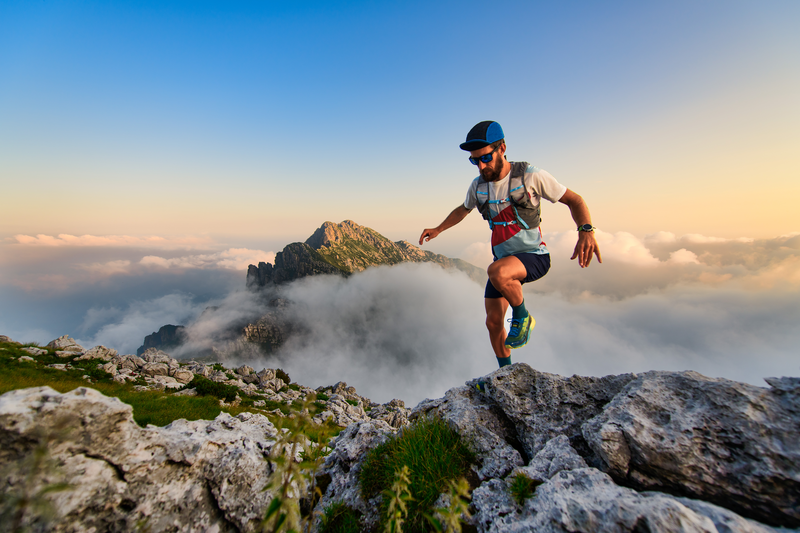
94% of researchers rate our articles as excellent or good
Learn more about the work of our research integrity team to safeguard the quality of each article we publish.
Find out more
ORIGINAL RESEARCH article
Front. Fungal Biol. , 26 January 2023
Sec. Fungi-Plant Interactions
Volume 3 - 2022 | https://doi.org/10.3389/ffunb.2022.968528
Recent studies have shown that entomopathogenic fungi, as endophytes, can have beneficial effects on plants, protecting them from defoliating insects. The potential of endophytic association by entomopathogenic fungi with the peanut crop has been little explored. In our study, we conducted experiments by inoculation of peanut seeds through a soil drench method with nine strains/species of entomopathogenic fungi of the genera Metarhizium, Beauveria and Cordyceps, subsequently these plants were consumed by two larval pests, Chrysodeixis includens and Spodoptera cosmioides. The parameters of larval growth rates, mortality, foliar consumption and larval period were observed during the development of larvae. In addition, the endophytic capacity of these fungi in peanut plants and their persistence in soil were investigated. In two replicate greenhouse trials for each larva, peanut plants were inoculated with fungi by the soil-drench method. We evaluated the performance of C. includens and S. cosmioides feeding on inoculated peanut plants starting at the 2nd larval instar. The larval and pupal weights of C. includens and S. cosmioides were significantly different among the fungal treatment groups, where insects feeding on control plants exhibited higher larval and pupal weights than insects feeding on treated plants. The differences in larval period showed that Control larvae pupated faster than the larvae fed on fungal-inoculated plants, fungal treatments had a larval period of 3 to 5 days more than the control. The mortality rates of C. includens and S. cosmioides were significantly different among the fungal treatment groups, insects fed on Control plants exhibited higher survival than insects fed on fungal-inoculated plants. The persistence of all Metarhizium fungi was higher in the soil compared to other fungi, and only Metarhizium and B. bassiana IBCB215 emerged from the phyllosphere of peanut plants. Although the fungus Cordyceps presented the worst performance among the fungal treatments. Overall, our results demonstrate the negative effects on the development of C. includens and S. cosmioides that were fed on fungal-inoculated peanut plants, the best results recorded were for Metarhizium strains and the fungus B. bassiana IBCB215.
The peanut (Arachis hypogaea L.) is an economically important oil seed crop that can be grown as a food resource, cover crop, green manure, forage, intercrop and hay (Akram et al., 2018). The peanut is important because it is the fourth most planted oilseed in the world, whose grains represent an important source of carbohydrates, protein and oil. In addition, it is rich in vitamins and amino acids and is considered a high-calorie food (Davis et al., 2016).
Brazil produced more than 700.5 mt of peanuts in 2021/22 and occupies the 11th position among the world’s largest producers, being the second largest exporter in Latin America (USDA, 2021). The largest concentration of Brazilian production is in the southeast, with the state of São Paulo being the largest producer in the country (Conab, 2022).
Nevertheless, the high agricultural cost due to the need for frequent applications of pesticides for pest control puts the viability of peanut agribusiness at risk (Santos et al., 2013; Pirotta et al., 2017). Michelotto et al. (2015) estimate that approximately 30% of the total cost of production of a hectare is spent on the control of pests and diseases affecting the crop. In addition, frequent applications of pesticides have caused negative effects on the populations of natural enemies, leading to unbalanced agrosystems and pest population outbreaks (Moscardi et al., 2011).
Due to concerns about the influence of pesticides on the environment and human safety, it is necessary to push forward the development of environmentally friendly and economically viable and reliable strategies (Mantzoukas and Eliopoulos, 2020).
Among the main pests that occur in peanuts, the lepidopterous genera Stegasta, Spodoptera, Chrysodeixis and Helicoverpa are the most important (Michelotto et al., 2012; Pinto et al., 2020; Virk et al., 2021; Costa et al., 2022). In addition to lepidoptera, the peanut crop is severely attacked by thrips, especially the honeysuckle thrips Enneothrips flavens Molton (Lourenção et al., 2007; Calore et al., 2012; Michelotto et al., 2012).
