- 1Department of Biodiversity and Plant Systematics, Centre for Organismal Studies Heidelberg, Heidelberg University, Heidelberg, Germany
- 2Laboratorio de Botánica y Sistemática, Departamento de Ciencias Biológicas, Universidad de los Andes, Bogotá DC, Colombia
The páramos, high-elevation Andean grasslands ranging from ca. 2800 m to the snow line, harbor one of the fastest evolving biomes worldwide since their appearance in the northern Andes 3–5 million years (Ma) ago. Hypericum (St. John's wort), with over 65% of its Neotropical species, has a center of diversity in these high Mountain ecosystems. Using nuclear rDNA internal transcribed spacer (ITS) sequences of a broad sample of New World Hypericum species we investigate phylogenetic patterns, estimate divergence times, and provide the first insights into diversification rates within the genus in the Neotropics. Two lineages appear to have independently dispersed into South America around 3.5 Ma ago, one of which has radiated in the páramos (Brathys). We find strong support for the polyphyly of section Trigynobrathys, several species of which group within Brathys, while others are found in temperate lowland South America (Trigynobrathys s.str.). All páramo species of Hypericum group in one clade. Within these páramo Hypericum species enormous phenotypic evolution has taken place (life forms from arborescent to prostrate shrubs) evidently in a short time frame. We hypothesize multiple mechanisms to be responsible for the low differentiation in the ITS region contrary to the high morphological diversity found in Hypericum in the páramos. Amongst these may be ongoing hybridization and incomplete lineage sorting, as well as the putative adaptive radiation, which can explain the contrast between phenotypic diversity and the close phylogenetic relationships.
Introduction
High altitude mountain regions in the tropics constitute an open grassland vegetation type that is characterized by large rosette and small cushion plants, bunch grasses, and evergreen sclerophyllous shrubs (Luteyn, 1999). These high-elevation grasslands occur above the tree-line and below the upper limits of plant life “along the crests of the highest mountain ranges or on isolated mountaintops, like islands in a sea of forest” (Luteyn, 1999). The páramos of South America are discontinuously distributed along the Andean Cordilleras between ca. 2800–5000 m a.s.l. in Venezuela, Colombia, Ecuador, and northern Peru, with outliers in the Cordillera de Talamanca in Costa Rica and adjacent Panama.
A hyperdiverse high-elevation ecosystem has evolved since the northern Andes were formed in the Pliocene above the modern tree-line that marks the lower limit of páramo vegetation (ca. 3–5 Ma ago; Gregory-Wodzicki, 2000; Hoorn et al., 2010), which makes it one of the youngest of the Neotropical ecosystems (Graham, 2009). Today, the páramos alone comprise around 3500 vascular plant species (Luteyn, 1999; Sklenár et al., 2010; Graham, 2011a), half of which are of temperate origin at the generic level (Smith and Cleef, 1988; Sklenár et al., 2010; Weigend et al., 2010; Antonelli and Sanmartín, 2011a) as in other high elevation tropical floras (Gehrke and Linder, 2009). In contrast, the páramo flora is the richest overall tropical mountain flora and has the largest number of genera and endemic elements (Smith and Cleef, 1988; Myers et al., 2000).
High species richness in the tropical Andes has been attributed to their geographic extent and history (Graham, 2011a), the availability of migration routes for Holarctic lineages along mountain chains (Bell and Donoghue, 2005; Hughes and Eastwood, 2006; Antonelli et al., 2009), and drivers promoting rapid diversification, such as climatic oscillations during the Plio- to Pleistocene, habitat turnover and heterogeneity, and founder effects attributable to isolation on sky-island-like mountain formations (van der Hammen and Hooghiemstra, 2000; von Hagen and Kadereit, 2001; Luteyn, 2002; Hughes and Eastwood, 2006; Losos, 2010; Sklenár et al., 2010; Luebert et al., 2011). Espeletia (Cuatrecasas, 1986; Rauscher, 2002), Gentianella and Halenia (Kadereit and von Hagen, 2003), or Lupinus (Hughes and Eastwood, 2006; Drummond et al., 2012) are well known examples of adaptive radiations in the high-elevation Andean grasslands.
The flowering plant genus Hypericum (St. John's wort, Hypericaceae) is a prominent and often abundant component of the flora with arborescent shrubs in the sub-páramo (H. laricifolium, H. irazuense), dwarf shrubs in pastures or meadows from elfin forest to higher zones (H. andinum, H. mexicanum, H. juniperinum), or on rocky and disturbed places (H. cardonae, H. humboldtianum), and prostrate plants in damp areas in the grass páramo (H. selaginella, H. prostratum). Around 65 of the ~100 species described in Hypericum in South America are páramo endemics. Overall, Hypericum is of temperate origin and has its main center of species richness in the Old World (Nürk and Blattner, 2010; Meseguer et al., 2013; Nürk et al., 2013). Molecular phylogenetic studies using sequences of the nuclear rDNA internal transcribed spacers (ITS) (Meseguer et al., 2013; Nürk et al., 2013) and chloroplast data (Meseguer et al., 2013) revealed the three large Hypericum sections Myriandra, Brathys and Trigynobrathys sensu Robson (1977, 1987, 1990, 2012) as a monophyletic group, which includes ca. 90% of the Hypericum species native to the New World1, and verified the genus Triadenum to be included within Hypericum as sister to the Myriandra+Brathys s.l. clade of Nürk et al. (2013); i.e., clade B in Meseguer et al. [2013; see Ruhfel et al. (2011) for taxonomic implications].
Hypericum sect. Myriandra species are distributed mainly in the Nearctic with some located in Honduras, Bermuda and the Caribbean. The majority of New World Hypericum is classified in sects. Brathys and Trigynobrathys (Table 1). Although predominately a high-elevation group in the Neotropics, a small number of herbaceous Hypericum species has adapted to lower elevations, distributed below 3000 to less than 1000 m in lowland regions of temperate South America (Robson, 1977 onwards).
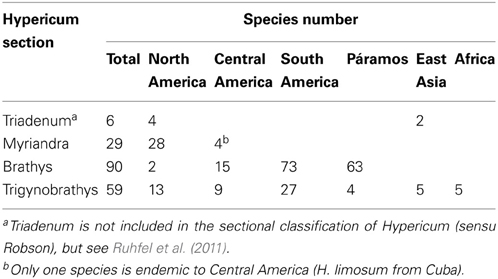
Table 1. Sections of Hypericum (sensu Robson, 1977 onwardsa) in this study, detailing species number and distribution.
While shrubs constitute the dominant life form in North America, a multitude of life forms evolved in the Neotropics—up to 6 m high sclerophylous arborescent to small dwarf shrubs, prostrate shrubs, and perennial to annual herbs. Most of this phenotypic diversity can be found in the páramos of the Andes (Figure 1). This diversity is mirrored by the great variety of ecological conditions occupied (for an overview see Crockett et al., 2010) and cytology; chromosome numbers range from (2n=) 8, 12, 16, 18, 22, 24, and 32 (Robson, 1987, 1990; Moraes et al., 2009), suggesting that polyploidization is involved in the evolution of Hypericum in South America. However, for only three species (H. irazuense, H. silenoides, H. brasiliense; multiple counts in the last) of Neotropical Hypericum have chromosome numbers been published (Robson, 1987, 1990; Moraes et al., 2009). This prevents comparative phylogenetic studies on the extent and distribution of polyploidy across the Neotropical species.
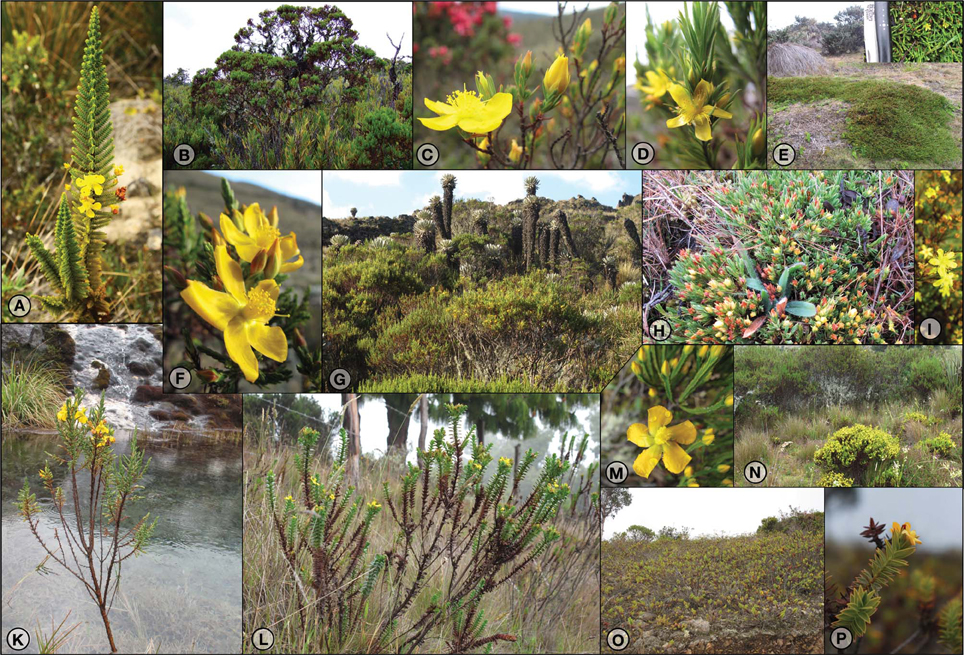
Figure 1. Diversity in life forms and ecology in Andean high-elevation Hypericum. (A) H. woodianum, 80–150 cm erect shrub, flowers 15–18 mm ∅, wet Andean forest to páramo; (B) H. irazuense, 70–500 cm shrub, flowers 25–30 mm ∅, open slopes or among bamboo in the páramos; (C) H. garciae, ca. 75 cm dwarf shrub, flowers 17–20 mm ∅, páramo, dry stony or sandy soil; (D,I,N) H. juniperinum, 20–100 (−250) cm erect (sub-) shrub, flowers 4–12 mm ∅, forest margins and damp or shaded subpáramo to páramo, often abundant in pastures/meadows; (E) H. prostratum, 10–30 cm prostrate shrub, flowers 4–8 mm ∅, open and wet páramo; (F,G,K) H. laricifolium, (10–) 180 (−600) cm shrub, flowers 15–30 mm ∅, open subpáramo to Espeletia páramo, well drained (G) to aquatic (K); (H) H. selaginella, 5–15 cm prostrate shrub, flowers 6–8 mm ∅, dry and stony to dampish páramo; (L) H. mexicanum, 15–70 (−150) cm stiff erect shrublet, flowers 15–25 mm ∅, talus or grassy slopes to open páramo; (M) H. tetrastichum, 5–100 cm dwarf shrub, flowers 10–15 mm ∅, wet and dry often exposed areas in páramo; (N) H. myricariifolium (in the back, with H. juniperinum in the front), up to 200 cm tall shrub, flowers 18–25 mm ∅, open slopes in páramo; (O,P) H. cardonae, 20–100 cm shrub, flowers 12–18 mm ∅, dry rocky talus and cliffs. Panel (1A) by J. Paal, Panel (1B) by S. Crockett, Panel (1P) by G. Atchison.
