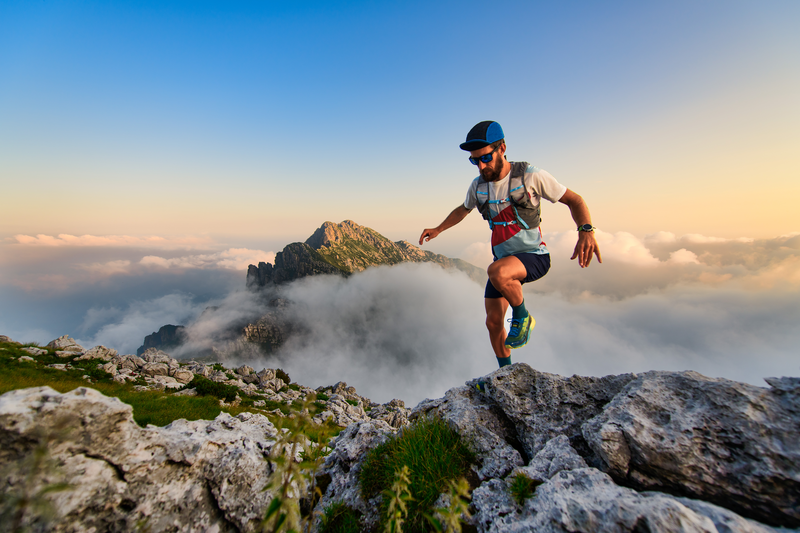
95% of researchers rate our articles as excellent or good
Learn more about the work of our research integrity team to safeguard the quality of each article we publish.
Find out more
ORIGINAL RESEARCH article
Front. Fungal Biol. , 11 June 2024
Sec. Fungal Biotechnology
Volume 5 - 2024 | https://doi.org/10.3389/ffunb.2024.1414349
Mushrooms are widely available around the world and have various nutritional as well as therapeutic values. Many Asian cultures believe that medicinal mushrooms can prolong life and improve vitality. This study aims to characterize the phytochemical and polysaccharide content, mainly β-glucan content, of mycelial biomass and fruiting bodies collected from the Himalayan region, particularly Uttarakhand. Through molecular analysis of the LSU F/R-rDNA fragment sequence and phylogenetic analysis, the strain was identified as Trametes sp. We performed screening of phytochemicals and polysaccharides in mushroom and biomass extracts using high-performance liquid chromatography (HPLC) and a PC-based UV-Vis spectrophotometer. The macrofungal biomass was found to be high in saponin, anthraquinone, total phenolic, flavonoid, and β-glucan content. In biomass extract, we observed a high level of saponin (70.6µg/mL), anthraquinone (14.5µg/mL), total phenolic (12.45 µg/mL), and flavonoid (9.500 µg/mL) content. Furthermore, we examined the contents of alkaloids, tannins, terpenoids, and sterols in the biomass and mushroom extracts; the concentration of these compounds in the ethanol extract tested was minimal. We also looked for antioxidant activity, which is determined in terms of the IC50 value. Trametes sp. mushroom extract exhibits higher DPPH radical scavenging activity (62.9% at 0.5 mg/mL) than biomass extract (59.19% at 0.5 mg/mL). We also analyzed β-glucan in Trametes sp. from both mushroom and biomass extracts. The biomass extract showed a higher β-glucan content of 1.713 mg/mL than the mushroom extract, which is 1.671 mg/mL. Furthermore, β-glucan analysis was confirmed by the Megazyme β-glucan assay kit from both biomass and mushroom extract of Trametes sp. β-glucans have a promising future in cancer treatment as adjuncts to conventional medicines. Producing pure β-glucans for the market is challenging because 90–95% of β glucan sold nowadays is thought to be manipulated or counterfeit. The present study supports the recommendation of Trametes sp. as rich in β-glucan, protein, phytochemicals, and antioxidant activities that help individuals with cancer, diabetes, obesity, etc.
● Obtained a high quantity of Trametes sp. biomass in submerged culture.
● Trametes sp. showed a high β-glucan content in macrofungal biomass extract (42%).
● Tested Trametes sp. biomass and mushroom extracts were high in protein and phytochemicals such as saponins, anthraquinones, total phenolic content (TPC), and total flavonoid content (TFC), and low in fat content.
● The biomass and mushroom extracts also exhibit high antioxidant activity.
In recent years, the higher fungi of the Agaricomycetes class (Basidiomycota division) have been found to have a wide range of natural, structurally diverse bioactive compounds with promising nutritional and therapeutic properties. These compounds have attracted the interest of researchers studying medicine worldwide because of their unique sensory qualities, health benefits, and pharmacological activity due to their synthesizing bioactive metabolites (Stastny et al., 2022). Basidiomycetes produce a variety of bioactive substances, such as lectins, phenolics, homo- and hetero-polysaccharides, flavonoids, terpenoids, sterols, and volatile organic compounds (Lin et al., 2019; Lu et al., 2020; Sevindik et al., 2020; Yadav and Negi, 2021).
The number of fungal species on Earth has been estimated to vary from 500,000 to over 5 million, and currently only 20 kinds of mushrooms have been industrially cultivated, while 35 species have been grown commercially (Hawksworth and Lücking, 2017; Bhambri et al., 2022). As a result, it is more important than ever to separate and recognize the unique bioactive substances that higher fungi produce and to identify the exact chemical that will have the desired medicinal effect. Beyond their nutritional value, mushroom metabolites have been shown to exhibit a plethora of bioactive properties such as immunomodulatory action, prebiotic activity, antibacterial (Selamoglu et al., 2020), antiviral activity (Chuang et al., 2020), anti-aging-properties, anti-hypoglycemic activities (Afiati et al., 2019), neuroprotective (Sevindik et al., 2021), antioxidant (Sevindik et al., 2017, 2018), anti-inflammatory (Bucić-Kojić et al., 2020), anticancer (Lowenthal et al., 2023), antimicrobial (Kothiyal and Singh, 2022), and antiparasitic activity (e.g., against Leishmania sp.) (Leliebre-Lara et al., 2016), anti-oxidant, as well as anti-Toxoplasma gondii properties (Sharma et al., 2023) etc. Mushrooms can also be used as pollution indicators. A study by Akgul et al. (2017) assessed the oxidative stress indices (OSI) in Auricularia auricula and Trametes versicolor that were collected from Köyceğiz-Muğla, Turkey. Their findings revealed high OSI values in both mushroom species. It was determined that eating mushrooms harvested in certain areas may result in health issues for individuals.
The medicinal mushroom that has been the subject of extensive research in the last 10 years is the fungus Trametes, widely distributed throughout Ireland and Britain (Grand and Vernia, 2002; Bhambri et al., 2022). It can be found throughout the European continent, ranging from northern Scandinavia to the Mediterranean region. Asia and North America are also home to this wood-rooting fungus (Habtemariam, 2020). Despite being the most extensively researched and powerful medicinal mushroom, Trametes sp. is not yet standardized in terms of cultivation and extraction of maximum β-glucan production, although it has well-known antiviral (Shi et al., 2022) and anti-cancerous properties.
The species is a member of the family Polyporaceae and phylum Basidiomycota (PUIA et al., 2018), classified in Table 1. Trametes sp. has been used for millennia in traditional medicine and is now included in cancer treatments (Yang et al., 1992; Piotrowski et al., 2015). The most well-studied biological activity carriers are polysaccharopeptides (PSP) derived from Trametes versicolor, which has been renowned as medicine in China and Japan (Cui and Chisti, 2003). PSP, a polysaccharide peptide, and polysaccharide krestin (PSK), a protein-bound polysaccharide, are the two immunologically active components (Habtemariam, 2020). Interestingly, it has been demonstrated that oral administration of PSP and PSK has an anti-cancer effect in vivo (Awadasseid et al., 2017).
Table 1 Classification of mushroom Trametes sp. data obtained from the CABI Bioscience Database of Fungal Names (Kirk, 2021).
Owing to the existence of secondary metabolites, mushrooms possess remarkable biological properties, and as a result, they are used in various industries. Figure 1 lists industries where mushrooms are employed.
Secondary metabolites isolated from Trametes sp., such as phenolic compounds, polysaccharides, terpenoids, and other compounds, are responsible for approximately 130 biological activities, which include immunomodulatory, hepatic protective, antioxidant, antimicrobial, antiviral, and anti-aging properties (Wasser, 2014), among which glycoconjugates are usually considered the main bioactive component (Wang et al., 2019).