Entomopathogenic fungi have emerged as a sustainable alternative in agroecosystems, as they can infect a large number of arthropods and maintain the pest population in balance through the field as a safe alternative to toxic chemical insecticides (Alves, 1998; Litwin et al., 2020). In Brazil, several national and multinational companies have been marketing entomopathogenic fungi as biopesticides for the control of major agricultural pests (Mascarin et al., 2019).
In addition to their use as biological insecticides, there is growing evidence that many species of entomopathogenic fungi can colonize the tissues of certain plants (Vega et al., 2008; Bamisile et al., 2018). Although only a few species have been reported as natural endophytes, there have been many successful attempts to artificially introduce the entomopathogenic fungi into plants using different techniques (Vega, 2018). This natural or artificial colonization can have several beneficial effects on plants, such as promotion of plant growth (Sasan and Bidochka, 2012), improved nutrient acquisition (Behie and Bidochka, 2014) and protection against phytopathogens and pests (Jaber and Enkerli, 2017; Vega, 2018).
Endophytic fungi can have significantly longer efficacy periods than nonendophytic organisms, since many are able to survive for at least the entire growing season of an annual crop (Harman et al., 2008). Endophytic strains of fungi, which colonize trees, can settle on the shoots, roots, or stems of perennial plants (Worapong et al., 2002; Hanada et al., 2008). Therefore, the use of entomopathogenic fungi as endophytes provides a new alternative for the biological control of pests and phytopathogens (Vidal and Jaber, 2015).
Most studies with entomopathogenic endophytic fungi have been conducted on annual and perennial crops of agronomic importance; however, studies with peanut plants are nonexistent (Vega, 2018; Mantzoukas et al., 2022). In this context, our study evaluated the effects of several species of entomopathogenic fungi on the development and plant consumption of Spodoptera cosmioides and Chrysodeixis includens in inoculated peanut plants.
Nine isolates of the fungi B. bassiana, M. anisopliae and C. fumosorosea were obtained from the Mycological Collection of EMBRAPA, CFI - Invertebrate Fungi (CG, Brasília, DF, Brazil) and Instituto Biológico (IBCB, Campinas, SP, Brazil) and used in bioassays (see Table 1).
Fungal isolates were grown on Petri dishes with potato-dextrose-agar (PDA; KASVI) medium and incubated in a B.O. D (Bio-Oxygen Demand) incubator in the dark at 26 ± 2°C for 10 days.
Subsequently, solid fermentation was performed to increase the amount of inoculum used for the experiments. For solid fermentation, 250 ml borosilicate glass bottles (Schott bottles) containing 50 g of parboiled rice were used. Initially, the substrate was hydrated with 100 ml of distilled water and kept at room temperature for 50 min. Then, the rice was sieved to remove excess water and returned to the Schott bottles to be autoclaved at 120°C for 20 min.
The inoculum of each fungal isolate was prepared by scraping the conidia present in the PDA culture medium and diluting with sterilized distilled water with 0.01% (v/v) Tween 80®. Inoculation of Schott bottles was performed in an aseptic laminar flow chamber by adding 5 ml of conidia suspension of each isolate, and then all flasks were kept in B.O.D. in the dark at 26± 2°C for 10 days.
After the proliferation of fungal isolates, a conidial suspension was prepared by washing the rice grains with 150 ml of 0.01% (v/v) Tween 80® in each flask, and the conidial concentration was adjusted to 1 x 108 conidia/ml by counting in a Neubauer hemocytometer (KASVI) with an optical microscope (LEICA DMLB).
To confirm the viability of the fungi used in the experiments, conidia germination was determined by the direct counting method in 4 ml of PDA corrected with 0.001% (v/v) Derosal® 500 SC (Carbendazim, Bayer CropScience, SP, Brazil) in Rodac® plates incubated with suspensions of 1x106 conidia/ml for each fungal species (Oliveira et al., 2015).
Subsequently, the Petri plates were kept in B.O.D. for 22 h in the dark at 26 ± 2°C. The number of germinated and nongerminated conidia was quantified under an optical microscope, estimating the percentage of germinated conidia. The suspensions were used only if germination rates were greater than 90%.
The larvae of C. includens and S. cosmioides were obtained from the Insect Biology Laboratory of the Department of Entomology and Acarology of ESALQ-USP, Piracicaba, State of São Paul, Brazil. The eggs of both species were placed in plastic jars (500 ml) containing moist filter paper and kept in a B.O.D. chamber at 26 ± 1°C for 12 h of photophase. First instar neonates were fed on control (uninoculated) peanut until they reached the 2nd instar to acclimate larvae to feed on live plants versus artificial diet.
Peanut seeds of the variety IAC OL-3 were obtained from COPLANA - Agroindustrial Cooperative, Jaboticabal, SP, Brazil. The seeds were transferred to plastic pots (0.7 L) containing a nonsterilized soil mixture of clay loam soil and sand (2:1). Four seeds per cup were sown at a depth of 2 cm.
The inoculation of fungal isolates and the control treatment were performed by direct application of the conidia suspension on the seed (soil drenching) according to the methodology of Parsa et al. (2013). The inoculation of each treatment was performed by applying 2 ml of the suspension with 1 x 108 conidia/ml on the seed after sowing. The control treatment was inoculated with 2 ml of sterile aqueous solution with 0.01% (v/v) Tween 80® without adding fungal inoculum.
All inoculated plants were grown in a greenhouse for 30 days at ~27 ± 3°C, with a natural photoperiod (10.5 to 13.5 hours) for the duration of the experiment. Pots were placed in a completely randomized design, watered as needed and fertilized biweekly with 3 g/dm³ of Osmocote® slow release pelletized fertilizer (N; P2O5; K2O - 14-14-14) per pot.
