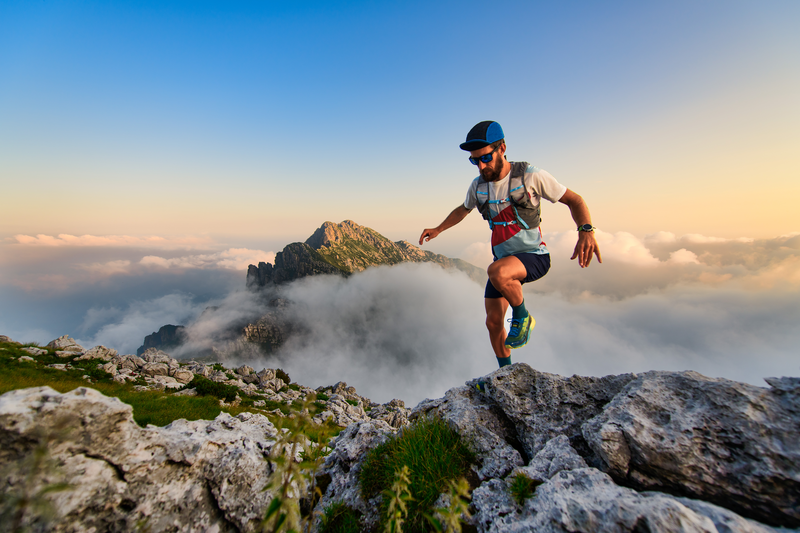
94% of researchers rate our articles as excellent or good
Learn more about the work of our research integrity team to safeguard the quality of each article we publish.
Find out more
REVIEW article
Front. Gastroenterol. , 07 November 2023
Sec. Therapy in Gastroenterology
Volume 2 - 2023 | https://doi.org/10.3389/fgstr.2023.1277094
This article is part of the Research Topic Rising Stars in Gastroenterology: 2023 View all 8 articles
Background: Since the advent of regenerative medicine, tissue engineering of the gastrointestinal tract (GIT) has been extensively studied in laboratory animals and humans. Various biologic scaffolds and cell sources have been trialed to repair or reconstruct different GIT defects. Achievements in this field have led to novel approaches in curing GIT diseases and circumventing the morbidity-related complications associated with current therapy.
Objective: This review aims to describe recent advances in GIT tissue engineering, with an emphasis on technologies with potential for clinical use.
Methods: A literature search was conducted in Ovid MEDLINE® ALL for relevant studies (2000–September 2023) using the keywords “tissue-engineering”, “scaffolds”, “organoids”, “cell-therapy”, “esophagus”, “stomach”, “small intestine”, “colon”, “rectum”, and “anus”. Articles were included if they were in vivo animal studies or clinical studies written in English that investigated tissue engineering for treating GIT defects.
Results: A total of 836 articles were identified in the initial search. Following duplicate removal, abstract, and full-text screening, 48 articles were included in the final review. Many studies on esophageal defects thus far have described the success of covering partial-thickness defects with autologous cell sheets and closing full-thickness defects with decellularized scaffolds in both animals and humans. A limited number of reports have also demonstrated the de novo organogenesis of the esophagus to repair short-segment circumferential esophageal defects with autologous pluripotent cells and scaffolds. In the stomach, multiple animal studies have reported on the feasibility of gastric epithelium regeneration using multipotent cells and/or scaffolds to correct partial- and full-thickness defects. One study observed the regeneration of whole-layer stomach defects using the organoids-on-polymer approach. Similarly, in the intestine, pluripotent cells and scaffolds were shown to effectively repair both partial- and full-thickness defects. Animal experiments have produced tissue-engineered small intestines (TESI) with the organoids-on-polymer approach. Furthermore, in the rectum and anus, mesenchymal stem cell therapies with or without bioscaffolds have shown promise for treating full-thickness defects, as demonstrated in multiple human trials.
Conclusion: Tissue-engineering approaches for repairing various types of GI defects in the esophagus, stomach, intestines, rectum, and anus have been extensively explored in animal models, with promising outcomes. Moreover, successful human trials have demonstrated the feasibility of reconstructing esophageal, rectal, and anal defects using these innovative approaches. Technologies such as mesenchymal stem cells, decellularization, organoids, and cell sheets are the most promising and closer to clinical translation. Collaboration between gastrointestinal surgery and regenerative medicine is expected to bring about novel therapeutic modalities in the future.
● Gastrointestinal tissue engineering has the potential to offer novel therapeutic solutions to repair various gastrointestinal defects and avoid the morbidity-related complications associated with current therapy.
● Many studies on esophageal defects have described the success of repairing partial- and full-thickness defects with autologous cell sheets and decellularized scaffolds in both animals and humans.
● A limited number of reports have also demonstrated the de novo organogenesis of the esophagus with autologous pluripotent cells and scaffolds to repair long-segment circumferential defects.
● In the stomach, multiple animal studies reported the feasibility of repairing partial- and full-thickness defects with multipotent cells and/or scaffolds. One study observed the regeneration of whole-layer stomach defects using the organoids-on-polymer approach.
● In the intestine, pluripotent cells and scaffolds were similarly used to successfully repair partial- and full-thickness defects. Animal experiments have produced tissue-engineered small intestines with the organoids-on-polymer approach.
● In the rectum and anus, mesenchymal stem cell therapies with or without bioscaffolds have shown promise for treating full-thickness defects (e.g., perianal fistulas), as demonstrated in multiple human trials.
● Collaboration between gastrointestinal surgery and regenerative medicine is fundamental in bringing novel therapeutic modalities in the future.
The gastrointestinal tract (GIT) is a non-redundant organ with a limited ability to regenerate. The partial or complete loss of any gastrointestinal (GI) segment has devastating and potentially life-threatening sequelae. Partial-thickness defects, such as post-endoscopic submucosal dissection (ESD), can lead to fibrosis and refractory strictures (1). Full-thickness defects, such as perforations, leaks, and fistulas, can occur due to various disease processes, trauma, or certain medical procedures, causing significant morbidity and potential mortality (2, 3). In addition, whole circumferential defects following surgical resection of the diseased GI segment are often associated with significant complications, leading to poor quality of life (4). The current medical and surgical treatments are suboptimal and are associated with various complications. There are unmet clinical needs that necessitate the development of alternative approaches for managing GI defects.
