- 1Department of Marine Sciences and Applied Biology, Laboratory of Plant Pathology, University of Alicante, Alicante, Spain
- 2Centro de Biotecnología y Genómica de Plantas (CBGP, UPM-INIA), Universidad Politécnica de Madrid (UPM)—Instituto Nacional de Investigación y Tecnología Agraria y Alimentaria (INIA), Madrid, Spain
Plants are exposed to large number of threats caused by herbivores and pathogens which cause important losses on crops. Plant pathogens such as nematodes can cause severe damage and losses in food security crops worldwide. Chemical pesticides were extendedly used for nematode management. However, due to their adverse effects on human health and the environment, they are now facing strong limitations by regulatory organisations such as EFSA (European Food Safety Authority). Therefore, there is an urgent need for alternative and efficient control measures, such as biological control agents or bio-based plant protection compounds. In this scenario, chitosan, a non-toxic polymer obtained from seafood waste mainly, is becoming increasingly important. Chitosan is the N-deacetylated form of chitin. Chitosan is effective in the control of plant pests and diseases. It also induces plants defence mechanisms. Chitosan is also compatible with some biocontrol microorganisms mainly entomopathogenic and nematophagous fungi. Some of them are antagonists of nematode pests of plants and animals. The nematophagous biocontrol fungus Pochonia chlamydosporia has been widely studied for sustainable management of nematodes affecting economically important crops and for its capability to grow with chitosan as only nutrient source. This fungus infects nematode eggs using hyphal tips and appressoria. Pochonia chlamydosporia also colonizes plant roots endophytically, stimulating plant defences by induction of salicylic and jasmonic acid biosynthesis and favours plant growth and development. Therefore, the combined use of chitosan and nematophagous fungi could be a novel strategy for the biological control of nematodes and other root pathogens of food security crops.
Rhizosphere microbiota and plant health
Ecosystems are largely conditioned by the interaction that plants have with soil-dwelling microorganisms, both beneficial and detrimental (Soliveres and García Palacios, 2019). Approximately 108-1010 microbial cells can be found in one gram of soil (Zhang et al., 2017). These complex communities are made up of viruses and various species of archaea, bacteria, and fungi. Soil health depends on biological, as well as physical and chemical factors. The addition of chemical pesticides for extensive agricultural production may compromise the balance between the above factors and damage soil microbial biodiversity (Roesch et al., 2007). Therefore, understanding rhizosphere interactions is essential to design sustainable agricultural strategies respectfully with soil health (Custódio et al., 2022).
Plants rely on other organisms to promote their growth. Beneficial organisms and mutualists include mycorrhizae and endophytic fungi and bacteria (Klein et al., 2007). These organisms increase crop yield (van der Heijden et al., 1998). On the other hand, phytophagous (insects and other herbivores) and pathogens damage plant health (Oerke, 2006). Roots modify the rhizospheric microbiota by secreting organic compounds (mainly secondary metabolites and hormones) in exudates or releasing dead cells (Wardle et al., 2004). Additionally, the soil microbial community can synthesize volatile organic compounds (VOCs) that interact with the multifactorial network of an agroecosystem either to repel pests and diseases or to attract beneficial microorganisms for biological control (Figure 1; Lozano-Soria et al., 2020). Crop monocultures may increase disease severity in soils affected by pathogens (Khan and Siddiqui, 2017). Soil microbiota plays a crucial role in the severity of pathogen infections. Thus, if the soil microbial community is not beneficial to a plant, it can increase its susceptibility to disease (Figure 2). If a plant grows in association with a rich microbiome over a period co-evolves with it (Figure 2A). Under these conditions a complex soil microbiota prevents plant disease (niche exclusion, antibiosis, parasitism/hyperparasitim). Non-specific fumigants may damage soil microbiota and generate poor soils (Figure 2B). A poor soil microbiome increases plant susceptibility to pathogen invasion (Figure 2C). On the other hand, when soil is treated with organic matter and pest-specific fumigants, the richness of the microbiome is preserved or even increased. This reduces plant susceptibility to pathogen infection even with pathogens present in soil (Figure 2D). Therefore, adaptation of the plant to a rich soil microbiome limits pathogen infection (Bakker et al., 2012).
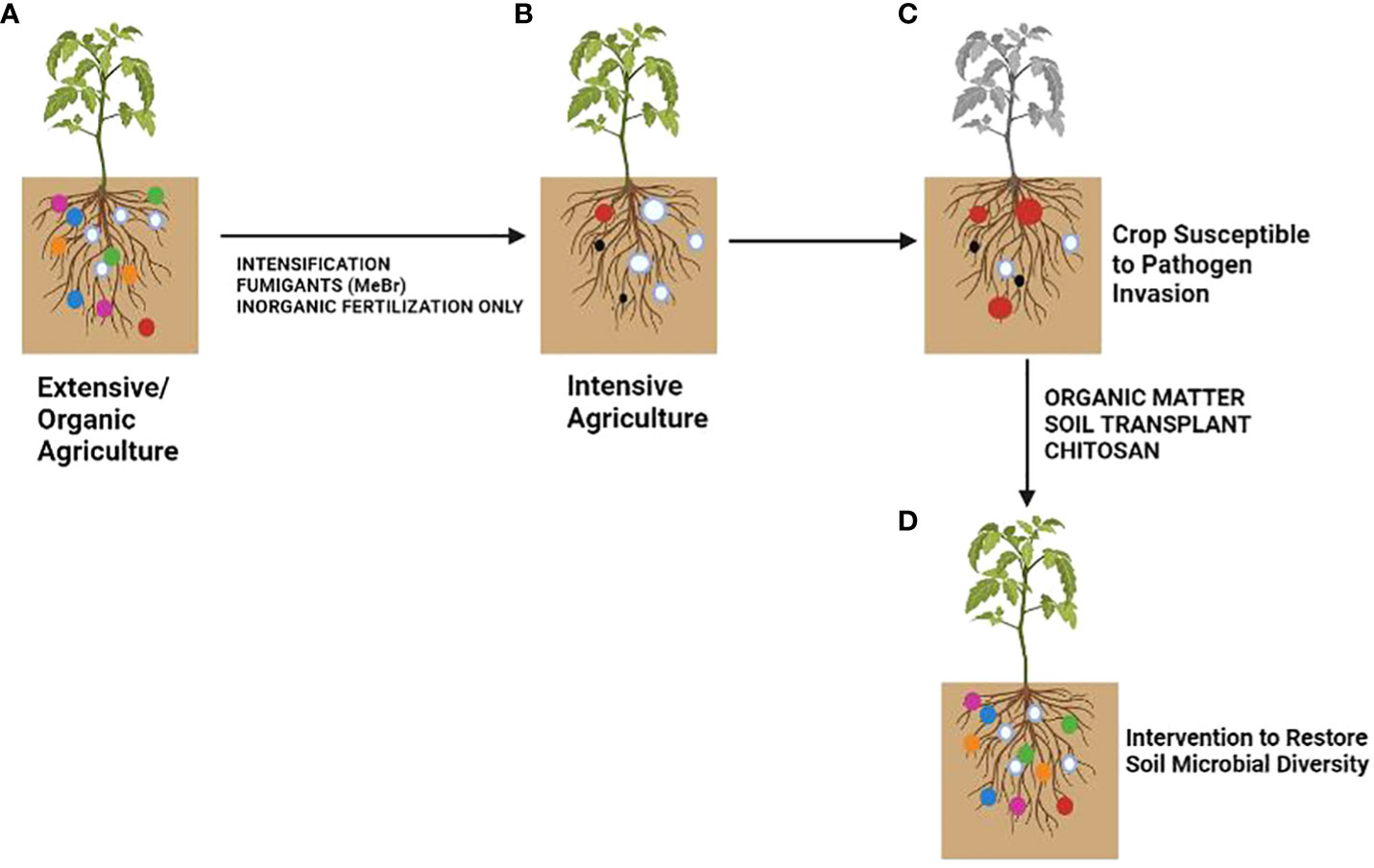
Figure 2 Effect of soil microbiota modification by agricultural practices on crop disease outcome. (A) Extensive/Organic farming, (B) Intensive farming, (C) Crops susceptible to pathogen invasion, (D) Soil after microbiota restoration. The colored circles represent different soil microbial taxa. The size represents the amount of inoculum. Pathogenic taxa are indicated by red circles. Modified from Bakker et al. (2012).