We conducted two experiments, repeated twice in time, for each larva to check the effects of nine entomopathogenic fungi, Metarhizium, B. bassiana and C. fumosorosea, on the survival and development of S. cosmioides and C. includens. S. cosmioides larval infestation times were March 5, 2020, for the first assay and August 15th, 2021, for the second assay. C. includens larvae were set up on April 3, 2020, and September 21, 2021, for the first and second assays, respectively.
For each experiment, 30-day-old peanut plants were inoculated with the entomopathogenic fungi described in Table 1 and the control treatment, and in each pot, a plastic layer was placed on the soil surface to isolate the larvae by direct contact with the conidia in the soil. It was necessary to avoid contamination by conidia to accurately assess the indirect effects of leaf and stem consumption by larvae (Figure 1).
Figure 1 Illustrative scheme. (A) The vessel used in the experiments. (B) Thinning process of peanut plants, inoculation, and after being consumed by the larva.
Each plant was infested with a 2nd instar larva and covered with a sheer Organza bag (7 in x 9 in: 0.3 oz) to prevent larvae from escaping and allow airflow. The C. includens larvae were observed for 27 days and S. cosmioides for 30 days because each larval species has a different developmental period starting from the first instar.
Each larva within each plant was considered a repetition, and each treatment had 30 repetitions, totaling 30 infested pots per treatment. Larvae were weighed every 3 days on a high-precision scale. Mortality was recorded daily, and days to pupation, pupal weight and foliar consumption from control and treated plants were also recorded during both greenhouse trials.
If the plant reached a high level of defoliation, it was replaced by a new plant of the same treatment to continue the experiment until the larvae reached the pupal stage. For the replacement of fungal-inoculated peanut plants, new plants were sown throughout the experiments. The sowing dates of the new lots were consecutive, to always have plants of the same age and not lack food for both larvae.
The estimate of leaf area consumed was analyzed with LeafByte mobile application (Getman-Pickering et al., 2020). Twenty-five plants 30 days old were collected and pricked, leaving only 3 pairs of 4 leaflets, and the leaves were measured to generate an estimate of leaf area for each plant.
The average was determined at 36.6 cm² for each plant. The entire consumption of a plant was considered when the larvae ate all the leaves (Figure 1), and then it was replenished with a new inoculated plant with the same age characteristics and fungal inoculation.
Peanut plants inoculated with fungal treatments were whole washed in distilled water to remove surface dirt on leaves and the soil at the roots 30 days after inoculation. Subsequently, the plant material (roots, stems and leaves) was cut with scissors into fragments.
For each treatment, 5 plants were randomly selected, totaling 60 plant fragments per treatment (20 roots, 20 stems and 20 leaves). These samples were superficially sterilized by immersion in 70% ethanol for 1 min, 1% sodium hypochlorite (NaOCl) for 2 min, and 70% ethanol for 1 min again, rinsed three times in sterile distilled water and dried on sterile filter paper. Sterilization efficacy was confirmed by plating 100 μl of the last rinse water on PDA media, and the absence of fungal or bacterial growth was considered indicative of a successful sterilization technique (Parsa et al., 2013), and each leaf section was printed on PDA media before and after sterilization (Greenfield et al., 2016).
Plant samples were then individually placed in Petri dishes (90×15 mm) containing 20 ml of PDA with 0.5 g/L cycloheximide, 0.2 g/L chloramphenicol, 0.5 g/L Dodine (65%) and 0.01 g/L Crystal Violet (Behie et al., 2015). Petri dishes were incubated in the dark at 24°C for 15 days. Prepared suspensions of the soil where the plants grew were also seeded on the same selective medium at the following four concentrations after serial dilution in distilled water + 0.05% Tween 80®: 1×10, 1×10-1, 1×10-2, and 1×10-3.
Petri dishes were incubated in the dark at 24°C for 15 days, and the presence of colonies (CFU) was quantified at each concentration after the incubation period. The presence of entomopathogenic fungi in plant tissues and diluted soil was confirmed by observing the growth of colonies of each fungal species on the culture medium and observing the reproductive structures under an optical microscope (morphological visualization), according to the taxonomic keys described by Humber (2012). The colonization frequency was estimated as the number of fragments colonized by entomopathogenic fungi in relation to the total number of plant fragments and expressed as a percentage.
For larval weight, period, and foliar consumption, as well as per pupal weight, homogeneity of variance was performed using the Kolmogorov-Smirnov test, which showed that the data were normally distributed. For the mortality variable, the homogeneity of variance test was performed using the Shapiro-Wilk test.
To assess S. cosmioides and C. includens mortality, larval weight, pupal weight, larval period and larval foliar consumption, an analysis of variance (ANOVA) was performed in the software R Studio® (library used “ExpDes.pt”, “agricolae” “nortest”) in a completely randomized design (CRD) (R Core Team, 2020). When a significant result was detected, the means were compared using Tukey’s test at 5% probability for mortality and Scott-Knott’s test at 5% probability for all other variables.
To compare the permanence of fungi, the quantification of colony forming units (CFU) per gram of soil was used. The log-transformed values were submitted to one-way ANOVA, considering a confidence level of 95%. Given the existence of differences, the means of the groups were compared pairwise using the Tukey honest significant difference (Tukey-HSD) test at a 5% significance level (p value < 0.05).
Statistical tests were conducted in R software (R Core Team, 2020) using native functions and the “HSD.test” function of the “agricolae” package (Mendiburu, 2021). The graphical representation of the comparison of means was obtained with the “ggplot2” package (Wickham, 2016).