Further, C. versicolor polysaccharopeptides have been associated with various physiological effects, such as immunopotentiation by triggering the release of interleukin-6, interferons, immunoglobulin-G, macrophages, and T-lymphocytes; antagonizing the immune-suppressive properties of chemotherapy, radiotherapy, and blood transfusion; antagonizing immune system suppression caused by tumors; and preventing the development of various cancers by inducing the production of superoxide dismutase (Dou et al., 2019). Additionally, the State Food and Drug Administration of China (SFDA) has approved 12 different types of Coriolus versicolor-based medications for clinical usage thus far (Chang et al., 2017). Table 2 lists a few medicines and health products derived from Coriolus versicolor.
Table 2 Some of the Coriolus versicolor-based medicine and health products that the China State Food and Drug Administration (SFDA) has authorized for use in clinical and commercial environments.
However, the majority of research has concentrated on the antioxidant properties of a particular wild mushroom species, while some studies have also looked at the antioxidant, immunostimulatory, and anticancer properties of mushrooms. As a consequence, there is now a large research void in the detailed analysis and comparative discussion of β-glucans across a wide variety of commercially cultivated mushrooms.
Therefore, our study aimed to investigate the composition of mycelium biomass and mushroom (broth) extract obtained by the collection of the medicinal mushroom Trametes sp. in the Himalayan region, especially in Uttarakhand, and their submerged cultivation, which had previously been studied for its various applications in the pharmaceutical industry. The evaluation of the antioxidant activity of biomass and mushroom extracts, together with the screening of the phytochemical and polysaccharide content of Trametes sp. in ethanol extracts using the high-performance liquid chromatography (HPLC) method and a PC-based UV-Vis spectrophotometer, were studied. In addition, we examined the amount of β-glucan in the mycelium that was acquired from submerged cultivation and verified it using the mushroom and yeast β-glucan assay kit (Megazyme Int., Dublin, Ireland).
The macrofungal strain fruiting body was collected from the Neelkantha forest, Rishikesh, Uttarakhand, situated at an altitude of 6,596 meters, latitude 30°43′48′′N, and longitude 79°24′20′′E.
Fruiting bodies were cleaned, and the inner mass of the mushroom was removed with a sterilized knife and cultivated for 14 days at 20°C in a suitable potato dextrose agar (PDA) medium. A pure culture was obtained and sustained after additional tissue culture was established and multiple subcultures were carried out to purify the culture. After generating further sub-cultures from the pure culture medium, the biomass was grown in a liquid medium (potato dextrose broth; PDB) in a 250-mL volumetric flask and incubated for 14 days at 20°C in the dark. This step was done in batches. Following that, biomass was filtered and dried at 50°C for 2 h. Using a pestle and mortar, the dried biomass was ground into a fine powder. The resulting powder was then used and prepared for analysis. The pure culture was stored in a suitable potato dextrose agar medium at 4°C and was subcultured every 30 days onto the fresh medium.
Genomic DNA was extracted from cultured samples by a simple lysis protocol, as described in Karn et al. (2011). In total, 125 ηg of extracted DNA is used for amplification, along with 10 pM of each primer. LSU F/R-rDNA fragment was amplified using the ABI 3130 Genetic Analyzer with the following set of primers: forward primer: 5’-TCCTGAGGGAAACTTCG-3’ and reverse primer: 5’-ACCCGCTGAACTTAAGC-3’. The reaction mixture of PCR contained 10x Taq DNA polymerase Assay Buffer, 2.5 mM each dNTP, 3.2 mM MgCl2, and 3 U/ml Taq DNA Polymerase in a final volume of 30 ml sterile MQ water. The PCR was performed with an initial denaturation at 94°C for 3 min, followed by 30 cycles of 94°C for 1 min, 50°C for 1 min, and 72°C for 2 min, and a final extension of 72°C for 7 min. The amplification product was gel-purified using ABI 3130xl gel sequencing. The PCR product was sequenced bi-directionally. The sequence data were aligned and compared against the available DNA sequences from type strains in GenBank (http://www.ncbi.nlm.nih.gov/) using the BLAST sequence match tool and analyzed to identify the mushroom and its closest neighbors joining by making a phylogenetic tree using the software Phylogenetic Tree Builder (Bruno et al., 2000).
Ethanol (80%, v/v) was used as an extracting solvent for obtaining MBE and BE. The dry biomass (10g) was ground and mixed with 100 ml of 80% ethanol, then left in a laboratory shaker at 25°C and 140 rpm for 24 h. After that, extracts were separated from the mycelium biomass by centrifugation. The process was then carried out once more after the solid had been redissolved in ethanol. Next, Whatman paper No. 4 was used to combine and filter the liquid. In a vacuum evaporator, the filtrate was concentrated, and the thick concentrated extract was collected and used for phytochemical and polysaccharide quantification research.
The total protein content in MBE and BE was determined using Lowry’s assay. An aliquot of 1 mL of extract is treated with 5 mL of Reagent A and kept in the dark for 10 min. Then the mixture was loaded with 0.5 mL of Folin-Ciocalteu reagent and left in a dark place for 30 min. After incubation, the absorbance was measured at 630nm. Protein concentration (mg/mL) was determined using the Bovine Serum Albumin (BSA) standard curve in the extracted samples.
Reagent A is made by combining 99 mL of reagent B (2% Na2CO3 and 0.1N NaOH), 0.5 mL of reagent C (2% of copper sulfate pentahydrate), and 0.5 mL of reagent D (2% of potassium sodium tartrate).
The total carbohydrate content of extracted samples was determined using the Anthrone technique, with glucose (10–80 mg/L) used as the standard. For the test, 1 mL of extract is treated with 5 mL of the anthrone reagent (dissolve 2g of anthrone in 1000 mL of concentrated sulfuric acid) and incubated at 100°C for 10 min. After incubation, the absorbance was measured at 620nm. Carbohydrate concentration (mg/mL) was determined using the glucose standard curve in the extracted samples.
The total flavonoid content (TFC) of fungal MBE and BE extracts was evaluated according to the method described by Wandati et al. (2013). An aliquot of 1 mL of the sample was added to 0.3 mL of 5% NaNO2, and 4 mL of distilled water was kept for 5 min. Then the mixture was loaded with 0.3 mL of 10% AlCl3 and allowed to sit for 6 min. Finally, 2 mL of 1 M NaOH was added to these tubes, followed by 10 ml of distilled water. The absorbance was measured at 415nm. Quercetin was used as a standard in a concentration range of 20–100 µg/mL, and the result was expressed as µg QE/g DW.
The total phenol content (TPC) of the fungal MBE and BE extracts was analyzed using the Folin-Ciocalteu method of Wandati et al. (2013), with some modifications. Each sample (1 mL) was mixed with 0.5 mL of 10% (v/v) Folin-Ciocalteu phenol reagent and 2 mL of 700 mM Na2CO3 in distilled H2O. The mixture was vortexed well and left for 30 min. After incubation, the absorbance was measured at 765nm. The TPC in the extract was expressed as μg gallic acid equivalent (GAE) per g dry weight (μg GAE/g DW).
The total amount of saponin in fungal MBE and BE extracts was analyzed as described by Le et al. (2018). An aliquot of 1 mL of the sample was added to 1 mL of 8% vanillin and 2.5 mL of 72% sulfuric acid. The mixture was incubated for 15 min at 60°C. After incubation, the absorbance was measured at 560nm. Aescin was used as a standard in a range of 20–100 µg/mL, and the result was expressed as mg Aescin/g DW.
The total anthraquinone content of fungal MBE and BE extracts was evaluated according to the method described by Sakulpanich and Gritsanapan (2008), with some modifications. In 1 mL of sample, 10 mL of distilled water was added. Then, 20 mL of 10.5% ferric chloride was added, and the mixture was mixed and refluxed for 10 min. Add 1 mL of sulfuric acid and reflux for another 10 min. Transfer the final content into a separating funnel, and extraction was done three times with 25 mL of ether. After that, the ether layer was evaporated to dryness to collect the residues. The residues were dissolved in 1 ml of 0.5% Mg(CH3COO)2 in CH3OH, and absorbance was measured at 515 nm. Rhein was used as a standard in a range of 20–100 µg/ml, and the results were expressed as µg Rhein/g DW.