In recent years, agricultural practices have been intensified due to an increased global demand for food, which requires high crop yields. Agricultural intensive practices have a negative impact on soil health, reducing soil fertility and biodiversity, which increases activity of plant pathogens, makes them resistant to chemicals, generates environmental pollution, or soil erosion (Babin et al., 2021).
A healthy soil can keep a proper balance with the environment, sustaining biological productivity and supporting soil micro- and macro-organisms (Larkin, 2015).
Restoring damaged soils is essential to preserve crop quality. Therefore, appropriate agricultural practices must be applied to ensure crop yields for future generations. Establishing an adequate soil microbiota is essential to maintain health of agricultural systems (Sharma et al., 2020). A better mechanistic understanding of rhizosphere interactions can provide new approaches to economically efficient and sustainable plant production (Bakker et al., 2012) while preserving soil biodiversity (Custódio et al., 2022).
Pathogens threaten global food security
Viruses, Bacteria, Fungi, Nematodes, Protozoa, Algae, and Arthropods can be found in any rhizosphere ecosystem (Mendes et al., 2013). Some of them can cause plant diseases, e.g., root-knot nematodes or cereal take-all caused by the fungus Gaeumannomyces tritici. Plants have evolved defence strategies against pest and pathogens. They can, for instance, recognise microbe-associated molecular patterns (MAMPs) and react to them by secreting degradative enzymes or secondary metabolites (Zhou and Zhang, 2020). Plants can also assemble microbial communities to promote pathogen suppression in the rhizosphere (Dudenhöffer et al., 2016). However, these strategies are not always successful. Pathogens have evolved to circumvent plant defences, for instance by secreting effectors (Lo Presti et al., 2015). It has been estimated that ca. 40% of global crop production is lost to pests and diseases (FAO, 2021). At least $ 80 billion is lost annually because of plant pathogenic nematodes (Palomares-Rius et al., 2021). Considering the growing population of our Planet (United Nations, 2019) and the reduction of fertile soils due to extensive non-agricultural land use and climate change (Borrelli et al., 2020), avoiding these crop losses due to pests is crucial. Reducing pests and diseases of economically important crops sustainably can help improve the quality of life for farmers, businesses and consumers while respecting the environment.
Plant pathogenic nematodes can cause severe losses in a wide range of crops, e.g., Meloidogyne spp. in tomato and banana crops. (Nicol et al., 2011; Silva et al., 2017). These pathogens use living plant resources to grow, compromising crop viability and making nutrient transport difficult leading to plant nutrition deficiency (Abdulkhair and Alghuthaymi, 2016). Chemical nematicides are highly toxic to both the soil and human health (Abawi and Widmer, 2000). Methyl Bromide (MeBr), a soil fumigant, has been used as a nematicide worldwide. MeBr causes ozone layer depletion, so its use has been progressively reduced, and is now completely banned (Nahed et al., 2017). In 1999, the EPA (Environmental Protection Agency) phased out the use of MeBr, except for critical allowable uses agreed in the Montreal Protocol (EPA, 2022). The use of MeBr was revoked in the EU in 2010 (CEE, 2018). Likewise chemical nematicides such as Nemacur® (composed of fenamiphos) and Carbofuran have also been banned for crops. Use of Dichloropropene, a non-specific soil fumigant like MeBr (with or without chloropicrin), has been restricted to fields with no crops (MAPA, 2022). Alternative and effective biological control measures, such as biological control agents or plant protection compounds of biological origin, as well as improved agricultural practices to minimise crop losses are urgently needed as alternatives to chemical control for nematode management (Sikder and Vestergård, 2020).
Nematode antagonists: biological control
Diverse organisms are natural antagonists of nematodes. For instance, Collembola and Mites predate on plant pathogenic nematodes in soils reducing their populations. In addition, bacteria such as Pasteuria spp. can efficiently suppress plant-parasitic nematodes in soils (Stirling, 2018). Nematophagous fungi are highly specialized in nematode parasitism and many of them can be cultured and mass-produced. Our knowledge of these fungi has increased from ca. 160 species described at the beginning of the century (Elshafie et al., 2006) to 700 species recently (Soares et al., 2018). Nematophagous fungi infect nematodes by various strategies and have been classified into 5 groups (Soares et al., 2018) accordingly: nematode trappers or predators, egg parasites, endoparasites, toxin-producers and those forming special attack devices (Table 1).
Some nematophagous fungi may use more than one strategy for killing nematodes. For instance, Coprinus comatus generates “spiny balls” to physically damage the nematode cuticle. Besides, this fungus produces toxins that immobilize and kill nematodes. The combination of both strategies makes nematode attack more efficient (Luo et al., 2007). However, the use of toxin-producing fungi for the management of nematodes in crops has not been widely studied because of possible “non-target” effects (Kumar, 2020). Pleurotus ostreatus has recently been shown to induce paralysis of the sensory cilia and calcium accumulation in the nematode, which results in nematode nervous system tissue necrosis (Lee et al., 2005). Brazilian mushrooms (including Pleurotus spp.) have been recently found to reduce hatching and mobility of root knot nematode Meloidogyne javanica (Hahn et al., 2019). On the other hand, the nematode immune system reacts to the presence of nematophagous fungi (Pujol et al., 2008).
Some nematophagous fungi also enhance plant growth and immunity (Escudero and Lopez-Llorca, 2012), besides their nematode parasitic abilities (Poveda et al., 2020). Arthrobotrys, Aspergillus, Catenaria, Dactylellina, Hirsutella, Paecilomyces, Pochonia, Purpureocillium and Trichoderma (Forghani and Hajihassani, 2020) are reported genera of plant parasitic nematode biocontrol agents. Pochonia and Trichoderma are good endophytes capable to promote growth, yield, nutrient uptake, and plant development (Zavala-Gonzalez et al., 2015; Mingot-Ureta et al., 2020; Tseng et al., 2020). Both fungi induce plant hormones (jasmonic acid, auxin, and gibberellin) and defence metabolites in tomato, barley, wheat, and Arabidopsis (Zavala-Gonzalez et al., 2017; Pentimone et al., 2019; Jaroszuk-Ściseł et al., 2019).
Trichoderma spp. are generalist fungi found in soil and roots. They can act as saprophytes (Rashmi et al., 2016), endophytes (Tseng et al., 2020), and pathogens of fungi and nematodes (Sahebani and Hadavi, 2008). This versatility allows them to survive well in the rhizosphere. Trichoderma can also induce systemic resistance to nematodes in plants (Medeiros et al., 2017). They are widely used for biocontrol and there are several commercial formulations available worldwide based, mainly, on T. asperellum.
Pochonia chlamydosporia has also been widely studied as a fungal parasite of eggs and females of plant parasitic nematodes, including cyst and root knot species (Meloidogyne spp). Pochonia chlamydosporia differentiates appressoria from hyphal tips that adhere to nematode eggshells, allowing penetration and infection (Lopez-Llorca et al., 2002). This process involves proteolytic and chitinolytic activities and secretion of adhesives (Tikhonov et al., 2002). P. chlamydosporia has already been used to manage nematodes in food security crops such as tomato (Escudero et al., 2017), potato (Varandas et al., 2020), soybean (Messa et al., 2020), cucumber (Swarnakumari et al., 2020), rice (Khan et al., 2021), banana (Silva et al., 2017) and root beet (Haj Nuaima et al., 2021). P. chlamydosporia has also been used for biocontrol of animal parasitic nematodes (Li et al., 2022) and can also infect insects in the laboratory, such as hemipters (Ferraz et al., 2021).