Tissue engineering and regenerative medicine are rapidly evolving fields that combine cell biology, materials science, and physiology to develop functional substitutes that either enhance repair in damaged sites or create constructs to replace the deficient tissue. Although the specific methods and materials used in tissue engineering can vary, all involve three primary elements: the cell, the supporting scaffolds, and the environmental modulator, the last of which serves to integrate and regulate the functional behavior of the first two (4).
In recent years, achievements have been made in the field of gut bioengineering that hold great promise for the development of therapeutic solutions to various GI defects and avoiding complications associated with current therapy. The purpose of this review is to describe the recent advances in tissue engineering of the gastrointestinal tract, with an emphasis on technologies that are closer to clinical translation. Some key factors to consider and challenges to overcome when transitioning GIT tissue engineering toward clinical translation will also be explored.
A narrative review of studies on GIT tissue engineering that took a systematic approach and was in accordance with PRISMA guidelines was conducted. A literature search (Appendix 1) was conducted in September 2023 using Ovid MEDLINE® ALL. Keywords included “tissue-engineering”, “regenerative medicine”, “scaffolds”, “organoids”, “cell- and tissue-based therapy”, “esophagus”, “stomach”, “small intestine”, “colon”, “rectum”, and “anus”. The search results were screened for inclusion and exclusion criteria (Table 1) and assessed for relevance by a single reviewer (YL). Studies were considered relevant if they were in vivo animal studies or clinical studies that investigated tissue engineering for treating GI defects.
The review process is summarized in the PRISMA flow diagram in Figure 1. A total of 288 articles on the esophagus, 233 articles on the stomach, 247 articles on the intestines, and 68 articles on the rectum and anus were initially identified through database searching. Duplications were then removed in EndNote (version X9.3.3). After screening by title and abstract, 57 full-text articles were retrieved and further assessed using the eligibility criteria outlined in Table 1. The reference lists of papers were also reviewed for additional relevant publications. Ultimately, 14 articles on the esophagus, 10 articles on the stomach, 7 articles on the intestines, and 17 articles on the rectum and anus were included in the final review (Figure 1). The first author, publication year, animal species or patient details, defect types, cell source, scaffolds, methodology, and outcome were collected from all included papers. Studies were grouped according to the anatomical locations and the type of defects investigated (Tables 2–6).
Partial-thickness defects of the esophagus commonly occur as a result of removing the dysplastic mucosa and/or superficial cancer tissue following ESD. If >50% of the mucosal circumference is resected, up to 70% of patients will develop fibrosis and strictures (50). Current methods to prevent post-ESD injury are limited, warranting novel approaches to stimulate mucosal regeneration. Tissue-engineering techniques such as cell therapy, cell sheet, and bioscaffolds have been studied in various animal models (Table 2) and have shown promising results in re-epithelializing the partial-thickness defects of the esophagus and reducing stricture formation (1, 5–7).
In 2010, Honda et al. carried out endoscopic injections of adipose tissue-derived stromal cells (ADSCs) into the submucosa following circumferential esophageal ESD in five dogs (5). Compared with the proportion of luminal narrowing in the control group (76%), the experimental group demonstrated a significant reduction in luminal constriction (45%). In a related context, Ohki et al. in 2006 conducted endoscopic transplantation of autologous oral mucosa epithelial cell sheets following semi-circumferential ESD in dogs (1). The cell sheets securely adhered to the wound site, effectively enhanced wound healing, and prevented the development of strictures and stenosis. Similar results were also observed by Kanai et al. in 2012 using autologous epidermal cell sheets, and by Nieponice et al. in 2009 in their application of dermal extracellular matrixes (6, 7).
In 2021, Ohki et al. attempted the first human clinical application of tissue-engineered cell sheet technology in an open-label, single-arm, single-institute study (Table 3) (13). Specimens of oral mucosal tissue were collected from nine patients with superficial esophageal cancer. Epithelial cell sheets were fabricated ex vivo from the harvested specimens. These autologous oral mucosal cell sheets were later transplanted endoscopically to the ulcer surfaces of patients who had undergone ESD of >50% of the circumference (Figure 2). These cell sheets easily adhered to the ESD site and completely re-epithelized within a median time of 3.5 weeks. With only one exception, no patients developed esophageal strictures, dysphagia, or other serious complications following the procedure.
Figure 2 Schematic explanation of cell sheet tissue engineering. Autologous cells are harvested from oral mucosa and subsequently cultured to form autologous oral mucosa epithelial cell sheets. The manufactured cell sheet is then cut into small patches, allowing endoscopic transplantation immediately after ESD procedures (13). Created with BioRender.com.
Although promising, this approach has limited practical utility as many centers do not have the expertise needed to fabricate tissue-engineered cell sheets. Hence, in 2017 Yamaguchi et al. studied the feasibility of endoscopic transplantation of oral mucosal cell sheets that had been manufactured and transported from a distant site 1,200 km away (12). In this experiment, 10 patients underwent complete circular or semicircular ESD for superficial esophageal neoplasms and were subsequently engrafted with cell sheets. Following cell sheet transplantation, re-epithelialization occurred within a median of 5 weeks without stricture formation.
Full-thickness patch defects of the esophagus, such as perforations, leaks, and fistulas, can occur spontaneously, as seen in Boerhaave syndrome, or as complications after certain procedures. These types of defects often result in esophageal distortion and, thus, to maintain the tubular structure of the esophagus during regeneration, the majority of studies have used extracellular matrices (ECMs) with removable stents (8). The native ECMs are derived from human or animal tissues after removal of the cellular and immunogenic components (2, 8). They maintain a highly dynamic structural network that promotes native tissue stem cell migration and maturation into site-specific phenotypic cells (8). ECMs can facilitate this process by releasing bioactive molecules, peptides, and ligands that provide signals to local and migrant stem cells to induce the de novo structural and functional regeneration of tissue (Figure 3). In 2000, Badylak et al. showed successful re-epithelialization with full esophageal wall regeneration at day 50 after applying xenogeneic ECM patches to full-thickness defects in dogs (8).
Figure 3 Schematic explanation of bioscaffold tissue engineering. Bioscaffold tissue provides a highly dynamic structural network that promotes native tissue stem cell migration and maturation into site-specific phenotypic cells. It facilitates this process by releasing bioactive molecules, peptides, and ligands that provide signals to local and migrant stem cells to induce the de novo structural and functional regeneration of tissue (8). Created with BioRender.com.