The total alkaloid content in macrofungal MBE and BE extracts was determined as described by Nantongo et al. (2018). A total of 50 mL of 10% acetic acid in ethanol was added to 100 mg of the sample; the mixture was covered and incubated for 4 h. After that, the extract was concentrated in a water bath to one-quarter of the original volume, followed by the addition of five drops of concentrated NH4OH to precipitate alkaloids. The precipitate was allowed to rest for 3 h to sediment before being rinsed with 10 mL of 0.1M NH4OH and filtered. The residue on the paper was dried at 60°C for 30 min and then reweighed. Mathematically, the % of the alkaloid is calculated as mentioned in Equation 1:
The terpenoid content in macrofungal MBE and BE extracts was determined as described in Malik (2017). A total of 9 mL of ethanol was added to 100 mg of extract and incubated in the mixture for 24 h. Using the separating funnel, the extract was filtered with 10 mL of petroleum ether. A pre-weighed glass vial was used to collect the ether extract. At room temperature, the solvent was allowed to completely evaporate and then reweighed. The % terpenoids content was measured using the formula given in Equation 2:
The total tannin content in macrofungal MBE and BE extracts was determined using the Folin-Denis reagent. 10 mL of distilled water was added to 1mg of extract, gently boiled for 1 h in a water bath, and filtered using Whatman filter paper in a 10 mL volumetric flask. To this content, 5 mL of Folin-Denis reagent and 10 mL of saturated Na2CO3 solution were added and incubated at 25°C in a water bath for 30 min. After incubation, the absorbance was measured at 700 nm. The amount of tannin in the extracts was calculated using the equation developed on the standard curve of tannic acid.
The sterol content in macrofungal MBE and BE extracts was determined using the Liebermann-Burchard reagent, and cholesterol was used as the standard concentration range of 0.2–1 mg/mL. In 1 ml of acetic acid, 1 mg of the extract was dissolved. Then, it was treated with 5 mL of Liebermann-Burchard reagent and incubated at 37°C in a water bath for 10 min. After incubation, the absorbance was measured at 650 nm.
The ability of the MBE and BE extracts to donate electrons and scavenge DPPH radicals was determined by the slightly modified method of Anjana et al. (2016). A freshly prepared 1 mM ethanol solution of DPPH was mixed with the mycelium biomass and mushroom extract at a ratio of 1:0.5 (v/v). The mixture was vortexed properly and incubated in the dark for 10 min. The light absorption was measured at 517 nm. The % scavenging value at the variable concentration was used to plot a regression curve, and the equation generated on the curve was used to estimate the IC50 value for both extract samples. The Equation 3 is used for % scavenging calculation:
The amount of β-glucan in fungal MBE and BE was measured based on the enzymatic release of glucose, as described in Toledo et al. (2013). This procedure calls for a standard reactive glucose solution with a concentration of 100 mg dL-1, an enzyme reactive mixture with glucose oxidase and peroxidase, color reagent 1 (4-aminophenazone, 25 mM, 920 mM Tris), and color reagent 2 (55 mM phenol). The working reagent was prepared in a 250-mL volumetric flask using 225 mL of distilled water, 12.5 mL of color reagents 1 and 2, and 0.75 mL of enzyme reactive. In total, 20 mL of each sample extract was added to 2 mL of working reagent in the reaction mixture and incubated at 37°C for 15 min. After that, the absorbance at 505 nm was determined using a spectrophotometer. Using 2 mL of the working reagent and 20 mL of standard reactive glucose, the reaction was carried out under the same conditions as above. The results were examined using Equation 4 below:
Where A denotes the absorbance of the sample at 505 nm, which is equal to 100 mg/dL of normal glucose, and 0.9 denotes the proportion of free glucose conversion that was established for anhydrous glucose, which occurs in β-glucan.
The contents of the total α- and β-glucans were determined in the MBE and BE using the mushroom and yeast β-glucan assay kit (Megazyme Int., Dublin, Ireland), following the instructions of the manufacturer. Briefly, to estimate the total glucan content in the samples, 2 mL of ice-cold 12M sulfuric acid was used for the hydrolysis of the polysaccharides in the samples at 100°C for 2 h. After neutralization, hydrolysis proceeded to glucose using a mixture of exo-β-(1,3)-D-glucanase + β-glucosidase in sodium acetate buffer (pH 4.5) at 40°C for 1 h. For α-glucan content estimation, enzymatic hydrolysis with amyloglucosidase and invertase was conducted. After that, a glucose oxidase/peroxidase reagent was added to estimate the total glucan and α-glucan content at 510nm. The β-glucan content was calculated by subtracting the α-glucan from the total glucan content. All values of total, α-, and β-glucans in biomass and mushrooms were expressed in g/100g of DW.
Determination of β-glucan: The β-glucan content was determined by subtracting the α glucan content from the total glucan content.
The identification and quantification of phytochemicals from mycelium biomass and mushroom extracts were performed using the high-performance liquid chromatography (PDA) technique. Chromatography was performed on a 250mm x 4.5mm RP C-18 column. Preliminary experiments, in which various ratios of methanol and water were examined, helped to optimize the condition. Quercetin, sesquiterpene glycosides, ergosterol, and RidentinB were the standards employed for the identification and quantification of total polyphenols, terpenoids, sterols, and sesquiterpenes, respectively. The flow rate, column temperature, and detector wavelength were all adjusted to 1 mL/min, 25°C, and 210 to 370 nm, respectively.
The formula used for calculation is mentioned in Equation 5:
All the experiments were conducted in triplicate, and the values were expressed as mean ± SD. Statistical significance was detected by the Statistical Package for the Social Sciences (SPSS); a value of p<0.05 indicated a statistical difference.
In the present study, the submerged cultivation of Trametes sp. was conducted in a liquid (PDB) and solid medium (PDA). Trametes sp. grew on a PDA plate covered with mycelium, and the cultivation lasted 14 days at 20°C. In the broth medium, the grown culture formed a layer of mycelium on top of it, and relatively high quantities of mycelium biomass were produced, as represented in Figure 2C. Trametes sp. resembles the tail of a strutting turkey, having arched bands of brown, tan, and white color, as depicted in Figures 2A and B. According to studies, submerged cultivation offers a substitute method for consistently obtaining macrofungal biomass that produces essential metabolites, as well as a technique to lower downstream processing costs, lower the risk of contamination, and ensure sustainability (Tang et al., 2007; Lu et al., 2020; Bakratsas et al., 2021). The production of biomass was highly dependent on the strain, growth methods, and recovery procedures. We reported a high amount of biomass, 96.96 g/L, and after drying the biomass at 50°C for 2 h, we recovered 3 g DW/L. In a work by Angelova et al. (2022), they used a lyophilized approach to achieve 10.22 ± 0.28 g DW/L of mycelium biomass. However, there are still many obstacles in the way of its cultivation and extraction of phytochemicals and polysaccharides, especially β-glucan from Trametes sp. So far, there is very little comparative study that includes analysis of most of the valuable phytochemicals and polysaccharides, mainly β-glucan in Trametes sp. from both MBE and BE.
Figure 2 Represents Trametes sp. (A) Mycelium grown on potato dextrose agar medium (PDA); (B) After 14 days of incubation; (C) Mycelium grown on potato dextrose broth medium (PDB).
Wild mushrooms have highly variable morphological features; identifying them solely based on physical characteristics may be deceptive. According to Hussein et al. (2014); Khatun et al. (2017); Raja et al. (2017), and Ao et al. (2020), combining morphological and molecular data was the most effective way to advance the field of mushroom identification research. Here, we performed a partial sequence of the isolates, analyzed by BLAST search. The BLAST analysis of this sequence showed 100% sequence similarity with the Trametes flavida voucher OAB0196 large subunit ribosomal RNA gene (Table 3). A phylogenetic tree was constructed based on LSU F/R-rDNA fragment neighbor-joining using Phylogenetic Tree Builder software. The phylogenetic analysis revealed that NK-2 (mushroom sample) was found to be the closest homolog to the Trametes flavida voucher OAB0196 large subunit ribosomal RNA gene with sequence ID: MK736947. The next closest homolog was found to be the Trametes flavida voucher OAB0047 large subunit ribosomal RNA gene with sequence ID: MK736946, hence designated as Trametes sp. NK-2 (Figure 3).
It is important to note that the chemical composition of macrofungal biomass is strain-specific and strongly dependent on the environment and cultivation technique (Bains et al., 2021). These days, numerous techniques can help in the extraction process, such as methanol extraction (Sezer et al., 2017), enzyme assistance, microwave assistance, ultrasonic assistance, ethanol extraction (Bae et al., 2015; Angelova et al., 2022), and hot water extraction (Park and Hong, 2014). In this study, we used an 80% ethanol extraction method to extract pure phytochemicals and polysaccharides from MBE and BE. Further, this method is to be optimized and compared with the classical ethanol precipitation procedures.
The total protein content found in the BE and ME extracts of Trametes sp. in our study was 0.0153 and 0.1088 mg/mL, respectively, as represented in Table 4. However, in the report, crude protein content for basidiomycetes varies between 10% and 49% (Bakratsas et al., 2021; Angelova et al., 2022). In our study, we found 10% protein content in biomass, which falls below the reported range. Since cultivation techniques and substrate composition strongly affect the chemical composition of mushrooms, including protein content, research is constantly sought for new sources of proteins to meet the increasing protein demand of a continuously growing world population. Mushrooms can serve as an important source of protein because they can provide all essential amino acid requirements.