Pochonia chlamydosporia also produces compounds suitable for crop protection, such as the insecticide Chlamyphilone (3,4,7-trimethyl-6,8-dioxo-7,8-dihydro-6H-isochromen-7-yl ester; Lacatena et al., 2019) and a wide array of other secondary metabolites with potential applicability (Escudero et al., 2014; Niu, 2017; Suarez-Fernandez et al., 2020). Pochonia chlamydosporia is also a root endophyte (Macia-Vicente et al., 2008; Escudero and Lopez-Llorca, 2012; Mingot-Ureta et al., 2020) and can live in the soil as a saprophyte (Bourne et al., 1996). Such versatility allows them to cope with environmental stress (Valadão et al., 2020), which makes it adequate for field application (Sellitto et al., 2016). Pochonia chlamydosporia formulations are being developed for practical use to manage plant pests and diseases (Sellitto et al., 2016; Dalla Pasqua et al., 2020; Swarnakumari et al., 2020).
Chitosan and biological control fungi
Chitosan is a linear polymer of beta-(1-4)-linked N-acetyl-2-amino-2-deoxy-D-glucose and 2-amino-2-deoxy-D-glucose (Kaur and Dhillon, 2014). It is an environmental-friendly biodegradable molecule with interesting features. It is non-toxic to human cells (including lymphocytes; Lopez-Moya et al., 2015) and displays antimicrobial and antioxidant activities (Abd El-Hack et al., 2020). Chitosan has been applied in agriculture (Chakraborty et al., 2020), animal health (Zheng et al., 2020), food (Picciotti et al., 2021), cosmetic (Seino et al., 2020) and medical (Geanaliu-Nicolae and Andronescu, 2020) industries. Chitosan results from the chemical or enzymatic chitin deacetylation. Chitin is present in the cell wall of fungi, in the exoskeleton of arthropods (e.g., insect cuticle) and in nematode eggshells. Chitosan enhances plant growth, development and defence induction under a highly regulated application (Ghasemi Pirbalouti et al., 2017; Lopez-Moya et al., 2017). It also protects plants from infections by pathogenic fungi (Chouhan and Mandal, 2021). This may be due to its plant defence elicitor effect, which can trigger physiological and structural responses in the plant, e.g., substances such as jasmonic acid (JA) and salicylic acid (SA). These mimic the action of plant signalling molecules and produce reactive oxygen species (ROS) that stimulate the plant to produce defence hormones and enzymatic or non-enzymatic antioxidant mechanisms to mitigate ROS damage to cells (Lopez-Moya et al., 2015; Lopez-Moya et al., 2019; Suarez-Fernandez et al., 2020). To this respect, induction of plant defences by chitosan also reduces severity of root-knot nematodes (Meloidogyne) in plants (Vasyukova et al., 2003, Escudero et al., 2017).
Trichoderma spp. and P. chlamydosporia genomes encode, hydrolases such as, proteases, chitosanases and chitinases (Kappel et al., 2020). Pochonia chlamydosporia genome encodes the largest set of chitosanases found in fungi (Larriba et al., 2014; Aranda-Martinez et al., 2016). Their use in tandem with chitin deacetylases could explain the ability of the fungus to degrade nematode eggshells. (Lopez-Moya et al., 2019; Lopez-Llorca et al., 2020). Combinations of chitosan and biocontrol (entomopathogenic and nematophagous) fungi are currently developed for enhancing fungal pathogenicity and plant defence abilities. This has been reported for Beauveria bassiana (Abdullah and Sukar, 2021) and Metarhizium anisopliae (Wu et al., 2021). Nematophagous fungi have also demonstrated to be more efficient for biological control when combined with chitosan than on their own (Escudero et al., 2017; Singh and Chittenden, 2021; Kappel et al., 2022). For P. chlamydosporia, addition of chitosan increases nematode parasitism by induction of appressoria differentiation on nematode eggshell (Escudero et al., 2016 and Escudero et al., 2017).
Chitosan: Mode of action in plants and fungi
Roots in the simulation (Figure 3) are colonized by a biocontrol fungus (P. chlamydosporia; Pc) and infected by a wilt pathogenic fungus (Fusarium oxysporum f. sp. radicis-lycopersici; FORL) and root knot nematodes (RKN). Chitosan depolarises the plasma membrane of plant root cells and increases reactive oxygen species (ROS). This is linked with an increase in jasmonic acid secretion and other oxylipins in root exudates (Suarez-Fernandez et al., 2020). Furthermore, chitosan permeabilises the plasma membrane of susceptible fungi (Palma-Guerrero et al., 2010; Hernández-Lauzardo et al., 2011) by generating reactive oxygen species (ROS) which resulted toxic to the organism (Lopez-Moya et al., 2015). This would activate the phenylpropanoid acid pathway, increasing the secretion and accumulation of phenolic compounds (Xoca-Orozco et al., 2019) and plant hormones (Kwak et al., 2006), such as indoleacetic acid. Evidence for activation of the phenyl ammonia lyase (PAL) pathway is consistent with the increase in salicylic acid found in root exudates in chitosan treated plants. These hormones could also accumulate in root tissues (Lopez-Moya et al., 2017). Root exudates of chitosan-treated plants inhibit root pathogens, such as FORL or nematodes (Suarez-Fernandez et al., 2020). The nematophagous fungus P. chlamydosporia in the rhizosphere also generates ROS in response to chitosan. P. chlamydosporia alters plant metabolite secretion to establish endophytic interactions enhancing plant defences (de Souza Gouveia et al., 2022). The fungal cell secretes chitosanases to degrade chitosan and increases the synthesis of sugar transporters to assimilate the catabolites of chitosan depolymerisation (Suarez-Fernandez et al., 2021). Alternative splicing events occur in P. chlamydosporia chitosanase encoding genes (e.g., csn3), probably to increase the efficiency of chitosan degradation (Sambles et al., 2022). When nematode eggs are present in the system, chitosan induces genes and proteins involved in adhesion, eggshell degradation, and parasitism (Escudero et al., 2016; Suarez-Fernandez et al., 2021). Increased secretion of peptidases or LysM effectors from the fungus could also be related to increased endophytism of the fungus in the plant root, favouring this fungal lifestyle and effective protection against nematodes and other plant pathogens. To summarize, the elicitor effect of chitosan on plant defences, as well as the enhancement of proteases and adhesives in P. chlamydosporia nematode egg infection, support the combined use of biocontrol fungi and chitosan for plant protection to important pests and diseases.