The recency of the páramo biome, the extent of ecological and phenotypic diversity (Figure 1), as well as high species richness (summarized in Robson, 2012) suggest that Hypericum could represent an adaptive radiation (Simpson, 1953) in the páramos of South America. Following Glor (2010), adaptive radiation is a response to natural selection and ecological opportunity involving diversification of species and associated adaptations. To define and diagnose adaptive radiation three operational criteria are considered in the phylogenetic context: (a) multiplication of species and common descent, (b) extraordinary diversification, and (c) adaptation via natural selection (phenotype-environment correlation and trait utility; Schluter, 2000; Sudhaus, 2004; Glor, 2010).
Insights into the evolutionary history of Hypericum in the Neotropics first demand phylogenetic hypotheses in an explicit framework to test questions about diversification rates and niche shifts, biogeography, key innovations, or polyploidization events (Harvey and Pagel, 1991; Emshwiller and Doyle, 1998; Pagel, 1999; Emshwiller and Doyle, 2002; Stephens and Wiens, 2003; Wiens and Donoghue, 2004; Moore and Donoghue, 2007, 2009; Crisp et al., 2011; Vamosi and Vamosi, 2011). The consideration of divergence times of lineages in the phylogenetic context is essential, as dispersal patterns and species richness are related to time (Ricklefs and Latham, 1992; Stephens and Wiens, 2003; Wiens and Donoghue, 2004), and area availability (Vamosi and Vamosi, 2010).
In a recent study, Meseguer et al. (2013) estimated divergence times and conducted biogeographic analyses based on chloroplast sequence variation (trnS-trnG, trnL-trnF, psbA-trnH) for the genus Hypericum. On the base of a rather reduced sampling of South American Hypericum (5 species, 7 accessions) they revealed a single Neotropical clade that had a mean crown group age of 3.9 Ma (Meseguer et al., 2013), sister to a clade containing North American and Asian species belonging to sect. Trigynobrathys (together called the “Brathys-group”). Furthermore, they suggest merging sect. Trigynobrathys into sect. Brathys sensu Robson (1977, 1987, 1990, 2012), as one species from sect. Trigynobrathys groups within a clade containing mainly species belonging to sect. Brathys, a result also revealed in phylogenetic studies analyzing morphological (Nürk and Blattner, 2010) and rDNA ITS data (Nürk et al., 2013).
In this study, we conduct new phylogenetic analyses and estimate divergence times in a Bayesian framework, using nuclear ITS sequences of a representative sampling of New World Hypericum. We employed a wide sampling across the New World species of Hypericum and a dense sampling within the Neotropics to expand prior phylogenetic hypotheses (Meseguer et al., 2013; Nürk et al., 2013) on Hypericum in South America. Despite potentially problematic issues resulting from the multi-copy nature of ITS (Baldwin, 1992; Baldwin et al., 1995) for phylogenetic inference (e.g., possible paralogs, and/or pseudogenes; Álvarez and Wendel, 2003; Bailey et al., 2003; Nieto Feliner and Rosselló, 2007) we used this marker system as it offers expanded species/accession sampling (Blattner, 1999; Nürk et al., 2013) while being aware of the potential challenges (see Discussion).
Based on a dense sampling within Neotropical Hypericum, we investigate phylogenetic relationships of the South American and especially the high-elevation Andean species. In particular, we aim to address the following questions: (1) Are Neotropical Hypericum species monophyletic, i.e., is there only one clade in the Neotropics, as suggested by Meseguer et al. (2013), or is there more than one? (2) Is the polyphyletic nature of sect. Trigynobrathys as reported in previous studies (Meseguer et al., 2013; Nürk et al., 2013) an artifact of species sampling? (3) Are ITS based divergence time estimations congruent with those previously reported from cpDNA (Meseguer et al., 2013)? (4) How many lineages colonized the páramos and what are the ages of these lineages? And finally, (5) are there differences in diversification rates between clades of New World Hypericum?
The first three questions examine previously published hypotheses and compare ITS age estimates to those inferred by analysis of chloroplast sequence variation. The latter two questions are a first attempt to diagnose adaptive radiation in Andean high-elevation Hypericum, by aiming at two of the three operational criteria that define adaptive radiation as proposed by Glor (2010), (a) multiplication of species and common descent, and (b) extraordinary diversification.
Materials and Methods
Taxon Sampling and Molecular Methods
Our approach involved broad sampling within New World Hypericum to ascertain hypotheses about backbone relationships (major lineages), as well as of closer related species from the Andean highlands. Samples were obtained from herbarium collections (ANDES, BM, GAT, HEID) and freshly collected silica-gel dried material. Forty-five ITS sequences selected from GenBank were included into the final data set, additionally to 135 sequences (representing 56 species) newly generated for this study (see Appendix, voucher), which have been submitted to the European Nucleotide Archive (http://www.ebi.ac.uk/ena/data/view/HG004646-HG004780, Accession No. HG004646–780). In total, the final data set contained 180 accessions (93 species), including 135 native to South America (56 species); a three- to ten-fold increase in species sampling in the Neotropics compared to previous studies (Meseguer et al., 2013; Nürk et al., 2013).
Genomic DNA was extracted with the Invisorb® Spin Plant Mini Kit (Stratec Molecular GmbH, Berlin, Germany) following the manufacturer's protocol. Amplification of the ITS region (including ITS-1, 5.8S rDNA, and ITS-2) followed the procedure detailed in Nürk et al. (2013), with separate amplification (and sequencing) of ITS-1 and ITS-2 for poorly preserved herbarium exsiccatae (Blattner, 1999). Cleaned amplification products were sent for sequencing to Eurofins MWG Operon (Ebersberg, Germany). Forward and reverse sequences from each template were manually edited and combined into a single consensus sequences with Geneious v5.4 (Biomatters, available from www.geneious.com). Sequences were checked for patterns in the chromatograms, which suggest multiple non-identical ITS copies (paralogs or pseudogenes), and multiple reads per site were coded as ambiguities (present in the spacer regions only, in 23 newly generated sequences with one to four ambiguous sites per sequence and in eight sequences downloaded from GenBank with one to seven ambiguous sites per sequence).
Phylogenetic Inference
Sequences were aligned using the L-INS-I algorithm implemented in the software Multiple Alignment using Fast Fourier Transform (MAFFT) v6.9 (Katoh et al., 2002; Katoh, 2005) and manually adjusted using PhyDE v0.9 (Available online: http://www.phyde.de). MrModeltest v2.3 (Nylander, 2004) was used to select the appropriate model of sequence evolution and the SYM + Γ model (Yang, 1993, 1994; Zharkikh, 1994) was chosen according to the Akaike Information Criterion (Akaike, 1974; Posada and Buckley, 2004). Phylogenetic analyses were performed under Maximum likelihood (ML; Felsenstein, 1981) and Bayesian Inference (BI; Mau et al., 1999). Hypericum faurei R.Keller [=Triadenum japonicum (Blume) Makino] belonging to the Triadenum clade was used as outgroup, following Nürk et al. (2013) who showed this clade to be sister to the rest. For ML analysis the RAxML GUI v1.1 (Stamatakis, 2006; Silvestro and Michalak, 2011) was used with the GTRCAT model and clade support was evaluated with 10000 rapid bootstrap pseudoreplicates (Stamatakis et al., 2008). For BI optimization MrBayes v3.2.1 (Ronquist and Huelsenbeck, 2003) was started with 4 independent runs, each with 4 chains for 10 million generations with the appropriate substitution model (SYM + Γ), setting temperature to 0.01, sampling every 1000 generations, and using the ML tree as a starting tree, but introducing random perturbations into the starting tree to initiate parameter calculation from different priors to enable detection of possible convergence problems (using the command “mcmcp nperts = 5”). Following the results of Meseguer et al. (2013), which showed a more realistic branch length estimation when introducing a lambda parameter correction in Bayesian phylogenetics on ITS sequence data in Hypericum, we used a ‘corrected’ exponential prior on branch length of 1/λ = 0.1 [“prset brlenspr = Unconstrained:Exp(100)”]. Convergence of the parameters was monitored using Tracer v1.5 (Rambaut and Drummond, 2007). After discarding 25% of the sampled trees as burnin, posterior probabilities were calculated on the BI stationary sample.
Divergence Time Estimations
We choose the Bayesian tree to test for rate constancy among lineages. The likelihood scores associated with branch length were calculated on this tree in PAUP* (Swofford, 2002) under the optimal model of sequence evolution and associated parameters with and without a strict molecular clock enforced. We followed the approach of Huelsenbeck and Rannala (1997) to assess significance. A global molecular clock was rejected (p < 0.05) for the rDNA sequence data. Therefore, divergence times were estimated under a relaxed molecular clock employing the uncorrelated lognormal (UCLN) model (Drummond et al., 2006) that assumes branch specific substitution rates to be drawn from a single lognormal distribution estimated from the data. Implementation of the UCLN model in BEAST v1.7.2 (Drummond and Rambaut, 2007) together with the use of Markov chain Monte Carlo (MCMC) sampling methods estimates both topology and substitution rates and calculates absolute divergence times and confidence intervals when calibrated with external data (fossils, or secondary calibration points like estimated ages revealed in other studies). The following calibration points were considered, relying on the age estimates reported in Meseguer et al. (2013). (1) The age of the root node estimated to (23.82−) 29.30 (−35, 23) Ma was constrained with a normal distribution that had a mean of 29, and a standard deviation of 5. (2) The age of the Myriandra+Brathys s.l. crown node estimated to (16.99−) 21.92 (−27, 33) Ma was constrained with a normal distribution that had a mean of 22, and a standard deviation of 4. (3) The age of the Myriandra crown node estimated to (9.41−) 13.59 (−18.48) Ma was constrained with a normal distribution that had a mean of 13.5, and a standard deviation of 3.
Analyses were performed in two independent runs in BEAST to test for convergence in divergence times, each consisted of 100 million generations, and sampling a tree every 10000 generation. Each run started from the tree obtained by ML search, after performing a semi-parametric method based on penalized likelihood (Sanderson, 2002) in R (R Development Core Team, 2013) with the “chronopl” command as implemented in the package APE (Paradis et al., 2004). The GTR model of nucleotide substitution was applied with the Γ model of site heterogeneity. The birth and death model of speciation considering incomplete sampling (Stadler, 2009) was set as tree prior. Accessions grouping in the BI tree within the Myriandra and Myriandra+Brathys s.l clades were constrained monophyletic. Convergence of the parameters was monitored using Tracer v1.5 (Rambaut and Drummond, 2007) and the resulting trees of the two runs were combined in LogCombiner (Drummond and Rambaut, 2007) with a burnin of 50%. Means and confidence intervals were calculated on the remaining 10002 trees in TreeAnnotator (Drummond and Rambaut, 2007) to obtain a final consensus tree [maximum clade credibility tree that has 95% of the highest posterior density (HPD)] for visualization in FigTree v1.3.1 (Rambaut, 2006–2013).