By applying similar principles, in 2014 Nieponice et al. conducted the first human study by using porcine urinary bladder ECMs as reconstructive patches to repair large, full-thickness defects in four patients (Table 3) (2). The ECM patches were sutured to the edges of the esophageal full-thickness defects. Clinical outcomes were favorable in all cases, with complete mucosal remodeling and normal epithelialization observed at 2 months. The immediate foreseeable application of this bioscaffold technology is to repair the large perforations, leaks, and fistulas that are not manageable with currently available endotherapy. More clinical trials, however, will be required to validate the results from this first human study.
Long-segment circumferential defects can occur in congenital esophageal atresia or post esophagectomy for either benign or malignant conditions. Significant morbidities and poor quality of life are associated with these types of defects. Animal studies using acellular allogeneic or xenogenic ECM alone failed to regenerate a circumferential, long-segment esophagus (9). This suggests that there are limitations to the size of defect that may be replaced using the ECM methodology. Instead, animal studies (Table 2) using an ECM cellularized with pluripotent cells either in a bioreactor or in vivo successfully achieved de novo organogenesis of the esophagus (9, 10). In 2017, Catry et al. carried out a 3 cm circumferential replacement of the abdominal esophagus in 10 mini pigs using an MSC-seeded matrix and compared its efficacy with that of a matrix alone, carried out in a control group (9). The graft area was covered with an esophageal removable stent. On day 45, all MSC group specimens exhibited mature squamous epithelia covering the entire graft area, whereas none of the control group specimens achieved this until day 95. In a more recent study published in 2022, Sundaram et al. successfully regrew the full-layered esophagus in a porcine model using the Cellspan Esophageal Implant™ (CEI), a polyurethane tubular mesh cell delivery device (Cellframe™ Technology) seeded with autologous ADMSCs (12). Furthermore, the extended 1-year follow-up period revealed the successful restoration of oral nutrition, normal animal growth, and the overall safety of this therapeutic approach.
By applying this principle, in 2016 Dua et al. reported the first human case of de novo regeneration of the esophagus in a 24-year-old patient (Table 3) (4). Through a large paraspinal abscess, a 5 cm full-thickness circumferential defect was formed in the upper esophagus, resulting in direct communication between the pharynx and mediastinum. Three self-expanding metal stents (SEMSs) were first placed endoscopically to maintain the tubular configuration of the esophagus. A commercially available ECM (AlloDerm™) was then placed around the stent and sprayed with autologous platelet-rich plasma. The platelet-derived growth factor (PDGF) from the autologous platelet-rich plasma assisted the migration of endogenous pluripotent cells in the patient’s thoracic cavity, which served as an in vivo bioreactor. The SEMSs were removed 3.5 years after their placement, and it was observed that the esophagus had regenerated to full thickness and that it contained a stratified squamous epithelium, a normal five-layer wall, and peristaltic motility with bolus transit. In a more recent case report published in 2021, Aho et al. successfully implanted the Cellspan Esophageal Implant™ (CEI) in a 75-year-old patient to replace a 4 cm full circumferential segment of esophagus that had been invaded by a tumor (15). Complete luminal epithelialization and partial esophageal tissue regeneration were observed after 7.5 months.
These results, from both case reports, hold significant promise. A biocompatible interposition graft that aids in esophageal regeneration holds significant clinical potential, particularly in the context of short-circumferential segment resection (15). Further clinical trials are warranted to validate these findings and potentially simplify the technique, which currently relies on practitioner(s) having specialized expertise (4, 15).
The principles used in stomach tissue engineering closely resemble those utilized in esophageal tissue engineering. Some promising results have been obtained in animal models, but, as of now, there have been no published human studies in the field of stomach tissue engineering.
Partial-thickness defects of the stomach can occur in peptic ulcer disease or as a result of ESD for the removal of superficial gastric Neoplasms. Both cell therapy and bioscaffold techniques have achieved success in promoting the healing of this type of defect in animal models (Table 4).
In 2008, Askarov et al. conducted a study in which precultured multipotent mesenchymal stromal cells (MMSCs) from autologous bone narrow were injected into the site of chronic gastric ulcers (5 mm–7 mm in diameter) in rats (16). This treatment resulted in a significant reduction in the ulcer defect area and accelerated epithelialization on days 10 and 20 compared with what was observed in the control group. On day 30, full epithelialization of the gastric mucosa was observed in the MMSC transplantation group. In 2008, Hayashi et al. and Nishida et al. observed similar results in their experiment on the topical transplantation of MMSCs to gastric ulcers in mice (17, 18). It was suggested that MMSCs delivered to the zone of the ulcer defect induce regeneration by releasing regulatory peptides, immunocytokines, and growth factors, in turn promoting neoangiogenesis and ulcer healing (17).
In a more recent study published in 2019, Xia et al. endoscopically applied adipose-derived mesenchymal stem cells (ADMSCs) to non-steroidal anti-inflammatory drug (NSAID)-related gastric ulcers in a porcine model (19). This approach resulted in the model exhibiting reduced inflammatory infiltration, enhanced re-epithelization, and neovascularization at both day 7 and day 21 compared with what was observed in the control group (which was injected with saline). However, it was observed that only a small proportion of engrafted ADMSCs displayed myofibroblast and epithelial cell characteristics in vivo, indicating that the healing process of the ulcer may be less dependent on stem cell transdifferentiation. Subsequent experiments involving the submucosal injection of MSC-derived secretome demonstrated a therapeutic effectiveness that was on par with stem cell transplantation. This indicates that the paracrine action of stem cells plays a central role in the healing process.
As an alternative to cell therapy, in 2021 Zhao et al. developed a polyurethane/small intestinal submucosa (PU/SIS) hydrogel treatment with high levels of biocompatibility, bioadhesion, and pH sensitivity (20). This modified ECM was delivered endoscopically to the ESD-induced ulcer site (3 cm in diameter) in a canine model. The PU/SIS hydrogel treatment was shown to significantly accelerate healing at the early stages compared with that observed in the control group (which received proton pump inhibitors). At 4 weeks, the ulcer was completely healed in the PU/SIS group, but complete healing took 7 weeks in the control group. It was suggested that this modified ECM provided a protective microenvironment that released growth factors, in turn promoting ulcer healing. However, more animal studies are recommended to validate the results obtained in these studies before these technologies are extrapolated to humans.