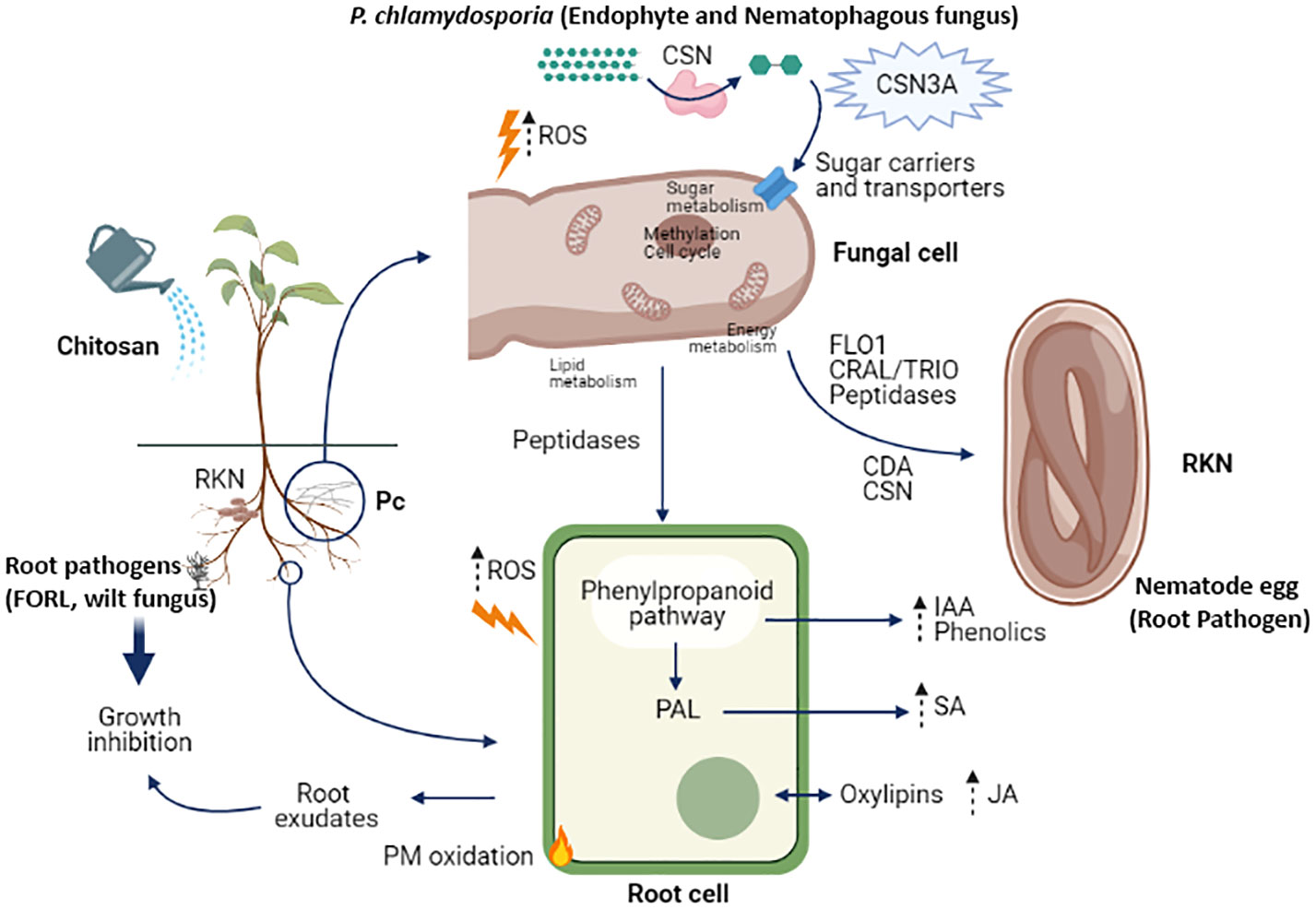
Figure 3 Cell and molecular effects of chitosan in the rhizosphere. Abbreviations: RKN (root-knot nematodes), FORL (Fusarium oxysporum f. sp. radicis lycopersici), ROS (reactive oxygen species), CSN (chitosanase), CSN3A (chitosanase 3 isoform A), CDA (chitin deacetylase), IAA (Indole-3-acetic acid), SA (salicylic acid), JA (jasmonic acid), PAL (phenyl ammonia lyase), PM (plasma membrane).
Application of chitosan nanoemulsions and nematophagous fungi for nematode management
Natural plant protection compounds with low environmental toxicity have a clear niche in agrobiotechnology. Some biocontrol agents such as P. chlamydosporia or B. bassiana are enhanced by chitosan which also acts as a plant defence elicitor. Due to the ban on many chemical plant protection compounds, chitosan-derived products started to be used in 1990 against plant diseases in crops and during post-harvest (Yin and Du, 2011). Recently, the European Union has recognised chitosan as an active compound suitable for agricultural use. Most plant protection products are hydrophobic, so their formulation requires the use of chemicals to keep their physicochemical properties (Liang et al., 2018). Nanoemulsions are small droplets dispersed in an immiscible fluid using a suitable emulsifier. They have recently generated interest in agrochemical fields due to the improved bioavailability of poorly water-soluble pesticides (Chadha, 2021). Zn-Chitosan nanoparticles (microscopic particles encapsulating a compound), reduce the severity of infection of Curvularia lunata infecting maize leaves, and Zn-chitosan also enhances the growth of nanoparticulate maize seedlings (Saharan et al., 2016; Choudhary et al., 2019).
Chitosan nanoparticles can also be used as coating agents for post-harvest disease prevention. Ripening bananas coated with 0.2% chitosan nanoparticles show a slower skin discolouration than untreated controls (Esyanti et al., 2019).
P. chlamydosporia can reduce damage caused by M. incognita on tomato plants in a single application (Yang et al., 2012). Chitosan has been combined with P. chlamydosporia for root knot nematode management in tomato (Escudero et al., 2017). Plants treated with chitosan had fewer galls and reduced nematode multiplication compared to controls. Pochonia chlamydosporia was the only fungus identified parasitizing nematode eggs in chitosan-treated soils. Soils in fields with root knot nematodes and P. chlamydosporia irrigated with chitosan showed 36.6% egg parasitism compared to 29.3% in non-irrigated and non-sterilised soils (Escudero et al., 2017). Soils in which P. chlamydosporia is naturally present treated with chitosan are expected to show increased colonisation of plant roots and increased parasitism of eggs by the fungus. This strategy could sustainably reduce the population of plant-parasitic nematodes in agroecosystems.
Our challenge would be to formulate chitosan and P. chlamydosporia in a suitable way for creating de novo nematode suppression in agroecosystems. Such studies are underway in our laboratory.
The application of microparticles containing chemicals and biological agents is an innovative approach for plant pests and diseases management (Jurić et al., 2021). Encapsulation of biocontrol agents in chitosan microparticles ensures their protection, survival, and selective delivery for pest management. Combining liquid or particulate chitosan formulations with the nematophagous fungus P. chlamydosporia is an effective way to recycle waste from seafood industries while generating a sustainable way for managing nematode infections in the field.
Conclusions and future prospects
Nematophagous fungi in combination with chitosan represents a new solution for sustainable management of plants parasitic nematodes which cause significant economic losses in crops worldwide. Understanding molecular interactions between organisms in the rhizosphere is essential to determine the best approach to manage crop pathogens without harming agroecosystems. The combination of chitosan with nematophagous fungi still needs to be explored further to determine the best formulation and timing for field applications, but it constitutes a promising tool for the sustainable management of pests and diseases of food security crops.
Author contributions
RL-N and MS-F conducted the bibliographic search, organised, and summarised the information, and wrote the manuscript draft. Supervision, methodology, co-writing (original draft), and reviewing: FL-M. Supervision, methodology, co-writing (original draft), reviewing and funding acquisition: LL-L. All authors have read and agreed to the published version of the manuscript.
Funding
This research was funded by PID2020-119734RB-I00 Project from the Spanish Ministry of Science and Innovation and by European Project H2020 MUSA no. 727624.
Acknowledgments
We would like to thank the Plant Pathology Laboratory members of the University of Alicante for their help and support.
Conflict of interest
The authors declare that the research was conducted in the absence of any commercial or financial relationships that could be construed as a potential conflict of interest.
Publisher’s note
All claims expressed in this article are solely those of the authors and do not necessarily represent those of their affiliated organizations, or those of the publisher, the editors and the reviewers. Any product that may be evaluated in this article, or claim that may be made by its manufacturer, is not guaranteed or endorsed by the publisher.
References
Abawi G. S., Widmer T. L. (2000). Impact of soil health management practices on soilborne pathogens, nematodes and root diseases of vegetable crops. Appl. Soil Ecol. 15, 37–47. doi: 10.1016/S0929-1393(00)00070-6
Abd El-Hack M. E., El-Saadony M. T., Shafi M. E., Zabermawi N. M., Arif M., Batiha G. E., et al. (2020). Antimicrobial and antioxidant properties of chitosan and its derivatives and their applications: A review. Int. J. Biol. Macromol. 164, 2726–2744. doi: 10.1016/j.ijbiomac.2020.08.153
Abdulkhair W. M., Alghuthaymi M. A. (2016) Plant pathogens in Plant growth Ed. Rigobelo E. C.. doi: 10.5772/65325
Abdullah R. R., Sukar N. A (2021). Enhancing the efficacy of the biopesticide Beauveria bassiana by adding chitosan to its secondary metabolites. International Journal of Entomology Research 6 (1), 30–35.