Diversification Rates
As approximation of net diversification rates (r) we used the simple macro-evolutionary constant rate, pure-birth (Yule model) taxonomic likelihood estimate of Magallón and Sanderson (2001) calculated as r = (ln N1 − ln N0)/t, where N1 = extant species (standing taxonomic diversity), N0 = initial species diversity, here taken as 1, and t = inferred clade age (time in Ma). We calculated r based on crown ages using the Bayesian mean and 95% HPD age estimates over the entire phylogeny, and clade-specific r for Triadenum (6 species), Myriandra (29 species), Trigynobrathys s.str. (≤52 species), Brathys (≥97 species), and the Páramo clade (≤67 species) and assigned species numbers according to species richness of the sections given in Robson (2012). The polyphyly of sect. Trigynobrathys, however, complicates the assignment of species richness to the Trigynobrathys s.str. and Brathys clades, as less than 30% of the species assigned to sect. Trigynobrathys are sampled in this study. Thus, we used the numbers given above, as we cannot approximate, which species belonging to sect. Trigynobrathys sensu Robson (1977 onwards) group within the Brathys and Trigynobrathys s.str. clade, respectively. For diversification rate estimation of the Páramo clade we assigned species richness in a conservative way, using only the number of species reported to be native to páramo habitats (Robson, 1987, 1990, 2012), i.e., used an underestimated number of species belonging to this clade.
To test the hypothesis of extraordinary diversification in the páramos, we applied the likelihood-based approach given in Magallón and Sanderson (2001). Specifically, we ask which of the major clades in our phylogeny are unexpectedly species-rich (or poor), given their age and the estimated net diversification rate for the entire phylogeny (i.e., the background rate for Triadenum+Myriandra+Brathys s.l.). We calculated 95% confidence intervals for the background net diversification rate (r) based on the crown group age using the formula kupper(t) = 1 + logβ0.025 (1 + α) /r (1 − α − β + αβ) + α + 2β − 1 for the upper boundary value, and klower(t) = 1 + logβ0.975 (1 + α)/r (1 − α − β + αβ) + α + 2β − 1 for the lower, where β = (ert − 1/ert − ε) and α = εβ, and assuming (1) no extinction (relative extinction rate ε = 0), and (2) a reasonably high relative extinction rate (ε = 0.9). The 95% confidence interval of the expected number of species is the range of values between kupper and klower in a semi-log plot for crown group ages of (log) species diversity vs. age at time t after the origin of a clade, under a given r and ε. Those clades that fall outside the confidence intervals are then regarded as being exceptionally species-rich or poor (Magallón and Sanderson, 2001).
Results
Phylogeny
The aligned data matrix comprised 755 characters, of which 366 were variable. The 50% majority-rule consensus tree of the Bayesian (BI) stationary sample (n = 30004 trees) (Figure 2) is highly congruent with the ML consensus tree (not shown) in the sense that there were no conflicts between strongly supported clades (>97% Bayesian posterior probability [BPP], >75% bootstrap support [BS]). Moreover, correction of the exponential prior (lambda) of branch length in the Bayesian analysis resulted in average branch length congruent with those inferred under ML. The trees and data sets produced in this study are available from TreeBase (http://www.treebase.org) study number 14179.
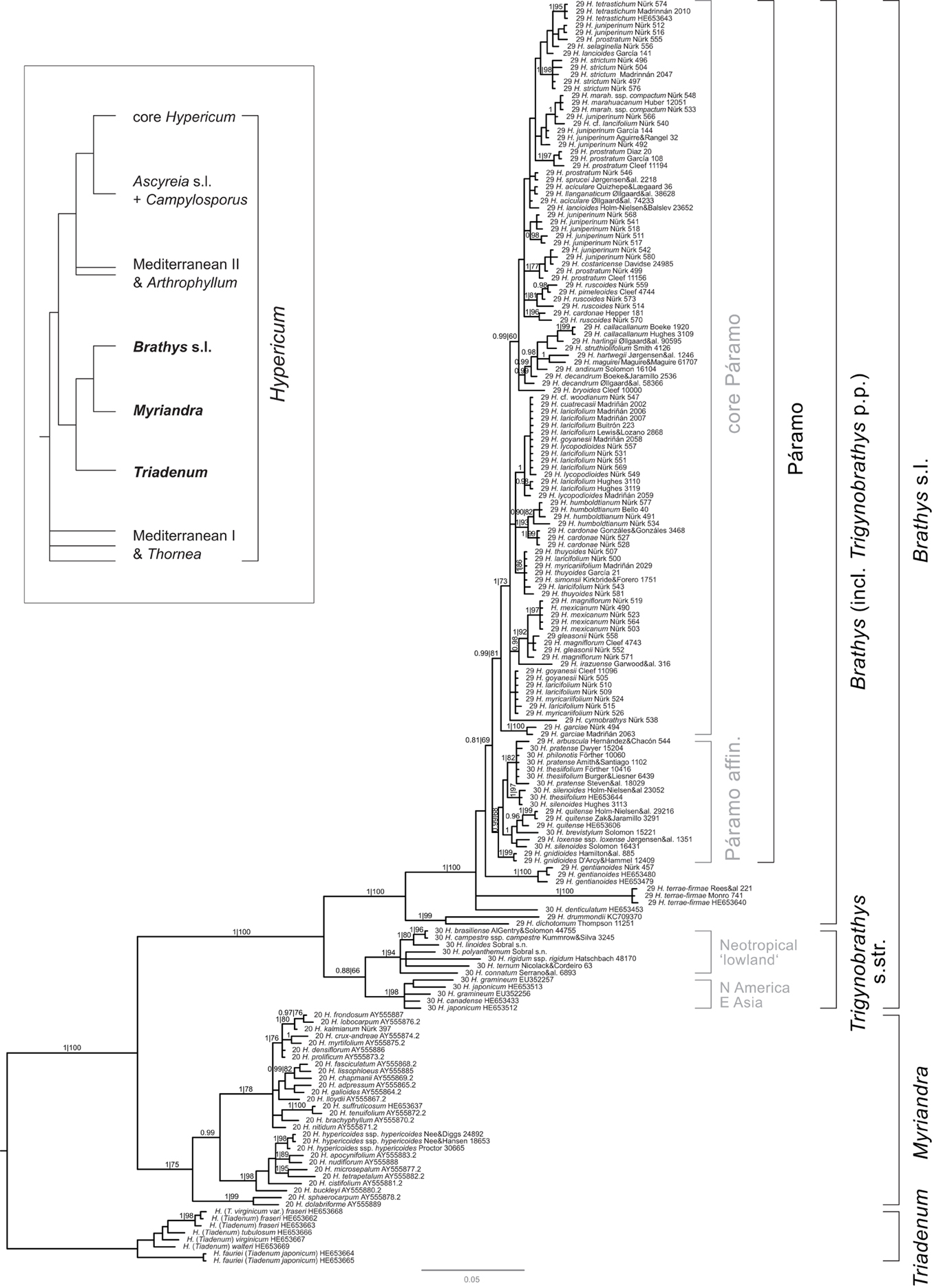
Figure 2. Phylogeny of New World Hypericum inferred from rDNA ITS sequence Bayesian analysis. In the tree sketch top left, the phylogenetic position of the species in this study within the genus Hypericum is marked by clade names in boldface. Bayesian posterior probabilities and ML bootstrap support is given above the branches (BPP|BS). Rooting is that of Nürk et al. (2013). Accession names consist of a section number (Robson, 2012), the species, and a collection/GenBank identifier. Clade names not considered in Figure 3B are given in gray.
Relationships among the major clades (Figure 2) are consistent with previous studies (Meseguer et al., 2013; Nürk et al., 2013). North American sect. Myriandra form a clade (1.00 BPP, 75% BS), sister to Brathys s.l. (1.00 BPP, 100 BS), the latter containing all, but not solely Neotropical taxa. Species from East Asia and North America group within the Trigynobrathys s.str. clade, which is not strongly supported in our analysis (0.88 BPP, 66 BS), but present with good support in other studies (Meseguer et al., 2013; Nürk et al., 2013). Within Trigynobrathys s.str. two subclades are supported, one containing the East Asian-North American species (1.00 BPP, 98 BS), and the other containing Neotropical “lowland” species (i.e., native to lowland and upland areas of Brazil, Bolivia, Paraguay, Uruguay, and Argentina; 1.00 BPP, 94 BS). All other Neotropical species group within the Brathys clade (1.00 BPP, 100 BS), which also includes some species belonging taxonomically to sect. Trigynobrathys. This verifies sect. Trigynobrathys sensu Robson (1977 onwards) polyphyletic (non-monophyletic).
Within the Brathys clade, relationships are well-supported at the most basal dichotomy, but are almost without support between the remaining taxa. However, all species native to the páramos group within one clade (Figure 2), although without strong support (0.81 BPP, 69 BS). The species belonging to sect. Trigynobrathys sensu Robson (1977 onwards) group within one subclade of the Páramo clade (0.99 BPP, 68 BS) sister to the remainder. This subclade contains species from Central America (H. pratense) and from lowland areas of South America (H. silenoides), together with páramo natives (H. gnidioides, H. arbuscula, H. thesiifolium), the so-called Páramo affinis clade. The core Páramo clade (0.99 BPP, 81 BS) contains only species native to the high-elevation Andes. Apart from few subclades, which received moderate to strong support (e.g., a clade containing H. cardonae and H. humboldtianum, with 1.00 BPP, 93 BS), relationships are almost not resolved in the core Páramo clade.
Age Estimates and Diversification Rates
The ultrametric time-calibrated maximum clade credibility tree (chronogram) obtained by the Bayesian relaxed clock analyses is shown in Figure 3A. The crown age estimates for the major clades are summarized in Table 2. The inferred divergence times of Brathys s.l. (this node was not constrained for calibration) is highly congruent with the ages reported by Meseguer et al. (2013). The same is true for the Trigynobrathys s.str. and the Brathys clade when compared to the ages revealed in Meseguer et al. (2013). According to our divergence time estimation, the subclade within Trigynobrathys s.str. containing the Neotropical “lowland” species diversified 4.1 Ma ago (2.2–6.5 95% HPD). The Páramo clade of Hypericum is 3.8 Ma old (2.3–5.6 95% HPD), with slightly younger estimates for the core Páramo clade (3.3 Ma, 1.9–4.8 95% HPD).
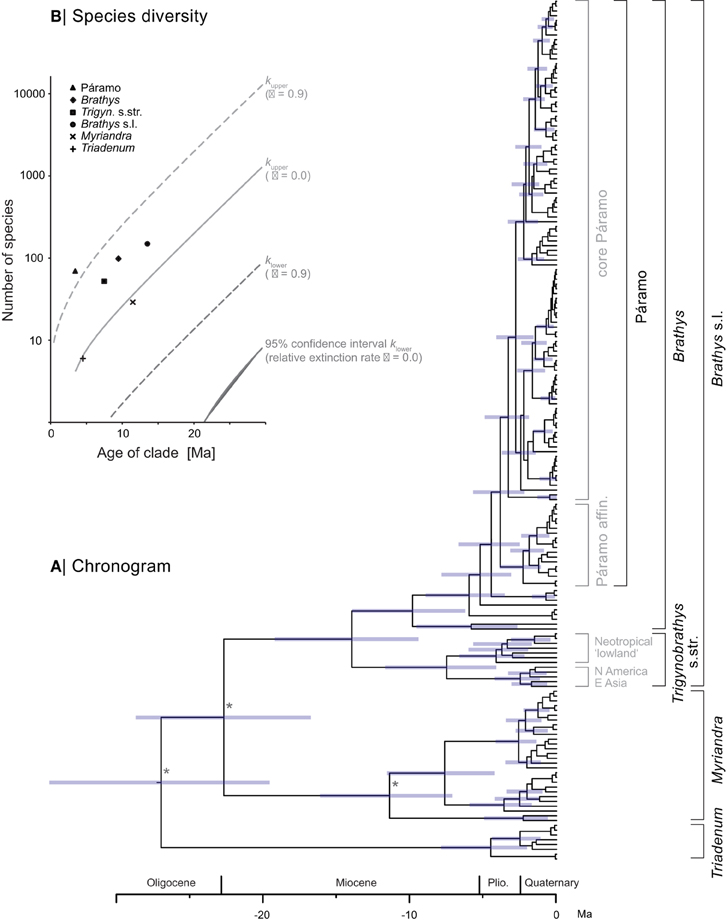
Figure 3. Maximum clade credibility chronogram (A) of New World Hypericum produced from Bayesian divergence time estimations. Asterisks mark nodes, which were time constrained for calibration. Clade names not considered in B are given in gray. (B) Test of exceptional species richness plotted for present species diversity (points) and expected (95% confidence interval, lines), assuming no extinction (solid lines, ε = 0) and high rates of extinction (dashed lines, ε = 0.9), based on crown ages. Note the species richness in the Páramo clade of Hypericum is higher than expected, under both high rates of extinction and no extinction.