For full-thickness patch defects of the stomach, both acellular and cellular tissue-engineering approaches have been reported in animal studies (Table 4). In terms of acellular approaches, in 2002 Hori et al. established a method for in situ stomach tissue engineering in a canine model by suturing an acellular collagen scaffold graft to a 4 cm × 4 cm anterior stomach wall defect (21). A silicone sheet was used as a patch on the luminal side to protect the scaffold from degradation by digestive juice. At 16 weeks, the stomach wall had regenerated, and a gastric mucosa covered the entire lesion. However, the silicone sheet presented technical difficulties for suturing and needed to be removed endoscopically after healing, and therefore has limited clinical usability. To avoid the need for endoscopic removal, in 2009 Araki et al. experimented with single-sheet collagen scaffolds reinforced with a biodegradable copolymer (22). This new scaffold was sutured to repair a 5 cm circular full-thickness defect on the anterior stomach in dog models. At 16 weeks, the serosal side of the implanted scaffold was fully covered by omentum, with mucosa regeneration observed on the luminal side. However, regeneration of the smooth muscle layer did not occur, and early shrinkage of the implanted scaffold was also observed.
Regarding cellular approaches, in 2009 Sirbu-Boeti et al. seeded a collagen-agarose scaffold with mesenchymal stem cells (MSCs) obtained from bone marrow (23). The MSC-enriched patches were sutured to anterior stomach wall defects (5 mm–7 mm in diameter) in rats. The full regeneration of all four layers of the stomach wall was observed at 48 days. In 2015, Nakatsu et al. demonstrated similar results by grafting MSC-seeded small intestinal submucosa to a 1 cm whole-layer stomach defect in a rat model (24). This combination of bioscaffold and cell therapy enabled the effective regeneration of the stomach wall both histologically and functionally.
To repair whole-layer circumferential defects of the stomach, animal studies (Table 4) successfully generated tissue-engineered stomach by implanting bioscaffolds loaded with pluripotent cells or organoids into the animals’ peritoneal cavities (Figure 4) (25). The stomach organoids were derived from gastric stem cells and contained a mixture of epithelia and mesenchymes. The mesenchymal–epithelial interactions in the organoids are crucial to the differentiation and morphogenesis of the engineered tissue (25).
Figure 4 Schematic explanation of organoids-on-polymer tissue engineering. The harvested tissue from donor sites is processed in vitro to form organoids that contain a mixture of epithelium and mesenchyme. The organoids are then seeded onto the scaffold and implanted in the omentum of the animal. Through in vivo organogenesis, tissue-engineered GI segment is then harvested and inserted to replace the defective segment (25, 31). Created with BioRender.com.
In 2004, Maemura et al. seeded stomach epithelium organoids to the biodegradable polymer tube and wrapped them in the omenta of adult Lewis rats (25). The organoid polymer constructs formed cyst-like tissue-engineered stomachs in 3–6 weeks. In rats that underwent gastrectomies, the autologous tissue-engineered stomach was then harvested and anastomosed to the esophagus and small intestine. The omentum attached to the tissue-engineered stomach was retained to maintain the blood supply to the stomach. At 24 weeks, a well-developed neostomach was observed, with a continuous mucosa lining the lumen and stratified, smooth muscle-like layers. Neither stenosis nor obstruction was observed at the anastomosis site. These findings suggest that the tissue-engineered stomach had functionally normal secretion and motility, similar to those of a native stomach. Nevertheless, this methodology appears to have limited applicability in large-animal models or in humans due to the tissue-engineered stomach needing to be formed in the abdominal cavity of the recipient. Furthermore, long-term follow-up studies are required to demonstrate the functionality and safety of tissue-engineered stomachs.
The intestinal epithelium layer is often damaged by various gastrointestinal diseases such as inflammatory bowel diseases (IBD). Animal studies seeking to address this issue have used both ECM bioscaffold and intestinal organoids to repair intestines with significant mucosal damage (Table 5).
In 2017 Keane et al. prepared an ECM hydrogel (ECMH) from a porcine small intestine submucosa and applied it to rats with ulcerative colitis via enema (26). The ECMH adhered to the colonic tissue and significantly reduced the clinical and histologic severity of the disease in the hydrogel-treated rats at days 7 and 14 compared with that in the control rats. This demonstrated the effect of ECMH treatment in restoring colonic epithelial barrier function.
On the other hand, in 2012 Yui et al. used single stem cells from a mouse colon to form colon organoids in vitro and transplanted them into superficially damaged mouse colon (27). The transplanted organoids colonized injured mucosa and formed functional donor-derived epithelium 4 weeks post transplantation. After long-term follow-up of more than 6 months, the graft remained stable and showed evidence of mucosal proliferation and renewal. These findings were supported by a study from Watanabe et al. in 2022, which showed similar results (28). The therapeutic application of ECMH or organoid culture to generate new intestinal tissue could provide a novel mechanism to restore barrier function in IBD, especially improving the prognosis of those with refractory ulcerative colitis.
Different bioscaffolds or cell sources have been used to repair full-thickness intestinal defects in various animal models (Table 5). In 2001 Chen et al. repaired a 7 cm × 3 cm full-thickness defect on the small intestinal wall with a small intestinal submucosa patch in a canine model (29). A histologic evaluation showed the presence of mucosa, submucosa, smooth muscle, and serosa in the newly formed bowel wall. However, the architecture of the submucosa and smooth muscle layers were not well organized. To improve this, in 2006 Nakase et al. used collagen scaffolds seeded with autologous smooth muscle cells (SMC) before implanting them to full-thickness ileal defects in dogs (30). At 12 weeks, a well-developed epithelial layer with villous structures and an organized smooth muscle layer was observed.
The ultimate goal in intestinal tissue engineering, however, is to generate a whole-layer circumferential intestinal graft for transplantation, as it may offer curative therapy for patients with short bowel syndrome. In 2004, Grikscheit et al. created tissue-engineered small intestines (TESI) through the transplantation of organoid units on a polymer scaffold before implanting it into the omentum of a rat (Figure 4) (31). The harvested TESI was then implanted to five rats that had underwent massive small bowel resection. The immunohistochemical studies of the neo-intestine revealed intact epithelial, muscular, vascular, and neural components. The postoperative weights and B12 absorption abilities of animals receiving the TESI were significantly improved compared with those animals that had the bowel resection alone, indicating that the TESI functioned in vivo to meet basic physiologic needs. However, further investigation is required to study the potential immunogenicity of the TESI.