The weight of S. cosmioides larvae was recorded for 27 days and C. includens for 24 days, but due to discrepancies in weight values, delays in larval development and occasional deaths, analyses of larval weights were made 15 days after the experiments were installed. The weights of S. cosmioides and C. includens larvae fed on fungal-inoculate plants differed from the control, with the Metarhizium strains (CG814, CG1123, CG1126 and IBCB348) causing the lowest larval weights for both insect species (see Table 2).
Table 2 S. cosmioides and C. includens larval weights after feeding for 15 days on peanut plants inoculated with entomopathogenic fungi and uninoculated control.
For the S. cosmioides trial, a maximum weight of 238.56 ± 5.30 mg and a minimum weight of 161.11 ± 4.93 mg were recorded, corresponding to the control and CG1126 treatments, respectively. For the C. includens assay, the maximum and minimum weights were 261.04 mg and 173.32 ± 4.59 mg, respectively, corresponding to the treatment with strain CG814 (see Table 2).
In both assays, there were significant differences, and their probabilities of significance (p value) were 2.2 x 10 -16 for both larvae. The normality values of the data, calculated by the Kolmogorov-Smirnov test, were 0.09107 for S. cosmioides and 0.6996 for C. includens.
All pupal weight treatments of both larvae were significantly different from the control, with the exception of IBCB130 and CG1123, which had no significant differences from the control for C. includens treatments. S. cosmioides individuals from fungi-inoculated treatments (CG1123, CG1126, IBCB215 and IBCB425) had the lowest pupal weights (see Table 3).
Table 3 S. cosmioides and C. includens pupal weights after feeding on peanut plants inoculated with entomopathogenic fungi and uninoculated control.
The lowest pupal weights for C. includens individuals with fungi inoculated treatments were IBCB215, IBCB66, CG1126 and CG814. The fungi B. bassiana IBCB215 and Metarhizium CG1126 were observed to show the significantly lowest pupal weight for both larvae S. cosmioides and C. includens.
The maximum and minimum average weights of S. cosmioides pupae were 327.26 ± 3.49 mg and 278.92 ± 2.77 mg, corresponding to the CG1126 and control treatments, respectively. However, C. includens treatments IBCB425 and IBCB215 recorded a weight of 273.97 ± 1.55 mg (max.) and 264.55 ± 2.23 mg (min.) (see Table 3). The probability values (p values) for the S. cosmioides and C. includens tests were 8.51 x 10-45 and 0.015023, respectively, and the normality of the data, according to the Kolmogorov-Smirnov test at 5%, was 0.1055 and 0.5418 for S. cosmioides and C. includens, respectively.
The larval periods of the S. cosmioides and C. includens treatments were significantly different from the larval period of the control, with the exception of IBCB130, which had no significant difference from the S. cosmioides control treatment (see Table 4).
Table 4 S. cosmioides and C. includens larval period after feeding on peanut plants inoculated with entomopathogenic fungi and uninoculated control.
Pairwise comparisons showed that control larvae pupated faster than the larvae fed on fungal-inoculated plants. The Metarhizium fungi treatments CG1123, CG814 and CG1126 had the longest S. cosmioides and C. includens larval periods. The fungi Metarhizium treatments CG1123 and CG814 showed the significantly longest larval delay for both larvae S. cosmioides and C. includens.
The S. Cosmioides treatments CG1123 and Control recorded the maximum (28.44 ± 0.29 days) and minimum (24.97 ± 0.31 days) larval periods; thus, there was a larval delay of approximately 3 days for fungal-inoculated plants in comparison to the control (see Table 4). For the C. includens treatments CG814 and Control, the maximum (25.81 ± 0.25 days) and minimum (20.30 ± 0.24 days) larval periods were recorded, exhibiting a larval delay of approximately 5 days for fungal-inoculated plants in comparison to the control.
The mean area of leaves consumed by both larvae differed significantly among treatments (S. cosmioides, p = 2.2 x 10 -16 and C. includens, p = 0.000176) (see Table 5). The control and CG814 treatments for the S. cosmioides and C. includens assays recorded the maximum (261.05 ± 5.76 cm²; 165.06 ± 6.43 cm²) and minimum (1st = 173.32 ± 7.94 cm²; 122.24 ± 7.93 cm²) leaf consumption values, respectively (see Table 5).
Table 5 Cumulative foliar consumption (cm²) of S. cosmioides and C. includens fed with peanut plants inoculated with entomopathogenic fungi and uninoculated control.
The normality values of the data, calculated by the Kolmogorov-Smirnov test, were 0.4032 for the S. cosmioides test and 00.69 for the C. includens test. The fungal Metarhizium strains CG1126, CG1123, and CG814 presented lower foliar consumption by both larval species.
Comparisons showed that control individuals lived longer on average than the individuals on fungal-treated plants. Metarhizium strains CG1126, CG814, CG1123 and B. bassiana IBCB215 caused the highest mortalities on both insect species (see Table 6). The highest mortality rates for both larvae were reported for the CG814 treatment, with 50 ± 2.82% for S. cosmioides and 38 ± 5.93% for C. includens.
Table 6 Mortality (%) of S. cosmioides and C. includens fed peanut plants inoculated with entomopathogenic fungi and the uninoculated control.
The lowest mortality rate in fungal treatments was recorded in IBCB130, 14 ± 4.56% for S. cosmioides and 8 ± 5.21% for C. includens (see Table 6). The probability of significance (p value) for S. cosmioides and C. includens trials was 5.12 x 10-08 and 7.31 x 10 -05, respectively. Additionally, the normality of the data was estimated using the Shapiro-Wilk test, obtaining values of 0.1912 and 0.06140.