Aranda-Martinez A., Lenfant N., Escudero N., Zavala-Gonzalez E. A., Henrissat B., Lopez-Llorca L. V. (2016). CAZyme content of Pochonia chlamydosporia reflects that chitin and chitosan modification are involved in nematode parasitism: CAZome of Pochonia chlamydosporia. Environ. Microbiol. 18, 4200–4215. doi: 10.1111/1462-2920.13544
Babin D., Leoni C., Neal A. L., Sessitsch A., Smalla K. (2021). Editorial to the thematic topic “Towards a more sustainable agriculture through managing soil microbiomes.”. FEMS Microbiol. Ecol. 97 (8), fiab094. doi: 10.1093/femsec/fiab094
Bakker M. G., Manter D. K., Sheflin A. M., Weir T. L., Vivanco J. M. (2012). Harnessing the rhizosphere microbiome through plant breeding and agricultural management. Plant Soil 360, 1–13. doi: 10.1007/s11104-012-1361-x
Borrelli P., Robinson D. A., Panagos P., Lugato E., Yang J. E., Alewell C., et al. (2020). Land use and climate change impacts on global soil erosion by water 2015-2070). Proc. Natl. Acad. Sci. U.S.A. 117, 21994–22001. doi: 10.1073/pnas.2001403117
Bourne J. M., Kerry B. R., De Leij F. A. A. M. (1996). The importance of the host plant on the interaction between root-knot nematodes (Meloidogyne spp.) and the nematophagous fungus, Verticillium chlamydosporium Goddard. Biocontrol Sci. Tech. 6, 539–548. doi: 10.1080/09583159631172
CEE (2018)2008/753/EC: Commission decision of 18 September 2008 concerning the non-inclusion of methyl bromide in annex I to council directive 91/414/EEC and the withdrawal of authorisations for plant protection products containing that substance (notified under document number c, (2008) 5076) (Text with EEA relevance). Available at: https://eur-lex.europa.eu/legal-content/EN/ALL/?uri=CELEX%3A32008D0753 (Accessed February 21, 2022).
Chadha S. (2021). “Recent advances in nano-encapsulation technologies for controlled release of biostimulants and antimicrobial agents,” in Jogaiah S., Bahadur-Singh S., Fernandes-Fraceto L., de Lima R (eds). Advances in nano-fertilizers and nano-pesticides in agriculture (Elsevier), 29–55. doi: 10.1016/B978-0-12-820092-6.00002-1
Chakraborty M., Hasanuzzaman M., Rahman M., Khan Md. A. R., Bhowmik P., Mahmud N. U., et al. (2020). Mechanism of plant growth promotion and disease suppression by chitosan biopolymer. Agriculture 10, 624. doi: 10.3390/agriculture10120624
Choudhary R. C., Kumaraswamy R. V., Kumari S., Sharma S. S., Pal A., Raliya R., et al. (2019). Zinc encapsulated chitosan nanoparticle to promote maize crop yield. Int. J. Biol. Macromol. 127, 126–135. doi: 10.1016/j.ijbiomac.2018.12.274
Chouhan D., Mandal P. (2021). Applications of chitosan and chitosan based metallic nanoparticles in agrosciences-a review. Int. J. Biol. Macromol. 166, 1554–1569. doi: 10.1016/j.ijbiomac.2020.11.035
Custódio V., Gonin M., Stabl G., Bakhoum N., Oliveira M. M., Gutjahr C., et al. (2022). Sculpting the soil microbiota. Plant J. 109, 508–522. doi: 10.1111/tpj.15568
Dalla Pasqua S., Dallemole-Giaretta R., dos Santos I., Reiner D. A., Lopes E. A. (2020). Combined application of Pochonia chlamydosporia and solid by-product of the wine industry for the control of Meloidogyne javanica. App Soil Ecol. 147, 103397. doi: 10.1016/j.apsoil.2019.103397
de Souza Gouveia A., Avelar Monteiro T. S., Dionísio Luiz P. H., Marcio Balbino H., Magalhães F. C., Silva de Moura V. A., et al. (2022). The nematophagous root endophyte Pochonia chlamydosporia alters tomato metabolome. Rhizosphere 22, 100531. doi: 10.1016/j.rhisph.2022.100531
Dudenhöffer J., Scheu S., Jousset A. (2016). Systemic enrichment of antifungal traits in the rhizosphere microbiome after pathogen attack. J. Ecol. 104, 1566–1575. doi: 10.1111/1365-2745.12626
Elshafie A. E., Al-Mueini R., Abdulaziz S. N. A.-B., Akindi Y., Mahmoud I., Al-Rawahi S. H. (2006) Diversity and trapping efficiency of nematophagous fungi from Oman. Phytopathol. Medit. 45, 266–270. doi: 10.14601/Phytopathol_Mediterr-1835
EPA (2022). Available at: https://web.archive.org/web/20091017081855/http://www.epa.gov/spdpublc/mbr (Accessed February 21, 2022).
Escudero N., Ferreira S. R., Lopez-Moya F., Naranjo-Ortiz M. A., Marin-Ortiz A. I., Thornton C. R., et al. (2016). Chitosan enhances parasitism of Meloidogyne javanica eggs by the nematophagous fungus Pochonia chlamydosporia. Fung. Biol. 120 (4), 572–585. doi: 10.1016/j.funbio.2015.12.005
Escudero N., Lopez-Llorca L. V. (2012). Effects on plant growth and root-knot nematode infection of an endophytic GFP transformant of the nematophagous fungus Pochonia chlamydosporia. Symbiosis 57 (1), 33–42. doi: 10.1007/s13199-012-0173-3
Escudero N., Lopez-Moya F., Ghahremani Z., Zavala-Gonzalez E. A., Alaguero-Cordovilla A., Ros-Ibañez C., et al. (2017). Chitosan increases tomato root colonization by Pochonia chlamydosporia and their combination reduces root-knot nematode damage. Front. Plant Sci. 81415. doi: 10.3389/fpls.2017.01415
Escudero N., Marhuenda-Egea F. C., Ibanco-Cañete R., Zavala-González E. A., Lopez-Llorca L. V. (2014). A metabolomic approach to study the rhizodeposition in the tritrophic interaction: tomato, Pochonia chlamydosporia and Meloidogyne javanica. Metabolomics 10 (5), 788–804. doi: 10.1007/s11306-014-0632-3
Esyanti R. R., Zaskia H., Amalia A. (2019). Chitosan nanoparticle-based coating as post-harvest technology in banana. in journal of physics: Conference series. IOP Publishing. Vol. 1204, No. 1, 12109. doi: 10.1088/1742-6596/1204/1/012109
FAO (2021)News article: Climate change fans spread of pests and threatens plants and crops, new FAO study. Available at: https://www.fao.org/news/story/en/item/1402920/icode/ (Accessed February 21, 2022).