To bring this organoid-on-scaffold approach a step closer to clinical practice, in 2020 Meran et al. generated the first patient-derived TESI with organoids and bioscaffolds obtained from children with intestinal failure (32). Patient-derived organoids were seeded into the decellurized human intestine matrix before being transferred to a bioreactor system for 11 days. The patient-derived TESI was then transplanted into immunodeficient mice for 1 week. Serial histology analyses showed that the patient-derived TESI retained jejunal epithelial identity, but that it did not fully recapitulate a mature crypt–villus morphology and was enterocyte-dominant. Future studies are needed to further differentiate patient-derived TESI into fully functional jejunum in vivo.
Perianal fistula is a type of full-thickness defect with a tract between the anorectal canal and the perianal skin. It is one of the most common colorectal diseases, with an incidence of 1–8 per 10,000 individuals, and with up to 25% of cases associated with Crohn’s disease (51). Although most simple perianal fistulas can be cured by surgical operation, the success rate of surgeries on complex and refractory fistulas is low, and such surgeries are also associated with a high rate of complications. These complications, such as fistula recurrence and fecal incontinence due to sphincter and perianal tissue destruction, can significantly impact on patients’ quality of life and result in high healthcare costs (51). Recently, tissue engineering techniques such as the local injection of mesenchymal stem cells (MSCs) have shown great promise in treating complex perianal fistulas and have a minimal risk of fecal incontinence. A number of human studies have now demonstrated the safety and efficacy of this novel therapy (Table 6) (33–49).
In 2016, Panes et al. conducted a well-known phase 3 randomized, double-blind controlled trial in Europe and Israel (ADMIRE-CD study) to assess the safety and efficacy of darvadstrocel Cx601 (a commercially available allogeneic adipose-derived stem cells preparation) for treating complex perianal fistulas in patients with Crohn’s disease who had not responded to conventional or biological treatments (35). Darvadstrocel Cx601 was administered by direct injection after curettage and primary closure of the fistula tract. At week 24, 50% of patients receiving darvadstrocel Cx601 demonstrated the clinically confirmed closure of all treated external openings and the absence of collections, and this effect was observed to have been maintained at the week 52 follow-up. Similar results were also observed in 2023 by Furukawa et al. in a phase 3 study on the use of darvadstrocel Cx601 in a Japanese patient population with refractory perianal fistulizing Crohn’s disease (47).
In 2020, Barnhoorn et al. evaluated the long-term safety and efficacy of allogeneic bone marrow-derived mesenchymal stromal cell (bmMSC) therapy in a patient with Crohn’s disease-associated perianal fistulas (40). After 4 years of follow-up, they observed the sustained high rate of fistula closure and noted a significant improvement in the quality of life reported by the bmMSC-treated patients. In addition, none of the patients who underwent bmMSC therapy developed anti-HLA antibodies within either the initial 24-week period or the extended 4-year time frame. In contrast, Panes et al. observed that 34% (18 out of 53) of patients who received Cx601 therapy and who were initially negative for anti-HLA class I antibodies developed these antibodies following MSC treatment (35). This difference in anti-HLA antibody formation may be attributed to the origin of the MSC product. Although the clinical significance of the presence of anti-HLA antibodies remains unclear, it is important to note that the current studies have not raised any safety or efficacy concerns (35, 40). Several studies further support the efficacy and safety of local MSCs therapy in refractory Crohn’s disease fistula (see Table 6) (33, 37, 39, 42, 44, 45, 48).
It is believed that MSCs exert immunosuppressive effects and modulate the functions of different immune cells, resulting in their exerting disease-modifying and healing effects in Crohn’s disease (35). Moreover, the absence of histocompatibility complex class 2 and the limited expression of human leukocyte antigen (HLA) class 1 in human MSCs have established their suitability for safe allogenic transplantation (35, 40, 47). This quality renders commercially available MSC preparations (such as darvadstrocel) a viable and pragmatic choice when transitioning the technique from the laboratory to real-world applications.
Satisfactory therapeutic outcomes were similarly evident with local MSC transplantation in the treatment of complex perianal fistula in non-Crohn’s-disease cases. In 2021, Ascanelli et al. administered autologous centrifuged adipose tissue containing progenitor cells to 58 patients with complex cryptoglandular anal fistulas (41). Their study revealed that 63.8% of patients who received this stem cell therapy achieved clinical healing within 4 weeks, and that 86.2% had achieved complete healing at the 6-month mark. In addition, the injection of adipose tissue-derived stem cells (ADSCs) significantly reduced postoperative anal pain and allowed an early return to daily activities for the patients. Similarly, in 2020 Garcia-Arranz et al. also observed long-term and sustained fistula healing following ADSCs therapy for the treatment of cryptoglandular fistula, with the healing rate maintained at 50% in the treatment group (38). Several other studies have provided additional evidence of the efficacy and safety of MSCs transplantation in non-Crohn’s-disease complex perianal fistula (Table 6) (33, 34, 36, 43, 46).
To further increase the remission rate, another novel approach has been devised, which entails the synergistic combination of MSCs with bioscaffolds to enhance cell retention at the site of injury. In 2017, Dietz et al. applied autologous MSC-loaded fistula plugs (MSC-MATRIX) to patients with refractory Crohn’s disease fistulas (48). At 6 months, 10 out of 12 patients (83%) exhibited complete clinical healing and radiographic response. In 2019, Dozois et al. conducted an additional evaluation of the safety and efficacy of MSC-MATRIX in patients with trans-sphincteric cryptoglandular fistulas (49). At 6 months, 11 out of 15 patients (73%) exhibited radiographic improvement, consisting of three patients who achieved complete clinical healing and eight who showed partial healing. These data collectively demonstrate the therapeutic potential for MSC-MATRIX in treating refractory diseases. However, both studies are limited by their small patient cohorts and short follow-up durations (48, 49). To rigorously assess bioscaffolds loaded with MSCs, it is imperative to conduct a randomized, controlled phase II trial. Furthermore, there are currently no standardized guidelines regarding MSCs source, cell types, or the optimal number of administrations, making cross-study comparison difficult to carry out. Further research is needed to elucidate whether or not these factors can influence treatment efficacy.
Major conceptual advances have been made toward GI tissue engineering, especially in animal models. The extrapolation of technologies used in animal studies to humans is still an experimental process that has been attempted only in the fields of esophageal, rectal, and anal tissue engineering. Although the initial results represent a promising start, several challenges must be overcome prior to the standard clinical application of these techniques. Firstly, the bioengineered tissues commonly lack proper connections to the vascular, nervous, and lymphatic systems in the rest of the GIT, posing a substantial challenge to the full integration of the grafts. In addition, scaling up the manufacture of grafts of different sizes remains an obstacle toward clinical translation. Furthermore, there still remain knowledge gaps in understanding the expansion limit and the in vivo stability of organoids and different stem cells, which poses potential long-term risks to recipient safety. Additional work, including prospective studies and long-term follow-up in large-animal models and in humans, is required to fully evaluate the in vivo safety and physiologic functions of the grafts.
