- 1Department of Bioscience and Biotechnology, Banasthali Vidyapith, Rajasthan, India
- 2Department of Botany, Government Adarsh Girls College Sheopur, Madhya Pradesh, India
The complex and dynamic interactions between fungi and plants constitute a critical arena in ecological science. In this comprehensive review paper, we explore the multifaceted relationships at the fungi-plant interface, encompassing both mutualistic and antagonistic interactions, and the environmental factors influencing these associations. Mutualistic associations, notably mycorrhizal relationships, play a pivotal role in enhancing plant health and ecological balance. On the contrary, fungal diseases pose a significant threat to plant health, agriculture, and natural ecosystems, such as rusts, smuts, powdery mildews, downy mildews, and wilts, which can cause extensive damage and lead to substantial economic losses. Environmental constraints encompassing abiotic and biotic factors are elucidated to understand their role in shaping the fungi-plant interface. Temperature, moisture, and soil conditions, along with the presence of other microbes, herbivores, and competing plants, significantly influence the outcome of these interactions. The interplay between mutualism and antagonism is emphasised as a key determinant of ecosystem health and stability. The implications of these interactions extend to overall ecosystem productivity, agriculture, and conservation efforts. The potential applications of this knowledge in bioremediation, biotechnology, and biocontrol strategies emphasise the importance of adapting to climate change. However, challenges and future directions in this field include the impacts of climate change, emerging fungal pathogens, genomic insights, and the role of the fungi-plant interface in restoration ecology. Hence, this review paper provides a comprehensive overview of fungi-plant interactions, their environmental influences, and their applications in agriculture, conservation, and ecological restoration.
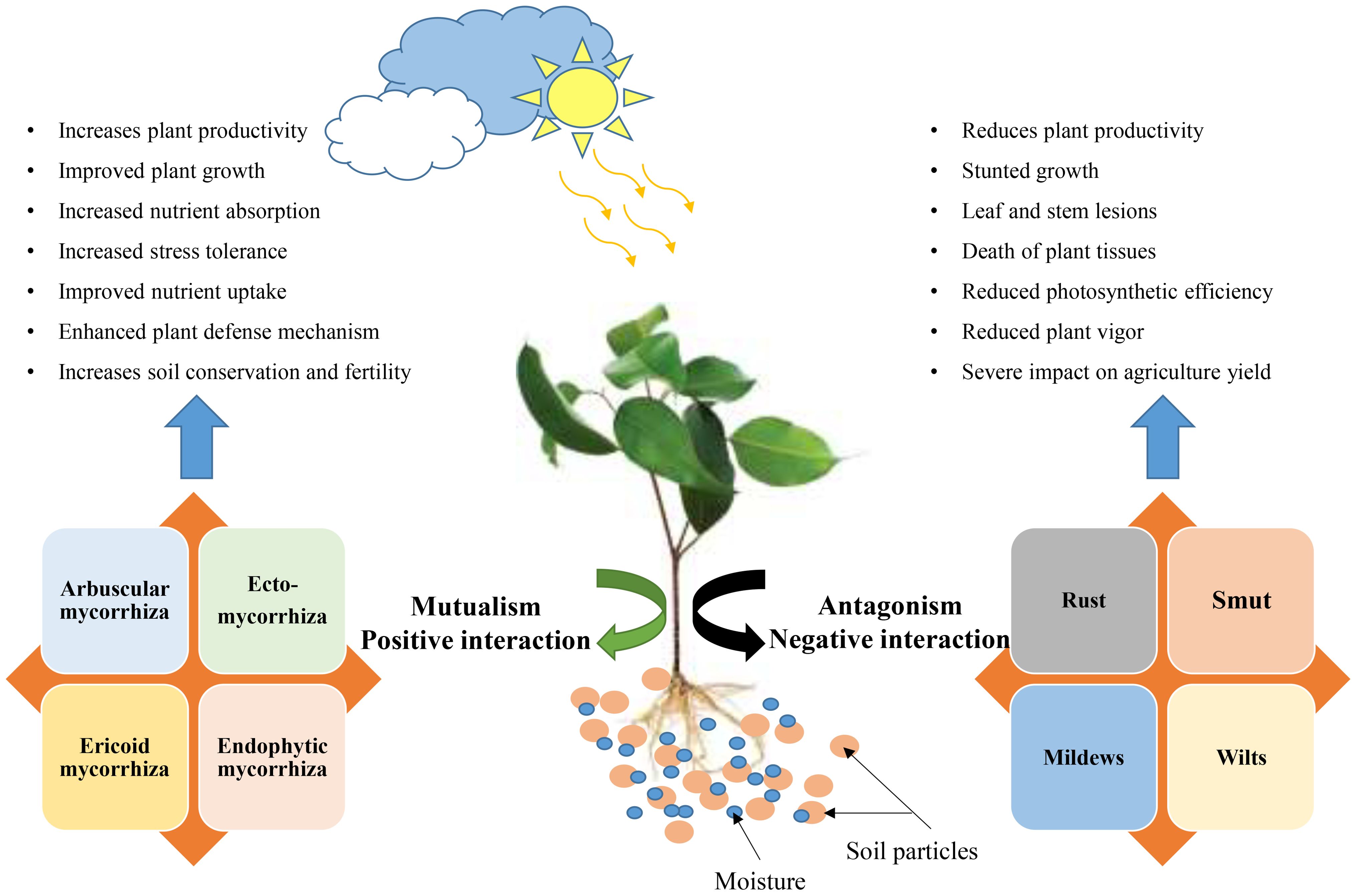
Graphical Abstract. Symbiotic and pathogenic interactions at the fungi-plant interface under environmental constraints (Chang et al., 2018).
1 Introduction
The intricate and ever-evolving interplay between fungi and plants is a pivotal and dynamic aspect of ecological systems, where a multitude of relationships are characterised by both mutualistic and antagonistic interactions (Zeilinger et al., 2016; Balestrini, 2021; Priyashantha et al., 2023). These interactions bear profound repercussions for the vitality and adaptability of plant life, as well as for the ecological equilibrium in diverse ecosystems (Zeilinger et al., 2016; Balestrini, 2021; Figueiredo et al., 2021; Priyashantha et al., 2023), as discussed in Figure 1. In this review, we embark on a journey into the multitude of domains of the fungi-plant interface, meticulously delving into the intricate mechanisms and ecological implications that underlie these interactions.
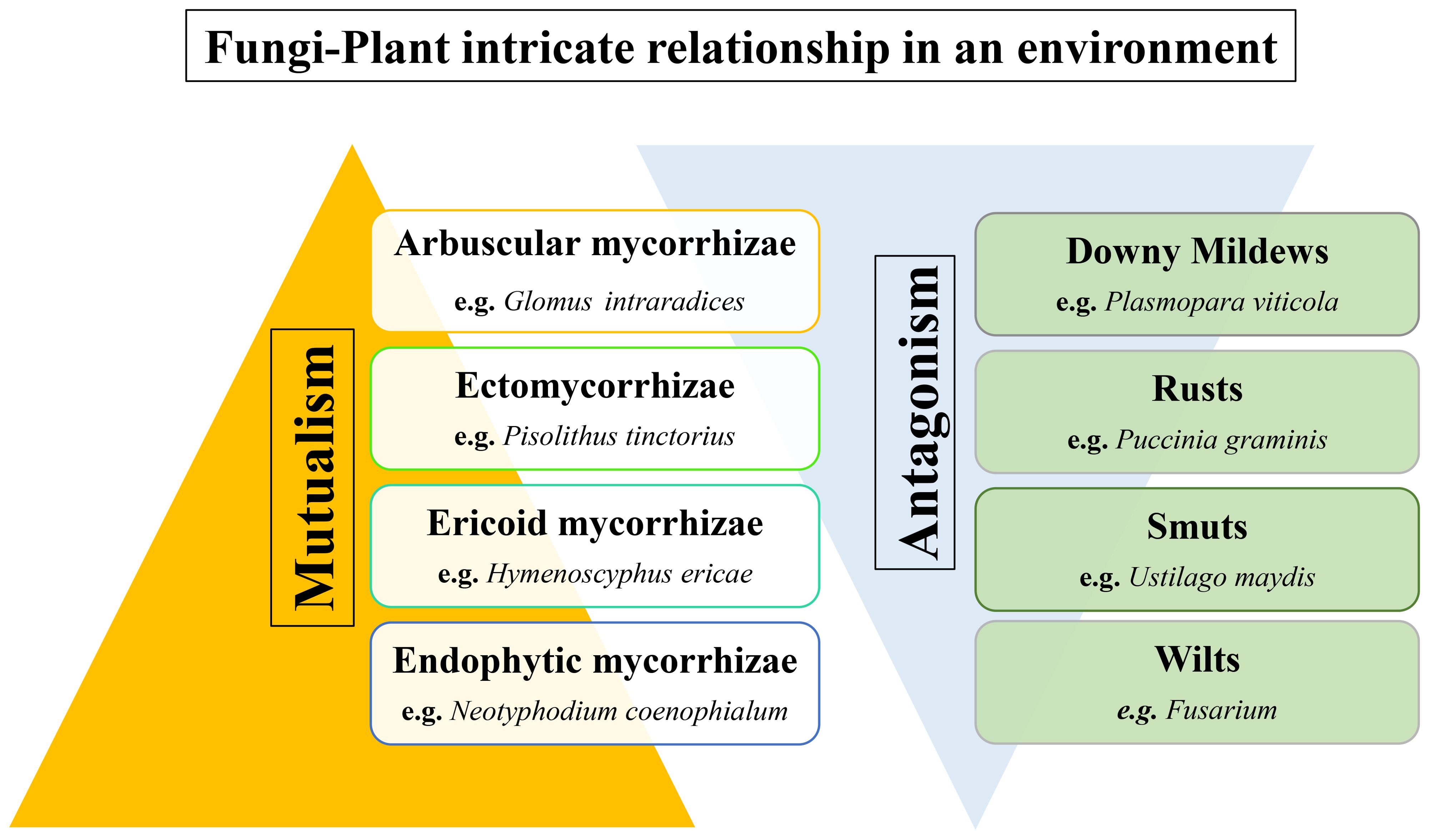
Figure 1. Fungi-plant intricate relationship showing both mutualistic and antagonistic behaviour on plant health.
Mycorrhizal symbiosis, in particular, has garnered significant attention from researchers. In this mutualistic relationship, certain fungi, predominantly arbuscular mycorrhizal fungi and ectomycorrhizal fungi, form intricate associations with plant roots. These fungi enhance the plant’s nutrient uptake, particularly phosphorus and nitrogen, by expanding the root’s absorptive surface area through the formation of mycorrhizal network structures (Lanfranco et al., 2016; Begum et al., 2019; Shi et al., 2023). In return, plants offer a steady supply of carbon compounds in the form of sugars to their fungal partners. This mutualistic exchange of resources enhances plant growth, nutrient acquisition, and stress tolerance, thereby promoting plant fitness (Stuart and Plett, 2020; Priyashantha et al., 2023).
However, not all interactions between fungi and plants are always beneficial. Antagonistic relationships in the form of pathogenic interactions often challenge the vitality of plants (Frey-Klett et al., 2011; Deveau et al., 2018; Balestrini, 2021). Many fungal species, such as rusts, smuts, and mildews, have evolved strategies to infiltrate plant tissues and exploit them as hosts. The consequences of such interactions can be detrimental, leading to reduced plant growth, low crop yield or production, and extensive ecological disruptions (Zeilinger et al., 2016; Peng et al., 2021; Mapuranga et al., 2022). Understanding the mechanisms that underpin fungi-plant pathogenesis is effective in devising strategies to mitigate the impact of such antagonistic relationships (Selin et al., 2016; Adnan et al., 2019; Dutta et al., 2023).
The modulation of these complex interactions is influenced by multiple factors. Environmental conditions such as temperature, humidity, and soil chemistry play a pivotal role in shaping the outcome of these associations (Canarini et al., 2019; Abdul Rahman et al., 2021; Rodrigues et al., 2023). Furthermore, plant genetic factors, including resistance genes, also contribute to the plant’s response to fungal partners, whether mutualistic or pathogenic. Recent research also highlighted the role of plant microinteractions in shaping the outcomes influencing fungal colonisation and plant health.
The broader implications of these interactions extend far beyond the fungus-plant interface. They have profound consequences for ecosystem dynamics. Mycorrhizal fungi not only benefit individual plants but can also influence the composition and structure of entire plant communities (Bonfante and Genre, 2010; Chen et al., 2018; Wahab et al., 2023). Furthermore, mycorrhizal networks can facilitate the transfer of nutrients between plants, connecting neighbouring individuals in complex underground exchanges.
However, the actions of pathogenic fungi can destabilise ecosystems by affecting the health and survival of key plant species, cascading through the food web, and potentially causing shifts in community composition (Gorzelak et al., 2015; Figueiredo et al., 2021).
The interactions between fungi and plants represent a captivating and intricate tapestry of mutualistic and antagonistic relationships. These associations are governed by an interplay of environmental, genetic, and microbial factors, with far-reaching consequences for plant health and ecosystem dynamics (Zeilinger et al., 2016; Alam et al., 2021; Bastías Campos et al., 2023; Priyashantha et al., 2023). With a comprehensive understanding of the mechanisms and ecological implications of these interactions, we gain valuable insights into the functioning of our natural world and pave the way for more informed strategies for the management and preservation of ecosystems. In this review, we are exploring the fungi-plant interplay both in terms of mutualism and antagonism.
2 Mutualistic associations
Mycorrhizal relationships are among the most intriguing and ecologically significant mutualistic associations in the complex realm of the fungi-plant interface. Mycorrhizal fungi encompass a diverse array of taxa and form mutually beneficial symbiotic partnerships with an astonishing majority of plant species, spanning the evolutionary spectrum from humble liverworts to advanced angiosperms, thus underscoring their ubiquity and central role in shaping plant life (Lanfranco et al., 2016; Chen et al., 2018; Huey et al., 2020; Wahab et al., 2023). They play a pivotal role in bolstering nutrient acquisition and overall plant fitness, as discussed in Table 1. These associations have evolved into several distinct types, each finely tuned to the specific requirements and ecological niches of different plant species and environments (Huey et al., 2020; Shi et al., 2023).