On the other hand, polysaccharides were only partially soluble since the total carbohydrate content in BE and MBE extracts reported in our study was 0.0257 and 0.0184 mg/mL, respectively, as depicted in Table 4. Hence, the polysaccharide solubility could be negatively affected by precipitation or drying methods, and its decrease was probably due to conformational changes.
The phytochemical compositions of the MBE and BE extracts were used to be analyzed by the systronic PC-based UV-Vis spectrophotometer 220.
The results of flavonoid in BE and MBE extracts were 11.16 µg/mL and 9.50 µg/mL, respectively, and the results of TPC in BE and MBE extracts were 12.7 µg/mL and 12.4 µg/mL, respectively, as represented in Table 5. Our results show high TFC and TPC content in 80% ethanol extraction compared to the result reported by Angelova et al. (2022) in methanol and ethanol extraction for both TPC and TFC; however, their water extraction proved to be a good choice in T. versicolor biomass treatment.
Table 5 The content of total flavonoids, phenolic content, saponins, and anthraquinones in BE and MBE extracts.
Other phytochemicals, such as saponin and anthraquinones, obtained the highest content among all phytochemicals in MBE compared to BE. Saponin was found to be the dominant phytochemical in biomass extract. The results of saponin in BE and MBE extracts were 8.60 µg/mL and 70.6 µg/mL, respectively, against the standard Aescin concentration range of 20–100 µg/mL. For anthraquinones, the standard uses a Rhein concentration range of 20–100 mg/mL. After saponin, anthraquinone has the second-highest content in MBE at 14.5 and 13.5 µg/mL in BE extract, as represented in Table 5. The result also suggests that the condition provided in submerged cultivation for biomass extraction was good; therefore, we obtained a high amount of saponin and anthraquinone content in biomass extract rather than mushroom extract. Similarly, Ogidi et al. (2021) carried out the anti-microbial efficacy of wild edible mushrooms, where they found saponin content was 0.15 ± 0.01%, suggesting the usefulness of the mushroom as a potential antimicrobial, anticarcinogenic, antimalarial, antiulcer, and hepaticidal effect. On the other hand, phytochemical studies by Doris (2018) on Trametes sp. suggest that they did not find anthraquinone content in ethanol extraction.
The percentage of alkaloid and terpenoid content was calculated using Equations 1 and 2, respectively. The results of alkaloids were 1.21% and 1.53% in BE and MBE extracts, respectively, and the results of terpenoids were 3.65% and 4.21% in BE and MBE extracts, as mentioned in Table 6. However, Doris (2018) phytochemical studies on Trametes sp. collected from Ondo, Akure, and Ipele districts in Ondo State in southwest Nigeria, using extractions from three solvents—ethanol, ethyl acetate, and hexane—determined the qualitative and quantitative analysis of nine secondary metabolites (alkaloids, tannins, saponins, flavonoids, terpenoid, steroid, and phlabotannin). All of the secondary metabolites examined were present in all of the mushrooms investigated, except for the alkaloids, phlabotannin, and anthraquinone.
Similarly, the results of tannin in BE and MBE extracts are 0.0778 and 0.0882 mg/mL, respectively, as represented in Table 6. However, Chaudhary et al. (2023) reported six wild mushrooms: Scleroderma citrinum, Suilluspunctatipes, Coriolushirsutus, Russulasanguinea,Heterobasidionannosum, and Cavimalum indicum, of which S. punctatipes had the maximum amount of tannin concentration (180 mg GAE/g).
The results of the sterol content in BE and MBE were 1.314 mg/mL and 1.592 mg/mL, respectively, as represented in Table 6.
In the present study, the antioxidant activity was assessed by the DPPH method. The IC50 value was used to measure antioxidant activity, and the IC50 value drops as antioxidant activity rises. As shown in Tables 7 and 8, MBE exhibited the highest antioxidant activity since its IC50 value is 405.042 µg/mL, while BE’s IC50 value is 376.032 µg/mL, suggesting the presence of a high content of TPC, TFC, saponin, and anthraquinone in biomass extract.
Sun et al. (2014) discovered six polysaccharide fractions of Coriolus versicolor (CVPS) among CVPS with low molecular weight, high protein content, and greater uronic acid content that showed a high radical scavenging effect, with CVPS-3 having the highest antioxidant activity and CVPS-3 exhibiting higher DPPH radical scavenging activity (64.9% at 0.8 mg/mL), respectively. The percentage of scavenging activity in BE and MBE extracts was calculated as mentioned in Equation 3. Compared with our result, Trametes sp. BE extract exhibits higher DPPH radical scavenging activity (62.9% at 0.5 mg/mL) than MBE (59.19% at 0.5 mg/mL).
In the current study, the content of β-glucan was calculated using Equation 4 in both MBE and BE obtained by submerged cultivation of Trametes sp. The MBE showed a higher β-glucan content of 1.713 mg/mL than the BE, which is 1.671 mg/mL, as reported in Table 9. Depending on the species, habitat, maturity, and cultivation techniques, the percentage of fiber found in the fruiting body or mycelium of a mushroom can range from 3.1% to 46.5% dry matter (DM) (Latgé, 2007; Kumari, 2020).
Further, to confirm the yield of β-glucan in Trametes sp. determined using the Mushroom and Yeast β-glucan assay kit (Megazyme Int., Dublin, Ireland), we obtained 42.5% in MBE. In our analysis, 300 mg of MBE contains total glucans 45.2025 (%w/w), α-glucan 2.6406 (%w/w), and β-glucan 42.5 (%w/w). However, in BE, the yield of β-glucan is comparatively low, 5.94%, which suggests that biomass extract could provide a good alternating source to obtain maximum pure β-glucan from Trametes sp.
The HPLC was used for the analysis of phytochemical total phenols, terpenoids, sterols, and sesquiterpene in both BE and MBE, represented in Figures 4–7, respectively, and their concentrations were measured using Equation 5, along with their standard (1 mg/mL). Peaks were detected by comparing them to the retention times of their respective standards. Due to a lack of standards, however, many of the compounds could not be identified. The peak that shows similarity with the standard one has that particular component that we were looking for in the sample; the other peak represents some other compounds that we will investigate in our next studies.
Figure 4 HPLC chromatograms of the standard quercetin (A) and the extract of MBE and BE (B) and (C), respectively. For the identification and quantification of phytochemical total polyphenols, methanol and 0.4% phosphoric acid (47:53) are used in the mobile phase.
Figure 5 HPLC chromatograms of the standard sesquiterpene glycosides (A) and the extract of MBE and BE (B) and (C), respectively. For the identification of phytochemical terpenoids, 0.1% formic acid and acetonitrile (60%–40%) are used in the mobile phase.
Figure 6 HPLC chromatograms of the standard ergosterol (A) and the extract of MBE and BE (B) and (C), respectively. For the identification and quantification of phytochemical sterols, methanol and acetonitrile (80:20) are used in the mobile phase.
Figure 7 HPLC chromatograms of the standard RidentinB (A) and the extract of MBE and BE (B) and (C), respectively. For the identification and quantification of phytochemical sesquiterpene, 60% acetonitrile water is used in the mobile phase.
The retention time for the standard quercetin was 8.320, as shown in Figure 4A, used for total phenolic content in MBE and BE extracts. Peak 10 with a retention time of 8.396 min represents MBE, as shown in Figure 4B, and peak 8 with a retention time of 8.327 min represents BE, as shown in Figure 4C. Bristy et al. (2022) also evaluated the phenolic content of three varieties of mushrooms growing in Bangladesh, namely G. lucidum, G. tropicum, and C. indica, using the HPLC-DAD technique, and their findings show that the locally cultivated mushroom G. lucidum has the greatest total phenolic content.
The retention time for the standard sesquiterpene glycosides was 4.256 min, as shown in Figure 5A, used for terpenoid content in MBE and BE. Peak 4 with a retention time of 4.259 min represents MBE, shown in Figure 5B, and peak 5 with a retention time of 4.205 min represents BE, shown in Figure 5C, respectively, as shown in Figure 3. According to Peng and Shahidi (2022), screening and characterization of secondary metabolites of Newfoundland chaga mushroom using HPLC-ToF-MS (high-performance liquid chromatography coupled with time-of-flight mass spectra). Their chaga sample contains 108 terpenoids that have been tentatively identified, but only 30 can be recognized. The remaining 78 terpenoids were discovered to be novel.