Metarhizium (CG814, CG112, CG11236, IBCB348) and B. bassiana IBCB215 isolates became endophytic with relatively low colonization levels at 30 days after peanut seed inoculation (see Table 7). For Metarhizium, roots and leaves were colonized, while B. bassiana colonized stems and leaves (see Table 7). None of the target fungi were recovered from the plant tissue in the control treatment, C. fumosorosea isolates (IBCB 130, IBCB 867) and B. bassiana IBCB66. Occasionally, other unidentified fungi were grown from the plant tissues but with no apparent relationship to the treatment.
Table 7 Occurrence (%) of entomopathogenic fungi in peanut plant fragments at 30 days after inoculation.
In general, the presence of all entomopathogenic fungi was higher in the soil than in the phyllosphere. For instance, the presence of some Metarhizium strains was significantly higher in soil (CG814 7.7 x 106 CFU/g, CG1126 6.2 x 106 CFU/g, CG1123 4.0 x 106 CFU/g and IBCB 348 9.5 x 105 CFU/g), followed by B. bassiana IBCB215 (1.6 x 105 CFU/g) and M. anisopliae IBCB425 (3.1 x 105 CFU/g) (see Figure 2).
Figure 2 Presence of entomopathogenic fungi in CFU/g soil on peanut plants 30 days after inoculation.
Most studies of entomopathogenic fungi in agriculture over time have focused on their pathogenicity to insects by spraying formulated products on plants. However, recent studies of the ecological interaction of these fungi in soils and plants are providing a novel alternative for the management of insect pests, such as the potential of the endophytic relationship of these fungi with plants (Bamisile et al., 2018; Vega et al., 2018; Mantzoukas et al., 2022).
The ability to minimize attack from lepidopterous larvae has widely been reported by Vega et al. (2018) for many crops, but to date, no studies have been conducted with peanut plants in this sense.
Our results provide the first report on survivorship and performance parameters for S. cosmioides and C. includens fed inoculated peanut plants with entomopathogenic fungi (Metarhizium, Beauveria and Cordyceps).
Fungal endophytes can provide protection for crop plants against insect attack. However, some fungal and plant endophytic associations do not necessarily protect the plant from pest attacks, and only a few species associations function as defensive mutualism (Bamisile et al., 2021).
Several studies investigating the role of endophytes in protecting different crops against lepidopteran species did not measure insect performance parameters; however, they observed a reduction in the damage caused by pests (Jaber, L. R., Enkerli, J. (2017); Bamisile et al., 2018; Vega et al., 2018).
Environmental factors play a vital role in entomopathogenic fungi survival. Generally, entomopathogenic fungi survive well in nature between 10°C and 30°C, and the ideal temperature and higher humidity with frequent water spray observed in our study area could favor the activity of the fungal pathogens, as reported by Mc Coy et al. (1988).
Studying the persistence of entomopathogenic fungal pathogens is essential for their successful application and efficacy in the soil (Enkerli et al., 2004). In our study, the fungus B. bassiana was found in stems and leaves, while Metarhizium was mostly found within the plant roots and leaves.
Our results are similar to those obtained by Behie et al. (2015), who reported that B. bassiana and Pochonia chlamydosporia were localized within the stems and leaves, while Metarhizium spp. was mostly found within the plant roots.According to Tefera and Vidal (2009), colonization by B. bassiana is more widespread in leaves and stems than in roots.
Our study used the soil-drench technique to inoculate the fungi in peanut plants. Russo et al. (2019) demonstrated the effectiveness of three fungal inoculation methods (leaf spray, seed immersion and root immersion) to establish the fungus B. bassiana as an endophyte in soybeans, and the leaf spray inoculation method was the most successful.
However, other authors, such as Parsa et al. (2013), obtained good results in colonizing bean plants using the method performed in our study, and according to these authors and Bamisile et al. (2018), the specific outcome of endophytic colonization may depend on the target crop species or variety, the strain or isolate of entomopathogenic fungi species used, and the plant growth conditions.
Our study showed that Metarhizium strains had greater endophytic capacity and greater presence in the soil than the other fungi tested. Jaber and Enkerli (2017) described that fungal entomopathogens may colonize particular host plants more efficiently than others, which might consequently influence the level of plant protection by the colonizing fungi.
A limitation of many studies is that they did not measure the consumption of plant material by larvae feeding on plants inoculated with fungi or control plants, which could show possible inhibition or compensatory feeding behavior by larvae. Measuring the leaf area of whole plants is a difficult task, but in our study, we tested a method using peanut plants of the same age and the same number of leaves after trimming, so it was possible to perform a predetermined average of the whole plants.
Our study showed that the foliar consumption of S. cosmioides and C. includens larvae was drastically lower for some fungal treatments than for the control.In a similar study, Barta (2018) described that Cameraria ohridella larvae that fed on horse-chestnut leaves inoculated with Beauveria presented leaf areas up to 5 times smaller than the leaf areas of control plants.
Cabezas et al. (2013) demonstrated that S. cosmioides larvae consumed a daily average of 5.58 cm² to 15.3 cm² on castor and barbados nut leaves during development from the third instar to the pupal stage, while Bueno et al. (2011) mentioned that C. includens larvae can consume average values of 64 cm2 to 200 cm2 during the larval cycle. These results are similar to our study based on control treatment for both larvae.
We observed that the consumption of larvae that fed on plants with fungus was reduced by approximately 88 cm2 for S. cosmioides and 43 cm2 for C. includens.