Ferraz C. M., Soares F. E. F., Senna C. C., Silva L. P. C., Araújo J. V., Moreira T. F., et al. (2021). Interaction of the nematophagous fungus Pochonia chlamydosporia on eggs of Spartocera dentiventris (Berg) (Hemiptera: Coreidae) under laboratory conditions. Braz. J. Biol. 81, 1122–1124. doi: 10.1590/1519-6984.231550
Flores Francisco B. G., Ponce I. M., Plascencia Espinosa M.Á., Mendieta Moctezuma A., López y López V. E. (2021). Advances in the biological control of phytoparasitic nematodes via the use of nematophagous fungi. J. Microbiol. Biotechnol. 37 (10), 1–14. doi: 10.1007/s11274-021-03151-x
Forghani F., Hajihassani A. (2020). Recent advances in the development of environmentally benign treatments to control root-knot nematodes. Front Plant Sci. 111125. doi: 10.3389/fpls.2020.01125
Geanaliu-Nicolae R.-E., Andronescu E. (2020). Blended natural support materials–collagen based hydrogels used in biomedicine. Materials 13 (24)5641. doi: 10.3390/ma13245641
Ghasemi Pirbalouti A., Malekpoor F., Salimi A., Golparvar A. (2017). Exogenous application of chitosan on biochemical and physiological characteristics, phenolic content and antioxidant activity of two species of basil (Ocimum ciliatum and Ocimum basilicum) under reduced irrigation. Sci. Hortic. 217, 114–122. doi: 10.1016/j.scienta.2017.01.031
Hahn M. H., De Mio L. L. M., Kuhn O. J., Duarte H. D. S. S. (2019). Nematophagous mushrooms can be an alternative to control Meloidogyne javanica. Biol. Control. 138, 104024. doi: 10.1016/j.biocontrol.2019.104024
Haj Nuaima R., Ashrafi S., Maier W., Heuer H. (2021). Fungi isolated from cysts of the beet cyst nematode parasitized its eggs and counterbalanced root damages. J. Pest Sc.,i 94, 563–572. doi: 10.1007/s10340-020-01254-2
Hernández-Lauzardo A. N., Vega-Pérez J., Velázquez-del Valle M. G., Sánchez N. S., Peña A., Guerra-Sánchez G. (2011). Changes in the functionality of plasma membrane of Rhizopus stolonifer by addition of chitosan: Change in plasma membrane of R. stolonifer by chitosan. J. Phytopathol. 159 (7-8), 563–568. doi: 10.1111/j.1439-0434.2011.01802.x
Jaroszuk-Ściseł J., Tyśkiewicz R., Nowak A., Ozimek E., Majewska M., Hanaka A., et al. (2019). Phytohormones (Auxin, gibberellin) and ACC deaminase In vitro synthesized by the mycoparasitic Trichoderma DEMTkZ3A0 strain and changes in the level of auxin and plant resistance markers in wheat seedlings inoculated with this strain conidia. Int. J. Mol. Sci. 20, E4923. doi: 10.3390/ijms20194923
Jurić S., Jurić M., Režek Jambrak A., Vinceković M. (2021). Tailoring Alginate/Chitosan microparticles loaded with chemical and biological agents for agricultural application and production of value-added foods. Appl. Sci. 11 (9), 4061. doi: 10.3390/app11094061
Kappel L., Kosa N., Gruber S. (2022). The multilateral efficacy of chitosan and Trichoderma on sugar beet. J. Fungi 8, 137. doi: 10.3390/jof8020137
Kappel L., Münsterkötter M., Sipos G., Escobar Rodriguez C., Gruber S. (2020). Chitin and chitosan remodeling defines vegetative development and Trichoderma biocontrol. PloS Pathog. 16, e1008320. doi: 10.1371/journal.ppat.1008320
Kaur S., Dhillon G. S. (2014). The versatile biopolymer chitosan: potential sources, evaluation of extraction methods and applications. Crit. Rev. Microbiol. 40, 155–175. doi: 10.3109/1040841X.2013.770385
Khan M. R., Haque Z., Ahamad F., Zaidi B. (2021). Biomanagement of rice root-knot nematode Meloidogyne graminicola using five indigenous microbial isolates under pot and field trials. J. Appl. Microbiol. 130, 424–438. doi: 10.1111/jam.14788
Khan M., Siddiqui Z. A. (2017). Effect of simultaneous and sequential inoculations of Meloidogyne incognita, Ralstonia solanacearum and Phomopsis vexans on eggplant in sand mix and fly ash mix soil. Phytoparasitica 45, 599–609. doi: 10.1007/s12600-017-0613-y
Klein A.-M., Vaissière B. E., Cane J. H., Steffan-Dewenter I., Cunningham S. A., Kremen C., et al. (2007). Importance of pollinators in changing landscapes for world crops. Proc. R. Soc B. 274, 303–313. doi: 10.1098/rspb.2006.3721
Kumar K. K. (2020). “Fungi: A bio-resource for the control of plant parasitic nematodes,” in Agriculturally important fungi for sustainable agriculture (Cham: Springer), 285–311. doi: 10.1007/978-3-030-48474-3_10
Kwak J. M., Nguyen V., Schroeder J. I. (2006). The role of reactive oxygen species in hormonal responses. Plant Phys. 141 (2), 323–329. doi: 10.1104/pp.106.079004
Lacatena F., Marra R., Mazzei P., Piccolo A., Digilio M., Giorgini M., et al. (2019). Chlamyphilone, a novel Pochonia chlamydosporia metabolite with insecticidal activity. Molecules 24, 750. doi: 10.3390/molecules24040750
Larkin R. P. (2015). Soil health paradigms and implications for disease management. Annu. Rev. Phytopathol. 53, 199–221. doi: 10.1146/annurev-phyto-080614-120357
Larriba E., Jaime M. D., Carbonell-Caballero J., Conesa A., Dopazo J., Nislow C., et al. (2014). Sequencing and functional analysis of the genome of a nematode egg-parasitic fungus, pochonia chlamydosporia. Fungal Genet. Biol. 65, 69–80. doi: 10.1016/j.fgb.2014.02.002
Lee Y.-S., Kim Y., ng-Ho, Kim S.-B. (2005). Changes in the respiration, growth, and vitamin c content of soybean sprouts in response to chitosan of different molecular weights. HortScience 40 (5), 1333–1335. doi: 10.21273/HORTSCI.40.5.1333
Liang W., Yu A., Wang G., Zheng F., Jia J., Xu H. (2018). Chitosan-based nanoparticles of avermectin to control pine wood nematodes. Int. J. Biol. Macromol. 112, 258–263. doi: 10.1016/j.ijbiomac.2018.01.174
Li S., Wang D., Gong J., Zhang Y. (2022). Individual and combined application of nematophagous fungi as biological control agents against gastrointestinal nematodes in domestic animals. Pathogens 11, 172. doi: 10.3390/pathogens11020172
Li Y., Yu H., Araújo J. P., Zhang X., Ji Y., Hulcr J. (2021). Esteya floridanum sp. nov.: An ophiostomatalean nematophagous fungus and its potential to control the pine wood nematode. Phytopathology 111 (2), 304–311. doi: 10.1094/PHYTO-06-20-0229-R
Lopez-Llorca L. V., Hernandez-Valdivia I., Lopez-Moya F. (2020). “Chitosan biosynthesis and degradation: A way to modulate plant defenses in endophytic biocontrol agents?,” inDe Cal A., Melgarejo P., Magan N. (eds). How research can stimulate the development of commercial biological control against plant disease. Progress in Biological Control (Cham:Springer) 21, 109–125. doi: 10.1007/978-3-030-53238-3_8
Lopez-Llorca L. V., Olivares-Bernabeu C., Salinas J., Jansson H.-B., Kolattukudy P. E. (2002). Pre-penetration events in fungal parasitism of nematode eggs. Mycol. Res. 106, 499–506. doi: 10.1017/S0953756202005798
Lopez-Moya F., Colom-Valiente M. F., Martinez-Peinado P., Martinez-Lopez J. E., Puelles E., Sempere-Ortells J. M., et al. (2015). Carbon and nitrogen limitation increase chitosan antifungal activity in Neurospora crassa and fungal human pathogens. Fung. Biol. 119 (2-3), 154–169. doi: 10.1016/j.funbio.2014.12.003
Lopez-Moya F., Escudero N., Zavala-Gonzalez E. A., Esteve-Bruna D., Blázquez M. A., Alabadí D., et al. (2017). Induction of auxin biosynthesis and WOX5 repression mediate changes in root development in Arabidopsis exposed to chitosan. Sci. Rep. 7 (1), 16813. doi: 10.1038/s41598-017-16874-5
Lopez-Moya F., Suarez-Fernandez M., Lopez-Llorca L. (2019). Molecular mechanisms of chitosan interactions with fungi and plants. Int. J. Mol. Sci. 20, 332. doi: 10.3390/ijms20020332
Lo Presti L., Lanver D., Schweizer G., Tanaka S., Liang L., Tollot M., et al. (2015). Fungal effectors and plant susceptibility. Annu. Rev. Plant Biol. 66, 513–545. doi: 10.1146/annurev-arplant-043014-114623
Lozano-Soria A., Picciotti U., Lopez-Moya F., Lopez-Cepero J., Porcelli F., Lopez-Llorca L. V. (2020). Volatile organic compounds from entomopathogenic and nematophagous fungi, repel banana black weevil (Cosmopolites sordidus). Insects 11, 509. doi: 10.3390/insects11080509
Luo H., Liu Y., Fang L., Li X., Tang N., Zhang K. (2007). Coprinus comatus damages nematode cuticles mechanically with spiny balls and produces potent toxins to immobilize nematodes. Appl. Environ. Microbiol. 73, 3916–3923. doi: 10.1128/AEM.02770-06
Macia-Vicente J. G., Jansson H. B., Mendgen K., Lopez-Llorca L. V. (2008). Colonization of barley roots by endophytic fungi and their reduction of take-all caused by Gaeumannomyces graminis var. tritici. Can. J. Microbiol. 54 (8), 600–609. doi: 10.1139/W08-047
MAPA (2022)(Ministerio de agricultura, pesca y alimentación) reglamento (CE) n° 1107/2009 del parlamento europeo y del consejo, de 21 de octubre de 2009, relativo a la comercialización de productos fitosanitarios y por el que se derogan las directivas 79/117/CEE y 91/414/CEE del consejo. Available at: https://www.mapa.gob.es/agricultura/pags/fitos/registro/fichas/pdf/Lista_Sustancias_activas_aceptadas_excluidas.pdf (Accessed February 21, 2022).