The retention time for the standard ergosterol was 9.016 min, as shown in Figure 6A, used for sterol content in MBE and BE extracts. Peak 6 with a retention time of 9.014 min represents MBE, as shown in Figure 6B, and peak 11 with a retention time of 9.015 min represents BE, as shown in Figure 6C. Gil-Ramirez et al. (2011) performed an aGC column and observed that, except for G. lucidum, all of the analyzed samples contained equal amounts of ergosterol and ergosta-7,22-dienol. The distribution of ergosterol derivatives varied depending on the strain. The selected strain ergosterol concentrations ranged from 0.7 to 5.7 mg/g, with B. edulis having the highest levels and C. cornucopioides having the lowest.
The retention time for the standard RidentinB used for sesquiterpene content was 5.784 min, as shown in Figure 7A; peak 6 represents MBE in Figure 7B with a retention time of 5.344 min; and peak 2 represents BE in Figure 7C with a retention time of 4.04 min. Further, for the first time, Lee et al. (2020) reported linear sesquiterpene carboxylic acids in Phallus gluteus. They used liquid chromatography-mass spectrometry to investigate the methanol extract of Phallus gluteus and discovered two novel sesquiterpenes, phallic acid A and B.
Additionally, Gogoi et al. (2019) observed that reverse-phase high-pressure liquid chromatography (RP-HPLC) revealed that mushroom extract had a significant amount of gallic acid, caffeic acid, ascorbic acid, and quercetin and can be considered a good source of natural antioxidants.
Mushrooms have high biological value, help to strengthen the body, flush out toxins, dissipate heat, boost immunity, and uplift the spirit and vitality (Bolla et al., 2010; Feng et al., 2016, 2017; Liu and Zhang, 2018; Yin et al., 2020). Trametes sp. possesses a highly bioactive mycelium (Benson et al., 2019). It will be highly beneficial because this fungus has numerous applications in the food (Bakratsas et al., 2021), pharmaceutical (Leliebre-Lara et al., 2015), bioremediation (Han, 2021), cosmetic (Vaithanomsat et al., 2022), and health sectors. Moreover, the macrofungal biomass could be considered more beneficial in detoxification processes and oxidative stress prevention than the pure macrofungal extracts (Valverde et al., 2015). After cultivating various strains of basidiomycetes for 14 days, the most recorded biomass yields varied from 0.1 g/L to 16.0 g/L (Maziero et al., 1999; Jaros et al., 2018). Nevertheless, we reported a high amount of biomass, which is 96.96 g/L, and after drying the biomass, we obtained 3 g of DW/L biomass. A few studies have been conducted on bioactive metabolites from medicinal mushrooms, primarily Trametes sp., and those that have attempted to do so have found that the conditions required for the cultivation and extraction of metabolites from mushrooms are costly and hence not economical (Kothiyal and Singh, 2022). As Trametes sp. is the most researched and effective medicinal fungus, no established technique exists for cultivating and extracting the maximum quantity of β-glucan from the mushroom.
However, ethanol extraction was discovered to be the most effective method for extracting phytochemicals and polysaccharides from the biomass of mushrooms and their fruiting bodies. Shomali et al. (2019) examined the polyphenolic contents and biological activities of four wild mushrooms and found that the ethanolic extracts of I. hispidus had the highest total phenolic and flavonoid contents when compared to other mushroom species. Our results also showed high β-glucan, total phenolic, flavonoid, saponin, and anthraquinone content. The phytochemical screening of P. ostreatus by Rahimah et al. (2019) revealed that 70% ethanol extract appeared to contain 6.67 μg/mL total phenolic content, which is almost half of what we found in terms of biomass and mushroom extract. In addition, Nkadimeng et al.’s (Nkadimeng et al., 2020) study on Psilocybenatalensis mushroom showed that phytochemical analysis supported the higher beneficial effects of ethanol extracts than water extracts. Furthermore, Rahimah et al. (2019) found that, based on the foam produced, the phytochemical screening revealed that ethanol extract appeared to have the highest concentration of saponin, similar to our result. Similarly, the results of Kothiyal and Singh’s (Kothiyal and Singh, 2022) antimicrobial and phytochemical screening of wild mushrooms that are naturally found in the Garhwal Himalayan region of Uttarakhand, India, indicate that the ethanol extract of Trametes versicolor tested positive for terpenoids, steroids, glycosides, flavonoids, carbohydrates, and amino acids; however, the tests for alkaloids, flavonoids, phenolic compounds, carbohydrates (Benedict’s test, Barfoed test), amino acids (Millon’s test, Xanthoproteic), saponins, and inorganic acids were negative. Additionally, the ethanolic extract of Trametes sp. exhibits the highest level of total phenolic and flavonoid content when compared to our results.
Recently, research has focused on the many health benefits of dietary fibers with macrofungal origins. β-glucan exhibits potential as a therapy for diabetes and cardiovascular risk. The amount of β-glucan obtained in our work is significantly greater than that of estimates of β-glucan obtained from a variety of mushroom species, such as Russula alatoreticula (Khatua et al., 2021), Polyporus grammocephalus (Patra et al., 2021), Pleurotus djamor (Maity et al., 2019), and Pleurotus eryngii (Al-Saffar et al., 2020). There are currently no published reports available from India on the extraction of β-glucan from Trametes sp. Moreover, our target audience will be cancer patients, given that the disease has probably affected people for as long as life itself. Al-Saffar et al. (2020) investigated the antioxidant activities of β-glucan that was extracted from Pleurotus eryngii using a hot water extraction method; the yield was 7.9%. MCF-7 and HepG2 cell lines were used to test the anticancer activity of β-glucan at several doses. In the cell walls of mushrooms, glucans help to build the structure (Khan et al., 2018; Ruiz-Herrera and Ortiz-Castellanos, 2019). By dry weight, β-glucans make up as much as 50% and α-glucans as much as 10% of the cell walls. The most isolated glucans from mushrooms are β-glucans, which typically have -1,3-glucans with -1,6 branches and have an abundance of important bioactivities (Maity et al., 2017; Yin et al., 2020; Cognigni et al., 2021; Ruthes et al., 2021). β-glucan also exhibited anti-collagenase, anti-elastase, and anti-hyaluronidase activity and can serve as an important component in the cosmetics industry as well (Sangthong et al., 2022).
Concerning mushroom potential medical benefits, our main focus was identifying the maximum phytochemical and polysaccharide contents of biomass extract and mushroom fruiting bodies. The findings we obtained indicated a high concentration of these substances. Yet, there is not a standard extraction procedure for β-glucan derived from mushrooms. In the future, we plan to standardize methods of cultivation and extraction from mushrooms (Trametes sp.) to boost the yield of polysaccharides.
In the present study, Trametes sp. submerged cultivation was carried out. The phytochemical and polysaccharide content of mushroom fruiting bodies and biomass were determined, along with the feasibility of using them as healthy food supplements. Our results demonstrate that the medicinal fungus Trametes sp. biomass has the highest levels of saponin (70.6 µg/mL), phytochemicals followed by anthraquinone (14.5 µg/mL), total phenolic (12.45 µg/mL), and flavonoid (9.500 µg/mL). Also, the macrofungal biomass extract showed 42% β-glucan content in Trametes sp. On the other hand, alkaloid and terpenoid content were the lowest among all the phytochemicals. The findings of this study indicate that phytochemicals and polysaccharides extracted from submerged fungal biomass and fruiting bodies from Trametes sp. could represent an intriguing method to obtain minimally processed natural dietary supplements with significant health benefits. The findings of this study suggest that, despite the existence of secondary metabolites that typically have antioxidant action in Trametes sp. mushrooms, such as flavonoids, terpenoids, steroids, tannins, saponins, sterols, and total phenol, more research on mushrooms with diverse substrates and variables is needed to boost their phytochemical, polysaccharide, and antioxidant activity.
The original contributions presented in the study are included in the article/supplementary material, further inquiries can be directed to the corresponding author/s.
MS: Writing – review & editing, Resources, Funding acquisition. MK: Writing – review & editing, Data curation, Writing – original draft. SK: Writing – review & editing, Conceptualization, Formal analysis, Resources, Supervision, Validation, Project administration. AB: Writing – review & editing. VM: Writing – review & editing, Funding acquisition, Investigation. SM: Writing – review & editing, Funding acquisition, Investigation.
The author(s) declare financial support was received for the research, authorship, and/or publication of this article. The authors are thankful to SBS University, Dehradun, for providing a space facility to conduct this work. Similarly, the authors are also thankful to M/s Xcel Life Science, California, United States, for providing the funds to carry out this research work (Grant No. SBSU/XcelLs/2021/4/P1).