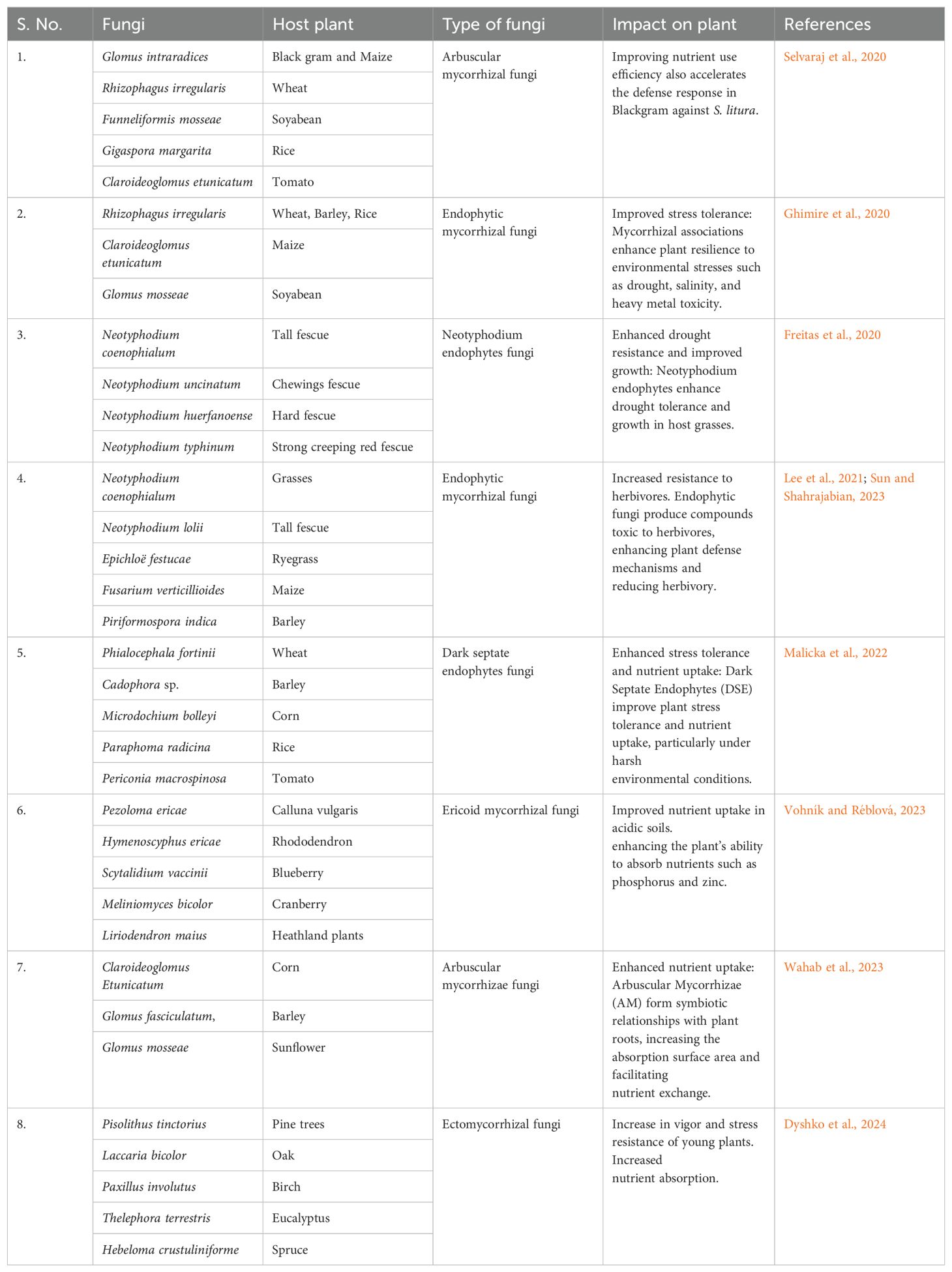
Table 1. Impact of mutualistic association of fungi-plant on the holistic growth of plant and improves stress management.
Arbuscular mycorrhiza represents one of the most widespread and ancient forms of mycorrhizal associations. These symbiotic partnerships primarily involve fungi from the Glomeromycota phylum. Arbuscular mycorrhizal fungi (AMF), such as Rhizophagus intraradices, Glomus mosseae, Funneliformis geosporum, and Claroideoglomus etunicatum, form intricate networks within the root cells of plants, extending their hyphal structures into the surrounding soil (Berruti et al., 2016; Chen et al., 2018). This architecture dramatically increases the effective surface area for nutrient uptake of essential elements like phosphorus and nitrogen, which are frequently limiting factors in plant growth (Giovannini et al., 2020; Mondal et al., 2022; Demir et al., 2022; Wahab et al., 2023). Moreover, the formation of arbuscules within plant root cells enables the efficient exchange of nutrients between the plant and the fungus, exemplifying the intricate nature of this association (Strack et al., 2003; Begum et al., 2019).
Ectomycorrhizal relationships predominantly involve fungi from the Basidiomycota and Ascomycota groups. These mycorrhizal associations entail the formation of a sheath around the tips of plant roots, contributing to nutrient absorption and enhancing plant resistance to multiple stresses (Tibbett and Sanders, 2002; Agerer, 2006; Gil-Martínez et al., 2018). Ectomycorrhizal fungi contribute significantly to forest ecosystems as they are frequently associated with woody plant species, including many tree species. Examples of ectomycorrhizal fungi include Pisolithus tinctorius, Amanita muscaria, Laccaria bicolor, and Suillus luteus. By facilitating nutrient uptake and conferring protection against diseases and environmental pressures, ectomycorrhizal associations become integral to the health and vitality of these long-lived and ecologically influential plant species (Itoo and Reshi, 2013; Policelli et al., 2020; Liu et al., 2020).
Ericoid mycorrhizae constitute another unique variant of mycorrhizal association and are commonly found in plants like heathers and other ericaceous species. The fungi involved in ericoid mycorrhizal associations predominantly belong to the Ascomycota and Basidiomycota groups (Perotto et al., 2018; Fehrer et al., 2019; Vohník, 2020). These fungi assist plants in accessing essential nutrients, particularly in acidic, nutrient-poor soils where other nutrient acquisition strategies may prove insufficient (Cairney and Meharg, 2003; Wei et al., 2022). The fact that ericoid mycorrhizal fungi such as Rhodotorula mucilaginosa, Oidiodendron maius, Meliniomyces variabilis, and Hymenoscyphus ericae have the ability to break down complex organic compounds in such adverse environments showcases their importance in plant adaptation to challenging ecological niches.
Moving beyond endophytic associations represents another fascinating facet of mutualistic interactions between fungi and plants. Endophytic fungi take up residence within plant tissues, inhabiting the plant’s interior without causing apparent harm. Instead, they offer an array of benefits to their host, including increased resistance to herbivores and pathogens, improved tolerance to abiotic stress, and enhanced nutrient uptake (Alam et al., 2021; Chaudhary et al., 2022; Akram et al., 2023). Endophytic associations involve Neotyphodium endophytes and grasses, particularly within the Poaceae family. These endophytes produce alkaloids that deter herbivores and confer a competitive advantage to the host plant (Malinowski and Belesky, 2006; Rasmussen et al., 2008; Caradus and Johnson, 2020). The presence of Neotyphodium endophytes can significantly enhance the survival and vigour of grasses, which has substantial implications for both natural ecosystems and agriculture (Fadiji and Babalola, 2020).
Dark-septate endophytes (DSE) are characterised by their diverse presence in various plant species. They often enhance plant stress tolerance and nutrient uptake, making them indispensable for plant adaptation to adverse environmental conditions (Hou et al., 2020; Farias et al., 2020; Malicka et al., 2022). Porras-Alfaro and Bayman (2011) highlighted the role of DSE in helping plants thrive in high-stress environments, including those with high salinity and heavy metal contamination, such as Phialophora fortinii, Cadophora finlandica, Darksidea sp., and Periconia macrospinosa. These fungi have demonstrated significant potential for supporting plant growth and resilience in challenging conditions, further emphasising their ecological importance.
3 Antagonistic interactions
Within the intricate tapestry of the fungi-plant interface, there exists a darker side characterised by antagonistic interactions between fungi and plants, as discussed in Table 2. This facet of the relationship involves the capacity of fungal pathogens to inflict devastating diseases upon their plant hosts. Fungal infections have profound consequences for plant health, agricultural production, and the equilibrium of natural ecosystems (Zeilinger et al., 2016; Peng et al., 2021; Priyashantha et al., 2023). Rusts and smuts are formidable adversaries belonging to the Pucciniales and Ustilaginales orders, respectively (Zuo et al., 2019; Thambugala et al., 2020; Peng et al., 2021). They inflict diseases on numerous crop plants, including staple grains such as wheat, barley, and oats. The terms “rust” and “smut” aptly describe the characteristic appearances of these diseases on plant surfaces, where they manifest as conspicuous, powdery lesions or dark, sooty pustules (Doehlemann et al., 2017; Zuo et al., 2019). These diseases are renowned for their yield losses, making them subjects of intensive study and management efforts in agriculture.
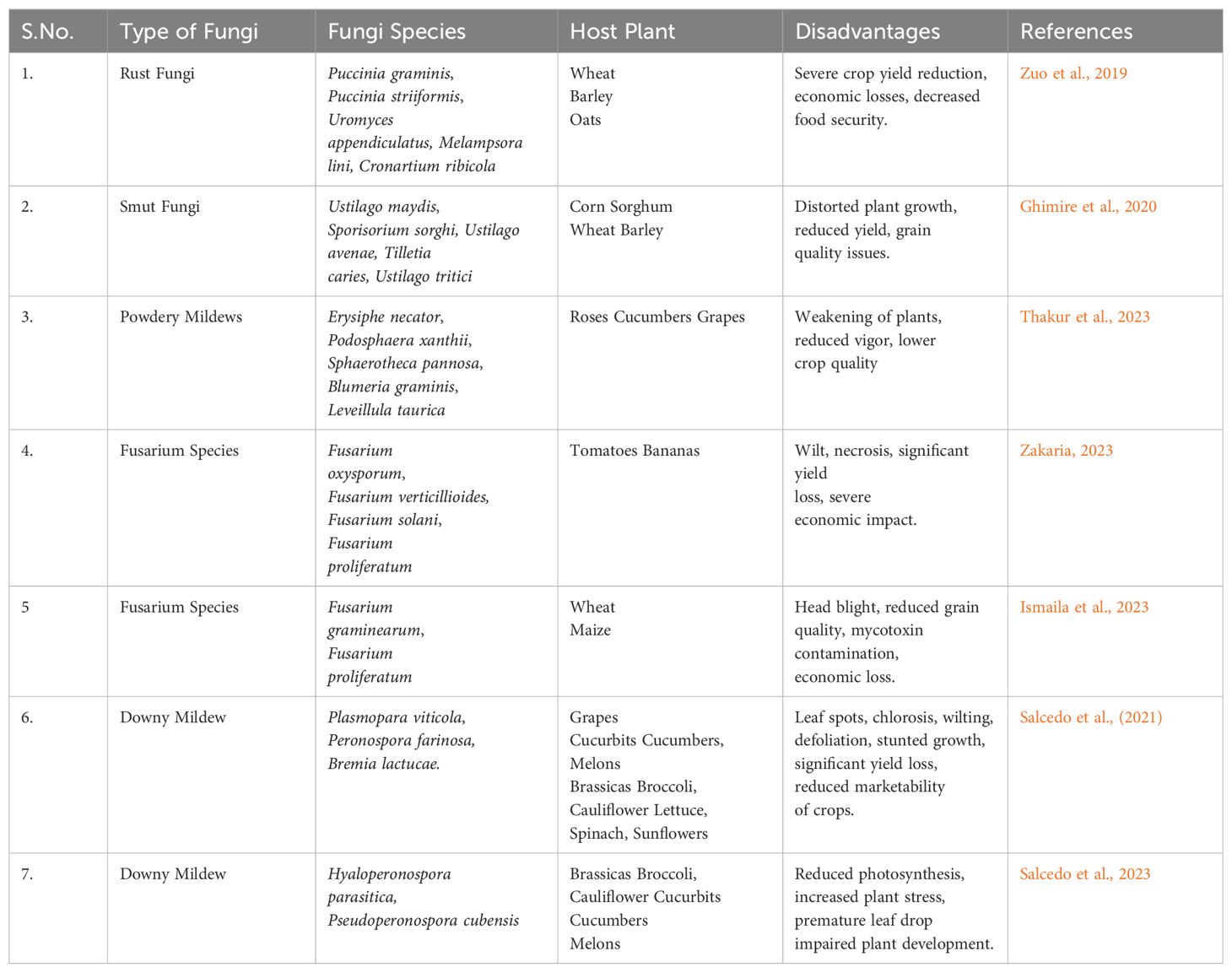
Table 2. Impact of antagonistic interaction between fungi-plant on the overall growth of plant species and productivity.
Powdery mildews, primarily originating from the Erysiphales order, are formidable fungal pathogens with a broad host range, infecting various plant species and targeting different parts such as leaves, stems, and flowers. The distinctive powdery white colonies on plant surfaces mark their presence (Micali et al., 2008; Kiss et al., 2020; Yeh et al., 2021). Although these fungi do not always cause catastrophic damage, they can weaken plants and reduce the quality of agricultural and horticultural products. For instance, Erysiphe cichoracearum affects many cucurbits, including cucumbers and melons, leading to significant yield losses (McGrath et al., 2001). Blumeria graminis is known for its impact on cereal crops like wheat and barley, causing severe reductions in crop yield and quality (Troch et al., 2014; Basandrai et al., 2023). Podosphaera leucotricha commonly infects apple and pear trees, affecting both leaves and fruits, resulting in economic losses in fruit production (Heidenreich and Turechek, 2016; Whitehead et al., 2021). Additionally, Golovinomyces orontii targets a wide range of ornamental plants and vegetables, including members of the Asteraceae family, such as lettuce and sunflower (Prahl et al., 2023). These examples highlight the diverse impact of powdery mildew fungi on agricultural and horticultural plants, emphasising the need for effective management strategies to mitigate their effects.
