed Abstract | Pubmed Full Text | CrossRef Full Text | Google Scholar
Solis, L. M., Behrens, C., Dong, W., Suraokar, M., Ozburn, N. C., Moran, C. A., et al. (2010). Nrf2 and Keap1 abnormalities in non-small cell lung carcinoma and association with clinicopathologic features. Clin. Cancer Res. 16, 3743–3753. doi: 10.1158/1078-0432.CCR-09-3352
Pubmed Abstract | Pubmed Full Text | CrossRef Full Text | Google Scholar
Sporn, M. B., and Liby, K. T. (2012). NRF2 and cancer: the good, the bad and the importance of context. Nat. Rev. Cancer 12, 564–571. doi: 10.1038/nrc3278
Pubmed Abstract | Pubmed Full Text | CrossRef Full Text | Google Scholar
Taguchi, K., Motohashi, H., and Yamamoto, M. (2011). Molecular mechanisms of the Keap1-Nrf2 pathway in stress response and cancer evolution. Genes Cells 16, 123–140. doi: 10.1111/j.1365-2443.2010.01473.x
Pubmed Abstract | Pubmed Full Text | CrossRef Full Text | Google Scholar
Yamadori, T., Ishii, Y., Homma, S., Morishima, Y., Kurishima, K., Itoh, K., et al. (2012). Molecular mechanisms for the regulation of Nrf2-mediated cell proliferation in non-small-cell lung cancers. Oncogene 31, 4768–4777. doi: 10.1038/onc.2011.628
Pubmed Abstract | Pubmed Full Text | CrossRef Full Text | Google Scholar
Yamamoto, T., Yoh, K., Kobayashi, A., Ishii, Y., Kure, S., Koyama, A., et al. (2004). Identification of polymorphisms in the promoter region of the human NRF2 gene. Biochem. Biophys. Res. Commun. 321, 72–79. doi: 10.1016/j.bbrc.2004.06.112
Pubmed Abstract | Pubmed Full Text | CrossRef Full Text | Google Scholar
Yamane, Y., Furuichi, M., Song, R., Van, N. T., Mulcahy, R. T., Ishikawa, T., et al. (1998). Expression of multidrug resistance protein/GS-X pump and γ-glutamylcysteine synthetase genes is regulated by oxidative stress. J. Biol. Chem. 273, 31075–31085. doi: 10.1074/jbc.273.47.31075
Pubmed Abstract | Pubmed Full Text | CrossRef Full Text | Google Scholar
Yoo, N. J., Kim, H. R., Kim, Y. R., An, C. H., and Lee, S. H. (2012). Somatic mutations of the KEAP1 gene in common solid cancers. Histopathology 60, 943–952. doi: 10.1111/j.1365-2559.2012.04178.x
Pubmed Abstract | Pubmed Full Text | CrossRef Full Text | Google Scholar
Keywords: drug resistance, anti-oxidant response element (ARE), ABCG2, NRF2, single nucleotide polymorphism (SNP)
Citation: Ishikawa T (2014) Genetic polymorphism in the NRF2 gene as a prognosis marker for cancer chemotherapy. Front. Genet. 5:383. doi: 10.3389/fgene.2014.00383
Received: 22 July 2014; Paper pending published: 20 September 2014;
Accepted: 17 October 2014; Published online: 04 November 2014.
Edited by:
Luis Abel Quiñones, University of Chile, ChileReviewed by:
Lucia Taja-Chayeb, Instituto Nacional de Cancerologia, MexicoCopyright © 2014 Ishikawa. This is an open-access article distributed under the terms of the Creative Commons Attribution License (CC BY). The use, distribution or reproduction in other forums is permitted, provided the original author(s) or licensor are credited and that the original publication in this journal is cited, in accordance with accepted academic practice. No use, distribution or reproduction is permitted which does not comply with these terms.
*Correspondence: Toshihisa Ishikawa, Personalized Medicine Research Institute, NGO Personalized Medicine and Healthcare, 4-17-30 Kirigaoka, Midori-ku, Yokohama 226-0016, Japan e-mail:dG9zaGloaXNhLmlzaGlrYXdhLnJAZ21haWwuY29t
Disclaimer: All claims expressed in this article are solely those of the authors and do not necessarily represent those of their affiliated organizations, or those of the publisher, the editors and the reviewers. Any product that may be evaluated in this article or claim that may be made by its manufacturer is not guaranteed or endorsed by the publisher.
Research integrity at Frontiers
Learn more about the work of our research integrity team to safeguard the quality of each article we publish.