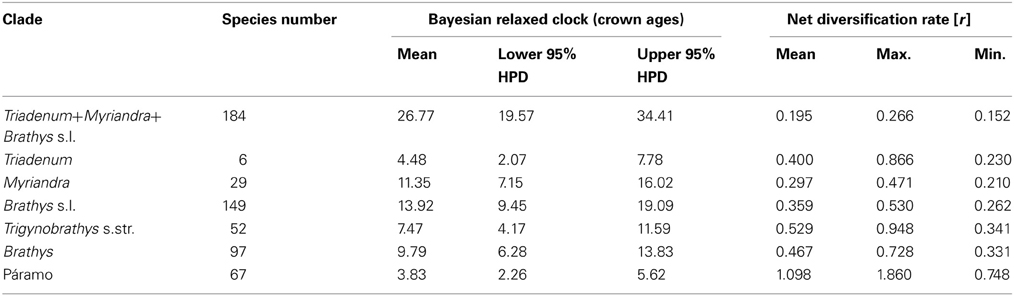
Table 2. Crown group ages of major clades in New World Hypericum and net diversification rates, calculated on the given number of species for the mean Bayesian posterior age estimate, and the lower (rMax.) and upper (rMin.) 95% highest posterior density (HPD).
Based on the ages obtained by Bayesian divergence time analysis, estimation of net diversification rates (r) under a Yule model and considering taxonomic richness reveals a higher rate for the Páramo clade of Hypericum [r = (0.75−) 1.10 (−1.86)] compared to all other clades (summarized in Table 2). The test of excessive species richness (Figure 3B) shows extraordinary species diversity in the Páramo clade when assuming a high relative extinction rate (ε = 0.9) for the entire phylogeny. The same is true when extinction is assumed to be zero (ε = 0.0). In the latter, however, all clades within the Brathys s.l. clade, that is, all clades containing Neotropical species, are revealed to be more species-rich than expected when compared to the background net diversification rate of the entire phylogeny.
Discussion
Our analysis confirms the monophyly of Myriandra and Brathys s.l and corroborates the relationships among key lineages recovered in previous studies (Meseguer et al., 2013; Nürk et al., 2013). Four major clades, Triadenum, Myriandra, Trigynobrathys s.str., and Brathys comprise around 184 Hypericum species, which constitute ca. 90% of the species diversity of Hypericum in the New World (Robson, 2012). Nevertheless, it partially rejects the traditional infrageneric classification (Robson, 1977 onwards), revealing sect. Trigynobrathys (Robson, 1990) to be non-monophyletic2, a result reported also in other studies (Nürk and Blattner, 2010; Meseguer et al., 2013; Nürk et al., 2013). The extensive sampling in this study highlights the number of species, which group in a polyphyletic position.
What emerges from our analyses is striking evidence that Neotropical species, which group within two distantly related clades, underwent two independent biogeographic histories, as might be expected from their phylogenetic position. Moreover, both lineages exhibit different ecologies and life forms: one containing herbaceous species native to the low- and uplands of South America (Trigynobrathys s.str., subclade Neotropic “lowland”), the other mostly shrubby high Andean species (the Páramo clade). Thus, we assume that dispersal into South America occurred twice in two independent lineages within Hypericum. This is in contrast to Meseguer et al. (2013), who revealed a single dispersal to South America (which is due to restricted sampling of New World species in their analysis). Further conclusions demand formal biogeographic analyses on the base of a sound phylogeny with comprehensive species sampling, to reveal the spatiotemporal evolution of species and source areas of dispersals. Similarly, the inferred monophyly of the páramo species of Hypericum, and thus, the hypothesis of secondary evolution of “lowland” species (H. silenoides; placed in derived positions within the Páramo affinis clade) needs to be tested by ancestral area reconstructions incorporating altitudinal variation. Until then, monophyly of páramo Hypericum is the parsimonious hypothesis and the conditional diversification rates (see below) are conservative estimates.
Bayesian relaxed clock analyses of rDNA ITS revealed age estimates, which are in congruence to those inferred from analyses of cpDNA sequence divergence (Meseguer et al., 2013). To the best of our knowledge, this is the first report of divergence times in Hypericum, which have been estimated from nuclear data. According to these estimates (Figure 3A; Table 2), the stem lineages of the major clades, Myriandra, Trigynobrathys s.str., and Brathys, diverged during the Miocene (5.3–23.0 Ma ago), with an early split of Triadenum from Myriandra+Brathys s.l. in the Oligocene (23.0–33.9 Ma ago), and Myriandra from Brathys s.l. at the Oligocene/Miocene boundary (ca. 23 Ma ago). In contrast, the main diversification within the major clades is revealed to have taken place from the Pliocene (5.3 Ma) onwards (Figure 3). Within the Páramo clade of Hypericum, the inferred time of the onset of species divergence (ca. 3.3–3.8 Ma ago) coincides with the final uplift of the Andes, and thus, with the (early) emergence of the páramos (Gregory-Wodzicki, 2000; Hooghiemstra et al., 2006; Graham, 2009, 2011b). Moreover, the fossil record for Hypericum in Andean high valleys (pollen fossils; van der Hammen et al., 1973; Wijninga and Kuhry, 1990; Wijninga, 1996) reported for the Late Pliocene (2.5–3.6 Ma ago) is in good agreement with the revealed age estimates. Nevertheless, the used secondary calibration approach (calibrating the nodes of the tree with the estimated ages of another study) is problematic in the sense that the uncertainty produced in the original study is accumulated in our analysis.
Although topology and age estimates inferred from ITS sequence variation is highly congruent to those reported from chloroplast sequence analyses (Meseguer et al., 2013), the multi-copy nature of the 18S–ITS1–5.8S–ITS2–26S nuclear ribosomal cistron potentially confounds species tree reconstruction due to the possible presence of paralogs (derived from gene duplication) or pseudogenes (non-functional copies; Álvarez and Wendel, 2003; Bailey et al., 2003). Intra-individual rDNA polymorphism in Hypericum has been documented by Nürk et al. (2013), suggesting incomplete concerted evolution. If not artifactual, evidence for polymorphic ITS types has also been found in this study (indicated by double peaks in the chromatograms). On the other hand, the polymorphisms observed within an individual do not exceed that expected for a heterozygous individual (e.g., Muir et al., 2001). The direct sequencing approach used here, however, does not permit full investigation of ITS copy variation. While comparison with cpDNA phylogenetics (Meseguer et al., 2013) indicates no cases of ITS paralogy across major clades, the position of several species in multiple subclades of the Páramo clade (e.g., H cardonae, H. juniperinum, H. prostratum; Figure 2), could be evidence that divergent ITS lineages are present within individuals (deep paralogy sensu Bailey et al., 2003; duplication and divergence prior to speciation). Multiple ITS types within species could also result from hybridization and allopolyploidization (Emshwiller and Doyle, 1998, 2002; Blattner, 2004; Soltis et al., 2008; Kiefer and Koch, 2012). The sympatric occurrence of species (e.g., H. mexicanum with H. juniperinum, or H. strictum with H. tetrastichum, H. prostratum, and H. selaginella) provides the background in which hybridization is possible. Hence, gene trees and reticulate evolution could confound species relationships within the Páramo clade of Hypericum. Also, the possible presence of non-functional ITS copies (pseudogenes) could result in long branch attraction (Felsenstein, 1978), or repulsion (Siddall and Whiting, 1999). If present, pseudogenes affect divergence time estimations by accumulating substitutions that cause increased branch length estimates, which, in turn, result in earlier divergence time estimates. Comparison to future studies analyzing chloroplast sequence divergence or single/low copy genes and employing a comprehensive species sampling will reveal the influence of possibly included pseudogenes and the accuracy of the age estimates provided in the present study. Hence, ages reported here can be used as a conservative estimate, when implemented in diversification rate estimations.
We calculated net diversification rates (r), in which taxonomic richness of clades is considered (Table 2). The taxonomic likelihood approximation (Magallón and Sanderson, 2001) reveals a two-fold higher net diversification rate for the Páramo clade (mean: 1.1 speciation events per million year), compared to the Trigynobrathys s.str. clade (0.5 sp/Ma), i.e., the second group containing Neotropical species. The inferred rate of net diversification of páramo Hypericum is in the range reported from other high Andean plant groups, e.g., Halenia with 1.0 sp/Ma (Kadereit and von Hagen, 2003; von Hagen and Kadereit, 2003), Gentianella with 1.7 sp/Ma (von Hagen and Kadereit, 2001), or Lupinus with exceptional 1.9–3.7 sp/Ma (Hughes and Eastwood, 2006). When minimum and maximum rates of Páramo Hypericum are considered (0.75–1.86 sp/Ma), it is similar to the average net diversification rate of the páramos estimated as 1.36 sp/Ma (Madriñán et al., unpublished data).
That is, the Neotropical radiation in Hypericum seems to be related to the emergence of high-altitude habitats, as unexpected high species richness is mainly detected in the Páramo clade, and is less pronounced in the second Neotropical clade containing “lowland” species. We hypothesize that the availability of the “new” páramo habitats have had a causal impact on diversification of Neotropical Hypericum, allowing species to adapt into different niches within these newly emerging ecosystem and to rapidly diversify. A further possibility is that Pleistocene climatic fluctuations, repeated fragmentations of the “sky-island”-like páramo habitats promoted allopatric speciation leading to increased diversification (Rauscher, 2002; Hughes and Eastwood, 2006; Moore and Donoghue, 2007). Additionally, the diversification analysis is inference of acceleration from method of moments (Magallón and Sanderson, 2001), as the Yule model assumes constant rates over time. Punctual extinction or decreasing extinction rates might produce a similar pattern (Antonelli and Sanmartín, 2011b; Crisp and Cook, 2011; Stadler, 2013) that might not be associated to an increase in speciation in Hypericum in the páramos. These hypotheses need to be tested and compared to the influence of morphological, physiological, and spatiotemporal patterns to reveal causal cohesive motives underlying the observed extraordinary species richness (de Aguiar et al., 2009; Moore and Donoghue, 2009; Crisp et al., 2011; Stadler, 2011).