Downy mildews, belonging to the oomycetes group, represent a significant threat to agriculture due to their devastating impact on various crops, including grapes and potatoes. These pathogens are notorious for their ability to rapidly spread and infect entire harvests. They produce sporangia that can be easily dispersed by wind and water, facilitating widespread infection of new plant hosts (Thakur and Mathur, 2002; Salcedo et al., 2021). For example, Plasmopara viticola, the causative agent of grapevine downy mildew, can lead to severe yield losses in vineyards if not properly managed (Gessler et al., 2011; Puelles et al., 2024). Phytophthora infestans, responsible for potato late blight, has historically caused catastrophic famines and continues to pose a major challenge to potato production worldwide (Haverkort et al., 2009). Peronospora destructor targets onion crops, significantly affecting bulb quality and yield (Schwartz and Mohan, 2008; Zhou, 2023). Additionally, Bremia lactucae is known to infect lettuce, leading to substantial losses in both field and greenhouse settings (Lebeda et al., 2008; Macioszek et al., 2023). The extensive damage caused by downy mildew necessitates vigilant monitoring and effective management practices to mitigate their impact on agriculture.
Fusarium wilt, caused by various Fusarium species, is yet another formidable fungal disease that strikes at the heart of agriculture. This disease targets a range of economically significant crops, including tomatoes, bananas, and cotton (Dita et al., 2018; De Lamo and Takken, 2020; Duvnjak et al., 2023; Zakaria, 2023). Fusarium wilt is characterised by the wilting and death of affected plants (Ismaila et al., 2023). The Fusarium fungi often enter plants through the roots, where they disrupt water and nutrient transport systems, leading to the characteristic wilting and necrosis of plant tissues (Dita et al., 2018; Ismaila et al., 2023; Zakaria, 2023).
Antagonistic fungal interactions with plants have significant ecological and economic ramifications. They necessitate a multifaceted approach to disease management, including cultural practices, resistant plant varieties, and, in some cases, chemical control measures (Thambugala et al., 2020; He et al., 2021). Understanding the molecular and ecological intricacies of these interactions is vital for the development of more effective and sustainable disease management strategies. Furthermore, these interactions underscore the need for ongoing research to combat the ever-present threat of fungal pathogens to global agriculture and natural ecosystems.
4 Effect of enviornment on plant fungi interaction
The versatile interconnection dynamic interplay between mutualistic and antagonistic interactions within the fungi-plant interface is far from static; it is profoundly influenced by a myriad of environmental factors that dictate the outcome of these associations, as shown in Figure 2. A comprehensive understanding of these environmental constraints is pivotal for predicting, managing, and harnessing these interactions effectively for the benefit of ecosystems and agriculture (Singh et al., 2023; Priyashantha et al., 2023). The environmental constraints are basically divided into two categories biotic and abiotic stress.
4.1 Abiotic factors
4.1.1 Temperature
Temperature significantly influences the fungi-plant interface, affecting both mutualistic and antagonistic interactions. Temperature fluctuations impact plant susceptibility to fungal infections and the growth patterns of fungal pathogens, determining disease severity (Singh et al., 2023; Priyashantha et al., 2023). Mycorrhizal associations are also temperature-sensitive, affecting their effectiveness in various conditions. It was reported that Glomus intraradices perform optimally at 20–25°C, enhancing nutrient uptake and plant growth, but its efficiency decreases at higher temperatures (Tian et al., 2023). Botrytis cinerea, a pathogen causing grey mould, shows increased virulence at 15–20°C, making cooler climates more prone to outbreaks (Bika et al., 2021). Piriformospora indica promotes plant growth and stress resistance best at 25–30°C, with reduced benefits in cooler conditions necessitating temperature consideration in agricultural use (Li et al., 2023).
4.1.2 Moisture
Soil moisture levels are crucial for mycorrhizal relationships and fungal pathogen activity. Arbuscular mycorrhizal fungi are less moisture-dependent but still affected, while ectomycorrhizal fungi are highly sensitive to soil water content. Moreover, moisture influences fungal spore germination, growth, and pathogenicity (Begum et al., 2019; Wahab et al., 2023). For example, the ectomycorrhizal fungus Pisolithus tinctorius requires high soil moisture for optimal symbiosis with pines (Dyshko et al., 2024). Rhizophagus irregularis, an arbuscular mycorrhizal fungus, thrives best in moderate moisture conditions but is adaptable to a variety of other environments (Wahab et al., 2023). The pathogen Phytophthora infestans, causing potato blight, thrives in moist conditions, enhancing spore germination and infection (Ivanov et al., 2021). Fusarium oxysporum, a pathogen that causes wilt, depends on sufficient soil moisture for its spores to germinate and spread (Purohit et al., 2023).
4.1.3 Soil conditions
Soil characteristics, including pH, nutrient availability, and organic matter content, significantly influence the type and effectiveness of mycorrhizal associations (Huey et al., 2020). Some mycorrhizal fungi exhibit adaptations to specific soil conditions, such as ericoid mycorrhizae thriving in acidic soils (Huey et al., 2020; Wahab et al., 2023). Soil pH can dramatically affect the availability of essential nutrients like phosphorus, impacting the benefits of mycorrhizal associations. Fungal pathogens also exhibit preferences for particular soil types, affecting antagonistic interactions (Begum et al., 2019). For example, ericoid mycorrhizae such as Rhizoscyphus ericae thrive in acidic soils, aiding plants in nutrient-poor conditions (Yang et al., 2023). The arbuscular mycorrhizal fungus Glomus mosseae performs well in neutral to slightly acidic soils, enhancing phosphorus uptake (Wahab et al., 2023; Ding et al., 2024). The pathogen Verticillium dahliae, causing wilt, prefers alkaline soils, where it becomes more virulent (Zhang et al., 2024). The beneficial fungus Pseudomonas fluorescens, which can suppress soil-borne pathogens, shows enhanced activity in soils with high organic matter (Chen et al., 2023).
4.2 Biotic factors
4.2.1 Microbes
In the soil ecosystem, various microbes interact with symbiotic and antagonistic fungi, impacting mycorrhizal associations differently. Bacteria like Pseudomonas spp. may compete for resources, while Bacillus sp. enhances plant growth. Rhizobium sp. influences mycorrhizal colonisation. Some fungi, like Trichoderma sp. and Gliocladium sp., inhibit mycorrhizal growth, while others, like Penicillium sp., either compete or cooperate. Protozoa, such as Amoeba sp., graze on mycorrhizal fungi. Methanotrophic archaea affect soil microbial communities. Mycoviruses infect fungi, altering their competitiveness. Understanding these interactions is crucial for comprehending the intricate web of relationships in soil ecosystems (Netherway et al., 2021; Santoyo et al., 2021). For example, Pseudomonas fluorescens competes with mycorrhizal fungi for nutrients, potentially reducing their effectiveness. Bacillus subtilis enhances plant growth and can promote mycorrhizal colonisation by producing beneficial compounds (Kulkova et al., 2023). Rhizobium leguminosarum positively influences mycorrhizal colonisation by improving nutrient availability through nitrogen fixation (Dhiman et al., 2024). Trichoderma harzianum inhibits mycorrhizal fungi through the production of antifungal compounds (Rahman et al., 2023). Penicillium bilaii can either compete with or assist mycorrhizal fungi in phosphate solubilisation, depending on environmental conditions (Suraby et al., 2023).
4.2.2 Herbivores
Herbivores such as insects and mammals indirectly impact the fungi-plant interface by damaging plant tissues and creating entry points for fungal pathogens. This damage can render plants more susceptible to infections, particularly by fungal pathogens that exploit wounds or weaken plant defenses. Conversely, certain endophytic fungi residing within plant tissues produce compounds that deter herbivores from feeding, thus indirectly protecting their host plants (Lu et al., 2021; Chaudhary et al., 2022). These interactions highlight the interconnectedness of different biotic factors and their repercussions on the fungi-plant interface. For example, insect herbivory by caterpillars on leaves creates wounds that can be exploited by the fungal pathogen Botrytis cinerea, leading to increased infection rates (Ngah et al., 2024). Mammalian herbivores, such as deer, can damage bark and stems, providing entry points for fungal pathogens like Armillaria spp., which cause root rot (Kranjec Orlović et al., 2024). Aphid feeding can weaken plant defences, making them more susceptible to fungal infections like Verticillium dahliae, which cause wilt (Sun et al., 2023). Endophytic fungi such as Neotyphodium sp. produce alkaloids that deter herbivores like grasshoppers, thereby protecting their host plants (Lin et al., 2024). The endophytic fungus Piriformospora indica enhances plant resistance to herbivores by inducing systemic defence responses, reducing damage from pests like aphids (Adedayo and Babalola, 2023).
4.2.3 Competing plants
The presence of other plant species can profoundly affect the fungi-plant interface. In the context of mycorrhizal fungi, competition for resources in the rhizosphere significantly influences the distribution and effectiveness of these mutualistic associations. Some plants have stronger mycorrhizal associations, leading to disparities in nutrient acquisition and competitive advantage (Figueiredo et al., 2021; Wahab et al., 2023). These dynamics impact plant community composition and ecosystem function. For example, legumes like Trifolium repens (white clover) often form strong mycorrhizal associations, enhancing their nutrient uptake and competitiveness (Tello-García et al., 2023). Grasses such as Lolium perenne (perennial ryegrass) can outcompete other plants by efficiently utilising mycorrhizal networks to access soil nutrients (Sudharsan et al., 2023). Helianthus annuus (sunflower) may dominate in nutrient-poor soils due to its effective mycorrhizal associations, giving it a competitive edge over less mycorrhizal-dependent species (Wahab et al., 2023). Invasive species like Ailanthus altissima (the tree of heaven) can disrupt local mycorrhizal networks, negatively impacting native plant species that rely on these fungi (Raspor et al., 2023). Betula pendula (silver birch) forms strong ectomycorrhizal associations, allowing it to compete effectively in mixed forests by accessing deep soil nutrients.
The interactions within the fungi-plant interface are intricately woven into the fabric of the natural world, and they are highly contingent upon a multitude of abiotic and biotic factors. Understanding these environmental constraints is essential for predicting the outcomes of mutualistic and antagonistic associations, enabling more effective management strategies, and shedding light on the delicate balance that governs ecological and agricultural systems (Singh et al., 2011; Balestrini, 2021). These insights are crucial for mitigating the impact of fungal diseases in agriculture and harnessing the benefits of mycorrhizal symbiosis.
5 The significance and challenges of fungus-plant interaction in various fields
The fungi-plant interface represents a dynamic and ever-evolving realm where mutualistic and antagonistic interactions are perpetually at play. These interactions are emblematic of the intricate web of life that characterises the natural world. As we delve deeper into the intricacies of these associations, it becomes increasingly apparent that the equilibrium between mutualism and antagonism is a backbone in determining the health and stability of ecosystems, as shown in Figure 3 (Zeilinger et al., 2016; Mayer et al., 2023).
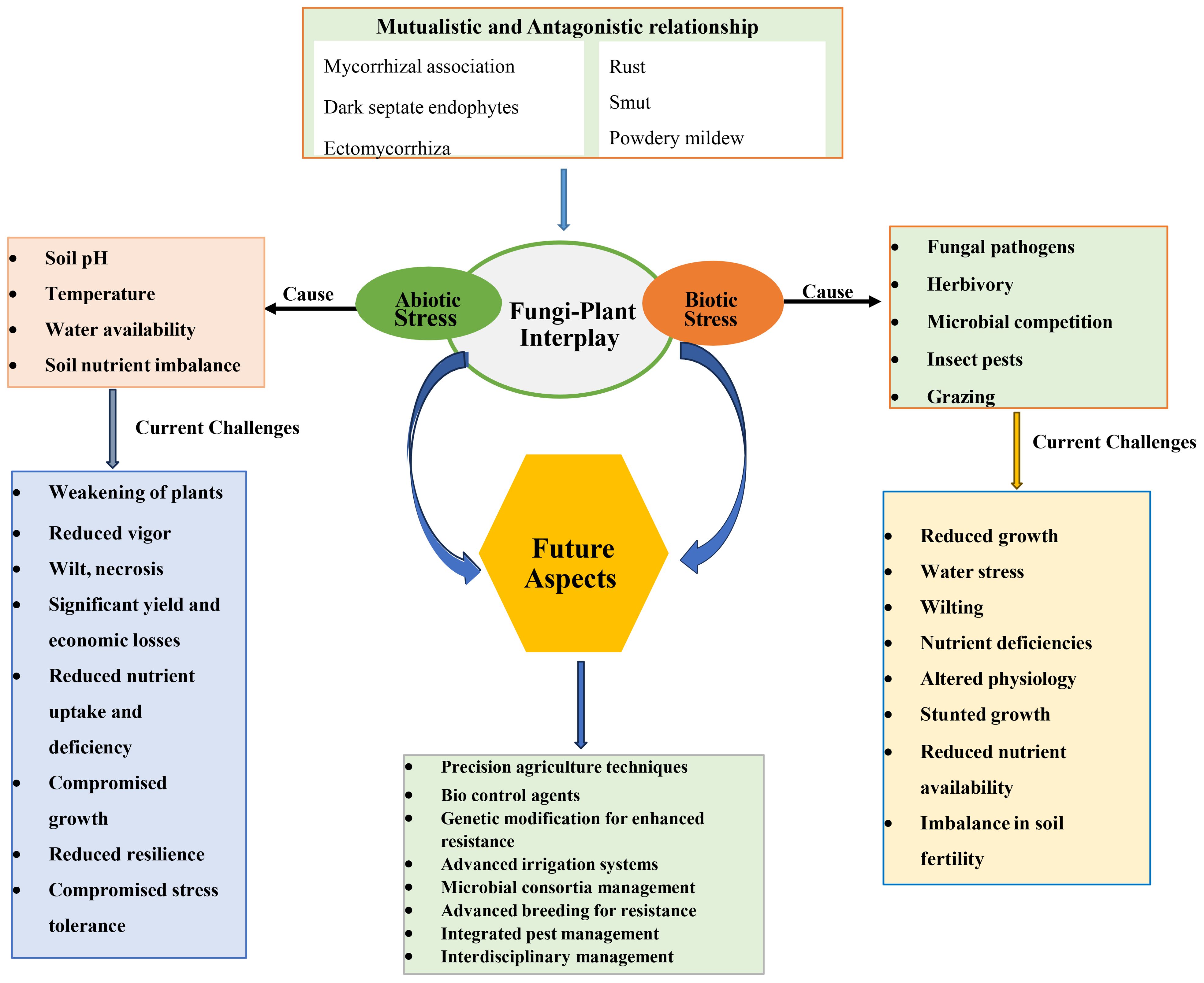
Figure 3. A holistic overview of fungi-plant interaction under environmental constraints, challenges and future aspects.