Authors are thankful to SBS University, Dehradun, for providing space facility to conduct this work. Similarly authors are also thankful to XCel Life Science, California for providing the fund to carry out this research work.
The authors declare that the research was conducted in the absence of any commercial or financial relationships that could be construed as a potential conflict of interest.
All claims expressed in this article are solely those of the authors and do not necessarily represent those of their affiliated organizations, or those of the publisher, the editors and the reviewers. Any product that may be evaluated in this article, or claim that may be made by its manufacturer, is not guaranteed or endorsed by the publisher.
Afiati F., Firza S. F., Kusmiati K., Aliya L. S. (2019). “The effectiveness β-glucan of shiitake mushrooms and Saccharomyces cerevisiae as antidiabetic and antioxidant in mice Sprague Dawley induced alloxan,” in AIP Conference Proceedings, Vol. 2120 (AIP Publishing).
Akgul H., Sevindik M., Coban C., Alli H., Selamoglu Z. (2017). New approaches in traditional and complementary alternative medicine practices: Auricularia auricula and Trametes versicolor. J. Tradit. Med. Clin. Natur. 6, 239. doi: 10.4172/2573-4555
Al-Saffar A. Z., Hadi N. A., Khalaf H. M. (2020). Antitumor activity of β-glucan extracted from Pleurotus eryngii. Indian J. Forensic Med. Toxicol. 14, 2493. doi: 10.37506/ijfmt.v14i3.10811
Angelova G., Brazkova M., Mihaylova D., Slavov A., Petkova N., Blazheva D., et al. (2022). Bioactivity of biomass and crude exopolysaccharides obtained by controlled submerged cultivation of medicinal mushroom Trametes versicolor. J. Fungi 8, p.738. doi: 10.3390/jof8070738
Anjana S. K. G., Balamurugan T. S. B., Manivasagan V., Babu N. G. R. (2016). Phytochemical, antioxidant and antitumor activity of edible mushroom Pleurotus ostreatus. Int. J. Adv. Res. Biol. Sci. 3, 170–177. doi: 10.22192/ijarbs.2016.03.09.024
Ao T., Deb C. R., Rao S. R. (2020). Molecular strategies for identification and characterization of some wild edible mushrooms of Nagaland, India. Mol. Biol. Rep. 47, 621–630. doi: 10.1007/s11033-019-05170-2
Awadasseid A., Eugene K., Jamal M., Hou J., Musa Hago A., Gamallat Y., et al. (2017). Effect of Coriolus versicolor glucan on the stimulation of cytokine production in sarcoma-180-bearing mice. Biomed. Rep. 7, 567–572. doi: 10.3892/br
Bae H. K., Hwang I. W., Hong H. D., Chung S. K. (2015). Antioxidant capacities and β-glucan content of ethanol extract from Phellinus baumii. Korean J. Food Preserv. 22, 721–726. doi: 10.11002/kjfp.2015.22.5.721
Bains A., Chawla P., Kaur S., Najda A., Fogarasi M., Fogarasi S. (2021). Bioactives from mushroom: health attributes and food industry applications. Materials 14, 7640. doi: 10.3390/ma14247640
Bakratsas G., Polydera A., Katapodis P., Stamatis H. (2021). Recent trends in submerged cultivation of mushrooms and their application as a source of nutraceuticals and food additives. Future Foods 4, 100086. doi: 10.1016/j.fufo.2021.100086
Barreras-Urbina C. G., Rodríguez-Félix F., Madera-Santana T. J., Montaño-Grijalva E. A., Figueroa-Enríquez C. E., Larez F. L. G., et al. (2023). “Turkey Tail (Trametes versicolor),” in Mushrooms: Nutraceuticals and Functional Foods (Boca Raton: CRC Press), 330–340.
Benson K. F., Stamets P., Davis R., Nally R., Taylor A., Slater S., et al. (2019). The mycelium of the Trametes versicolor (Turkey tail) mushroom and its fermented substrate each show potent and complementary immune activating properties in vitro. BMC complementary and alternative medicine. 19 (1), 1–14.
Bhambri A., Srivastava M., Mahale V. G., Mahale S., Karn S. K. (2022). Mushrooms as potential sources of active metabolites and medicines. Front. Microbiol. 13, 837266. doi: 10.3389/fmicb.2022.837266
Bolla K., Gopinath B. V., Shaheen S. Z., Charya M. S. (2010). Optimization of carbon and nitrogen sources of submerged culture process for the production of mycelial biomass and exopolysaccharides by Trametes versicolor. Yeast 14, 7–6. doi: 10.5897/IJBMBR.9000031
Bristy A. T., Islam T., Ahmed R., Hossain J., Reza H. M., Jain P. (2022). Evaluation of total phenolic content, HPLC analysis, and antioxidant potential of three local varieties of mushroom: A comparative study. Int. J. Food Sci. 2022, 11. doi: 10.1155/2022/3834936
Bruno W. J., Socci N. D., Halpern A. L. (2000). Weighted neighbor joining: a likelihood-based approach to distance-based phylogeny reconstruction. Mol. Biol. Evol. 17, 189–197. doi: 10.1093/oxfordjournals.molbev.a026231
Bucić-Kojić A., Fernandes F., Silva T., Planinić M., Tišma M., Šelo G., et al. (2020). Enhancement of the anti-inflammatory properties of grape pomace treated by Trametes versicolor. Food Funct. 11, 680–688. doi: 10.1039/C9FO02296A
Chang Y., Zhang M., Jiang Y., Liu Y., Luo H., Hao C., et al. (2017). Preclinical and clinical studies of Coriolus versicolor polysaccharopeptide as an immunotherapeutic in China. Discovery Med. 23, 207–219.
Chaudhary P., Panth N., Raut B. K., Pokhrel N., Shrestha N., Shakya S., et al. (2023). Biochemical, Antimicrobial, and Antioxidant activities of some wild Mushrooms from Nepal. BIBECHANA 20, 161–174. doi: 10.3126/bibechana.v20i2.54887
Chuang W. Y., Hsieh Y. C., Lee T. T. (2020). The effects of fungal feed additives in animals: A review. Animals 10, 805. doi: 10.3390/ani10050805
Cognigni V., Ranallo N., Tronconi F., Morgese F., Berardi R. (2021). Potential benefit of β-glucans as adjuvant therapy in immuno-oncology: A review. Explor. Target. Anti-tumor Ther. 2, 122. doi: 10.37349/etat
Cui J., Chisti Y. (2003). Polysaccharopeptides of Coriolus versicolor: physiological activity, uses, and production. Biotechnol. Adv. 21, 109–122. doi: 10.1016/S0734-9750(03)00002-8
Doris O. Y. (2018). Comparative phytochemical screening of trametes species: A wild mushroom collected from Ondo state Nigeria. SF J. Mycol. 1 (4).
Dou H., Chang Y., Zhang L. (2019). Coriolus versicolor polysaccharopeptide as an immunotherapeutic in China. Prog. Mol. Biol. Trans. Sci. 163, 361–381. doi: 10.1016/bs.pmbts.2019.03.001
Feng J., Feng N., Zhang J. S., Yang Y., Jia W., Lin C. C. (2016). A new temperature control shifting strategy for enhanced triterpene production by Ganoderma lucidum G0119 based on submerged liquid fermentation. Appl. Biochem. Biotechnol. 180, 740–752. doi: 10.1007/s12010-016-2129-1
Feng J., Zhang J. S., Feng N., Yan M. Q., Yang Y., Jia W., et al. (2017). A novel Ganoderma lucidum G0119 fermentation strategy for enhanced triterpenes production by statistical process optimization and addition of oleic acid. Eng. Life Sci. 17, 430–439. doi: 10.1002/elsc.201600071
Gil-Ramirez A., Clavijo C., Palanisamy M., Soler-Rivas C., Ruiz-Rodriguez A., Marín F. R., et al. (2011). “Edible mushrooms as potential sources of new hypocholesterolemic compounds,” in Proceedings of the 7th International Conference on Mushroom Biology and Mushroom Products (Villenaved'Ornon Cedex, France: Institut National de la Recherche Agronomique (INRA)), Vol. 2. 110–119.
Gogoi P., Chutia P., Singh P., Mahanta C. L. (2019). Effect of optimized ultrasound-assisted aqueous and ethanolic extraction of Pleurotus citrinopileatusmushroom on total phenol, flavonoids and antioxidant properties. J. Food Process Eng. 42, e13172. doi: 10.1111/jfpe.13172
Grand L. F., Vernia C. S. (2002). New Taxa and hosts of poroid wood-decay fungi in North Carolina. Castanea 67, 193–200.