Further investigations on the impact of traits and/or events on diversification of páramo Hypericum demands a supported phylogeny for this group, which is not provided in the ITS data. Likewise, the amount and influence of hybridization and/or incomplete lineage sorting needs to be investigated by incorporation of both further nuclear and chloroplast markers (Rieseberg and Soltis, 1991; Jakob and Blattner, 2006; Carine et al., 2007). Comparison of nuclear low copy genes and cpDNA marker will offer to investigate the amount of reticulation, and incomplete lineage sorting/introgression (Peters et al., 2007; Nosil et al., 2009; Brassac et al., 2012) involved in diversification of Hypericum in the Andes.
To summarize, we conclude that only one lineage in Hypericum dispersed and diversified in the páramos. The age estimate for the Páramo clade of Hypericum correlates with the early emergence of high-elevation Andean grasslands. Based on these age estimates, extraordinary diversification is inferred for páramo Hypericum, as species numbers within these high-Andean grasslands are excessively rich. Great phenotypic diversity has evolved in a short time frame (3.3–3.8 Ma) in páramo habitats, although low genotypic differentiation is observed in the nuclear rDNA. Thus, keeping in mind the limitations discussed above, we propose that adaptive radiation—ecological and phenotypic diversity driven by intraspecific selection (on regulatory divergence rather than protein structure; Schluter, 2000; Losos, 2010; Mariac et al., 2010)—under strong ecological pressure caused the morphological diversity. That is, the rapid radiation in páramo Hypericum has likely been promoted by the uplift of the Andes, via adaptive radiation and/or (allopatric) speciation induced by the orogeny and topography of the northern Andean Cordilleras.
Conflict of Interest Statement
The authors declare that the research was conducted in the absence of any commercial or financial relationships that could be construed as a potential conflict of interest.
Acknowledgments
We thank editors F. Luebert and L. Muller. Graham Muir, Nora Hohmann, and two reviewers are thanked for valuable comments on an earlier version of the manuscript. We are grateful to Antoine Cleef for precious insights into the páramo ecosystem, Colin Hughes for samples from Ecuador, and Sara Crockett, Jaanus Paal, and Guy Atchison for their pictures used in Figure 1, and Andrea Meseguer for her original MCC tree file. This work was supported by a grant to NMN from the Deutsche Forschungsgemeinschaft (DFG Grant NU 292/1).
Footnotes
1. ^The remaining 10% of the Hypericum species native to the New World, which are classified sensu Robson (1985, 2001, 2002, 2006) in sects. Umbraculoides (1 spec.), Roscyna (1 subsp.), Hypericum (1 native, 1 introduced spec.), Concinna (1 spec.), and Graveolentia (9 spec.), are distributed in North and Central America and belong phylogenetically to Hypericum clades remote from the large New World clade described above, and were thus, not included in this study.
2. ^Hence, we refer with Brathys s.l. to sects. Brathys + Trigynobrathys sensu Robson (1977 onwards), and with Trigynobrathys s.str. to the East Asian-North American species (H. japonicum, H. gramineum, etc.) and the species native to lowland and upland areas of South America (H. brasiliense, H. connatum, etc.). Consequently, Brathys refers to sect. Brathys including sect. Trigynobrathys pro parte.
References
Akaike, H. (1974). New look at statistical-model identification. IEEE Trans. Automat. Contr. AC-19, 716–723. doi: 10.1109/TAC.1974.1100705
Álvarez, I., and Wendel, J. F. (2003). Ribosomal ITS sequences and plant phylogenetic inference. Mol. Phylogenet. Evol. 29, 417–434. doi: 10.1016/S1055-7903(03)00208-2
Antonelli, A. A., Nylander, J. A. A., Persson, C., and Sanmartín, I. (2009). Tracing the impact of the Andean uplift on Neotropical plant evolution. Proc. Natl. Acad. Sci. U.S.A. 106, 9749–9754. doi: 10.1073/pnas.0811421106
Antonelli, A. A., and Sanmartín, I. (2011a). Why are there so many plant species in the Neotropics? Taxon 60, 403–414.
Antonelli, A. A., and Sanmartín, I. (2011b). Mass extinction, gradual cooling, or rapid radiation? Reconstructing the spatiotemporal evolution of the ancient angiosperm genus Hedyosmum (Chloranthaceae) using empirical and simulated approaches. Syst. Biol. 60, 596–615. doi: 10.1093/sysbio/syr062
Bailey, C. D., Carr, T. G., Harris, S. T., and Hughes, C. E. (2003). Characterization of angiosperm nrDNA polymorphism, paralogy, and pseudogenes. Mol. Phylogenet. Evol. 29, 435–455. doi: 10.1016/j.ympev.2003.08.021
Baldwin, B. G. (1992). Phylogenetic utility of the internal transcribed spacers of nuclear ribosomal DNA in plants: an example from the Compositae. Mol. Phylogenet. Evol. 1, 3–16. doi: 10.1016/1055-7903(92)90030-K
Baldwin, B. G., Sanderson, M. J., Porter, J. M., Wojciechowski, M. F., Campbell, C. S., and Donoghue, M. J. (1995). The ITS region of nuclear ribosomal DNA – A valuable source of evidence on angiosperm phylogeny. Ann. Mo. Bot. Gard. 82, 247–277. doi: 10.2307/2399880
Bell, C. D., and Donoghue, M. J. (2005). Phylogeny and biogeography of Valerianaceae (Dipsacales) with special reference to the South American valerians. Org. Divers. Evol. 5, 147–159. doi: 10.1016/j.ode.2004.10.014
Blattner, F. R. (1999). Direct amplification of the entire ITS region from poorly preserved plant material using recombinant PCR. Biotechniques 27, 1180–1186.
Blattner, F. R. (2004). Phylogenetic analysis of Hordeum (Poaceae) as inferred by nuclear rDNA ITS sequences. Mol. Phylogenet. Evol. 33, 289–299. doi: 10.1016/j.ympev.2004.05.012
Brassac, J., Jakob, S. S., and Blattner, F. R. (2012). Progenitor-derivative relationships of Hordeum polyploids (Poaceae, Triticeae) inferred from sequences of TOPO6, a nuclear low-copy gene region. PLoS ONE 7:e33808. doi: 10.1371/journal.pone.0033808
Carine, M. A., Robba, L., Little, R., Russell, S., and Guerra, A. S. (2007). Molecular and morphological evidence for hybridization between endemic Canary Island Convolvulus. Bot. J. Linn. Soc. 154, 187–204. doi: 10.1111/j.1095-8339.2007.00657.x
Crisp, M. D., and Cook, L. G. (2011). Cenozoic extinctions account for the low diversity of extant Gymnosperms compared with Angiosperms. New Phytol. 192, 997–1009. doi: 10.1111/j.1469-8137.2011.03862.x
Crisp, M. D., Trewick, S. A., and Cook, L. G. (2011). Hypothesis testing in biogeography. Trends Ecol. Evol. 26, 66–72. doi: 10.1016/j.tree.2010.11.005
Crockett, S. L., Eberhardt, M., Kunert, O., and Schuhly, W. (2010). Hypericum species in the Paramos of Central and South America: a special focus upon H. irazuense Kuntze ex N. Robson. Phytochem. Rev. 9, 255–269. doi: 10.1007/s11101-009-9148-2
Cuatrecasas, J. (1986). “Speciation and radiation of the Espeletiinae in the Andes,” in High Altitude Tropical Biogeography, eds F. Vuilleumier and M. Monasterio (New York, NY: Oxford University Press), 267–303.
de Aguiar, M. A. M., Baranger, M., Baptestini, E. M., Kaufman, L., and Bar-Yam, Y. (2009). Global patterns of speciation and diversity. Nature 460, 384–387. doi: 10.1038/nature08168
Drummond, A. J., Ho, S. Y. W., Phillips, M. J., and Rambaut, A. (2006). Relaxed phylogenetics and dating with confidence. PLoS Biol. 4:699–710. doi: 10.1371/journal.pbio.0040088
Drummond, A. J., and Rambaut, A. (2007). BEAST: Bayesian evolutionary analysis by sampling trees. BMC Evol. Biol. 7:214. doi: 10.1186/1471-2148-7-214
Drummond, C. S., Eastwood, R. J., Miotto, S. T. S., and Hughes, C. E. (2012). Multiple continental radiations and correlates of diversification in Lupinus (Leguminosae): testing for key innovation with incomplete taxon sampling. Syst. Biol. 61, 443–460. doi: 10.1093/sysbio/syr126
Emshwiller, E., and Doyle, J. J. (1998). Origins of domestication and polyploidy in oca (Oxalis tuberosa : Oxalidaceae): nrDNA ITS data. Am. J. Bot. 85, 975–985. doi: 10.2307/2446364
Emshwiller, E., and Doyle, J. J. (2002). Origins of domestication and polyploidy in oca (Oxalis tuberosa: Oxalidaceae). 2. Chloroplast-expressed glutamine synthetase data. Am. J. Bot. 89, 1042–1056. doi: 10.3732/ajb.89.7.1042
Felsenstein, J. (1978). Cases in which parsimony or compatibility methods will be positively misleading. Syst. Zool. 27, 401–410. doi: 10.2307/2412923
Felsenstein, J. (1981). Evolutionary trees from DNA sequences: a maximum likelihood approach. J. Mol. Evol. 17, 368–376. doi: 10.1007/BF01734359
Gehrke, B., and Linder, H. P. (2009). The scramble for Africa: pan-temperate elements on the African high mountains. Proc. R. Soc. B Biol. Sci. 276, 2657–2665. doi: 10.1098/rspb.2009.0334
Glor, R. E. (2010). Phylogenetic insights on adaptive radiation. Annu. Rev. Ecol. Evol. Syst. 41, 251–270. doi: 10.1146/annurev.ecolsys.39.110707.173447
Graham, A. (2009). The Andes: a geological overview from a biological perspective. Ann. Mo Bot. Gard. 96, 371–385. doi: 10.3417/2007146
Graham, A. (2011a). A Natural History of the New World. Chicago, IL: The University of Chicago Press.
Graham, A. (2011b). The age and diversification of terrestrial New World ecosystems through Cretaceous and Cenozoic time. Am. J. Bot. 98, 336–351.
Gregory-Wodzicki, K. M. (2000). Uplift history of the Central and Northern Andes: a review. Geol. Soc. Am. Bull. 112, 1091–1105.
Harvey, P. H., and Pagel, M. D. (1991). The Comparative Method in Evolutionary Biology. Oxford; New York; Tokyo: Oxford University Press.
Hooghiemstra, H., Wijninga, V., and Cleef, A. (2006). The paleobotanical record of Colombia: implications for biogeography and biodiversity. Ann. Mo. Bot. Gard. 93, 297–325.