Repairing different types of defects in the gastrointestinal tract using tissue-engineering technology have been extensively studied in animal models. Technologies such as mesenchymal stem cells, bio-scaffolds, organoids, and cell sheets are the most promising and closer to clinical translation. Collaboration between GI surgery and regenerative medicine is expected to bring novel therapeutic modalities in the future. Although major conceptual advances have been made, there remain several challenges to overcome, such as the upscaling of the manufacturing process, cost, regulation, and in vivo safety concerns. Future large-animal studies and clinical trials will be instrumental in furthering development in this field.
YL: Data curation, Investigation, Methodology, Writing - original draft, Writing - review and editing. LC: Conceptualization, Supervision, Writing - review and editing. MR: Conceptualization, Supervision, Writing - review and editing.
The author(s) declare that no financial support was received for the research, authorship, and/or publication of this article.
The authors declare that the research was conducted in the absence of any commercial or financial relationships that could be construed as a potential conflict of interest.
All claims expressed in this article are solely those of the authors and do not necessarily represent those of their affiliated organizations, or those of the publisher, the editors and the reviewers. Any product that may be evaluated in this article, or claim that may be made by its manufacturer, is not guaranteed or endorsed by the publisher.
1. Ohki T, Yamato M, Murakami D, Takagi R, Yang J, Namiki H, et al. Treatment of oesophageal ulcerations using endoscopic transplantation of tissue-engineered autologous oral mucosal epithelial cell sheets in a canine model. Gut (2006) 55:1704–10. doi: 10.1136/gut.2005.088518
2. Nieponice A, Ciotola FF, Nachman F, Jobe BA, Hoppo T, Londono R, et al. Patch esophagoplasty: esophageal reconstruction using biologic scaffolds. Ann Thorac Surg (2014) 97:283–8. doi: 10.1016/j.athoracsur.2013.08.011
3. Owen HA, Buchanan GN, Schizas A, Cohen R, Williams AB. Quality of life with anal fistula. Ann R Coll Surg Engl (2016) 98(5):334–8. doi: 10.1308/rcsann.2016.0136
4. Dua KS, Hogan WJ, Aadam AA, Gasparri M. In-vivo oesophageal regeneration in a human being by use of a non-biological scaffold and extracellular matrix. Lancet (2016) 388:55–61. doi: 10.1016/S0140-6736(15)01036-3
5. Honda M, Nakamura T, Hori Y, Shionoya Y, Nakada A, Sato T, et al. Process of healing of mucosal defects in the esophagus after endoscopic mucosal resection: histological evaluation in a dog model. Endoscopy (2010) 42:1092–5. doi: 10.1055/s-0030-1255741
6. Kanai N, Yamato M, Ohki T, Yamamoto M, Okano T. Fabricated autologous epidermal cell sheets for the prevention of esophageal stricture after circumferential ESD in a porcine model. Gastrointest Endosc (2012) 76:873–81. doi: 10.1016/j.gie.2012.06.017
7. Nieponice A, McGrath K, Qureshi I, Beckman EJ, Luketich JD, Gilbert TW, et al. An extracellular matrix scaffold for esophageal stricture prevention after circumferential EMR. Gastrointest Endosc (2009) 69:289–96. doi: 10.1016/j.gie.2008.04.022
8. Badylak S, Meurling S, Chen M, Spievack A, Simmons-Byrd A. Resorbable bioscaffold for esophageal repair in a dog model. J Pediatr Surg (2000) 35:1097–103. doi: 10.1053/jpsu.2000.7834
9. Catry J, Luong-Nguyen M, Arakelian L, Poghosyan T, Bruneval P, Domet T, et al. Circumferential esophageal replacement by a tissue-engineered substitute using mesenchymal stem cells: an experimental study in mini pigs. Cell Transplant (2017) 26:1831–9. doi: 10.1177/0963689717741498
10. La Francesca S, Aho JM, Barron MR, Blanco EW, Soliman S, Kalenjian L, et al. Long-term regeneration and remodeling of the pig esophagus after circumferential resection using a retrievable synthetic scaffold carrying autologous cells. Sci Rep (2018) 8:4123. doi: 10.1038/s41598-018-22401-x
11. Levenson G, Berger A, Demma J, Perrod G, Domet T, Arakelian L, et al. Circumferential esophageal replacement by a decellularized esophageal matrix in a porcine model. Surgery (2022) 171(2):384–92. doi: 10.1016/j.surg.2021.07.009
12. Sundaram S, Jensen T, Roffidal T, Paquin K, Wanczyk H, Cockman MD, et al. Esophageal regeneration following surgical implantation of a tissue engineered esophageal implant in a pediatric model. NPJ Regener Med (2022) 7(1):1. doi: 10.1038/s41536-021-00200-9
13. Ohki T, Yamato M, Ota M, Takagi R, Murakami D, Kondo M, et al. Prevention of esophageal stricture after endoscopic submucosal dissection using tissue-engineered cell sheets. Gastroenterology (2012) 143:582–8.e2. doi: 10.1053/j.gastro.2012.04.050
14. Yamaguchi N, Isomoto H, Kobayashi S, Kanai N, Kanetaka K, Sakai Y, et al. Oral epithelial cell sheets engraftment for esophageal strictures after endoscopic submucosal dissection of squamous cell carcinoma and airplane transportation. Sci Rep (2017) 7:17460. doi: 10.1038/s41598-017-17663-w
15. Aho JM, La Francesca S, Olson SD, Triolo F, Bouchard J, Mondano L, et al. First-in-human segmental esophageal reconstruction using a bioengineered mesenchymal stromal cell-seeded implant. JTO Clin Res Rep (2021) 2(9):100216. doi: 10.1016/j.jtocrr.2021.100216