5.1 Consequences for the ecosystem
Healthy mycorrhizal associations play a pivotal role in enhancing the overall productivity of ecosystems. They enhance nutrient cycling by improving the acquisition of vital elements, such as phosphorus and nitrogen. This nutrient cycling helps many plant communities grow and survive, not just individual plants (Begum et al., 2019; Huey et al., 2020; Priyashantha et al., 2023). These intricate networks of cooperation beneath the soil surface not only nourish individual plants but also facilitate the transfer of nutrients and signalling compounds between neighbouring plants. This interconnection influences the composition and structure of entire plant communities and has a cascading effect on the ecological balance of ecosystems (Yu et al., 2022; Shi et al., 2023).
On the contrary, outbreaks of fungal diseases can have devastating consequences for plant populations and the intricate food chains that depend on them. Such diseases can lead to a decline in plant species, reducing food resources for herbivores and, in turn, impacting their predators. These disruptions can reverberate through the entire ecosystem, leading to imbalances in predator-prey relationships and ultimately affecting ecosystem stability (Godfray et al., 2016; Almeida et al., 2019; Priyashantha et al., 2023). The consequences of these interactions emphasise the intricate interdependency within natural systems and the critical role of the fungi-plant interface in shaping the health and resilience of ecosystems.
5.2 Agriculture
In the agricultural domain, comprehending and managing the fungus-plant interface holds significant value. Farmers and agronomists can leverage mycorrhizal associations to enhance crop yields and reduce dependence on chemical fertilisers. These symbiotic interactions substantially augment nutrient uptake in crops, fostering improved plant growth and development. By facilitating the acquisition of essential nutrients, such mutualistic associations contribute to sustainable farming practices (Berruti et al., 2016; Wahab et al., 2023).
At the same time, developing strategies to mitigate fungal pathogens’ impact on crops is pivotal for preserving crop yields. Fungal diseases can cause considerable yield losses and economic setbacks in agriculture. Management techniques typically involve fungicide use, breeding for disease resistance, and cultural practices. Achieving a balance between harnessing the benefits of mutualistic associations and safeguarding crops from antagonistic interactions remains an ongoing challenge in the agricultural sector (Wahab et al., 2023).
Understanding the fungi-plant interface has sparked innovative applications in biotechnology. Mycorrhizal inoculants, including species such as Rhizophagus irregularis, Funneliformis mosseae, and Gigaspora margarita, form symbiotic partnerships with soybean roots, enhancing nutrient uptake, particularly phosphorus (Wahab et al., 2023). Research confirms that these inoculants significantly increase soybean growth, nitrogen fixation, and nutrient uptake, leading to improved yield and quality (Leite et al., 2024). Synergistic effects with nitrogen-fixing bacteria such as Bradyrhizobium japonicum further enhance plant biomass and nitrogen content (Leite et al., 2024). Mixed inoculant formulations, incorporating multiple mycorrhizal fungi species, have demonstrated enhanced growth and stress tolerance in soybeans compared to single-species inoculants (Igiehon and Babalola, 2021). Additionally, mycorrhizal inoculants have shown promising results in various other crops. In maize cultivation, Rhizophagus irregularis has been found to improve nutrient uptake and increase plant growth. Similarly, in wheat, mycorrhizal inoculants enhance phosphorus acquisition and improve crop yield (Wu et al., 2022; Wahab et al., 2023). Moreover, in leguminous crops like common bean, mycorrhizal symbiosis enhances nitrogen fixation and promotes plant growth (Yu et al., 2024). These findings underscore the broad applicability and effectiveness of mycorrhizal inoculants across different crops, reinforcing their role in sustainable agriculture practices aimed at reducing chemical fertiliser dependence and improving overall crop productivity.
Mycorrhizal inoculants have undergone testing across various crop systems, yielding promising results. These formulations contain carefully selected mycorrhizal fungal species that establish beneficial associations with crops, enhancing access to vital nutrients like phosphorus and nitrogen. By bolstering nutrient uptake, these inoculants can amplify crop yields and elevate agricultural product quality (Berruti et al., 2016; Emmanuel and Babalola, 2020; Etesami et al., 2021). This approach resonates with precision agriculture principles, emphasising efficient input utilisation to optimise productivity while minimising environmental impact (Wahab et al., 2023).
5.3 Conservation
In the realm of conservation biology, maintaining the equilibrium between mutualistic and antagonistic interactions in natural ecosystems is crucial for the preservation of biodiversity. Many plant species rely on mycorrhizal relationships for their growth and survival. Disruptions in these associations can have far-reaching consequences, particularly for rare and endangered species. The ghost orchid (Dendrophylax lindenii), which is native to the nutrient-poor swamps of Florida and Cuba, depends heavily on its mycorrhizal associations with specific fungal partners to thrive. Mycorrhizal symbiosis can be especially vital for plants in nutrient-poor environments, where they depend on their fungal partners to survive (Begum et al., 2019; Li et al., 2021). Conserving the diversity of mycorrhizal fungi and understanding their relationships with plants is pivotal for the protection of plant species and the maintenance of ecological balance in natural ecosystems.
The equilibrium between symbiotic relationships is crucial for ecosystem health and stability, impacting organisms and trophic networks alike (Bahram and Netherway, 2022; Shi et al., 2023). In agriculture, it supports sustainable practices and crop resilience, while in conservation biology, it emphasises the need to protect trophic interdependencies. Studying and utilising the mycorrhizal-plant interface holds promise for ecological restoration and agricultural sustainability in a changing world.
A recent study by Bahram and Netherway (2022) exemplifies the impact of mycorrhizal associations on plant nutrient acquisition and stress tolerance. They found that specific mycorrhizal fungi, such as Rhizophagus irregularis, enhance phosphorus uptake in plants, thereby increasing nutrient acquisition efficiency and improving resilience to environmental stresses like drought, as well as their role in shaping ecosystem dynamics and resilience (e.g., Shi et al., 2023).
5.4 Bioremediation
Mycorrhizal fungi, particularly arbuscular mycorrhizae, are integral to soil health and have garnered attention for their potential in bioremediation efforts. One of the most notable applications is in the restoration of degraded ecosystems and the remediation of contaminated soils (Asmelash et al., 2016; Sharma et al., 2021; Wahab et al., 2023). For instance, Rhizophagus irregularis has been extensively studied for its role in enhancing the uptake of pollutants, such as heavy metals, by plants, thereby aiding in the detoxification and removal of these contaminants from the soil (Boorboori and Zhang, 2022; Ma et al., 2022; Fall et al., 2022). Additionally, Glomus mosseae, Funneliformis mosseae, and Gigaspora margarita have also shown promising potential in bioremediation efforts through their ability to improve plant tolerance to environmental stresses and facilitate the degradation of various soil pollutants (Smith et al., 2018; Zhang et al., 2020; Li et al., 2023).
Arbuscular mycorrhizal fungi have been identified as forming symbiotic associations with plants thriving in polluted environments, enhancing the plant’s capability to uptake and immobilise heavy metals within their tissues (Begum et al., 2019; Boorboori and Zhang, 2022). These fungi establish a mycorrhizal network that extends the root system and acts as an efficient conduit for metal translocation from the soil to the plant’s above-ground parts. This mechanism can substantially decrease metal concentrations in the soil, thereby reducing its toxicity and aiding in ecosystem restoration (Smith et al., 2011; Bücking and Kafle, 2015). Additionally, the accumulation of heavy metals within plant biomass is of interest for phytoremediation, a strategy utilising hyperaccumulating plants to extract metals from polluted soils for subsequent harvesting and processing (Yan et al., 2020; Nedjimi, 2021; Kafle et al., 2022). Glomus intraradices have shown promise in facilitating the sequestration of heavy metals within plant tissues, thereby aiding in the remediation of contaminated soils (Boorboori and Zhang, 2022).
5.5 Biocontrol
Beneficial fungi, like Trichoderma species, are widely employed in biocontrol strategies against plant pathogens. Serving as natural antagonists, these biocontrol agents actively suppress the growth of harmful fungi, safeguarding plants from diseases and reducing reliance on chemical pesticides (Sun et al., 2023). Trichoderma, renowned for its mycoparasitic activity, parasitises various plant pathogens by producing enzymes and secondary metabolites that inhibit their growth and infection processes (Hermosa et al., 2012). By harnessing these natural antagonists, farmers can minimise agriculture’s environmental footprint, decrease pesticide residues in food, and promote the health and sustainability of agricultural ecosystems.
Understanding the fungi-plant interaction has led to practical applications with broad implications. From bioremediation to biocontrol, these applications demonstrate how insights into fungal-plant interactions can address crucial challenges in environmental restoration, agriculture, and sustainable land management. As we continue to explore and unlock the potential of these interactions, we pave the way for more efficient, eco-friendly, and sustainable solutions to pressing planetary issues.
5.6 Climate change impact
The fungi-plant interface faces significant challenges amidst the accelerating pace of global climate change. Escalating temperatures, shifting precipitation patterns, and heightened atmospheric carbon dioxide levels have the potential to disrupt established mutualistic and antagonistic interactions (Bennett and Classen, 2020; Duarte and Maherali, 2022). Such disruptions can affect fungal distribution, the virulence of pathogens, and the adaptability of mycorrhizal fungi to changing conditions. For instance, beneficial fungi like Trichoderma harzianum, known for its biocontrol properties, may encounter difficulties in suppressing plant pathogens due to these climatic shifts. Similarly, Rhizophagus irregularis and Glomus intraradices, both arbuscular mycorrhizal fungi that significantly enhance nutrient uptake in plants, are sensitive to temperature and moisture changes, potentially affecting their efficiency and the health of the plants they associate with (Ghorbanpour et al., 2018; Tyśkiewicz et al., 2022). On the pathogenic side, fungi such as Fusarium oxysporum could become more virulent under changing climate conditions, posing greater threats to crop health and agricultural productivity (Chakrapani et al., 2023; Ekwomadu and Mwanza, 2023). Addressing these challenges requires a deeper understanding of how environmental changes influence these intricate interactions and the development of strategies to enhance ecosystem resilience, ensuring the sustainability of both agricultural and natural ecosystems.
5.7 Emerging pathogens
The emergence of new fungal pathogens poses a continuous threat to agricultural and natural ecosystems, leading to disease outbreaks and economic losses. Effective mitigation requires identifying these pathogens, understanding their biology and host interactions, and integrating pathogen surveillance into disease management strategies for early detection and response. Notable examples of harmful fungi include Magnaporthe oryzae, which causes rice blast disease; Phytophthora infestans, responsible for late blight in potatoes and tomatoes; Puccinia graminis sp. tritici, which causes wheat stem rust; and Batrachochytrium dendrobatidis, which affects amphibians. These examples highlight the broader ecological threats posed by fungal diseases (Zhou et al., 2019; Ristaino et al., 2021). Addressing these emerging threats is crucial for protecting both agricultural productivity and ecosystem health.
6 Future directions
6.1 Harnessing fungal diversity
Exploring the vast diversity of fungi holds promise for uncovering novel beneficial species that could be utilised in agriculture and environmental restoration. Research efforts should focus on characterising fungal communities and their potential roles in enhancing plant health, soil fertility, and ecosystem resilience. For instance, Beauveria bassiana is valued for its entomopathogenic properties, making it a powerful biocontrol agent against insect pests. Penicillium bilaii has demonstrated the ability to solubilise phosphate, improving soil fertility and plant nutrient availability (Frąc et al., 2018; Noorjahan et al., 2022). Rhizophagus irregularis and Glomus intraradices are notable mycorrhizal fungi that enhance nutrient uptake and support robust plant growth (Kakabouki et al., 2021; Roussis et al., 2022). Additionally, Trichoderma harzianum is known for its ability to suppress plant pathogens and promote overall plant health (Zin and Badaluddin, 2020; Yao et al., 2023). By studying these and other fungi, we can develop innovative strategies to boost agricultural productivity and support effective ecosystem restoration, ensuring sustainable and resilient environments.
6.2 Restoration ecology
Restoration ecology increasingly relies on mycorrhizal fungi to restore soil health and enhance the success of ecological restoration projects. These fungi facilitate the establishment and growth of native plant species in degraded and nutrient-poor soils. For example, Glomus intraradices and Rhizophagus irregularis are commonly used to improve plant nutrient uptake and soil structure (Boorboori and Zhang, 2022). Future directions in restoration ecology involve the targeted use of mycorrhizal inoculants, such as Pisolithus tinctorius, to accelerate ecosystem recovery and enhance resilience to environmental stressors (Usman et al., 2021; Atala et al., 2023). Additionally, fungi like Scleroderma citrinum are being studied for their role in establishing mycorrhizal networks that support plant communities in challenging environments (Rajapitamahuni et al., 2023). Understanding plant-specific mycorrhizal associations informs tailored restoration approaches for diverse ecosystems and habitats, ensuring more effective and sustainable restoration outcomes.