The weights of S. cosmioides and C. includens larvae and pupae were significantly different between the fungal treatment groups in our greenhouse trials. Similar results were obtained in some studies.
The authors McGee (2002); Powell et al. (2009); Leckie et al. (2008) found an effect of endophytes on the gained weight of lepidopteran species H. armigera, H. punctigera and H. zea. However, these effects were observed by incorporating fungal broth extracts of the endophytes into artificial diets rather than evaluating effects on insect growth via in planta feeding assays.
Some studies similar to ours that utilized in planta feeding assays (Jallow et al., 2004; Jaber and Vidal, 2010) found a significant decrease in the relative growth rate of H. armigera larvae feeding on A. strictum-inoculated plants, but they did not observe a difference in pupal weights among treatments.
Additionally, Barta (2018) found that C. ohridella larvae fed horse chestnut leaves had lower pupal weights than larvae fed control leaves (without fungus). Lopez and Sword (2015) did not observe a difference in pupal and larval weights of H. zea larvae feeding on cotton-inoculated plants.
Our results showed significant larval mortality rates for S. cosmioides and C. includens of the fungal endophyte treatment on peanut plants; almost all strains of Metarhizium and only one strain of B. bassiana in our study showed the highest rates of mortality. However, the strains of C. fumosorosea (IBCB 130 and IBCB 867) and B. bassiana IBCB66 did not show high rates of larval mortality compared to other fungi.
Other studies showed similar results with different fungal isolates, plants and insects, including the study conducted by Resquín-Romero et al. (2016), who tested the effects of inoculating the fungi B. bassiana and M. brunneum on alfalfa, tomato and melon plants on Spodoptera littoralis larvae.
The results showed that S. littoralis fed on inoculated plants had mortalities ranging from 41.7 to 76.6%. Another study by Peña-peña et al. (2015) showed mortality rates of 50% in Anomala cincta larvae that fed on corn plants inoculated with M. pingshaense.
Mantzoukas et al. (2015) also showed larval mortality rates of 70 to 100% in Sesamia nonagrioides feeding on sorghum plants inoculated with the fungi B. bassiana, M. robertsii and I. fumosorosea. Leckie (2002); Powell et al. (2009) and Lopez and Sword (2015) reported a reduction in the damage caused and mortality of H. zea in tomato plants after treatment with B. bassiana.
Another study by Sánchez-Rodríguez et al. (2018) showed that 30-57% of S. littoralis larvae died after consuming endophytically colonized wheat leaves. Klieber and Reineke (2016) additionally recorded 50% mortality of all larval instars and reduced longevity of Tuta absoluta larvae fed with B. bassiana colonized with tomato leaves.
In this context, according to Bamisile et al. (2018), entomopathogenic fungal endophytes activate the production of plant defense proteins in their colonizing hosts. Thus, the induced systemic responses produced by fungal endophytes are related to the amplification of expressed genes in pathogenesis (Fadiji and Babalola, 2020).
Their capacity to increase the production of important metabolites, proteins and other defense enzymes in peanuts has been demonstrated by Senthilraja et al. (2013) in peanut seedlings inoculated with B. bassiana and Pseudomonas fluorescens. In another study, a similar combination of B. bassiana and P. fluorescens strains significantly reduced damage caused by leafminer (Aproaerema modicella Deventer) on peanuts (Senthilraja et al., 2010)
Our study demonstrated developmental delay up to the pupal stage for both larvae that fed on peanut plants inoculated with fungi, and the differences in larval delay in fungi treatments relative to the control reached an average of 3 to 5 days. A similar delay in pupation was observed by Jaber and Vidal (2010), who showed that H. armigera feeding on endophyte-treated plants in planta trials exhibited slower larval and prepupal developmental times than individuals feeding on control plants.
In contrast, Leckie et al. (2008), using H. zea individuals feeding on B. bassiana broth extracts incorporated into an artificial diet, showed that larvae feeding on endophyte treatments pupated faster than the control (13 vs. 15 days). Lopez and Sword (2015) also observed any difference in days to pupation of H. zea consuming cotton plants inoculated with B. bassiana endophyte in greenhouse trials, but they observed that control insects reached the adult stage faster than the B. bassiana endophyte treatment. In our study, we did not evaluate the time from pupa to adult stage of insects.
In conclusion, the manipulation of endophytic fungi has the potential to protect plants from insect herbivores. Our study demonstrated for the first time the positive effects of the endophytic entomopathogens Metarhizium and B. bassiana in peanut plants. We observed negative effects on the survival and development of two herbivorous insect pests, S. cosmioides and C. includens. Importantly, although these effects were shown in greenhouse trials, more tests need to be done to achieve field conditions using a simple seed treatment inoculation.
Similarly, a variety of other studies have reported the successful manipulation of fungal endophytes in plants with positive effects against lepidopteran insects under greenhouse and field conditions (Vakili, 1990; Bing and Lewis, 1991; Bing and Lewis, 1992a; Bing and Lewis, 1992b; Cherry et al., 1999; Cherry et al., 2004; Powell et al., 2007; Powell et al., 2009; Reddy et al., 2009; Batta, 2013; Castillo Lopez et al., 2014; Lopez and Sword, 2015; Mantzoukas et al., 2015; Qayyum et al., 2015; Shrivastava et al., 2015; Vidal and Jaber, 2015; Klieber and Reineke, 2016; Ramírez-Rodríguez and Sánchez-Peña, 2016; Resquín-Romero et al., 2016; Sánchez-Rodríguez et al., 2018; Silva et al., 2020).