Habtemariam S. (2020). Trametes versicolor (Synn. Coriolus versicolor) polysaccharides in cancer therapy: Targets and efficacy. Biomedicines 8, 135. doi: 10.3390/biomedicines8050135
Han M. (2021). The Mycoremediation of Escherichia coli by Pleurotus ostreatus, Stropharia rugosoannulata, and Trametes versicolor in Contaminated Water. J. South Carolina Acad. Sci. 19, 9.
Hawksworth D. L., Lücking R. (2017). Fungal diversity revisited: 2.2 to 3.8 million species. Microbiol. Spectr. 5, 10–1128. doi: 10.1128/microbiolspec.FUNK-0052-2016
Hussein J. M., Tibuhwa D. D., Mshandete A. M., Kivaisi A. K. (2014). Molecular phylogeny of saprophytic wild edible mushroom species from Tanzania based on ITS and nLSU rDNA sequences. Curr. Res. Environ. Appl. Mycol 4, 250–260. doi: 10.5943/cream/
Jaros D., Köbsch J., Rohm H. (2018). Exopolysaccharides from Basidiomycota: Formation, isolation and techno-functional properties. Eng. Life Sci. 18, 743–752. doi: 10.1002/elsc.201800117
Karn S. K., Chakrabarti S. K., Reddy M. S. (2011). Degradation of pentachlorophenol by Kocuria sp. CL2 isolated from secondary sludge of pulp and paper mill. Biodegradation 22, 63–69. doi: 10.1007/s10532-010-9376-6
Khan A. A., Gani A., Khanday F. A., Masoodi F. A. (2018). Biological and pharmaceutical activities of mushroom β-glucan discussed as a potential functional food ingredient. Bioactive carbohydrates and dietary fibre. 16, 1–13.
Khatua S., Chandra S., Acharya K. (2021). Hot alkali-extracted antioxidative crude polysaccharide from a novel mushroom enhances immune response via TLR-mediated NF-κB activation: a strategy for full utilization of a neglected tribal food. J. Food Biochem. 45, e13594. doi: 10.1111/jfbc.13594
Khatun S., Islam A., Gupta K., Gupta B. (2017). Detection of edible mushroom species by using molecular markers. Mol. Markers Mycol.: Diagn. Marker Develop., 201–224.
Kirk P. (2021). The CABI Bioscience Database of Fungal Names (Great Britain, Kew). Available at: http://www.indexfungorum.org.
Kothiyal G., Singh K. (2022). Antimicrobial and phytochemical screening of selected wild mushrooms naturally found in Garhwal Himalayan region, Uttarakhand, India. Journal of Advanced Biotechnology and Experimental Therapeutics (JABET). 57, 417-432. doi: 10.5455/jabet
Kumari K. (2020). Mushrooms as source of dietary fiber and its medicinal value: A review article. J. Pharmacog. Phytochem. 9, 2075–2078.
Latgé J. P. (2007). The cell wall: a carbohydrate armour for the fungal cell. Mol. Microbiol. 66, 279–290. doi: 10.1111/j.1365-2958.2007.05872.x
Le A. V., Parks S. E., Nguyen M. H., Roach P. D. (2018). Improving the vanillin-sulphuric acid method for quantifying total saponins. Technologies 6, 84. doi: 10.3390/technologies6030084
Lee S., Ryoo R., Choi J. H., Kim J. H., Kim S. H., Kim K. H. (2020). Trichothecene and tremulane sesquiterpenes from a hallucinogenic mushroom Gymnopilusjunonius and their cytotoxicity. Arch. pharmacal Res. 43, 214–223. doi: 10.1007/s12272-020-01213-6
Leliebre-Lara V., García M., Nogueiras C., Monzote L. (2015). Qualitative analysis of an ethanolic extract from Trametes versicolor and biological screening against Leishmania amazonensis. Emirates J. Food Agric., 592–595. doi: 10.9755/ejfa.
Leliebre-Lara V., Monzote Fidalgo L., Pferschy-Wenzig E. M., Kunert O., Nogueiras Lima C., Bauer R. (2016). In vitro antileishmanial activity of sterols from Trametes versicolor (Bres. Rivarden). Molecules 21, 1045. doi: 10.3390/molecules21081045
Lin H. C., Hewage R. T., Lu Y. C., Chooi Y. H. (2019). Biosynthesis of bioactive natural products from Basidiomycota. Organ. Biomol. Chem. 17, 1027–1036. doi: 10.1039/C8OB02774A
Liu S. R., Zhang W. R. (2018). Hyperproduction of exopolysaccharides by submerged mycelial culture of Ganoderma lucidum using a solid seed grown in fine-powder of wheat bran and in vitro evaluation of the antioxidant activity of the exopolysaccharides produced. Food Sci. Biotechnol. 27, 1129–1136. doi: 10.1007/s10068-018-0343-z
Lowenthal R., Taylor M., Gidden J. A., Heflin B., Lay J. O. Jr, Avaritt N., et al. (2023). The mycelium of the Trametes versicolor synn. Coriolus versicolor (Turkey tail mushroom) exhibit anti-melanoma activity in vitro. Biomed. Pharmacother. 161, 114424. doi: 10.1016/j.biopha.2023.114424
Lu H., Lou H., Hu J., Liu Z., Chen Q. (2020). Macrofungi: A review of cultivation strategies, bioactivity, and application of mushrooms. Compr. Rev. Food Sci. Food Saf. 19, 2333–2356. doi: 10.1111/1541-4337.12602
Maity G. N., Maity P., Choudhuri I., Bhattacharyya N., Acharya K., Dalai S., et al. (2019). Structural studies of a water insoluble β-glucan from Pleurotus djamor and its cytotoxic effect against PA1, ovarian carcinoma cells. Carbohydr. polym. 222, 114990. doi: 10.1016/j.carbpol.2019.114990
Maity P., Nandi A. K., Manna D. K., Pattanayak M., Sen I. K., Bhanja S. K., et al. (2017). Structural characterization and antioxidant activity of a glucan from Meripilus giganteus. Carbohydr. Polym. 157, 1237–1245. doi: 10.1016/j.carbpol.2016.11.006
Malik S. K. (2017). Qualtitative and quantitative estimation of terpenoid contents in some important plants of Punjab, Pakistan. Pakistan J. Sci. 69 (2). doi: 10.57041/pjs.v69i2.364
Maziero R., Cavazzoni V., Bononi V. L. R. (1999). Screening of basidiomycetes for the production of exopolysaccharide and biomass in submerged culture. Rev. microbiol. 30, 77–84. doi: 10.1590/S0001-37141999000100015
Nantongo J. S., Odoi J. B., Abigaba G., Gwali S. (2018). Variability of phenolic and alkaloid content in different plant parts of Carissa edulis Vahl and Zanthoxylum chalybeum Engl. BMC Res. Notes 11, 1–5. doi: 10.1186/s13104-018-3238-4
Nkadimeng S. M., Nabatanzi A., Steinmann C. M., Eloff J. N. (2020). Phytochemical, cytotoxicity, antioxidant and anti-inflammatory effects of Psilocybenatalensis magic mushroom. Plants 9, 1127. doi: 10.3390/plants9091127
Ogidi O. I., Oguoma L. M. O., Adigwe P. C., Anthony B. B. (2021). Phytochemical properties and in-vitro antimicrobial potency of wild edible mushrooms (Pleurotus ostreatus) obtained from Yenagoa, Nigeri. J. Phytopharmacol. 10, 180–184. doi: 10.31254/phyto
Park H. M., Hong J. H. (2014). Antioxidant activity of extracts with extraction methods from Phellinus linteus mycelium on Mori ramulus. Food Sci. Preserv. 21, 565–572. doi: 10.11002/kjfp.2014.21.4.565
Patra S., Maity P., Chakraborty I., Sen I. K., Ghosh D., Rout D., et al. (2021). Structural studies of immunomodulatory (1→ 3)-,(1→ 4)-α glucan from an edible mushroom Polyporus grammocephalus. Int. J. Biol. Macromol. 168, 649–655. doi: 10.1016/j.ijbiomac.2020.11.121
Peng H., Shahidi F. (2022). Qualitative analysis of secondary metabolites of chaga mushroom (Inonotus Obliquus): phenolics, fatty acids, and terpenoids. J. Food Bioact. 17. doi: 10.31665/JFB.2022.17304
Piotrowski J., Jedrzejewski T., Kozak W. (2015). Immunomodulatory and antitumor properties of polysaccharide peptide (PSP). Postepy Hig. Med. Dosw 69, 91–97. doi: 10.5604/17322693.1137086
Puia I. C., Aida P. U.I.A., Chedea V. S., Leopold N., Bocsan I. C., Buzoianu A. D. (2018). Characterization of Trametes versicolor: Medicinal mushroom with important health benefits. NotulaeBotanicae Horti Agrobotanici Cluj-Napoca 46(2), 343–349.