6.3 Precision agriculture integration
Integrating fungal knowledge into precision agriculture practices can optimise resource utilisation and minimise environmental impacts. By leveraging insights into fungi-plant interactions, farmers can tailor management practices to specific crop and soil conditions, enhancing productivity while reducing inputs such as fertilisers and pesticides. For example, Rhizophagus irregularis can improve nutrient uptake in crops, reducing the need for chemical fertilisers (Roy and George, 2020; Iqbal et al., 2023; Yadav et al., 2023; Ahmed et al., 2024). Trichoderma harzianum is effective in controlling soil-borne diseases, decreasing reliance on chemical pesticides (Yao et al., 2023). Penicillium bilaii helps solubilise phosphate in the soil, making this essential nutrient more available to plants and reducing the need for phosphate fertilisers (Sánchez-Esteva et al., 2016). Additionally, Beauveria bassiana can be used as a biocontrol agent against insect pests, providing an environmentally friendly alternative to chemical insecticides (Bamisile et al., 2021; Furuie et al., 2022; Islam et al., 2023). By integrating these fungi into precision agriculture, farmers can achieve higher crop yields and healthier soils with lower environmental footprints.
6.4 Biotechnological innovations and genomic insights
Advancements in genomics provide deep insights into the genetic and molecular mechanisms underlying beneficial and pathogenic interactions within the fungi-plant interface. Understanding these genetic bases allows for the development of targeted strategies to manage both mutualistic and antagonistic associations (Sharma et al., 2020; Diwan et al., 2022; Müller et al., 2023). For instance, identifying genes associated with mycorrhizal symbiosis can inform crop breeding for enhanced compatibility with fungi like Rhizophagus irregularis, thereby improving nutrient uptake. Similarly, genomic insights into the virulence factors of pathogens such as Magnaporthe oryzae, which causes rice blast disease, can facilitate the development of disease-resistant rice varieties. Research on Fusarium graminearum responsible for Fusarium head blight in cereals has led to the identification of genes involved in toxin production, aiding in the breeding of resistant crops (Mateus et al., 2020; Guigard et al., 2023; Priyashantha et al., 2023). Additionally, understanding the genetic pathways of beneficial fungi like Trichoderma harzianum has paved the way for novel biocontrol agents that can effectively suppress plant pathogens (Collinge et al., 2022; Yao et al., 2023). These genomic advancements are crucial for developing innovative solutions in agriculture and ecosystem management.
Continued advancements in biotechnology offer opportunities to develop novel tools and products for managing fungal interactions in agriculture and environmental conservation. Engineered mycorrhizal fungi such as Glomus intraradices with enhanced nutrient uptake capabilities can significantly boost crop yields while reducing fertiliser dependence (Odoh et al., 2020; Afridi et al., 2022; Sun et al., 2023). Trichoderma viride, a natural biocontrol agent, can be further optimised to more effectively target specific plant pathogens, minimising the need for chemical pesticides (Lahlali et al., 2022; Yao et al., 2023). Metarhizium anisopliae, known for its entomopathogenic properties, can be engineered to improve its efficacy against a broader range of insect pests, providing a sustainable alternative to chemical insecticides (St. Leger and Wang, 2020; Bamisile et al., 2021). Additionally, Aspergillus niger can be modified to enhance its ability to produce organic acids that aid in soil nutrient solubilisation, ensuring better nutrient availability in soils (do Nascimento et al., 2021). These biotechnological innovations have the potential to revolutionise sustainable farming and ecosystem restoration practices, leading to more resilient agricultural systems and healthier environments.
6.5 Climate resilience strategies
Developing climate-resilient agricultural and ecological systems requires integrating fungus- and plant-based solutions. Research efforts should focus on identifying fungal species and traits that confer resilience to climate change stressors, such as drought and heat. For example, Rhizophagus irregularis is known for its ability to enhance plant drought tolerance by improving water uptake (Poudel et al., 2021; Verma et al., 2022). Pisolithus tinctorius is another mycorrhizal fungus that can help plants withstand extreme heat and poor soil conditions. Trichoderma harzianum not only acts as a biocontrol agent but also promotes plant growth under stressful environmental conditions (Baptista et al., 2021; Tyśkiewicz et al., 2022). Additionally, Piriformospora indica has been shown to increase plant resistance to both drought and salinity (Boorboori and Zhang, 2022; Li et al., 2023). Harnessing these fungi in breeding programmes and ecosystem restoration initiatives can enhance the adaptability and sustainability of agricultural and natural systems, ensuring their resilience in the face of climate change.
6.6 Interdisciplinary collaboration
Addressing the challenges and opportunities of the fungi-plant interface requires interdisciplinary collaboration among scientists, policymakers, farmers, and conservationists. By working together, we can develop comprehensive strategies to utilise fungal diversity for sustainable agriculture and ecosystem management, ensuring food security and environmental sustainability. For example, Piriformospora indica enhances plant growth and stress tolerance under drought and salinity conditions. Serendipita vermifera improves nutrient uptake and promotes root growth, benefiting agricultural productivity (Jeger et al., 2021; Bouri et al., 2023). Hirsutella thompsonii serves as a biocontrol agent against mite pests, reducing the need for chemical treatments (Kumar, 2010; Palevsky et al., 2022). Mortierella elongata contributes to soil health by decomposing organic matter and enhancing soil nutrient cycling (Li et al., 2018; Zhang et al., 2020). These examples demonstrate the potential of fungal-based solutions to address global environmental challenges effectively through collaborative efforts.
7 Conclusion
This review has highlighted the intricate and dynamic nature of interactions at the fungus-plant interface, underscoring their critical role in ecological and agricultural systems. Mutualistic relationships, particularly mycorrhizal associations, are pivotal for enhancing plant nutrient acquisition, stress tolerance, and overall ecosystem productivity. These beneficial interactions are essential for maintaining ecological balance and supporting sustainable agricultural practices. However, pathogenic fungi pose significant threats to plant health, leading to substantial agricultural losses and ecosystem disruption. The competition between these antagonistic and mutualistic interactions is profoundly influenced by environmental constraints such as temperature, moisture level, soil composition, competing plants, and other microorganisms. Rising temperatures, altered precipitation patterns, and increased atmospheric CO2 levels can disrupt established fungi-plant relationships, potentially leading to increased pathogen virulence and decreased efficiency of beneficial fungi. Future research should focus on integrating genomic insights to unravel the genetic and molecular mechanisms governing these interactions. Such knowledge can inform the development of crops with enhanced resistance to pathogens and improved compatibility with beneficial fungi. Additionally, applying this understanding to restoration ecology can enhance the success of efforts aimed at rehabilitating degraded ecosystems by leveraging the symbiotic potential of mycorrhizal fungi. Through interdisciplinary collaboration and innovative research, we can develop strategies that support the health and productivity of both natural and managed ecosystems, ultimately contributing to global food security and environmental conservation.
Author contributions
SM: Writing – original draft, Writing – review & editing. ASr: Writing – review & editing. ASi: Data curation, Formal analysis, Funding acquisition, Investigation, Resources, Visualization, Writing – review & editing. GP: Conceptualization, Data curation, Formal analysis, Funding acquisition, Investigation, Methodology, Project administration, Resources, Software, Supervision, Validation, Visualization, Writing – original draft, Writing – review & editing. GS: Conceptualization, Data curation, Formal analysis, Funding acquisition, Investigation, Methodology, Project administration, Resources, Software, Supervision, Validation, Visualization, Writing – original draft, Writing – review & editing.
Funding
The author(s) declare that no financial support was received for the research, authorship, and/or publication of this article.
Conflict of interest
The authors declare that the research was conducted in the absence of any commercial or financial relationships that could be construed as a potential conflict of interest.
Publisher’s note
All claims expressed in this article are solely those of the authors and do not necessarily represent those of their affiliated organizations, or those of the publisher, the editors and the reviewers. Any product that may be evaluated in this article, or claim that may be made by its manufacturer, is not guaranteed or endorsed by the publisher.
References
Abdul Rahman N. S. N., Abdul Hamid N. W., Nadarajah K. (2021). Effects of abiotic stress on soil microbiome. Int. J. Mol. Sci. 22, 9036. doi: 10.3390/ijms22169036
Adedayo A. A., Babalola O. O. (2023). Fungi that promote plant growth in the rhizosphere boost crop growth. J. Fungi. 9, 239. doi: 10.3390/jof9020239
Adnan M., Islam W., Shabbir A., Khan K. A., Ghramh H. A., Huang Z., et al. (2019). Plant defense against fungal pathogens by antagonistic fungi with Trichoderma in focus. Microb. Pathogene. 129, 7–18. doi: 10.1016/j.micpath.2019.01.042
Afridi M. S., Javed M. A., Ali S., De Medeiros F. H. V., Ali B., Salam A., et al. (2022). New opportunities in plant microbiome engineering for increasing agricultural sustainability under stressful conditions. Front. Plant Sci. 13, 899464. doi: 10.3389/fpls.2022.899464
Agerer R. (2006). Fungal relationships and structural identity of their ectomycorrhizae. Mycol. Prog. 5, 67–107. doi: 10.1007/s11557-006-0505-x
Ahmed N., Zhang B., Deng L., Bozdar B., Li J., Chachar S., et al. (2024). Advancing horizons in vegetable cultivation: a journey from ageold practices to high-tech greenhouse cultivation—a review. Front. Plant Sci. 15, 1357153. doi: 10.3389/fpls.2024.1357153
Akram S., Ahmed A., He P., He P., Liu Y., Wu Y., et al. (2023). Uniting the role of endophytic fungi against plant pathogens and their interaction. J. Fungi. 9, 72. doi: 10.3390/jof9010072
Alam B., Lï J., Gě Q., Khan M. A., Gōng J., Mehmood S., et al. (2021). Endophytic fungi: From symbiosis to secondary metabolite communications or vice versa? Front. Plant Sci. 12, 3060. doi: 10.3389/fpls.2021.791033
Almeida F., Rodrigues M. L., Coelho C. (2019). The still underestimated problem of fungal diseases worldwide. Front. Microbiol. 10, 214. doi: 10.3389/fmicb.2019.00214
Asmelash F., Bekele T., Birhane E. (2016). The potential role of arbuscular mycorrhizal fungi in the restoration of degraded lands. Front. Microbiol. 7, 1095. doi: 10.3389/fmicb.2016.01095
Atala C., Reyes S. A., Molina-Montenegro M. A. (2023). Assessing the importance of native mycorrhizal fungi to improve tree establishment after wildfires. J. Fungi. 9, 421. doi: 10.3390/jof9040421
Bahram M., Netherway T. (2022). Fungi as mediators linking organisms and ecosystems. FEMS Microbiol. Rev. 46, fuab058. doi: 10.1093/femsre/fuab058
Balestrini R. (2021). Grand challenges in fungi-plant interactions. Front. Fungal Biol. 2, 750003. doi: 10.3389/ffunb.2021.750003
Bamisile B. S., Akutse K. S., Siddiqui J. A., Xu Y. (2021). Model application of entomopathogenic fungi as alternatives to chemical pesticides: Prospects, challenges, and insights for next-generation sustainable agriculture. Front. Plant Sci. 12, 741804. doi: 10.3389/fpls.2021.741804
Baptista P., Guedes de Pinho P., Moreira N., Malheiro R., Reis F., Padrão J., et al. (2021). In vitro interactions between the ectomycorrhizal Pisolithus tinctorius and the saprotroph Hypholoma fasciculare fungi: Morphological aspects and volatile production. Mycology 12, 216–229. doi: 10.1080/21501203.2021.1876778
Basandrai A. K., Mehta A., Basandrai D. (2023). Virulence structure of wheat powdery mildew pathogen, Blumeria graminis tritici: a review. Indian Phytopathol. 76, 21–45. doi: 10.1007/s42360-022-00571-z
Bastías Campos D. A., Applegate E., Johnson L., Card S. (2023). Factors controlling the effects of mutualistic bacteria on plants associated with fungi. Ecology Letters. 4, 3387–3398. doi: 10.1111/pce.14455
Begum N., Qin C., Ahanger M. A., Raza S., Khan M. I., Ashraf M., et al. (2019). Role of arbuscular mycorrhizal fungi in plant growth regulation: implications in abiotic stress tolerance. Front. Plant Sci. 10, 1068. doi: 10.3389/fpls.2019.01068
Bennett A. E., Classen A. T. (2020). Climate change influences mycorrhizal fungal–plant interactions, but conclusions are limited by geographical study bias. Ecology 101, e02978. doi: 10.1002/ecy.2978
Berruti A., Lumini E., Balestrini R., Bianciotto V. (2016). Arbuscular mycorrhizal fungi as natural biofertilizers: let’s benefit from past successes. Front. Microbiol. 6, 1559. doi: 10.3389/fmicb.2015.01559
Bika R., Baysal-Gurel F., Jennings C. (2021). Botrytis cinerea management in ornamental production: a continuous battle. Can. J. Plant Pathol. 43, 345–365. doi: 10.1080/07060661.2020.1807409
Bonfante P., Genre A. (2010). Mechanisms underlying beneficial plant–fungus interactions in mycorrhizal symbiosis. Nat. Commun. 1, 48. doi: 10.1038/ncomms1046
Boorboori M. R., Zhang H. Y. (2022). The role of Serendipita indica (Piriformospora indica) in improving plant resistance to drought and salinity stresses. Biology 11, 952. doi: 10.3390/biology11070952
Bouri M., Arslan K. S., Şahin F. (2023). Climate-smart pest management in sustainable agriculture: promises and challenges. Sustainability 15, 4592. doi: 10.3390/su15054592
Bücking H., Kafle A. (2015). Role of arbuscular mycorrhizal fungi in the nitrogen uptake of plants: current knowledge and research gaps. Agronomy 5, 587–612. doi: 10.3390/agronomy5040587
Cairney J. W., Meharg A. A. (2003). Ericoid mycorrhiza: a partnership that exploits harsh edaphic conditions. Eur. J. Soil Sci. 54, 735–740. doi: 10.1046/j.1351-0754.2003.0555.x
Canarini A., Kaiser C., Merchant A., Richter A., Wanek W. (2019). Root exudation of primary metabolites: mechanisms and their roles in plant responses to environmental stimuli. Front. Plant Sci. 10, 157. doi: 10.3389/fpls.2019.00157
Caradus J. R., Johnson L. J. (2020). Epichloë fungal endophytes—From a biological curiosity in wild grasses to an essential component of resilient high performing ryegrass and fescue pastures. J. Fungi. 6, 322. doi: 10.3390/jof6040322
Chakrapani K., Chanu W. T., Sinha B., Thangjam B., Hasan W., Devi K. S., et al. (2023). Deciphering growth abilities of fusarium oxysporum f. sp. pisi under variable temperature, pH and nitrogen. Front. Microbiol. 14, 1228442. doi: 10.3389/fmicb.2023.1228442
Chang H. X., Noel Z. A., Sang H., Chilvers M. I. (2018). Annotation resource of tandem repeat-containing secretory proteins in sixty fungi. Fungal Genet. Biol. 119, 7–19. doi: 10.1016/j.fgb.2018.07.004
Chaudhary P., Agri U., Chaudhary A., Kumar A., Kumar G. (2022). Endophytes and their potential in biotic stress management and crop production. Front. Microbiol. 13, 933017. doi: 10.3389/fmicb.2022.933017
Chen M., Arato M., Borghi L., Nouri E., Reinhardt D. (2018). Beneficial services of arbuscular mycorrhizal fungi–from ecology to application. Front. Plant Sci. 9, 1270. doi: 10.3389/fpls.2018.01270
Chen W., Modi D., Picot A. (2023). Soil and phytomicrobiome for plant disease suppression and management under climate change: A review. Plants 12, 2736. doi: 10.3390/plants12142736
Collinge D. B., Jensen D. F., Rabiey M., Sarrocco S., Shaw M. W., Shaw R. H. (2022). Biological control of plant diseases–What has been achieved and what is the direction? Plant Pathol. 71, 1024–1047. doi: 10.1016/j.mib.2022.102177
De Lamo F. J., Takken F. L. (2020). Biocontrol by Fusarium oxysporum using endophyte-mediated resistance. Front. Plant Sci. 11, 37. doi: 10.3389/fpls.2020.00037
Demir S., Danesh Y. R., Boyno G., Najafi S. (2022). Arbuscular mycorrhizal fungi in biotic and abiotic stress conditions: Function and management in horticulture. Sustain. Horticult., 157–183. doi: 10.1016/B978-0-323-91861-9.00011-2
Deveau A., Bonito G., Uehling J., Paoletti M., Becker M., Bindschedler S., et al. (2018). Bacterial–fungal interactions: ecology, mechanisms and challenges. FEMS Microbiol. Rev. 42, 335–352. doi: 10.1093/femsre/fuy008
Dhiman N., Uthoff J., Scharf B., Kumar V. (2024). “Plant-microbe interaction to improve soil health,” in Advancements in Microbial Biotechnology for Soil Health (Springer Nature Singapore, Singapore), 189–226.