Hoorn, C., Wesselingh, F., Ter Steege, H., Bermudez, M., Mora, A., Sevink, J., et al. (2010). Amazonia through time: Andean uplift, climate change, landscape evolution, and biodiversity. Science 330, 927–931. doi: 10.1126/science.1194585
Huelsenbeck, J. P., and Rannala, B. (1997). Phylogenetic methods come of age: testing hypotheses in an evolutionary context. Science 276, 227–232. doi: 10.1126/science.276.5310.227
Hughes, C. E., and Eastwood, R. (2006). Island radiation on a continental scale: exceptional rates of plant diversification after uplift of the Andes. Proc. Natl. Acad. Sci. U.S.A. 103, 10334–10339. doi: 10.1073/pnas.0601928103
Jakob, S. S., and Blattner, F. R. (2006). A chloroplast genealogy of Hordeum (Poaceae): long-term persisting haplotypes, incomplete lineage sorting, regional extinction, and the consequences for phylogenetic inference. Mol. Biol. Evol. 23, 1602–1612. doi: 10.1093/molbev/msl018
Kadereit, J. W., and von Hagen, K. B. (2003). The evolution of flower morphology in Gentianaceae-Swertiinae and the roles of key innovations and niche width for the diversification of Gentianella and Halenia in South America. Int. J. Plant Sci. 164, 441–452. doi: 10.1086/376880
Katoh, K. (2005). MAFFT version 5: improvement in accuracy of multiple sequence alignment. Nucleic Acids Res. 33, 511–518. doi: 10.1093/nar/gki198
Katoh, K., Misawa, K., Kuma, K.-I., and Miyata, T. (2002). MAFFT: a novel method for rapid multiple sequence alignment based on fast Fourier transform. Nucleic Acids Res. 30, 3059–3066. doi: 10.1093/nar/gkf436
Kiefer, C., and Koch, M. A. (2012). A continental-wide perspective: the genepool of nuclear encoded ribosomal DNA and single-copy gene sequences in North American Boechera (Brassicaceae). PLoS ONE 7:e36491. doi: 10.1371/journal.pone.0036491
Losos, J. B. (2010). Adaptive radiation, ecological opportunity, and evolutionary determinism. Am. Nat. 175, 623–639. doi: 10.1086/652433
Luebert, F., Hilger, H. H., and Weigend, M. (2011). Diversification in the Andes: age and origins of South American Heliotropium lineages (Heliotropiaceae, Boraginales). Mol. Phylogenet. Evol. 61, 90–102. doi: 10.1016/j.ympev.2011.06.001
Luteyn, J. L. (1999). Páramos: a Checklist of Plant Diversity, Geographical Distribution, and Botanical Literature. New York, NY: Scientific Publications Department New York Botanical Garden.
Luteyn, J. L. (2002). Diversity, adaptation, and endemism in Neotropical Ericaceae: biogeographical patterns in the Vaccinieae. Bot. Rev. 68, 55–87. doi: 10.1663/0006-8101(2002)068[0055:DAAEIN]2.0.CO;2
Magallón, S. S., and Sanderson, M. J. (2001). Absolute diversification rates in Angiosperm clades. Evolution 55, 1762–1780. doi: 10.1111/j.0014-3820.2001.tb00826.x
Mariac, C., Jehin, L., Saïdou, A.-A., Thuillet, A.-C., Couderc, M., Sire, P., et al. (2010). Genetic basis of pearl millet adaptation along an environmental gradient investigated by a combination of genome scan and association mapping. Mol. Ecol. 20, 80–91. doi: 10.1111/j.1365-294X.2010.04893.x
Mau, B., Newton, M. A., and Larget, B. (1999). Bayesian phylogenetic inference via Markov chain Monte Carlo methods. Biometrics 55, 1–12. doi: 10.1111/j.0006-341X.1999.00001.x
Meseguer, A. S., Aldasoro, J. J., and Sanmartín, I. (2013). Bayesian inference of phylogeny, morphology and range evolution reveals a complex evolutionary history in St. John's wort (Hypericum). Mol. Phylogenet. Evol. 67, 379–403. doi: 10.1016/j.ympev.2013.02.007
Moore, B. R., and Donoghue, M. J. (2007). Correlates of diversification in the plant clade Dipsacales: geographic movement and evolutionary innovations. Am. Nat. 170, S28–S55. doi: 10.1086/519460
Moore, B. R., and Donoghue, M. J. (2009). A Bayesian approach for evaluating the impact of historical events on rates of diversification. Proc. Natl. Acad. Sci. U.S.A. 106, 4307–4312. doi: 10.1073/pnas.0807230106
Moraes, I. C. R., Pinto-Maglio, C. A. F., and Lombello, R. A. (2009). Reproductive biology and cytology of Hypericum brasiliense Choisy (Hypericaceae). Revista Brasileira de Botánica 32, 539–544.
Muir, G., Fleming, C. C., and Schlötterer, C. (2001). Three divergent rDNA clusters predate the species divergence in Quercus petraea (Matt.) Liebl. and Quercus robur L. Mol. Biol. Evol. 18, 112–119. doi: 10.1093/oxfordjournals.molbev.a003785
Myers, N., Mittermeier, R. A., Mittermeier, C. G., da Fonseca, G. A. B., and Kent, J. (2000). Biodiversity hotspots for conservation priorities. Nature 403, 853–858. doi: 10.1038/35002501
Nieto Feliner, G., and Rosselló, J. A. (2007). Better the devil you know? Guidelines for insightful utilization of nrDNA ITS in species-level evolutionary studies in plants. Mol. Phylogenet. Evol. 44, 911–919. doi: 10.1016/j.ympev.2007.01.013
Nosil, P., Harmon, L. J., and Seehausen, O. (2009). Ecological explanations for (incomplete) speciation. Trends Ecol. Evol. 24, 145–156. doi: 10.1016/j.tree.2008.10.011
Nürk, N. M., and Blattner, F. R. (2010). Cladistic analysis of morphological characters in Hypericum (Hypericaceae). Taxon 59, 1495–1507.
Nürk, N. M., Madriñán, S., Carine, M. A., Chase, M. W., and Blattner, F. R. (2013). Molecular phylogenetics and morphological evolution of St. John's wort (Hypericum; Hypericaceae). Mol. Phylogenet. Evol. 66, 1–16. doi: 10.1016/j.ympev.2012.08.022
Nylander, J. A. A. (2004). MrModeltest. v 2.3 ed. Program distributed by the author. Uppsala: Evolutionary Biology Centre, Uppsala University.
Pagel, M. (1999). Inferring the historical patterns of biological evolution. Nature 401, 877–884. doi: 10.1038/44766
Paradis, E., Claude, J., and Strimmer, K. (2004). APE: analyses of phylogenetics and evolution in R language. Bioinformatics 20, 289–290. doi: 10.1093/bioinformatics/btg412
Peters, J. L., Zhuravlev, Y., Fefelov, I., Logie, A., and Omland, K. E. (2007). Nuclear loci and coalescent methods support ancient hybridization as cause of mitochondrial paraphyly between gadwall and falcated duck (Anas spp.). Evolution 61, 1992–2006. doi: 10.1111/j.1558-5646.2007.00149.x
Posada, D., and Buckley, T. R. (2004). Model selection and model averaging in phylogenetics: advantages of Akaike information criterion and Bayesian approaches over likelihood ratio tests. Syst. Biol. 53, 793–808. doi: 10.1080/10635150490522304
R Development Core Team (2013). “R”. Vienna: R foundation for statistical computing. Available online at: http://www.R-project.org
Rambaut, A. (2006–2013). “FigTree v1.4”. Available online at: http://tree.bio.ed.ac.uk/software/figtree/
Rambaut, A., and Drummond, A. J. (2007). “Tracer v1.4”. Available online at: http://beast.bio.ed.ac.uk/Tracer
Rauscher, J. T. (2002). Molecular phylogenetics of the Espeletia complex (Asteraceae): evidence from nrDNA ITS sequences on the closest relatives of an Andean adaptive radiation. Am. J. Bot. 89, 1074–1084. doi: 10.3732/ajb.89.7.1074
Ricklefs, R., and Latham, R. (1992). Intercontinental correlation of geographical ranges suggests stasis in ecological traits of relict genera of temperate perennial herbs. Am. Nat. 139, 1305–1321. doi: 10.1086/285388
Rieseberg, L. H., and Soltis, D. E. (1991). Phylogenetic consequences of cytoplasmic gene flow in plants. Evol. Trends Plants 5, 65–84.
Robson, N. K. B. (1977). Studies in the genus Hypericum L. (Guttiferae): 1. Infrageneric classification. Bull. Brit. Mus. Nat. Hist. Bot. 5, 291–355.
Robson, N. K. B. (1985). Studies in the genus Hypericum L. (Guttiferae): 3. Sections 1. Campylosporus to 6a. Umbraculoides. Bull. Brit. Mus. Nat. Hist. Bot. 12, 1–325.
Robson, N. K. B. (1987). Studies in the genus Hypericum L. (Guttiferae): 7. Section 29. Brathys (part 1). Bull. Brit. Mus. Nat. His. Bot. 16, 1–106.
Robson, N. K. B. (1990). Studies in the genus Hypericum L. (Guttiferae): 8. Sections 29. Brathys (part 2) and 30. Trigynobrathys. Bull. Nat. Hist. Mus. Bot. Lond. 20, 1–151.
Robson, N. K. B. (2001). Studies in the genus Hypericum L. (Guttiferae) 4(1). Sections 7. Roscyna to 9. Hypericum sensu lato (part 1). Bull. Nat. Hist. Mus. Bot. Lond. 31, 37–88.
Robson, N. K. B. (2002). Studies in the genus Hypericum L. (Guttiferae) 4(2). Section 9. Hypericum sensu lato (part 2): subsection 1. Hypericum series 1. Hypericum. Bull. Nat. His. Mus. Bot. Lond. 32, 61–123.
Robson, N. K. B. (2006). Studies in the genus Hypericum L. (Clusiaceae). Section 9. Hypericum sensu lato (part 3): subsection 1. Hypericum series 2. Senanensia, subsection 2. Erecta and section 9b. Graveolentia. Syst. Biodivers. 4, 19–98. doi: 10.1017/S1477200005001842
Robson, N. K. B. (2012). Studies in the genus Hypericum L. (Hypericaceae) 9. Addenda, corrigenda, keys, lists and general discussion. Phytotaxa 72, 1–111.
Ronquist, F., and Huelsenbeck, J. P. (2003). MrBayes 3: bayesian phylogenetic inference under mixed models. Bioinformatics 19, 1572–1574. doi: 10.1093/bioinformatics/btg180
Ruhfel, B., Bittrich, V., Bove, C., Gustafsson, M., Philbrick, C., Rutishauser, R., et al. (2011). Phylogeny of the clusioid clade (Malpighiales): evidence from the plastid and mitochondrial genomes. Am. J. Bot. 98, 306–325. doi: 10.3732/ajb.1000354
Sanderson, M. J. (2002). Estimating absolute rates of molecular evolution and divergence times: a penalized likelihood approach. Mol. Biol. Evol. 19, 101–109. doi: 10.1093/oxfordjournals.molbev.a003974
Schluter, D. (2000). The Ecology of Adaptive Radiation. Oxford; New York;, NY: Oxford University Press.