Rahimah S. B., Djunaedi D. D., Soeroto A. Y., Bisri T. (2019). The phytochemical screening, total phenolic contents and antioxidant activities in vitro of white oyster mushroom (Pleurotus ostreatus) preparations. Open Access Macedonian J. Med. Sci. 7, 2404. doi: 10.3889/oamjms.2019.741
Raja H. A., Miller A. N., Pearce C. J., Oberlies N. H. (2017). Fungal identification using molecular tools: a primer for the natural products research community. J. Natural prod. 80, 756–770. doi: 10.1021/acs.jnatprod.6b01085
Ruiz-Herrera J., Ortiz-Castellanos L. (2019). Cell wall glucans of fungi. A review. The Cell Surface. 5, 100022.
Ruthes A. C., Cantu-Jungles T. M., Cordeiro L. M., Iacomini M. (2021). Prebiotic potential of mushroom d-glucans: Implications of physicochemical properties and structural features. Carbohydr. Polym. 262, 117940. doi: 10.1016/j.carbpol.2021.117940
Sakulpanich A., Gritsanapan W. (2008). Extraction method for high content of anthraquinones from Cassia fistula pods. J. Health Res. 22, 167–172.
Sangthong S., Pintathong P., Pongsua P., Jirarat A., Chaiwut P. (2022). Polysaccharides from Volvariella volvacea mushroom: Extraction, biological activities and cosmetic efficacy. J. Fungi 8, 572. doi: 10.3390/jof8060572
Selamoglu Z., Sevindik M., Bal C., Ozaltun B., Sen İ., Pasdaran A. (2020). Antioxidant, antimicrobial and DNA protection activities of phenolic content of Tricholoma virgatum (Fr.) P. Kumm. Biointerface Research in Applied Chemistry 10, 8. doi: 10.33263/BRIAC103.500506
Sevindik M., Akgul H., Akata I., Selamoglu Z. (2017). Geastrum pectinatum as an alternative antioxidant source with some biochemical analysis. Med. Mycol.: Open Access 3, 1–4. doi: 10.21767/2471-8521
Sevindik M., Akgul H., Dogan M., Akata I., Selamoglu Z. (2018). Determination of antioxidant, antimicrobial, DNA protective activity and heavy metals content of Laetiporus sulphureus. Fresenius Environmental Bulletin 27, 1946-1952.
Sevindik M., Akgul H., Selamoglu Z., Braidy N. (2020). Antioxidant and antigenotoxic potential of Infundibulicybe geotropa mushroom collected from Northwestern Turkey. Oxid. Med. Cell. Longevity 2020. 10, 8. doi: 10.1155/2020/5620484
Sevindik M., Akgul H., Selamoglu Z., Braidy N. (2021). Antioxidant, antimicrobial and neuroprotective effects of Octaviania asterosperma in vitro. Mycology 12, 128–138. doi: 10.1080/21501203.2020.1816584
Sezer Y.Ç., Süfer Ö., Sezer G. (2017). Extraction of phenolic compounds from oven and microwave dried mushrooms (Agaricus bisporus and Pleurotus ostreatus) by using methanol, ethanol and aceton as solvents. Indian J. Pharm. Educ. Res. 51, S393–S397. doi: 10.5530/ijper
Sharma H. N., Catrett J., Nwokeocha O. D., Boersma M., Miller M. E., Napier A., et al. (2023). Anti-Toxoplasma gondii activity of Trametes versicolor (Turkey tail) mushroom extract. Sci. Rep. 13, 8667. doi: 10.1038/s41598-023-35676-6
Shi S., Yin L., Shen X., Dai Y., Wang J., Yin D., et al. (2022). β-Glucans from Trametes versicolor (L.) Lloyd is effective for prevention of influenza virus infection. Viruses 14, 237. doi: 10.3390/v14020237
Shomali N., Onar O., Alkan T., Demirtaş N., Akata I., Yildirim Ö. (2019). Investigation of the polyphenol composition, biological activities, and detoxification properties of some medicinal mushrooms from Turkey. Turk. J. Pharm. Sci. 16, 155–160. doi: 10.4274/tjps
Stastny J., Marsik P., Tauchen J., Bozik M., Mascellani A., Havlik J., et al. (2022). Antioxidant and anti-inflammatory activity of five medicinal mushrooms of the genus Pleurotus. Antioxidants 11, 1569. doi: 10.3390/antiox11081569
Sun X., Sun Y., Zhang Q., Zhang H., Yang B., Wang Z., et al. (2014). Screening and comparison of antioxidant activities of polysaccharides from Coriolus versicolor. Int. J. Biol. macromol. 69, 12–19. doi: 10.1016/j.ijbiomac.2014.05.027
Tang Y. J., Zhu L. W., Li H. M., Li D. S. (2007). Submerged culture of mushrooms in bioreactors-challenges, current state-of-the-art, and future prospects. Food Technol. Biotechnol. 45, 221–229.
Toledo R. C. C., Carvalho M. A., Lima L. C. O., de Barros Vilas-Boas E. V., Dias E. S. (2013). Measurement of β-glucan and other nutritional characteristics in distinct strains of Agaricus subrufescens mushrooms. Afr. J. Biotechnol. 12, 6203–6209. doi: 10.5897/AJB
Vaithanomsat P., Boonlum N., Chaiyana W., Tima S., Anuchapreeda S., Trakunjae C., et al. (2022). Mushroom β-glucan recovered from antler-type fruiting body of ganoderma lucidum by enzymatic process and its potential biological activities for cosmeceutical applications. Polymers 14, 4202. doi: 10.3390/polym14194202
Valverde M. E., Hernández-Pérez T., Paredes-López O. (2015). Edible mushrooms: improving human health and promoting quality life. Int. J. Microbiol. 2015, 14. doi: 10.1155/2015/376387
Wandati T. W., Kenji G. M., Onguso J. M. (2013). Phytochemicals in edible wild mushrooms from selected areas in Kenya. J. Food Res. 2, 137. doi: 10.5539/jfr.v2n3p137
Wang K. L., Lu Z. M., Mao X., Chen L., Gong J. S., Ren Y., et al. (2019). Structural characterization and anti-alcoholic liver injury activity of a polysaccharide from Coriolus versicolor mycelia. Int. J. Biol. macromol. 137, 1102–1111. doi: 10.1016/j.ijbiomac.2019.06.242
Wasser S. (2014). Medicinal mushroom science: Current perspectives, advances, evidences, and challenges. Biomed. J. 37. doi: 10.4103/2319-4170.138318
Yadav D., Negi P. S. (2021). Bioactive components of mushrooms: Processing effects and health benefits. Food Res. Int. 148, 110599. doi: 10.1016/j.foodres.2021.110599
Yang Q. Y., Jong S. C., Li X. Y., Zhou J. X., Chen R. T., Xu L. Z. (1992). Antitumor and immunomodulating activities of the polysaccharide-peptide (PSP) of coriolus-versicolor. Eos-Rivista di Immunol. Ed Immunofarmacol. 12, 29–34.
Keywords: Trametes sp., β-glucan, phytochemicals, flavonoid, phenol, saponin
Citation: Srivastava M, Kumari M, Karn SK, Bhambri A, Mahale VG and Mahale S (2024) Submerged cultivation and phytochemical analysis of medicinal mushrooms (Trametes sp.). Front. Fungal Biol. 5:1414349. doi: 10.3389/ffunb.2024.1414349
Received: 10 April 2024; Accepted: 14 May 2024;
Published: 11 June 2024.
Edited by:
Mustafa Sevindik, Osmaniye Korkut Ata University, TürkiyeReviewed by:
Zeliha Selamoglu, Niğde Ömer Halisdemir University, TürkiyeCopyright © 2024 Srivastava, Kumari, Karn, Bhambri, Mahale and Mahale. This is an open-access article distributed under the terms of the Creative Commons Attribution License (CC BY). The use, distribution or reproduction in other forums is permitted, provided the original author(s) and the copyright owner(s) are credited and that the original publication in this journal is cited, in accordance with accepted academic practice. No use, distribution or reproduction is permitted which does not comply with these terms.
*Correspondence: Santosh Kumar Karn, c2FudG9zaGthcm5AZ21haWwuY29t
Disclaimer: All claims expressed in this article are solely those of the authors and do not necessarily represent those of their affiliated organizations, or those of the publisher, the editors and the reviewers. Any product that may be evaluated in this article or claim that may be made by its manufacturer is not guaranteed or endorsed by the publisher.
Research integrity at Frontiers
Learn more about the work of our research integrity team to safeguard the quality of each article we publish.