Ding X., Jia X., Zhao Y., Gao Y., Zhang C. (2024). Responses of root exudates of alfalfa to arbuscular mycorrhizal fungi colonisation, high temperature, and cadmium exposure. J. Soil Sci. Plant Nutr. 24, 1–17.
Dita M., Barquero M., Heck D., Mizubuti E. S., Staver C. P. (2018). Fusarium wilt of banana: current knowledge on epidemiology and research needs toward sustainable disease management. Front. Plant Sci. 9, 1468. doi: 10.3389/fpls.2018.01468
Diwan D., Rashid M. M., Vaishnav A. (2022). Current understanding of plant-microbe interaction through the lenses of multi-omics approaches and their benefits in sustainable agriculture. Microbiol. Res. 265, 127180. doi: 10.1016/j.micres.2022.127180
Doehlemann G., Ökmen B., Zhu W., Sharon A. (2017). Plant pathogenic fungi. Microbiol. Spectr. 5, 5.1. 14. doi: 10.1128/9781555819583.ch34
do Nascimento J. M., Netto J. A. F. V., Valadares R. V., de Oliveira Mendes G., da Silva I. R., Vergütz L., et al. (2021). Aspergillus Niger as a key to unlock fixed phosphorus in highly weathered soils. Soil Biol. Biochem. 156, 108190. doi: 10.1016/j.soilbio.2021.108190
Duarte A. G., Maherali H. (2022). A meta-analysis of the effects of climate change on the mutualism between plants and arbuscular mycorrhizal fungi. Ecol. Evol. 12, e8518. doi: 10.1002/ece3.8518
Dutta P., Mahanta M., Singh S. B., Thakuria D., Deb L., Kumari A., et al. (2023). Molecular interaction between plants and Trichoderma species against soil-borne plant pathogens. Front. Plant Sci. 14, 1145715. doi: 10.3389/fpls.2023.1145715
Duvnjak T., Vrandecic K., Sudaric A., Cosic J., Siber T., Matosa Kocar M. (2023). First report of hemp fusarium wilt caused by fusarium oxysporum in Croatia. Plants 12, 3305. doi: 10.3390/plants12183305
Dyshko V., Hilszczańska D., Davydenko K., Matić S., Moser W. K., Borowik P., et al. (2024). An overview of mycorrhiza in pines: research, species, and applications. Plants 13, 506. doi: 10.3390/plants13040506
Ekwomadu T. I., Mwanza M. (2023). Fusarium fungi pathogens, identification, adverse effects, disease management, and global food security: A review of the latest research. Agriculture 13, 1810. doi: 10.3390/agriculture13091810
Emmanuel O. C., Babalola O. O. (2020). Productivity and quality of horticultural crops through co-inoculation of arbuscular mycorrhizal fungi and plant growth promoting bacteria. Microbiol. Res. 239, 126569. doi: 10.1016/j.micres.2020.126569
Etesami H., Jeong B. R., Glick B. R. (2021). Contribution of arbuscular mycorrhizal fungi, phosphate–solubilizing bacteria, and silicon to P uptake by plant. Front. Plant Sci. 12, 699618. doi: 10.3389/fpls.2021.699618
Fadiji A. E., Babalola O. O. (2020). Elucidating mechanisms of endophytes used in plant protection and other bioactivities with multifunctional prospects. Front. Bioeng. Biotechnol. 8, 467. doi: 10.3389/fbioe.2020.00467
Fall A. F., Nakabonge G., Ssekandi J., Founoune-Mboup H., Apori S. O., Ndiaye A., et al. (2022). Roles of arbuscular mycorrhizal fungi on soil fertility: Contribution in the improvement of physical, chemical, and biological properties of the soil. Front. Fungal Biol. 3. doi: 10.3389/ffunb.2022.723892
Farias G. C., Nunes K. G., Soares M. A., de Siqueira K. A., Lima W. C., Neves A. L. R., et al. (2020). Dark septate endophytic fungi mitigate the effects of salt stress on cowpea plants. Braz. J. Microbiol. 51, 243–253. doi: 10.1007/s42770-019-00173-4
Fehrer J., Réblová M., Bambasová V., Vohník M. (2019). The root-symbiotic Rhizoscyphus ericae aggregate and Hyaloscypha (Leotiomycetes) are congeneric: Phylogenetic and experimental evidence. Stud. Mycol. 92, 195–225. doi: 10.1016/j.simyco.2018.10.004
Figueiredo A. F., Boy J., Guggenberger G. (2021). Common mycorrhizae network: a review of the theories and mechanisms behind underground interactions. Front. Fungal Biol. 2, 48. doi: 10.3389/ffunb.2021.735299
Frąc M., Hannula S. E., Bełka M., Jędryczka M. (2018). Fungal biodiversity and their role in soil health. Front. Microbiol. 9, 316246. doi: 10.3389/fmicb.2018.00707
Freitas P. P., Hampton J. G., Rolston M. P., Glare T. R., Miller P. P., Card S. D. (2020). A tale of two grass species: Temperature affects the symbiosis of a mutualistic Epichloë endophyte in both tall fescue and perennial ryegrass. Front. Plant Sci. 11, 530. doi: 10.3389/fpls.2020.00530
Frey-Klett P., Burlinson P., Deveau A., Barret M., Tarkka M., Sarniguet A. (2011). Bacterial-fungal interactions: hyphens between agricultural, clinical, environmental, and food microbiologists. Microbiol. Mol. Biol. Rev. 75, 583–609. doi: 10.1128/MMBR.00020-11
Furuie J. L., Stuart A. K. D. C., Voidaleski M. F., Zawadneak M. A. C., Pimentel I. C. (2022). Isolation of Beauveria strains and their potential as control agents for lema bilineata germar (Coleoptera: Chrysomelidae). Insects 13, 93. doi: 10.3390/insects13010093
Gessler C., Pertot I., Perazzolli M. (2011). Plasmopara viticola: a review of knowledge on downy mildew of grapevine and effective disease management. Phytopathol. Mediterr. 50, 3–44.
Ghimire B., Sapkota S., Bahri B. A., Martinez-Espinoza A. D., Buck J. W., Mergoum M. (2020). Fusarium head blight and rust diseases in soft red winter wheat in the southeast united states: State of the art, challenges and future perspective for breeding. Front. Plant Sci. 11, 1080.
Ghorbanpour M., Omidvari M., Abbaszadeh-Dahaji P., Omidvar R., Kariman K. (2018). Mechanisms underlying the protective effects of beneficial fungi against plant diseases. Biol. Control. 117, 147–157. doi: 10.1016/j.biocontrol.2017.11.006
Gil-Martínez M., López-García Á., Domínguez M. T., Navarro-Fernández C. M., Kjøller R., Tibbett M., et al. (2018). Ectomycorrhizal fungal communities and their functional traits mediate plant–soil interactions in trace element contaminated soils. Front. Plant Sci. 9, 1682. doi: 10.3389/fpls.2018.01682
Giovannini L., Palla M., Agnolucci M., Avio L., Sbrana C., Turrini A., et al. (2020). Arbuscular mycorrhizal fungi and associated microbiota as plant biostimulants: research strategies for the selection of the best performing inocula. Agronomy 10, 106. doi: 10.3390/agronomy10010106
Godfray H. C. J., Mason-D’Croz D., Robinson S. (2016). Food system consequences of a fungal disease epidemic in a major crop. Philos. Trans. R. Soc. B.: Biol. Sci. 371, 20150467. doi: 10.1098/rstb.2015.0467
Gorzelak M. A., Asay A. K., Pickles B. J., Simard S. W. (2015). Inter-plant communication through mycorrhizal networks mediates complex adaptive behaviour in plant communities. AoB. Plants 7, plv050. doi: 10.1093/aobpla/plv050
Guigard L., Jobert L., Busset N., Moulin L., Czernic P. (2023). Symbiotic compatibility between rice cultivars and arbuscular mycorrhizal fungi genotypes affects rice growth and mycorrhiza-induced resistance. Front. Plant Sci. 14, 1278990. doi: 10.3389/fpls.2023.1278990
Haverkort A. J., Struik P. C., Visser R. G. F., Jacobsen E. J. P. R. (2009). Applied biotechnology to combat late blight in potato caused by phytophthora infestans. Potato Res. 52, 249–264.
He D. C., He M. H., Amalin D. M., Liu W., AlvIndia D. G., Zhan J. (2021). Biological control of plant diseases: an evolutionary and eco-economic consideration. Pathog. 2021. 10, 1311. doi: 10.3390/pathogens10101311
Hermosa R., Viterbo A., Chet I., Monte E. (2012). Plant-beneficial effects of Trichoderma and of its genes. Microbiol. 158, 17–25.
Hou L., Yu J., Zhao L., He X. (2020). Dark septate endophytes improve the growth and the tolerance of Medicago sativa and Ammopiptanthus mongolicus under cadmium stress. Front. Microbiol. 10, 3061. doi: 10.3389/fmicb.2019.03061
Huey C. J., Gopinath S. C., Uda M. N. A., Zulhaimi H. I., Jaafar M. N., Kasim F. H., et al. (2020). Mycorrhiza: a natural resource assists plant growth under varied soil conditions. 3. Biotech. 10, 1–9. doi: 10.1007/s13205-020-02188-3
Igiehon O. N., Babalola O. O. (2021). Rhizobium and mycorrhizal fungal species improved soybean yield under drought stress conditions. Curr. Microbiol. 78, pp.1615–1627. doi: 10.1007/s00284-021-02432-w
Iqbal B., Li G., Alabbosh K. F., Hussain H., Khan I., Tariq M., et al. (2023). Advancing environmental sustainability through microbial reprogramming in growth improvement, stress alleviation, and phytoremediation. Plant Stress 10, 100283. doi: 10.1016/j.stress.2023.100283
Islam S. M. N., Chowdhury M. Z. H., Mim M. F., Momtaz M. B., Islam T. (2023). Biocontrol potential of native isolates of Beauveria bassiana against cotton leafworm Spodoptera litura (Fabricius). Sci. Rep. 13, 8331. doi: 10.1038/s41598-023-35415-x
Ismaila A. A., Ahmad K., Siddique Y., Wahab M. A. A., Kutawa A. B., Abdullahi A., et al. (2023). Fusarium wilt of banana: Current update and sustainable disease control using classical and essential oils approaches. Hortic. Plant J. 9, 1–28. doi: 10.1016/j.hpj.2022.02.004
Itoo Z. A., Reshi Z. A. (2013). The multifunctional role of ectomycorrhizal associations in forest ecosystem processes. Bot. Rev. 79, 371–400. doi: 10.1007/s12229-013-9126-7
Ivanov A. A., Ukladov E. O., Golubeva T. S. (2021). Phytophthora infestans: An overview of methods and attempts to combat late blight. J. Fungi. 7, 1071. doi: 10.3390/jof7121071
Jeger M., Beresford R., Bock C., Brown N., Fox A., Newton A., et al. (2021). Global challenges facing plant pathology: multidisciplinary approaches to meet the food security and environmental challenges in the mid-twenty-first century. CABI. Agric. Biosc. 2, 1–18. doi: 10.1186/s43170-021-00042-x
Kafle A., Timilsina A., Gautam A., Adhikari K., Bhattarai A., Aryal N. (2022). Phytoremediation: Mechanisms, plant selection and enhancement by natural and synthetic agents. Environ. Adv. 8, 100203. doi: 10.1016/j.envadv.2022.100203
Kakabouki I., Mavroeidis A., Tataridas A., Kousta A., Efthimiadou A., Karydogianni S., et al. (2021). Effect of Rhizophagus irregularis on Growth and Quality of Cannabis sativa Seedlings. Plants 10, 1333. doi: 10.3390/plants10071333
Kiss L., Vaghefi N., Bransgrove K., Dearnaley J. D., Takamatsu S., Tan Y. P., et al. (2020). Australia: A continent without native powdery mildews? The first comprehensive catalog indicates recent introductions and multiple host range expansion events, and leads to the re-discovery of Salmonomyces as a new lineage of the Erysiphales. Front. Microbiol. 11, 1571. doi: 10.3389/fmicb.2020.01571
Kranjec Orlović J., Diminić D., Ištok I., Volenec I., Hodak L., Grubešić M., et al. (2024). Fungal Presence and Changes of Wood Structure in Bark Stripping Wounds Made by Red Deer (Cervus elaphus L.) on Stems of Fraxinus angustifolia (Vahl). Forests 15, 314. doi: 10.3390/f15020314
Kulkova I., Dobrzyński J., Kowalczyk P., Bełżecki G., Kramkowski K. (2023). Plant growth promotion using Bacillus cereus. Int. J. Mol. Sci. 24, 9759. doi: 10.3390/ijms24119759
Kumar P. S. (2010). “Hirsutella thompsonii as a mycoacaricide for Aceria guerreronis on coconut in India: research, development, and other aspects,” in Trends in Acarology: Proceedings of the 12th International Congress. 441–444 (Springer Netherlands).