Finally, these results continue to highlight the viability of incorporating the use of fungal endophytes as functional components of integrated pest management (IPM) practices to protect plants from pests. More evaluation under field conditions is necessary to maximize efficacy when trying to incorporate fungal entomopathogens as endophytes within IPM programs.
The datasets presented in this study can be found in the online repository: https://repositorio.unesp.br/handle/11449/238519.
FV and EL contributed to conception and design of the study. FV organized the database. JN and LD performed the statistical analysis. FV wrote the first draft of the manuscript. FV, CR, LR, and NS wrote sections of the manuscript. All authors contributed to the article and approved the submitted version.
This study was financed by the Coordenação de Aperfeiçoamento de Pessoal de Nível Superior-Brasil (CAPES)-Finance Code 001.
The authors would like to thank the Agricultural Microbiology Program (University of São Paulo State, UNESP, Campus Jaboticabal) and the Coordination for the Improvement of Higher Education Personnel (CAPES). We also thank the COPLANA - Agroindustrial Cooperative for giving us the peanut seeds and Neide Graciano Zério (Department of Entomology and Acarology from the University of ESALQ-USP) for assisting us with the insects used in this study.
The authors declare that the research was conducted in the absence of any commercial or financial relationships that could be construed as a potential conflict of interest.
All claims expressed in this article are solely those of the authors and do not necessarily represent those of their affiliated organizations, or those of the publisher, the editors and the reviewers. Any product that may be evaluated in this article, or claim that may be made by its manufacturer, is not guaranteed or endorsed by the publisher.
Akram N. A., Shafiq F., Ashraf M. (2018). Peanut (Arachis hypogaea L.): A prospective legume crop to offer multiple health benefits under changing climate. Compr. Rev. Food Sci. Food Saf. 17, 1325–1338. doi: 10.1111/1541-4337.12383
Bamisile B. S., Akutse K. S., Siddiqui J. A., Xu Y. (2021). Model application of entomopathogenic fungi as alternatives to chemical pesticides: Prospects, challenges, and insights for next-generation sustainable agriculture. Front. Plant Sci. 12. doi: 10.3389/fpls.2021.741804
Bamisile B. S., Dash C. K., Akutse K. S., Keppanan R., Wang L. (2018). Fungal endophytes: Beyond herbivore management. Front. Microbiol. 9. doi: 10.3389/fmicb.2018.00544
Barta M. (2018). In planta bioassay on the effects of endophytic Beauveria strains against larvae of horse-chestnut leaf miner (Cameraria ohridella). Biol. Control 121, 88–98. doi: 10.1016/j.biocontrol.2018.02.013
Batta Y. A. (2013). Efficacy of endophyic and applied Metarhizium anisopliae (Metch.) sorokin (Ascomycota: Hypocreales) against larvae of Plutella xyllostella l. (Yponomeutidae: Lepidoptera) infesting brassica napus plants. Crop Prot. 44, 128–134. doi: 10.1016/j.cropro.2012.11.001
Behie S. W., Bidochka M. J. (2014). Ubiquity of insect-derived nitrogen transfer to plants by endophytic insect-pathogenic fungi: an additional branch of the soil nitrogen cycle. Appl. Environ. Microbiol. 80, 1553–1560. doi: 10.1128/AEM.03338-13
Behie S. W., Jones S. J., Bidochka M. J. (2015). Plant tissue localization of the endophytic insect pathogenic fungi metarhizium and beauveria. Fungal Ecology 13, 112–119. doi: 10.1016/J.FUNECO.2014.08.001
Bing L. A., Lewis L. C. (1991). Suppression of Ostrinia nubilalis (Hübner) (Lepidoptera: Pyralidae) by endophytic Beauveria bassiana (Balsamo) vuillemin. Environ. Entomol. 20, 1207–1211. doi: 10.1093/ee/20.4.1207
Bing L. A., Lewis L. C. (1992a). Temporal relationships between zea mays, Ostrinia nubilalis (Lep.: Pyralidae) and endophytic Beauveria bassiana. Entomophaga 37, 525–536. doi: 10.1007/BF02372322
Bing L. A., Lewis L. C. (1992b). Endophytic Beauveria bassiana (Balsamo) vuillemin in corn: the influence of the plant growth stage and Ostrinia nubilalis (Hübner). Biocontrol Sci. Technol. 2, 39–47. doi: 10.1080/09583159209355216
Bueno R. C. O. F., Bueno A. F., Moscardi F., Parra J. R. P., hoffmann-Campo C. B. (2011). Lepidopteran larvae consumption of soybean foliage: basis for developing multiple-specieseconomic thresholds for pest management decisions. Pest Manage. Sci. 67, 170–174. doi: 10.1002/ps.2047
Cabezas M. F., Nava D. E., Geissler L. O., Melo M., Garcia M. S., Krüger R. (2013). Development and leaf consumption by Spodoptera cosmioides (Walker) (Lepidoptera: Noctuidae) reared on leaves of agroenergy crops. Neotrop. entomol. 42, 588–594. doi: 10.1007/s13744-013-0169-6
Calore R. A., Boiça Júnior A. L., Chagas Filho N. R., de Souza J. R. (2012). Determinação do nível de controle econômico de enneothrips flavens Moulton 1941 (Thysanoptera: Thripidae) em cultivar de amendoim de porte ereto. Arq. Inst. Biol. 79, 263–272. doi: 10.1590/S1808-16572012000200015
Castillo Lopez D., Zhu-Salzman K., Ek-Ramos M. J., Sword G. A. (2014). The entomopathogenic fungal endophytes Purpureocillium lilacinum (Formerly Paecilomyces lilacinus) and Beauveria bassiana negatively affect cotton aphid reproduction under both greenhouse and field conditions. PLoS ONE 9, 1–8. doi: 10.1371/journal.pone.0103891
Cherry A. J., Banito A., Djegui D., Lomer C. (2004). Suppression of the stem-borer Sesamia calamistis (Lepidoptera; noctuidae) in maize following seed dressing, topical application and stem injection with African isolates of Beauveria bassiana. Int. J. Pest Manage. 50, 67–73. doi: 10.1080/09670870310001637426
Cherry A. J., Lomer C. J., Djegui D., Shulthess F. (1999). Pathogen incidence and their potential as microbial control agents in IPM of maize stem borers in West Africa. BioControl 44, 301–327. doi: 10.1023/A:1009991724251
Conab (2022) Safra brasileira de grãos. Available at: https://www.conab.gov.br/ultimas-noticias/4503-sao-paulo-producao-de-amendoim-aumenta-14-7-em-relacao-a-safra-anterior (Accessed March 14, 2022).