Medeiros H. A., de Araújo Filho J. V., de Freitas L. G., de Castillo P., Rubio M. B., Hermosa R., et al. (2017). Tomato progeny inherit resistance to the nematode Meloidogyne javanica linked to plant growth induced by the biocontrol fungus Trichoderma atroviride. Sci. Rep. 7, 40216. doi: 10.1038/srep40216
Mendes R., Garbeva P., Raaijmakers J. M. (2013). The rhizosphere microbiome: significance of plant beneficial, plant pathogenic, and human pathogenic microorganisms. FEMS Microbiol. Rev. 37, 634–663. doi: 10.1111/1574-6976.12028
Messa V. R., Torres da Costa A. C., Kuhn O. J., Stroze C. T. (2020). Nematophagous and endomycorrhizal fungi in the control of Meloidogyne incognita in soybean. Rhizosphere 15, 100222. doi: 10.1016/j.rhisph.2020.100222
Mingot-Ureta C., Lopez-Moya F., Lopez-Llorca L. V. (2020). Isolates of the nematophagous fungus Pochonia chlamydosporia are endophytic in banana roots and promote plant growth. Agronomy 10, 1299. doi: 10.3390/agronomy10091299
Nahed M., Ramesh K., Huaiqing W., J (Hans) van L. (2017). Soil ozonation for nematode disinfestation as an alternative to methyl bromide and nematicides. Adv. Environ. Stud. 1. doi: 10.36959/742/197
Nicol J. M., Turner S. J., Coyne D. L., Nijs L. D., Hockland S., Maafi Z. T. (2011). “Current nematode threats to world agriculture,” in Genomics and molecular genetics of plant-nematode interactions (Dordrecht: Springer), 21–43. doi: 10.1007/978-94-007-0434-3_2
Niu X.-M. (2017). “Secondary metabolites from pochonia chlamydosporia and other species of pochonia,” in .Manzanilla-López R., Lopez-Llorca L. (eds). Perspectives in sustainable nematode management through pochonia chlamydosporia applications for root and rhizosphere health (Cham:Springer International Publishing), 131–168. doi: 10.1007/978-3-319-59224-4_7
Palma-Guerrero J., Lopez-Jimenez J. A., Pérez-Berná A. J., Huang I.-C., Jansson H.-B., Salinas J., et al. (2010). Membrane fluidity determines sensitivity of filamentous fungi to chitosan. Mol. Microbiol. 75 (4), 1021–1032. doi: 10.1111/j.1365-2958.2009.07039.x
Palomares-Rius J. E., Hasegawa K., Siddique S., Vicente C. S. L. (2021). Editorial: Protecting our crops - approaches for plant parasitic nematode control. Front. Plant Sci. 12. doi: 10.3389/fpls.2021.726057
Pentimone I., Colagiero M., Ferrara M., Nigro F., Rosso L. C., Ciancio A. (2019). Time-dependent effects of Pochonia chlamydosporia endophytism on gene expression profiles of colonized tomato roots. Appl. Microbiol. Biotechnol. 103, 8511–8527. doi: 10.1007/s00253-019-10058-z
Picciotti U., Massaro A., Galiano A., Garganese F. (2021). Cheese fortification: review and possible improvements. Food Rev. Intern. 1-27. doi: 10.1080/87559129.2021.1874411
Poveda J., Abril-Urias P., Escobar C. (2020). Biological control of plant-parasitic nematodes by filamentous fungi inducers of resistance: Trichoderma, mycorrhizal and endophytic fungi. Front. Microbiol. 11. doi: 10.3389/fmicb.2020.00992
Pujol N., Cypowyj S., Ziegler K., Millet A., Astrain A., Goncharov A., et al. (2008). Distinct innate immune responses to infection and wounding in the C. elegans epidermis. Curr. Biol. 18, 481–489. doi: 10.1016/j.cub.2008.02.079
Rashmi S., Maurya S., Upadhyay R. S. (2016). The improvement of competitive saprophytic capabilities of Trichoderma species through the use of chemical mutagens. Braz. J. Microbiol. 47, 10–17. doi: 10.1016/j.bjm.2015.11.003
Roesch L. F. W., Fulthorpe R. R., Riva A., Casella G., Hadwin A. K. M., Kent A. D., et al. (2007). Pyrosequencing enumerates and contrasts soil microbial diversity. ISME J. 1, 283–290. doi: 10.1038/ismej.2007.53
Saharan V., Kumaraswamy R. V., Choudhary R. C., Kumari S., Pal A., Raliya R., et al. (2016). Cu-Chitosan nanoparticle mediated sustainable approach to enhance seedling growth in maize by mobilizing reserved food. J. Agric. Food Chem. 64, 6148–6155. doi: 10.1021/acs.jafc.6b02239
Sahebani N., Hadavi N. (2008). Biological control of the root-knot nematode Meloidogyne javanica by Trichoderma harzianum. Soil Biol. Biochem. 40, 2016–2020. doi: 10.1016/j.soilbio.2008.03.011
Sambles C., Suarez-Fernandez M., Lopez-Moya F., Lopez-Llorca L. V., Studholme D. J. (2022). Chitosan induces differential transcript usage of chitosanase 3 encoding gene (csn3) in the biocontrol fungus Pochonia chlamydosporia 123. BMC Genomics 23, 101. doi: 10.1186/s12864-021-08232-7
Seino H., Kawaguchi N., Arai Y., Ozawa N., Hamada K., Nagao N. (2020). Investigation of partially myristoylated carboxymethyl chitosan, an amphoteric-amphiphilic chitosan derivative, as a new material for cosmetic and dermal application. J. Cosmet. Dermatol. 20 (7), 2332–2340. doi: 10.1111/jocd.13833
Sellitto V. M., Curto G., Dallavalle E., Ciancio A., Colagiero M., Pietrantonio L., et al. (2016). Effect of Pochonia chlamydosporia -based formulates on the regulation of root-knot nematodes and plant growth response. Front. Life Sci. 9, 177–181. doi: 10.1080/21553769.2016.1193827
Sharma P., Sharma M. M. M., Kapoor D., Rani K., Singh D., Barkodia M. (2020). Role of microbes for attaining enhanced food crop production. In: Singh J., Vyas A., Wang S., Prasad R. (eds).Microbial Biotechnology: Basic Research and Applications. Environmental and Microbial Biotechnology (Singapore:Springer) 55-78. doi: 10.1007/978-981-15-2817-0_3
Sikder M. M., Vestergård M. (2020). Impacts of root metabolites on soil nematodes. Front. Plant Sci. 10. doi: 10.3389/fpls.2019.01792
Silva S. D., Carneiro R. M. D. G., Faria M., Souza D. A., Monnerat R. G., Lopes R. B. (2017). Evaluation of Pochonia chlamydosporia and Purpureocillium lilacinum for suppression of Meloidogyne enterolobii on tomato and banana. J. Nematol. 49, 77–85. doi: 10.21307/jofnem-2017-047
Singh T., Chittenden C. (2021). Synergistic Ability of Chitosan and Trichoderma harzianum to Control the Growth and Discolouration of Common Sapstain Fungi of Pinus radiata. Forests 12 (5), 542. doi: 10.3390/f12050542
Soares F. E., de F., Sufiate B. L., de Queiroz J. H. (2018). Nematophagous fungi: Far beyond the endoparasite, predator and ovicidal groups. Agric. Nat. Resour. 52, 1–8. doi: 10.1016/j.anres.2018.05.010
Soliveres S., García-Palacios P. (2019). Secondary succession, biotic interactions and the functioning of roadside communities: plant-soil interactions matter more than plant-plant interactions. Ecosistemas 28, 50–60. doi: 10.7818/ECOS.1718
Stirling G. R. (2018). “Biological control of plant-parasitic nematodes,” in Poinar G.O. Jr, Hans-Börje J. Diseases of nematodes (CRC Press), 103–150.