Lahlali R., Ezrari S., Radouane N., Kenfaoui J., Esmaeel Q., El Hamss H., et al. (2022). Biological control of plant pathogens: A global perspective. Microorganisms 10, 596. doi: 10.3390/microorganisms10030596
Lanfranco L., Bonfante P., Genre A. (2016). The mutualistic interaction between plants and arbuscular mycorrhizal fungi. Microbiol. Spectr. 4, 4–6. doi: 10.1128/microbiolspec.FUNK-0012-2016
Lebeda A., Sedlářová M., Petřivalský M., Prokopová J. (2008). Diversity of defence mechanisms in plant–oomycete interactions: a case study of lactuca spp. and bremia lactucae. Eur. J. Plant Pathol. 122, 71–89.
Lee K., Missaoui A., Mahmud K., Presley H., Lonnee M. (2021). Interaction between grasses and Epichloë endophytes and its significance to biotic and abiotic stress tolerance and the rhizosphere. Microorganisms 9, 2186. doi: 10.3390/microorganisms9112186
Leite R. D. C., Hungria M., Leite R. D. C., Souza de Ávila W., da Silva G. B. (2024). Co-inoculation of soybean with Bradyrhizobium spp. and Amazonian strains of Trichoderma asperellum and Bacillus amyloliquefaciens increases the productivity and profitability of the crop. Crop Sci. 64, 422–441. doi: 10.1002/csc2.21091
Li F., Chen L., Redmile-Gordon M., Zhang J., Zhang C., Ning Q., et al. (2018). Mortierella elongata’s roles in organic agriculture and crop growth promotion in a mineral soil. Land. Degradation. Dev. 29, 1642–1651. doi: 10.1002/ldr.2965
Li L., Feng Y., Qi F., Hao R. (2023). Research progress of Piriformospora indica in improving plant growth and stress resistance to plant. J. Fungi. 9, 965. doi: 10.3390/jof9100965
Li T., Wu S., Yang W., Selosse M. A., Gao J. (2021). How mycorrhizal associations influence orchid distribution and population dynamics. Front. Plant Sci. 12, 647114. doi: 10.3389/fpls.2021.647114
Lin D. J., Zhou J. X., Ali A., Fu H. Y., Gao S. J., Jin L., et al. (2024). Biocontrol efficiency and characterization of insecticidal protein from sugarcane endophytic Serratia marcescens (SM) against oriental armyworm Mythimna separata (Walker). Int. J. Biol. Macromol. 262, 129978. doi: 10.1016/j.ijbiomac.2024.129978
Liu Y., Li X., Kou Y. (2020). Ectomycorrhizal fungi: Participation in nutrient turnover and community assembly pattern in forest ecosystems. Forests 11, 453. doi: 10.3390/f11040453
Lu H., Wei T., Lou H., Shu X., Chen Q. (2021). A critical review on communication mechanism within plant-endophytic fungi interactions to cope with biotic and abiotic stresses. J. Fungi. 7, p.719. doi: 10.3390/jof7090719
Ma Y., Tiwari J., Bauddh K. (2022). Plant-mycorrhizal fungi interactions in phytoremediation of geogenic contaminated soils. Front. Microbiol. 13, 843415. doi: 10.3389/fmicb.2022.843415
Macioszek V. K., Marciniak P., Kononowicz A. K. (2023). Impact of Sclerotinia sclerotiorum Infection on Lettuce (Lactuca sativa L.) Survival and Phenolics Content—A Case Study in a Horticulture Farm in Poland. Pathogens 12, 1416. doi: 10.3390/pathogens12121416
Malicka M., Magurno F., Piotrowska-Seget Z. (2022). Plant association with dark septate endophytes: When the going gets tough (and stressful), the tough fungi get going. Chemosphere 302, 134830. doi: 10.1016/j.chemosphere.2022.134830
Malinowski D. P., Belesky D. P. (2006). Ecological importance of Neotyphodium spp. grass endophytes in agroecosystems. Grassland. Sci. 52, 1–14. doi: 10.1111/j.1744-697X.2006.00041.x
Mapuranga J., Zhang N., Zhang L., Chang J., Yang W. (2022). Infection strategies and pathogenicity of biotrophic plant fungal pathogens. Front. Microbiol. 13, 799396. doi: 10.3389/fmicb.2022.799396
Mateus I. D., Rojas E. C., Savary R., Dupuis C., Masclaux F. G., Aletti C., et al. (2020). Coexistence of genetically different Rhizophagus irregularis isolates induces genes involved in a putative fungal mating response. ISME. J. 14, 2381–2394. doi: 10.1038/s41396-020-0694-3
Mayer V. E., Voglmayr H., Blatrix R., Orivel J., Leroy C. (2023). Fungi as mutualistic partners in ant-plant interactions. Front. Fungal Biol. 4. doi: 10.3389/ffunb.2023.1213997
McGrath D. A., Duryea M. L., Cropper W. P. (2001). Soil phosphorus availability and fine root proliferation in amazonian agroforests 6 years following forest conversion. Agric. Ecosyst. Environ. 83, 271–284.
Micali C., Göllner K., Humphry M., Consonni C., Panstruga R. (2008). The powdery mildew disease of Arabidopsis: a paradigm for the interaction between plants and biotrophic fungi. Arabidopsis. Book/American. Soc. Plant Biol. 6, 8. doi: 10.1199/tab.0115
Mondal S., Halder S. K., Mondal K. C. (2022). “Revisiting soil-plant-microbes interactions: Key factors for soil health and productivity,” in Trends of Applied Microbiology for Sustainable Economy (Academic Press), 125–154.
Müller M., Kües U., Budde K. B., Gailing O. (2023). Applying molecular and genetic methods to trees and their fungal communities. Appl. Microbiol. Biotechnol. 107, 2783–2830. doi: 10.1007/s00253-023-12480-w
Nedjimi B. (2021). Phytoremediation: a sustainable environmental technology for heavy metals decontamination. SN. Appl. Sci. 3, 286. doi: 10.1007/s42452-021-04301-4
Netherway T., Bengtsson J., Krab E. J., Bahram M. (2021). Biotic interactions with mycorrhizal systems as extended nutrient acquisition strategies shaping forest soil communities and functions. Basic. Appl. Ecol. 50, 25–42. doi: 10.1016/j.baae.2020.10.002
Ngah N., Thomas R. L., Fellowes M. D. (2024). Does this look infected? Hidden host plant infection by the pathogen botrytis cinerea alters interactions between plants, aphids and their natural enemies in the field. Insects 15, 347. doi: 10.3390/insects15050347
Noorjahan A., Mahesh S., Aiyamperumal B., Anantharaman P. (2022). “Exploring marine fungal diversity and their applications in agriculture,” in Fungal diversity, ecology and control management (Springer Nature Singapore, Singapore), 293–310.
Odoh C. K., Eze C. N., Obi C. J., Anyah F., Egbe K., Unah U. V., et al. (2020). “Fungal biofertilizers for sustainable agricultural productivity,” in Agriculturally Important Fungi for Sustainable Agriculture: Volume 1: Perspective for Diversity and Crop Productivity, 199–225.
Palevsky E., Konopická J., Rueda-Ramírez D., Zemek R. (2022). A review of prospective biocontrol agents and sustainable soil practices for bulb mite (Acari: acaridae) management. Agronomy 12, 1491. doi: 10.3390/agronomy12071491
Peng Y., Li S. J., Yan J., Tang Y., Cheng J. P., Gao A. J., et al. (2021). Research progress on phytopathogenic fungi and their role as biocontrol agents. Front. Microbiol. 12, 670135. doi: 10.3389/fmicb.2021.670135
Perotto S., Daghino S., Martino E. (2018). Ericoid mycorrhizal fungi and their genomes: another side to the mycorrhizal symbiosis? New Phytol. 220, 1141–1147. doi: 10.1111/nph.15218
Policelli N., Horton T. R., Hudon A. T., Patterson T. R., Bhatnagar J. M. (2020). Back to roots: The role of ectomycorrhizal fungi in boreal and temperate forest restoration. Front. Forests. Global Change 3, 97. doi: 10.3389/ffgc.2020.00097
Porras-Alfaro A., Bayman P. (2011). Hidden fungi, emergent properties: endophytes and microbiomes. Annu. Rev. Phytopathol. 49, 291–315.
Poudel M., Mendes R., Costa L. A., Bueno C. G., Meng Y., Folimonova S. Y., et al. (2021). The role of plant-associated bacteria, fungi, and viruses in drought stress mitigation. Front. Microbiol. 12, 743512. doi: 10.3389/fmicb.2021.743512
Prahl R. E., Khan S., Deo R. C. (2023). Ampelomyces mycoparasites of powdery mildews–A review. Can. J. Plant Pathol. 45, 391–404. doi: 10.1080/07060661.2023.2206378
Priyashantha A. H., Dai D. Q., Bhat D. J., Stephenson S. L., Promputtha I., Kaushik P., et al. (2023). Plant–fungi interactions: where it goes? Biology 12, 809. doi: 10.3390/biology12060809
Puelles M., Arbizu-Milagro J., Castillo-Ruiz F. J., Peña J. M. (2024). Predictive models for grape downy mildew (Plasmopara viticola) as a decision support system in Mediterranean conditions. Crop Prot. 175, 106450. doi: 10.1016/j.cropro.2023.106450
Purohit A., Ghosh S., Chaudhuri R. K., Chakraborti D. (2023). “Biological control of Fusarium wilt in legumes,” in Plant Stress Mitigators (Academic Press), 435–454.
Rahman M., Borah S. M., Borah P. K., Bora P., Sarmah B. K., Lal M. K., et al. (2023). Deciphering the antimicrobial activity of multifaceted rhizospheric biocontrol agents of solanaceous crops viz., Trichoderma harzianum MC2, and Trichoderma harzianum NBG. Front. Plant Sci. 14, 1141506. doi: 10.3389/fpls.2023.1141506
Rajapitamahuni S., Kang B. R., Lee T. K. (2023). Exploring the roles of arbuscular mycorrhizal fungi in plant–iron homeostasis. Agriculture 13, 1918. doi: 10.3390/agriculture13101918
Rasmussen S., Parsons A. J., Fraser K., Xue H., Newman J. A. (2008). Metabolic profiles of Lolium perenne are differentially affected by nitrogen supply, carbohydrate content, and fungal endophyte infection. Plant Physiol. 146, 1440–1453. doi: 10.1104/pp.107.111898
Raspor M., Berić T., Ćosić T., Stanković S. (2023). “The rhizosphere–A hub of emerging importance for plant biotic interactions,” in New Frontiers in Plant-Environment Interactions: Innovative Technologies and Developments (Springer Nature Switzerland, Cham), 289–327.
Ristaino J. B., Anderson P. K., Bebber D. P., Brauman K. A., Cunniffe N. J., Fedoroff N. V., et al. (2021). The persistent threat of emerging plant disease pandemics to global food security. Proc. Natl. Acad. Sci. 118, e2022239118. doi: 10.1073/pnas.2022239118
Rodrigues C. I. D., Brito L. M., Nunes L. J. (2023). Soil carbon sequestration in the context of climate change mitigation: A review. Soil Syst. 7, 64. doi: 10.3390/soilsystems7030064
Roussis I., Beslemes D., Kosma C., Triantafyllidis V., Zotos A., Tigka E., et al. (2022). The influence of arbuscular mycorrhizal fungus Rhizophagus irregularis on the growth and quality of processing tomato (Lycopersicon esculentum mill.) seedlings. Sustainability 14, 9001. doi: 10.3390/su14159001
Roy T., George K. J. (2020). “Precision farming: A step towards sustainable, climate-smart agriculture,” in Global climate change: Resilient and smart agriculture, 199–220.
Salcedo A. F., Purayannur S., Standish J. R., Miles T., Thiessen L., Quesada-Ocampo L. M. (2021). Fantastic downy mildew pathogens and how to find them: Advances in detection and diagnostics. Plants 10, 435. doi: 10.3390/plants10030435
Salcedo A., Parada-Rojas C. H., Guerrero R., Stahr M., D’Arcangelo K. N., McGregor C., et al. (2023). The NLR family of disease resistance genes in cultivated watermelon and other cucurbits: opportunities and challenges. Watermelon Genome. 37–67.