16. Askarov MB, Onischenko NA. Multipotent mesenchymal stromal cells of autologous bone marrow stimulate neoangiogenesis, restore microcirculation, and promote healing of indolent ulcers of the stomach. Bull Exp Biol Med (2008) 146(4):512–6. doi: 10.1007/s10517-009-0324-6
17. Nishida T, Tsuji S, Tsujii M, Ishii S, Yoshio T, Shinzaki S, et al. Cultured bone marrow cell local implantation accelerates healing of ulcers in mice. J Gastroenterol (2008) 43(2):124–35. doi: 10.1007/s00535-007-2137-6
18. Hayashi Y, Tsuji S, Tsujii M, Nishida T, Ishii S, Iijima H, et al. Topical transplantation of mesenchymal stem cells accelerates gastric ulcer healing in rats. Am J Physiol Gastrointest Liver Physiol (2008) 294(3):G778–86. doi: 10.1152/ajpgi.00468.2007
19. Xia X, Chan KF, Wong GTY, Wang P, Liu L, Yeung BPM, et al. Mesenchymal stem cells promote healing of nonsteroidal anti-inflammatory drug-related peptic ulcer through paracrine actions in pigs. Sci Transl Med (2019) 11(516). doi: 10.1126/scitranslmed.aat7455
20. Zhao LM, Gong M, Wang R, Yuan QJ, Zhang Y, Pi JK, et al. Accelerating ESD-induced gastric ulcer healing using a pH-responsive polyurethane/small intestinal submucosa hydrogel delivered by endoscopic catheter. Regener Biomater (2021) 8(1):rbaa056. doi: 10.1093/rb/rbaa056
21. Hori Y, Nakamura T, Kimura D, Kaino K, Kurokawa Y, Satomi S, et al. Functional analysis of the tissue-engineered stomach wall. Artif Organs (2002) 26(10):868–72. doi: 10.1046/j.1525-1594.2002.07006.x
22. Araki M, Tao H, Sato T, Nakajima N, Hyon SH, Nagayasu T, et al. Development of a new tissue-engineered sheet for reconstruction of the stomach. Artif Organs (2009) 33(10):818–26. doi: 10.1111/j.1525-1594.2009.00808.x
23. Sirbu-Boeti MP, Chivu M, Paslaru LL, Efrimescu C, Herlea V, Pecheanu C, et al. Transplantation of mesenchymal stem cells cultured on biomatrix support induces repairing of digestive tract defects, in animal model. Chirurgia (Bucur) (2009) 104(1):55–65.
24. Nakatsu H, Ueno T, Oga A, Nakao M, Nishimura T, Kobayashi S, et al. Influence of mesenchymal stem cells on stomach tissue engineering using small intestinal submucosa. J Tissue Eng Regener Med (2015) 9(3):296–304. doi: 10.1002/term.1794
25. Maemura T, Ogawa K, Shin M, Mochizuki H, Vacanti JP. Assessment of tissue-engineered stomach derived from isolated epithelium organoid units. Transplant Proc (2004) 36(5):1595–9. doi: 10.1016/j.transproceed.2004.05.020
26. Keane TJ, Dziki J, Sobieski E, Smoulder A, Castleton A, Turner N, et al. Restoring mucosal barrier function and modifying macrophage phenotype with an extracellular matrix hydrogel: potential therapy for ulcerative colitis. J Crohns Colitis (2017) 11(3):360–8. doi: 10.1093/ecco-jcc/jjw149
27. Yui S, Nakamura T, Sato T, Nemoto Y, Mizutani T, Zheng X, et al. Functional engraftment of colon epithelium expanded in vitro from a single adult Lgr5+ stem cell. Nat Med (2012) 18(4):618–23. doi: 10.1038/nm.2695
28. Watanabe S, Kobayashi S, Ogasawara N, Okamoto R, Nakamura T, Watanabe M, et al. Transplantation of intestinal organoids into a mouse model of colitis. Nat Protoc (2022) 17(3):649–71. doi: 10.1038/s41596-021-00658-3
29. Chen MK, Badylak SF. Small bowel tissue engineering using small intestinal submucosa as a scaffold. J Surg Res (2001) 99(2):352–8. doi: 10.1006/jsre.2001.6199
30. Nakase Y, Hagiwara A, Nakamura T, Kin S, Nakashima S, Yoshikawa T, et al. Tissue engineering of small intestinal tissue using collagen sponge scaffolds seeded with smooth muscle cells. Tissue Eng (2006) 12(2):403–12. doi: 10.1089/ten.2006.12.403
31. Grikscheit TC, Siddique A, Ochoa ER, Srinivasan A, Alsberg E, Hodin RA, et al. Tissue-engineered small intestine improves recovery after massive small bowel resection. Ann Surg (2004) 240(5):748–54. doi: 10.1097/01.sla.0000143246.07277.73
32. Meran L, Massie I, Campinoti S, Weston AE, Gaifulina R, Tullie L, et al. Engineering transplantable jejunal mucosal grafts using patient-derived organoids from children with intestinal failure. Nat Med (2020) 26(10):1593–601. doi: 10.1038/s41591-020-1024-z
33. Garcia-Olmo D, Herreros D, Pascual I, Pascual JA, Del-Valle E, Zorrilla J, et al. Expanded adipose-derived stem cells for the treatment of complex perianal fistula: a phase II clinical trial. Dis Colon Rectum (2009) 52(1):79–86. doi: 10.1007/DCR.0b013e3181973487
34. Herreros MD, Garcia-Arranz M, Guadalajara H, De-La-Quintana P, Garcia-Olmo D. Autologous expanded adipose-derived stem cells for the treatment of complex cryptoglandular perianal fistulas: a phase III randomized clinical trial (FATT 1: fistula Advanced Therapy Trial 1) and long-term evaluation. Dis Colon Rectum (2012) 55(7):762–72. doi: 10.1097/DCR.0b013e318255364a
35. Panés J, García-Olmo D, Van Assche G, Colombel JF, Reinisch W, Baumgart DC, et al. Expanded allogeneic adipose-derived mesenchymal stem cells (Cx601) for complex perianal fistulas in Crohn's disease: a phase 3 randomised, double-blind controlled trial. Lancet (2016) 388(10051):1281–90. doi: 10.1016/S0140-6736(16)31203-X
36. Topal U, Eray IC, Rencüzoğulları A, Yalav O, Alabaz Ö. Short-term results of adipose-derived stem cell therapy for the treatment of complex perianal fistula A single center experience. Ann Ital Chir (2019) 90:583–9.