Siddall, M. E., and Whiting, M. F. (1999). Long-branch abstractions. Cladistics 15, 9–24. doi: 10.1111/j.1096-0031.1999.tb00391.x
Silvestro, D., and Michalak, I. (2011). raxmlGUI: a graphical front-end for RAxML. Org. Divers. Evol. 12, 335–337. doi: 10.1007/s13127-011-0056-0
Sklenár, P., Dušková, E., and Balslev, H. (2010). Tropical and temperate: evolutionary history of páramo flora. Bot. Rev. 77, 71–108. doi: 10.1007/s12229-010-9061-9
Smith, J., and Cleef, A. (1988). Composition and origins of the world's tropicalpine floras. J. Biogeogr. 15, 631–645. doi: 10.2307/2845441
Soltis, D. E., Mavrodiev, E., Doyle, J. J., Rauscher, J., and Soltis, S. P. (2008). ITS and ETS sequence data and phylogeny reconstruction in allopolyploids and hybrids. Syst. Bot. 33, 7–20. doi: 10.1600/036364408783887401
Stadler, T. (2009). On incomplete sampling under birth-death models and connections to the sampling-based coalescent. J. Theor. Biol. 261, 58–66. doi: 10.1016/j.jtbi.2009.07.018
Stadler, T. (2011). Inferring speciation and extinction processes from extant species data. Proc. Natl. Acad. Sci. U.S.A. 108, 16145–16146. doi: 10.1073/pnas.1113242108
Stadler, T. (2013). Recovering speciation and extinction dynamics based on phylogenies. J. Evol. Biol. 26, 1203–1219.
Stamatakis, A. (2006). RAxML-VI-HPC: maximum likelihood-based phylogenetic analyses with thousands of taxa and mixed models. Bioinformatics 22, 2688–2690. doi: 10.1093/bioinformatics/btl446
Stamatakis, A., Hoover, P., and Rougemont, J. (2008). A rapid bootstrap algorithm for the RAxML web servers. Syst. Biol. 57, 758–771. doi: 10.1080/10635150802429642
Stephens, P. R., and Wiens, J. J. (2003). Explaining species richness from continents to communities: the time-for-speciation effect in emydid turtles. Am. Nat. 161, 112–128. doi: 10.1086/345091
Sudhaus, W. (2004). Radiation within the framework of evolutionary ecology. Org. Divers. Evol. 4, 127–134. doi: 10.1016/j.ode.2004.04.001
Swofford, D. L. (2002). PAUP*. Phylogenetic Analyses Using Parsimony (*and Other Methods). v4.0b10. Sunderland: Sinauer Associates.
Vamosi, J. C., and Vamosi, S. M. (2010). Key innovations within a geographical context in flowering plants: towards resolving Darwin's abominable mystery. Ecol. Lett. 13, 1270–1279. doi: 10.1111/j.1461-0248.2010.01521.x
Vamosi, J. C., and Vamosi, S. M. (2011). Factors influencing diversification in Angiosperms: at the crossroads of intrinsic and extrinsic traits. Am. J. Bot. 98, 460–471. doi: 10.3732/ajb.1000311
van der Hammen, T., and Hooghiemstra, H. (2000). Neogene and quaternary history of vegetation, climate, and plant diversity in Amazonia. Quat. Sci. Rev. 19, 725–742. doi: 10.1016/S0277-3791(99)00024-4
van der Hammen, T., Werner, J. H., and Van Dommelen, H. (1973). Palynological record of the upheaval of the northern Andes: a study of the Pliocene and Lower Quaternary of the Colombian eastern Cordillera and the early evolution of its high-Andean biota. Rev. Palaeobot. Palynol. 16, 1–122. doi: 10.1016/0034-6667(73)90031-6
von Hagen, K. B., and Kadereit, J. W. (2001). The phylogeny of Gentianella (Gentianaceae) and its colonization of the Southern Hemisphere as revealed by nuclear and chloroplast DNA sequence variation. Org. Divers. Evol. 1, 61–79. doi: 10.1078/1439-6092-00005
von Hagen, K. B., and Kadereit, J. W. (2003). The diversification of Halenia (Gentianaceae): ecological opportunity versus key innovation. Evolution 57, 2507–2518.
Weigend, M., Gottschling, M., Hilger, H. H., and Nürk, N. M. (2010). Five new species of Lithospermum L. (Boraginaceae tribe Lithospermeae) in Andean South America: another radiation in the Amotape-Huancabamba zone. Taxon 59, 1161–1179.
Wiens, J. J., and Donoghue, M. J. (2004). Historical biogeography, ecology and species richness. Trends Ecol. Evol. 19, 639–644. doi: 10.1016/j.tree.2004.09.011
Wijninga, V. M., and Kuhry, P. (1990). A Pliocene flora from the Subachoque Valley (Cordillera Oriental, Colombia). Rev. Palaeobot. Palynol. 62, 249–254. doi: 10.1016/0034-6667(90)90091-V
Wijninga, V. M. (1996). Neogene ecology of the Salto de Tequendama site (2475 m altitude, Cordillera Oriental, Colombia): the paleobotanical record of montane and lowland forests. Rev. Palaeobot. Palynol. 92, 97–156. doi: 10.1016/0034-6667(94)00100-6
Yang, Z. H. (1993). Maximum-likelihood estimation of phylogeny from DNA sequences when substitution rates differ over sites. Mol. Biol. Evol. 10, 1396–1401.
Yang, Z. H. (1994). Maximum-likelihood phylogenetic estimation from DNA sequences with variable rates over sites: approximate methods. J. Mol. Evol. 39, 306–314. doi: 10.1007/BF00160154
Zharkikh, A. (1994). Estimation of evolutionary distances between nucleotide sequences. J. Mol. Evol. 39, 315–329. doi: 10.1007/BF00160155
Appendix
List of taxa included in the study of New World Hypericum. Information is given in the following order: Species, Voucher specimen/reference (for the sequences new to this study), ENA/GenBank ID.
H. aciculare Kunth, B. Øllgaard, J.E. Madsen & L. Christensen 74233 (BM), HG004646; H. aciculare Kunth, W.W. Quizhepe & S. Lægaard 36 (BM), HG004649; H. adpressum W.P.C.Barton, AY555865.2; H. andinum Gleason, J.C. Solomon 16104 (BM), HG004725; H. apocynifolium Small, AY555883.2; H. arbuscula Stanley & Steyerm., H.M. Hernández & A. Chacón 544 (BM), HG004734; H. brachyphyllum (Spach) Steud., AY555870.2; H. brasiliense Choisy, Al Gentry & J.C. Solomon 44755 (BM), HG004770; H. brevistylum Choisy, J.C. Solomon 15221 (BM), HG004740; H. bryoides Gleason, A.M. Cleef 10000 (BM), HG004691; H. buckleyi M.A.Curtis, AY555880.2; H. callacallanum N.Robson, J.D. Boeke 1920 (BM), HG004726; H. callacallanum N.Robson, C.E. Hughes 3109 (HEID), HG004727; H. campestre subsp. campestre Cham. & Schltdl., R. Kummrow & J.M. Silva 3245 (BM), HG004771; H. canadense L., HE653433; H. cardonae Cuatrec., N.M. Nürk & G. Atchison 527 (ANDES), HG004689; H. cardonae Cuatrec., N.M. Nürk & G. Atchison 528 (ANDES), HG004690; H. cardonae Cuatrec., D.N. Hepper 181 (BM), HG004686; H. cardonae Cuatrec., F. & R. Gonzáles 3468 (BM), HG004688; H. chapmanii W.P.Adams, AY555869.2; H. cistifolium Lam., AY555881.2; H. connatum Lam., M. Serrano, J. Villalobos, A. lliully, J.A. Peñaranda & R. Lozano 6893 (BM), HG004774; H. costaricense N.Robson, G. Davidse 24985 (BM), HG004684; H. crux-andreae (L.) Crantz, AY555874.2; H. cuatrecasii Gleason, S. Madriñán 2002 (BM), HG004709; H. cymobrathys N.Robson, N.M. Nürk & G. Atchison 538 (ANDES), HG004743; H. decandrum Turcz., B. Øllgaard, S. Lægaard, K. Thomsen, J. Korning & T. Illum 58366 (BM), HG004730; H. decandrum Turcz., J.D. Boeke & J. Jaramillo 2536 (BM), HG004728; H. densiflorum Pursh, AY555886; H. denticulatum Walter, HE653453; H. dichotomum Lam., S.A. Thompson 11251 (BM), HG004760; H. dolabriforme Vent., AY555889; H. drummondii (Grev. & Hook.) Torr. & A.Gray, KC709370; H. fasciculatum Lam., AY555868.2; H. fauriei (Triadenum japonicum) R.Keller, HE653664; H. fauriei (Triadenum japonicum) R.Keller, HE653665; H. (T. virginicum var.) fraseri (Spach) Steudel, HE653668; H. (Triadenum) fraseri (Spach) Steudel, HE653663; H. (Triadenum) fraseri (Spach) Steudel, HE653662; H. frondosum Michx., AY555887; H. galioides Lam., AY555864.2; H. garciae Pierce, N.M. Nürk & G. Atchison 494 (ANDES), HG004741; H. garciae Pierce, S. Madriñán 2063 (BM), HG004742; H. gentianoides (L.) Britton, Sterns & Poggenb., N.M. Nürk 457 (GAT), HG004757; H. gentianoides (L.) Britton, Sterns & Poggenb., HE653479; H. gentianoides (L.) Britton, Sterns & Poggenb., HE653480; H. gleasonii N.Robson, N.M. Nürk & G. Atchison 558 (ANDES), HG004750; H. gleasonii N.Robson, N.M. Nürk & G. Atchison 552 (ANDES), HG004752; H. gnidioides Seem., C. Hamilton, H. Stockwell & A. Aiello 885 (BM), HG004738; H. gnidioides Seem., W.G. D'Arcy & B. Hammel 12409 (BM), HG004739; H. goyanesii Cuatrec., A.M. Cleef 11096 (ANDES), HG004703; H. goyanesii Cuatrec., N.M. Nürk & G. Atchison 505 (ANDES), HG004704; H. goyanesii Cuatrec., S. Madriñán 2058 (BM), HG004714; H. gramineum G.Forst., EU352256; H. gramineum G.Forst., EU352257; H. harlingii N.Robson, B. Øllgaard, J.E. Madsen & S. Calderón 90595 (BM), HG004729; H. hartwegii Benth., P.M. JØrgensen, C. Ulloa, H. Vargas & P. Lozano 1246 (BM), HG004731; H. humboldtianum Steud., N.M. Nürk & G. Atchison 534 (ANDES), HG004674; H. humboldtianum Steud., N.M. Nürk & G. Atchison 577 (ANDES), HG004753; H. humboldtianum Steud., N.M. Nürk & G. Atchison 491 (ANDES), HG004755; H. humboldtianum Steud., M.A. Bello 40 (BM), HG004754; H. hypericoides subsp. hypericoides (L.) Crantz, G.R. Proctor 30665 (BM), HG004779; H. hypericoides subsp. hypericoides (L.) Crantz, M. Nee & B.F. Hansen 18653 (BM), HG004778; H. hypericoides subsp. hypericoides (L.) Crantz, M. Nee & G. Diggs 24892 (BM), HG004777; H. irazuense