Sánchez-Esteva S., Gómez-Muñoz B., Jensen L. S., de Neergaard A., Magid J. (2016). The effect of Penicillium bilaii on wheat growth and phosphorus uptake as affected by soil pH, soil P and application of sewage sludge. Chem. Biol. Technol. Agric. 3, 1–11. doi: 10.1186/s40538-016-0075-3
Santoyo G., Gamalero E., Glick B. R. (2021). Mycorrhizal-bacterial amelioration of plant abiotic and biotic stress. Front. Sustain. Food Syst. 5, 672881. doi: 10.3389/fsufs.2021.672881
Schwartz H. F., Mohan S. K. (2008). “Back matter,” in Compendium of onion and garlic diseases and pests, second edition (The American Phytopathological Society), 107–127.
Selin C., De Kievit T. R., Belmonte M. F., Fernando W. D. (2016). Elucidating the role of effectors in plant-fungal interactions: progress and challenges. Front. Microbiol. 7, 600. doi: 10.3389/fmicb.2016.00600
Selvaraj A., Thangavel K., Uthandi S. (2020). Arbuscular mycorrhizal fungi (Glomus intraradices) and diazotrophic bacterium (Rhizobium BMBS) primed defense in blackgram against herbivorous insect (Spodoptera litura) infestation. Microbiol. Res. 231, 126355. doi: 10.1016/j.micres.2019.126355
Sharma K., Gupta S., Thokchom S. D., Kapoor R. (2021). The Potential Roles of arbuscular mycorrhizal fungi in soil health and conservation. Int. J. Plant Environ. 7, 39–48. doi: 10.18811/ijpen.v7i01.4
Sharma M., Sudheer S., Usmani Z., Rani R., Gupta P. (2020). Deciphering the omics of plant-microbe interaction: Perspectives and new insights. Curr. Genomics 21, 343–362. doi: 10.2174/1389202921999200515140420
Shi J., Wang X., Wang E. (2023). Mycorrhizal symbiosis in plant growth and stress adaptation: From genes to ecosystems. Annu. Rev. Plant Biol. 74, 569–607. doi: 10.1146/annurev-arplant-061722-090342
Singh B. K., Delgado-Baquerizo M., Egidi E., Guirado E., Leach J. E., Liu H., et al. (2023). Climate change impacts on plant pathogens, food security and paths forward. Nat. Rev. Microbiol. 21, 1–17. doi: 10.1038/s41579-023-00900-7
Singh L. P., Gill S. S., Tuteja N. (2011). Unraveling the role of fungal symbionts in plant abiotic stress tolerance. Plant Signaling Behav. 6, 175–171. doi: 10.4161/psb.6.2.14146
Smith G. R., Steidinger B. S., Bruns T. D., Peay K. G. (2018). Competition–colonization tradeoffs structure fungal diversity. ISME J. 12, 1758–1767.
Smith S. E., Jakobsen I., Grønlund M., Smith F. A. (2011). Roles of arbuscular mycorrhizas in plant phosphorus nutrition: interactions between pathways of phosphorus uptake in arbuscular mycorrhizal roots have important implications for understanding and manipulating plant phosphorus acquisition. Plant Physiol. 156 (3), 1050–1057.
St. Leger R. J., Wang J. B. (2020). Metarhizium: jack of all trades, master of many. Open Biol. 10, 200307. doi: 10.1098/rsob.200307
Strack D., Fester T., Hause B., Schliemann W., Walter M. H. (2003). Arbuscular mycorrhiza: biological, chemical, and molecular aspects. J. Chem. Ecol. 29, 1955–1979. doi: 10.1023/A:1025695032113
Stuart E. K., Plett K. L. (2020). Digging deeper: in search of the mechanisms of carbon and nitrogen exchange in ectomycorrhizal symbioses. Front. Plant Sci. 10, 1658. doi: 10.3389/fpls.2019.01658
Sudharsan M. S., Rajagopal K., Banu N. (2023). “An insight into fungi in forest ecosystems,” in Plant mycobiome: diversity, interactions and uses (Springer International Publishing, Cham), 291–318.
Sun W., Shahrajabian M. H. (2023). The application of arbuscular mycorrhizal fungi as microbial biostimulant, sustainable approaches in modern agriculture. Plants 12, 3101. doi: 10.3390/plants12173101
Sun Y., Shi M., Wang D., Gong Y., Sha Q., Lv P., et al. (2023). Research progress on the roles of actin-depolymerizing factor in plant stress responses. Front. Plant Sci. 14, 1278311. doi: 10.3389/fpls.2023.1278311
Suraby E. J., Agisha V. N., Dhandapani S., Sng Y. H., Lim S. H., Naqvi N. I., et al. (2023). Plant growth promotion under phosphate deficiency and improved phosphate acquisition by new fungal strain, Penicillium olsonii TLL1. Front. Microbiol. 14, 1285574. doi: 10.3389/fmicb.2023.1285574
Tello-García E., Mandolini E., Tasser E., Probst M., Peintner U., Tappeiner U., et al. (2023). Red clover productivity under drought: Are soil microbes a burden or a treasure? Environ. Exp. Bot. 214, 105486. doi: 10.1016/j.envexpbot.2023.105486
Thakur R. P., Mathur K. (2002). Downy mildews of India. Crop Prot. 21, 333–345. doi: 10.1016/S0261-2194(01)00097-7
Thakur M. P., van der Sloot M. A., Wilschut R. A., Hannula S. E., Hooven F. T., Geisen S., et al. (2023). Soil legacies of extreme droughts enhance the performance of invading plants. bioRxiv 1, 2023–2001. doi: 10.1101/2023.01.30.526304
Thambugala K. M., Daranagama D. A., Phillips A. J., Kannangara S. D., Promputtha I. (2020). Fungi vs. fungi in biocontrol: An overview of fungal antagonists applied against fungal plant pathogens. Front. Cell. Infection. Microbiol. 10, 604923. doi: 10.3389/fcimb.2020.604923
Tian Y., Xu J., Lian X., Wei B., Ma X., Wu P. (2023). Effect of Glomus intraradices on root morphology, biomass production and phosphorous use efficiency of Chinese fir seedlings under low phosphorus stress. Front. Plant Sci. 13, 1095772. doi: 10.3389/fpls.2022.1095772
Tibbett M., Sanders F. (2002). Ectomycorrhizal symbiosis can enhance plant nutrition through improved access to discrete organic nutrient patches of high resource quality. Ann. Bot. 89, 783–789. doi: 10.1093/aob/mcf129
Troch V., Audenaert K., Wyand R. A., Haesaert G., Höfte M., Brown J. K. (2014). Formae speciales of cereal powdery mildew: close or distant relatives? Mol. Plant Pathol. 15, 304–314.
Tyśkiewicz R., Nowak A., Ozimek E., Jaroszuk-Ściseł J. (2022). Trichoderma: The current status of its application in agriculture for the biocontrol of fungal phytopathogens and stimulation of plant growth. Int. J. Mol. Sci. 23, 2329. doi: 10.3390/ijms23042329
Usman M., Ho-Plágaro T., Frank H. E., Calvo-Polanco M., Gaillard I., Garcia K., et al. (2021). Mycorrhizal symbiosis for better adaptation of trees to abiotic stress caused by climate change in temperate and boreal forests. Front. Forests. Global Change 4, 742392. doi: 10.3389/ffgc.2021.742392
Verma A., Shameem N., Jatav H. S., Sathyanarayana E., Parray J. A., Poczai P., et al. (2022). Fungal endophytes to combat biotic and abiotic stresses for climate-smart and sustainable agriculture. Front. Plant Sci. 13, 953836. doi: 10.3389/fpls.2022.953836
Vohnik M. (2020). Ericoid mycorrhizal symbiosis: theoretical background and methods for its comprehensive investigation. Mycorrhiza 30, 671–695. doi: 10.1007/s00572-020-00989-1
Vohník M., Réblová M. (2023). Fungi in hair roots of Vaccinium spp.(Ericaceae) growing on decomposing wood: colonisation patterns, identity, and in vitro symbiotic potential. Mycorrhiza 33, 69–86. doi: 10.1007/s00572-023-01101-z
Wahab A., Muhammad M., Munir A., Abdi G., Zaman W., Ayaz A., et al. (2023). Role of arbuscular mycorrhizal fungi in regulating growth, enhancing productivity, and potentially influencing ecosystems under abiotic and biotic stresses. Plants 12, 3102. doi: 10.3390/plants12173102
Wei X., Zhang W., Zulfiqar F., Zhang C., Chen J. (2022). Ericoid mycorrhizal fungi as biostimulants for improving propagation and production of ericaceous plants. Front. Plant Sci. 13, 1027390. doi: 10.3389/fpls.2022.1027390
Whitehead S. R., Wisniewski M. E., Droby S., Abdelfattah A., Freilich S., Mazzola M. (2021). “The apple microbiome: structure, function, and manipulation for improved plant health,” in The apple genome, 341–382.
Wu S., Shi Z., Chen X., Gao J., Wang X. (2022). Arbuscular mycorrhizal fungi increase crop yields by improving biomass under rainfed condition: a meta-analysis. PeerJ 10, e12861. doi: 10.7717/peerj.12861
Yadav A., Yadav K., Ahmad R., Abd-Elsalam K. A. (2023). Emerging frontiers in nanotechnology for precision agriculture: Advancements, hurdles and prospects. Agrochemicals 2, 220–256. doi: 10.3390/agrochemicals2020016
Yan A., Wang Y., Tan S. N., Mohd Yusof M. L., Ghosh S., Chen Z. (2020). Phytoremediation: a promising approach for revegetation of heavy metal-polluted land. Front. Plant Sci. 11, 359. doi: 10.3389/fpls.2020.00359
Yang N., Zhang W., Wang D., Cao D., Cao Y., He W., et al. (2023). A novel endophytic fungus strain of Cladosporium: its identification, genomic analysis, and effects on plant growth. Front. Microbiol. 14, 1287582. doi: 10.3389/fmicb.2023.1287582
Yao X., Guo H., Zhang K., Zhao M., Ruan J., Chen J. (2023). Trichoderma and its role in biological control of plant fungal and nematode disease. Front. Microbiol. 14, 1160551. doi: 10.3389/fmicb.2023.1160551
Yeh Y. W., Chou P. Y., Hou H. Y., Kirschner R. (2021). First records of powdery mildew fungi (Erysiphales) on medicinal plants in Taiwan. Bot. Stud. 62, 1–12. doi: 10.1186/s40529-020-00307-0
Yu H. Y., He W. X., Zou Y. N., Alqahtani M. D., Wu Q. S. (2024). Arbuscular mycorrhizal fungi and rhizobia accelerate plant growth and N accumulation and contribution to soil total N in white clover by difficultly extractable glomalin-related soil protein. Appl. Soil Ecol. 197, 105348. doi: 10.1016/j.apsoil.2024.105348
Yu L., Zhang W., Geng Y., Liu K., Shao X. (2022). Cooperation with arbuscular mycorrhizal fungi increases plant nutrient uptake and improves defenses against insects. Front. Ecol. Evol. 10, 833389. doi: 10.3389/fevo.2022.833389
Zakaria L. (2023). Fusarium species associated with diseases of major tropical fruit crops. Horticulturae 9, p.322. doi: 10.3390/horticulturae9030322
Zeilinger S., Gupta V. K., Dahms T. E., Silva R. N., Singh H. B., Upadhyay R. S., et al. (2016). Friends or foes? Emerging insights from fungal interactions with plants. FEMS Microbiol. Rev. 40, pp.182–pp.207. doi: 10.1093/femsre/fuv045
Zhang K., Bonito G., Hsu C. M., Hameed K., Vilgalys R., Liao H. L. (2020). Mortierella elongata increases plant biomass among non-leguminous crop species. Agronomy 10, p.754. doi: 10.3390/agronomy10050754
Zhang Y. D., Ji X. B., Zong J., Dai X. F., Klosterman S. J., Subbarao K. V., et al. (2024). Functional analysis of the mating type genes in Verticillium dahliae. BMC Biol. 22, pp.1–pp14. doi: 10.1186/s12915-024-01900-6
Zhou M. (2023). Management of Fusarium basal rot disease of onion (Allium cepa L.) By using plant growth promoting rhizobacteria in seaweed formulation (Doctoral dissertation).
Zhou R. Q., Jin J. J., Li Q. M., Su Z. Z., Yu X. J., Tang Y., et al. (2019). Early detection of magnaporthe oryzae-infected barley leaves and lesion visualization based on hyperspectral imaging. Front. Plant Sci. 9, 1962. doi: 10.3389/fpls.2018.01962
Zin N. A., Badaluddin N. A. (2020). Biological functions of Trichoderma spp. for agriculture applications. Ann. Agric. Sci. 65, 168–178. doi: 10.1016/j.aoas.2020.09.003
Keywords: fungi-plant interface, mycorrhizal relationship, restoration ecology, mutualism, antagonism
Citation: Mishra S, Srivastava A, Singh A, Pandey GC and Srivastava G (2024) An overview of symbiotic and pathogenic interactions at the fungi-plant interface under environmental constraints. Front. Fungal Biol. 5:1363460. doi: 10.3389/ffunb.2024.1363460
Received: 30 December 2023; Accepted: 22 August 2024;
Published: 25 October 2024.
Edited by:
Soumyadev Sarkar, Arizona State University, United StatesReviewed by:
Sudeep Tiwari, University of Nebraska-Lincoln, United StatesDharmendra Kumar, Central Potato Research Institute (ICAR), India
Copyright © 2024 Mishra, Srivastava, Singh, Pandey and Srivastava. This is an open-access article distributed under the terms of the Creative Commons Attribution License (CC BY). The use, distribution or reproduction in other forums is permitted, provided the original author(s) and the copyright owner(s) are credited and that the original publication in this journal is cited, in accordance with accepted academic practice. No use, distribution or reproduction is permitted which does not comply with these terms.
*Correspondence: Girish Chandra Pandey, Z2lyaXNoY2hhbmRyYXBhbmRleUBiYW5hc3RoYWxpLmlu; Garima Srivastava, Z2FyaW1hc3JpdmFzdGF2YUBiYW5hc3RoYWxpLmlu