Suarez-Fernandez M., Marhuenda-Egea F. C., Lopez-Moya F., Arnao M. B., Cabrera-Escribano F., Nueda M. J., et al. (2020). Chitosan induces plant hormones and defenses in tomato root exudates. Front. Plant Sci. 11. doi: 10.3389/fpls.2020.572087
Suarez-Fernandez M., Sambles C., Lopez-Moya F., Nueda M. J., Studholme D. J., Lopez-Llorca L. V. (2021). Chitosan modulates Pochonia chlamydosporia gene expression during nematode egg parasitism. Environ. Microbiol. 23, 4980–4997. doi: 10.1111/1462-2920.15408
Swarnakumari N., Sindhu R., Thiribhuvanamala G., Rajaswaminathan V. (2020). Evaluation of oil dispersion formulation of nematophagus fungus Pochonia chlamydosporia against root-knot nematode Meloidogyne incognita in cucumber. J. Asia-Pac. Entomol. 23, 1283–1287. doi: 10.1016/j.aspen.2020.10.008
Tikhonov V. E., Lopez-Llorca L. V., Salinas J., Jansson H.-B. (2002). Purification and characterization of chitinases from the nematophagous fungi Verticillium chlamydosporium and V. suchlasporium. Fungal Genet. Biol. 35, 67–78. doi: 10.1006/fgbi.2001.1312
Tseng Y.-H., Rouina H., Groten K., Rajani P., Furch A. C. U., Reichelt M., et al. (2020). An endophytic Trichoderma strain promotes growth of its hosts and defends against pathogen attack. Front. Plant Sci. 11. doi: 10.3389/fpls.2020.573670
United Nations Population United Nations. Available at: https://www.un.org/en/global-issues/population (Accessed February 23, 2022).
Valadão M. C., Millena de Carvalho L., Vieira Í S., Neves P. H., Ferreira V. M., Campos A. K., et al. (2020). Germination capacity of the Pochonia chlamydosporia fungus after its passage through the gastrointestinal tract of domestic chickens (Gallus gallus domesticus). Exp. Parasitol. 216, 107936. doi: 10.1016/j.exppara.2020.107936
van der Heijden M. G. A., Klironomos J. N., Ursic M., Moutoglis P., Streitwolf-Engel R., Boller T., et al. (1998). Mycorrhizal fungal diversity determines plant biodiversity, ecosystem variability and productivity. Nature 396, 69–72. doi: 10.1038/23932
Varandas R., Egas C., Conceição I. L. (2020). Potato cyst nematodes: New solutions to an old problem. Crop Prot. 137, 105303. doi: 10.1016/j.cropro.2020.105303
Vasyukova N. I., Zinov’eva S. V., Udalova Z., Panina Y., Ozeretskovskaya O. L., Sonin M. D. (2003). The role of salicylic acid in systemic resistance of tomato to nematodes. Dokl. Biol. Sci. 391, 343–345. doi: 10.1023/A:1025158702692
Wardle D. A., Bardgett R. D., Klironomos J. N., Setälä H., van der Putten W. H., Wall D. H. (2004). Ecological linkages between aboveground and belowground biota. Science 304, 1629–1633. doi: 10.1126/science.1094875
Wu J., Du C., Zhang J., Yang B., Cuthbertson A. G. S., Ali S. (2021). Synthesis of Metarhizium anisopliae–chitosan nanoparticles and their pathogenicity against Plutella xylostella (Linnaeus). Microorganisms 10, 1. doi: 10.3390/microorganisms10010001
Xoca-Orozco L.Á., Aguilera-Aguirre S., Vega-Arreguín J., Acevedo-Hernández G., Tovar-Pérez E., Stoll A., et al. (2019). Activation of the phenylpropanoid biosynthesis pathway reveals a novel action mechanism of the elicitor effect of chitosan on avocado fruit epicarp. Food Res. Int. 121, 586–592. doi: 10.1016/j.foodres.2018.12.023
Yang J.-I., Loffredo A., Borneman J., Becker J. O. (2012). Biocontrol efficacy among strains of Pochonia chlamydosporia obtained from a root-knot nematode suppressive soil. J. Nematol. 44, 67–71.
Yin H., Du Y. (2011). “Mechanism and application of chitin/chitosan and their derivatives in plant protection,” in Chitin, chitosan, oligosaccharides and their derivatives: biological activities and applications. Ed. Kim S. K. (Boca Raton: CRC Press, Taylor & Francis Group LLC), 605–618.
Zavala-Gonzalez E. A., Escudero N., Lopez-Moya F., Aranda-Martinez A., Exposito A., Ricaño-Rodríguez J., et al. (2015). Some isolates of the nematophagous fungus Pochonia chlamydosporia promote root growth and reduce flowering time of tomato. Ann. Appl. Biol. 166, 472–483. doi: 10.1111/aab.12199
Zavala-Gonzalez E. A., Rodríguez-Cazorla E., Escudero N., Aranda-Martinez A., Martínez-Laborda A., Ramírez-Lepe M., et al. (2017). Arabidopsis thaliana root colonization by the nematophagous fungus Pochonia chlamydosporia is modulated by jasmonate signaling and leads to accelerated flowering and improved yield. New Phytol. 213, 351–364. doi: 10.1111/nph.14106
Zhang Y., Li S., Li H., Wang R., Zhang. K.-Q., Xu J. (2020). Fungi–nematode interactions: Diversity, ecology and biocontrol prospects in agriculture. J. Fungi 6, 206. doi: 10.3390/jof6040206
Zhang Z., Qu Y., Li S., Feng K., Wang S., Cai W., et al. (2017). Soil bacterial quantification approaches coupling with relative abundances reflecting the changes of taxa. Sci. Rep. 7 (1)1–10. doi: 10.1038/s41598-017-05260-w
Zheng Y., Yan S., Qi J., Zhao Y., Guo X., Shi B. (2020). Protective effect of chitosan oligosaccharide against oxidative damage of peripheral blood mononuclear cells in dairy cows induced by diethylenetriamine/nitric oxide via NF-κB signalling pathway. Ital. J. Anim. Sci. 19, 602–609. doi: 10.1080/1828051X.2020.1772131
Keywords: chitosan, nematophagous fungi, nematode biomanagement, Pochonia chlamydosporia, agrobiotechnology, biocontrol
Citation: Lopez-Nuñez R, Suarez-Fernandez M, Lopez-Moya F and Lopez-Llorca LV (2022) Chitosan and nematophagous fungi for sustainable management of nematode pests. Front. Fungal Biol. 3:980341. doi: 10.3389/ffunb.2022.980341
Received: 28 June 2022; Accepted: 30 September 2022;
Published: 24 October 2022.
Edited by:
Filippe Elias De Freitas Soares, Universidade Federal de Lavras, BrazilReviewed by:
Octavio Loera, Universidad Autónoma Metropolitana, MexicoErnesto A. Zavala Gonzalez, Independent Researcher, Villena, Spain
Spiridon Mantzoukas, University of Ioannina, Greece
Copyright © 2022 Lopez-Nuñez, Suarez-Fernandez, Lopez-Moya and Lopez-Llorca. This is an open-access article distributed under the terms of the Creative Commons Attribution License (CC BY). The use, distribution or reproduction in other forums is permitted, provided the original author(s) and the copyright owner(s) are credited and that the original publication in this journal is cited, in accordance with accepted academic practice. No use, distribution or reproduction is permitted which does not comply with these terms.
*Correspondence: Federico Lopez-Moya, ZmVkZXJpY28ubG9wZXpAdWEuZXM=; Luis Vicente Lopez-Llorca, bHYubG9wZXpAdWEuZXM=