Kunze ex N.Robson, N. Garwood, M. Gibby, R.J. Hampshire & C.J. Humphries 316 (BM), HG004733; H. japonicum Thunb. ex Murray, HE653512; H. japonicum Thunb. ex Murray, HE653513; H. juniperinum Kunth, N.M. Nürk & G. Atchison 492 (ANDES), HG004654; H. juniperinum Kunth, N.M. Nürk & G. Atchison 566 (ANDES), HG004658; H. juniperinum Kunth, N.M. Nürk & G. Atchison 512 (ANDES), HG004672; H. juniperinum Kunth, N.M. Nürk & G. Atchison 516 (ANDES), HG004673; H. juniperinum Kunth, N.M. Nürk & G. Atchison 568 (ANDES), HG004675; H. juniperinum Kunth, N.M. Nürk & G. Atchison 541 (ANDES), HG004676; H. juniperinum Kunth, N.M. Nürk & G. Atchison 518 (ANDES), HG004677; H. juniperinum Kunth, N.M. Nürk & G. Atchison 511 (ANDES), HG004678; H. juniperinum Kunth, N.M. Nürk & G. Atchison 517 (ANDES), HG004679; H. juniperinum Kunth, N.M. Nürk & G. Atchison 542 (ANDES), HG004681; H. juniperinum Kunth, N.M. Nürk & G. Atchison 580 (ANDES), HG004682; H. juniperinum Kunth, C. García 144 (BM), HG004651; H. juniperinum Kunth, J. Aguirre & O. Rangel 32 (BM), HG004652; H. kalmianum L., N.M. Nürk 397 (GAT), HG004780; H. cf. lancifolium Gleason, N.M. Nürk & G. Atchison 540 (ANDES), HG004660; H. lancioides subsp. congestiflorum (Triana & Planch.) N.Robson, C. García 141 (BM), HG004653; H. lancioides subsp. lancioides Cuatrec., L. Holm-Nielsen & H. Balslev 23652 (BM), HG004680; H. laricifolium Juss., N.M. Nürk & G. Atchison 500 (ANDES), HG004697; H. laricifolium Juss., N.M. Nürk & G. Atchison 543 (ANDES), HG004701; H. laricifolium Juss., N.M. Nürk & G. Atchison 510 (ANDES), HG004705; H. laricifolium Juss., N.M. Nürk & G. Atchison 509 (ANDES), HG004706; H. laricifolium Juss., N.M. Nürk & G. Atchison 531 (ANDES), HG004716; H. laricifolium Juss., N.M. Nürk & G. Atchison 551 (ANDES), HG004717; H. laricifolium Juss., N.M. Nürk & G. Atchison 569 (ANDES), HG004718; H. laricifolium Juss., N.M. Nürk & G. Atchison 515 (ANDES), HG004722; H. laricifolium Juss., G.P. Lewis & P. Lozano 2868 (BM), HG004713; H. laricifolium Juss., S. Madriñán 2006 (BM), HG004710; H. laricifolium Juss., S. Madriñán 2007 (BM), HG004711; H. laricifolium Juss., X. Buitrón 223 (BM), HG004712; H. laricifolium Juss., C.E. Hughes 3110 (HEID), HG004720; H. laricifolium Juss., C.E Hughes 3119 (HEID), HG004724; H. linoides A.St.-Hil., M. Sobral s.n. (2007) (BM), HG004772; H. lissophloeus W.P.Adams, AY555885; H. llanganaticum N.Robson, B. Øllgaard, L. Holm-Nielsen, B. Boysen-Larsen, L.P. Kvist, A.R. Jensen & S. Wium-Andersen 38628 (BM), HG004650; H. lloydii (Svenson) W.P.Adams, AY555867.2; H. lobocarpum Gatt., AY555876.2; H. loxense subsp. loxense Benth., P.M. JØrgensen, C. Ulloa, H. Vargas & P. Lozano 1351 (BM), HG004735; H. lycopodioides Triana & Planch., N.M. Nürk & G. Atchison 557 (ANDES), HG004715; H. lycopodioides Triana & Planch., N.M. Nürk & G. Atchison 549 (ANDES), HG004719; H. lycopodioides Triana & Planch., S. Madriñán 2059 (BM), HG004721; H. magniflorum Cuatrec., N.M. Nürk & G. Atchison 519 (ANDES), HG004744; H. magniflorum Cuatrec., N.M. Nürk & G. Atchison 571 (ANDES), HG004749; H. magniflorum Cuatrec., A.M. Cleef 4743 (BM), HG004751; H. maguirei N.Robson, B. & C.K. Maguire 61707 (BM), HG004732; H. marahuacanum subsp. chimantaicum N.Robson, O. Huber 12051 (BM), HG004656; H. marahuacanum subsp. compactum (Triana & Planch.) N.Robson, N.M. Nürk & G. Atchison 548 (ANDES), HG004655; H. marahuacanum subsp. compactum (Triana & Planch.) N.Robson, N.M. Nürk & G. Atchison 533 (ANDES), HG004657; H. mexicanum L., N.M. Nürk & G. Atchison 490 (ANDES), HG004745; H. mexicanum L., N.M. Nürk & G. Atchison 523 (ANDES), HG004746; H. mexicanum L., N.M. Nürk & G. Atchison 564 (ANDES), HG004747; H. mexicanum L., N.M. Nürk & G. Atchison 503 (ANDES), HG004748; H. microsepalum (Torr. & A.Gray) A.Gray ex S.Watson, AY555877.2; H. myricariifolium Hieron., N.M. Nürk & G. Atchison 524 (ANDES), HG004707; H. myricariifolium Hieron., N.M. Nürk & G. Atchison 526 (ANDES), HG004723; H. myricariifolium Hieron., S. Madriñán 2029 (BM), HG004698; H. myrtifolium Lam., AY555875.2; H. nitidum Lam., AY555871.2; H. nudiflorum Michx., AY555888; H. philonotis Cham. & Schltdl., H. Förther 10060 (BM), HG004764; H. pimeleoides Planch. & Linden ex Triana & Planch., A.M. Cleef 4744 (BM), HG004693; H. polyanthemum Klotzsch ex Reichardt, M. Sobral s.n. (2007) (BM), HG004773; H. pratense Cham. & Schltdl., J. Amith & R. Santiago 1102 (BM), HG004765; H. pratense Cham. & Schltdl., J.D. Dwyer 15204 (BM), HG004763; H. pratense Cham. & Schltdl., W.D. Stevens, B.A. Krukoff & O.M. Montiel 18029 (BM), HG004768; H. prolificum L., AY555873.2; H. prostratum Cuatrec., A.M. Cleef 11194 (ANDES), HG004669; H. prostratum Cuatrec., A.M. Cleef 11156 (ANDES), HG004683; H. prostratum Cuatrec., N.M. Nürk & G. Atchison 546 (ANDES), HG004647; H. prostratum Cuatrec., N.M. Nürk & G. Atchison 555 (ANDES), HG004668; H. prostratum Cuatrec., N.M. Nürk & G. Atchison 499 (ANDES), HG004685; H. prostratum Cuatrec., A. Diaz 20 (BM), HG004670; H. prostratum Cuatrec., C. García 108 (BM), HG004671; H. quitense R.Keller, L. Holm-Nielsen, J. Jaramillo & F. Coello 29216 (BM), HG004736; H. quitense R.Keller, V. Zak & J. Jaramillo 3291 (BM), HG004737; H. quitense R.Keller, HE653606; H. rigidum subsp. rigidum A.St.-Hil., G. Hatschbach 48170 (BM), HG004775; H. ruscoides Cuatrec., N.M. Nürk & G. Atchison 570 (ANDES), HG004687; H. ruscoides Cuatrec., N.M. Nürk & G. Atchison 559 (ANDES), HG004692; H. ruscoides Cuatrec., N.M. Nürk & G. Atchison 573 (ANDES), HG004694; H. ruscoides Cuatrec., N.M. Nürk & G. Atchison 514 (ANDES), HG004695; H. selaginella N.Robson, N.M. Nürk & G. Atchison 556 (ANDES), HG004659; H. silenoides Juss., J.C. Solomon 16431 (BM), HG004762; H. silenoides Juss., L. Holm-Nielsen, J. Jaramillo & E. Bravo 23052 (BM), HG004761; H. silenoides Juss., C.E. Hughes 3113 (HEID), HG004769; H. simonsii N.Robson, J.H. Kirkbride & E. Forero 1751 (BM), HG004700; H. sphaerocarpum Michx., AY555878.2; H. sprucei N.Robson, P.M. JØrgensen, C. Ulloa & J. Caranqui 2218 (BM), HG004648; H. strictum Kunth, N.M. Nürk & G. Atchison 497 (ANDES), HG004661; H. strictum Kunth, N.M. Nürk & G. Atchison 576 (ANDES), HG004662; H. strictum Kunth, S. Madriñán 2047 (BM), HG004664; H. strictum Kunth, N.M. Nürk & G. Atchison 504 (ANDES), HG004663; H. strictum Kunth, N.M. Nürk & G. Atchison 496 (ANDES), HG004665; H. struthiolifolium Juss., D.N. Smith 4126 (BM), HG004756; H. suffruticosum W.P.Adams, HE653637; H. tenuifolium Pursh, AY555872.2; H. ternum A.St.-Hil., V. Nicolack & J.Cordeiro 63 (BM), HG004776; H. terrae-firmae Sprague & Riley, A.K. Monro 741 (BM), HG004759; H. terrae-firmae Sprague & Riley, R.G. Rees, K. Sidwell, G. Reid, R. Sundin & C. Bol 221 (BM), HG004758; H. terrae-firmae Sprague & Riley, HE653640; H. tetrapetalum Lam., AY555882.2; H. tetrastichum Cuatrec., N.M. Nürk & G. Atchison 574 (ANDES), HG004666; H. tetrastichum Cuatrec., S. Madriñán 2010 (BM), HG0S04667; H. tetrastichum Cuatrec., HE653643; H. thesiifolium Kunth, H. Förther 10416 (BM), HG004766; H. thesiifolium Kunth, W.C. Burger & R.L. Liesner 6439 (BM), HG004767; H. thesiifolium Kunth, HE653644; H. thuyoides Kunth, N.M. Nürk & G. Atchison 507 (ANDES), HG004696; H. thuyoides Kunth, N.M. Nürk & G. Atchison 581 (ANDES), HG004702; H. thuyoides Kunth, C. García 21 (BM), HG004699; H. (Triadenum) tubulosum Walter, HE653666; H. (Triadenum) virginicum L., HE653667; H. (Triadenum) walteri J.F.Gmel., HE653669; H. cf. woodianum I.M.Johnst., N.M. Nürk & G. Atchison 547 (ANDES), HG004708.
Keywords: adaptive radiation, Andes Mountains, rDNA ITS phylogenetics, Neotropics, Páramos, St. John's wort
Citation: Nürk NM, Scheriau C and Madriñán S (2013) Explosive radiation in high Andean Hypericum—rates of diversification among New World lineages. Front. Genet. 4:175. doi: 10.3389/fgene.2013.00175
Received: 27 May 2013; Accepted: 22 August 2013;
Published online: 11 September 2013.
Edited by:
Federico Luebert, Freie Universität Berlin, GermanyReviewed by:
Andrea S. Meseguer, Institute National de la research agricultural, FranceColin Hughes, University of Zurich, Switzerland
Copyright © 2013 Nürk, Scheriau and Madriñán. This is an open-access article distributed under the terms of the Creative Commons Attribution License (CC BY). The use, distribution or reproduction in other forums is permitted, provided the original author(s) or licensor are credited and that the original publication in this journal is cited, in accordance with accepted academic practice. No use, distribution or reproduction is permitted which does not comply with these terms.
*Correspondence: Nicolai M. Nürk, Department of Biodiversity and Plant Systematics, Centre for Organismal Studies Heidelberg, Heidelberg University, Im Neuenheimer Feld 345, 69120 Heidelberg, Germany e-mail: n.nuerk@cos.uni-heidelberg.de