37. Dige A, Hougaard HT, Agnholt J, Pedersen BG, Tencerova M, Kassem M, et al. Efficacy of injection of freshly collected autologous adipose tissue into perianal fistulas in patients with Crohn's disease. Gastroenterology (2019) 156(8):2208–16.e1. doi: 10.1053/j.gastro.2019.02.005
38. Garcia-Arranz M, Garcia-Olmo D, Herreros MD, Gracia-Solana J, Guadalajara H, de la Portilla F, et al. Autologous adipose-derived stem cells for the treatment of complex cryptoglandular perianal fistula: a randomized clinical trial with long-term follow-up. Stem Cells Transl Med (2020) 9(3):295–301. doi: 10.1002/sctm.19-0271
39. Laureti S, Gionchetti P, Cappelli A, Vittori L, Contedini F, Rizzello F, et al. Refractory complex crohn's perianal fistulas: a role for autologous microfragmented adipose tissue injection. Inflammation Bowel Dis (2020) 26(2):321–30. doi: 10.1093/ibd/izz051
40. Barnhoorn MC, Wasser M, Roelofs H, Maljaars PWJ, Molendijk I, Bonsing BA, et al. Long-term evaluation of allogeneic bone marrow-derived mesenchymal stromal cell therapy for Crohn's disease perianal fistulas. J Crohns Colitis (2020) 14(1):64–70. doi: 10.1093/ecco-jcc/jjz116
41. Ascanelli S, Zamboni P, Campioni D, Grazia Sibilla M, Chimisso L, Zollino I, et al. Efficacy and safety of treatment of complex idiopathic fistula-in-ano using autologous centrifuged adipose tissue containing progenitor cells: a randomized controlled trial. Dis Colon Rectum (2021) 64(10):1276–85. doi: 10.1097/DCR.0000000000001924
42. Cabalzar-Wondberg D, Turina M, Biedermann L, Rogler G, Schreiner P. Allogeneic expanded adipose-derived mesenchymal stem cell therapy for perianal fistulas in Crohn's disease: a case series. Colorectal Dis (2021) 23(6):1444–50. doi: 10.1111/codi.15587
43. Maciel Gutiérrez VM, Gutiérrez Guillen SG, Centeno Flores MW, Valenzuela Pérez JA, Abarca Rendón FM, Hernández García FS, et al. Safety of allogeneic adipose tissue-derived mesenchymal stem cells for the treatment of complex perianal fistulas not associated with Crohn's disease: a phase I clinical trial. Dis Colon Rectum (2021) 64(3):328–34. doi: 10.1097/DCR.0000000000001863
44. Guillo L, Grimaud F, Houser F, Prost C, Jouve E, Philandrianos C, et al. Three-year outcome of local injection of autologous stromal vascular fraction cells and microfat in refractory perianal fistulas of Crohn's disease. Stem Cell Res Ther (2022) 13(1):67. doi: 10.1186/s13287-022-02738-x
45. Lightner AL, Reese J, Ream J, Nachand D, Jia X, Dadgar N, et al. A phase IB/IIA study of ex vivo expanded allogeneic bone marrow-derived mesenchymal stem cells for the treatment of perianal fistulizing Crohn's disease. Dis Colon Rectum (2023) 66(10):1359–72. doi: 10.1097/DCR.0000000000002567
46. Pak H, Hadizadeh A, Heirani-Tabasi A, Soleimani M, Asbagh RA, Fazeli MS, et al. Safety and efficacy of injection of human placenta mesenchymal stem cells derived exosomes for treatment of complex perianal fistula in non-Crohn's cases: Clinical trial phase I. J Gastroenterol Hepatol (2023) 38(4):539–47. doi: 10.1111/jgh.16110
47. Furukawa S, Mizushima T, Nakaya R, Shibata M, Yamaguchi T, Watanabe K, et al. Darvadstrocel for complex perianal fistulas in Japanese adults with Crohn's disease: A phase 3 study. J Crohns Colitis (2023) 17(3):369–78. doi: 10.1093/ecco-jcc/jjac144
48. Dietz AB, Dozois EJ, Fletcher JG, Butler GW, Radel D, Lightner AL, et al. Autologous mesenchymal stem cells, applied in a bioabsorbable matrix, for treatment of perianal fistulas in patients with Crohn's disease. Gastroenterology (2017) 153(1):59–62.e2. doi: 10.1053/j.gastro.2017.04.001
49. Dozois EJ, Lightner AL, Mathis KL, Chua HK, Kelley SR, Fletcher JG, et al. Early results of a phase I trial using an adipose-derived mesenchymal stem cell-coated fistula plug for the treatment of transsphincteric cryptoglandular fistulas. Dis Colon Rectum (2019) 62(5):615–22. doi: 10.1097/DCR.0000000000001333
50. Chung A, Bourke MJ, Hourigan LF, Lim G, Moss A, Williams SJ, et al. Complete Barrett's excision by stepwise endoscopic resection in short-segment disease: long term outcomes and predictors of stricture. Endoscopy (2011) 43(12):1025–32. doi: 10.1055/s-0030-1257049
51. Jimenez M, Mandava N. Anorectal Fistula. In: StatPearls. Treasure Island (FL: StatPearls Publishing (2023). Available at: https://www.ncbi.nlm.nih.gov/books/NBK560657/.
Keywords: tissue engineering, regenerative medicine, gastrointestinal tract, stem cells, organoids, scaffolds
Citation: Liu Y, Chong L and Read M (2023) Current status and clinical applications of tissue engineering of the gastrointestinal tract: a systematized narrative review. Front. Gastroenterol. 2:1277094. doi: 10.3389/fgstr.2023.1277094
Received: 14 August 2023; Accepted: 02 October 2023;
Published: 07 November 2023.
Edited by:
Endrit Shahini, National Institute of Gastroenterology S. de Bellis Research Hospital (IRCCS), ItalyReviewed by:
Federica Rubbino, Humanitas Research Hospital, ItalyCopyright © 2023 Liu, Chong and Read. This is an open-access article distributed under the terms of the Creative Commons Attribution License (CC BY). The use, distribution or reproduction in other forums is permitted, provided the original author(s) and the copyright owner(s) are credited and that the original publication in this journal is cited, in accordance with accepted academic practice. No use, distribution or reproduction is permitted which does not comply with these terms.
*Correspondence: Yilin Liu, eWlsaW5saXUxQG91dGxvb2suY29t
Disclaimer: All claims expressed in this article are solely those of the authors and do not necessarily represent those of their affiliated organizations, or those of the publisher, the editors and the reviewers. Any product that may be evaluated in this article or claim that may be made by its manufacturer is not guaranteed or endorsed by the publisher.
Research integrity at Frontiers
Learn more about the work of our research integrity team to safeguard the quality of each article we publish.