Costa E. N., de Souza B. H. S., Eduardo W. I. (2022). Effects of peanut cultivars and neem oil on the feeding preference, growth and mortality of fall armyworm and velvet bean caterpillar (Lepidoptera: Noctuidae). Phytoparasitica. 50, 1059–1073. doi: 10.1007/s12600-022-00995-3
Davis J. P., Price K. M., Dean L. L., Sweigart D. S., Cottonaro J. M., Sanders T. H. (2016). Peanut oil stability and physical properties across arange of industrially relevant oleic acid/linoleic acid ratios. Peanut Sci. 43, 1–11. doi: 10.3146/0095-3679-43.1.1
Enkerli J., Widmar F., Keller S. (2004). Long-term field persistence of Beauveria brongniartii strains applied as biocontrol agents against European cockchafer larvae in Switzerland. Biol. Control 29, 115–123. doi: 10.1016/S1049-9644(03)00131-2
Fadiji A. E., Babalola O. O. (2020). Elucidating mechanisms of endophytes used in plant protection and other bioactivities with multifunctional prospects. Front. Bioeng. Biotechnol. 8. doi: 10.3389/fbioe.2020.00467
Getman-Pickering Z. L., Campbell A., Aflitto N., Grele A., Davis J., Ugine T. A., et al. (2020). LeafByte: A mobile application that measures leaf area and herbivory quickly and accurately. Methods Ecol. Evol. 11, 215–221. doi: 10.1111/2041-210X.13340
Greenfield M., Gómez-Jiménez M. I., Ortiz V., Vega F. E., Kramer M., Parsa S. (2016). Beauveria bassiana and Metarhizium anisopliae endophytically colonize cassava roots following soil drench inoculation. Biol. Control 95, 40–48. doi: 10.1016/j.biocontrol.2016.01.002
Hanada R. E., de Jorge Souza T., Pomella A. W. V., Hebbar K. P., Pereira J. O., Ismaiel A., et al. (2008). Trichoderma martiale sp. nov., a new endophyte from sapwood of Theobroma cacao with a potential for biological control. Mycol. Res. 112, 1335–1343. doi: 10.1016/j.mycres.2008.06.022
Harman G. E., Björkman T., Ondik K., Shoresh M. (2008). Changing paradigms on the mode of action and uses of trichoderma spp. for biocontrol. Outlooks Pest Manage. 19, 24. doi: 10.1564/19feb08
Humber R. A. (2012). “Humber Identification of entomopathogenic fungi,” in Manual of techniques in invertebrate pathology. Ed. Lacey L. A. (USA: Academic Press), 151–187.
Jaber L. R., Enkerli J. (2017). Fungal entomopathogens as endophytes: can they promote plant growth? Biocontrol Sci. Technol. 27, 28–41. doi: 10.1080/09583157.2016.1243227
Jaber L. R., Vidal S. (2010). Fungal endophyte negative effects on herbivory are enhanced on intact plants and maintained in a subsequent generation. Ecological Entomology 35, 25–36. doi: 10.1111/j.1365-2311.2009.01152.x
Jallow F. A., Cunningham J. P., Zalucki M. P. (2004). Intra-specific variation for host plant use in Helicoverpa armigera (Hübner) (Lepidoptera: Noctuidae): implications for management. Crop Protect. 23, 955–964. doi: 10.1016/j.cropro.2004.02.008
Klieber J., Reineke A. (2016). The entomopathogen Beauveria bassiana has epiphytic and endophytic activity against the tomato leaf miner Tuta absoluta. Journal of Applied Entomology 140, 580–589. doi: 10.1111/jen.12287
Leckie B. M. (2002). Effects of Beauveria bassiana mycelia and metabolites incorporated into synthetic diet and fed to larval Helicoverpa zea; and detection of endophytic Beauveria bassiana in tomato plants using PCR and ITS primers. [dissertation/master’s thesis]. (Knoxville, TN: University of Tennessee).
Leckie B. M., Ownley B. H., Pereira R. M., Klingeman W. E., Jones C. J., Gwinn K. D. (2008). Mycelia and spent fermentation broth of beauveria bassiana incorporated into synthetic diets affect mortality, growth and development of larval Helicoverpa zea (Lepidoptera: Noctuidae). Biocontrol Sci. Technol. 18, 697–710. doi: 10.1080/09583150802262906
Litwin A., Nowak M., Różalska S. (2020). Entomopathogenic fungi: unconventional applications. Rev. Environ. Sci. Biotechnol. 19, 23–42. doi: 10.1007/s11157-020-09525-1
Lopez D. C., Sword G. A. (2015). The endophytic fungal entomopathogens Beauveria bassiana and purpureocillium lilacinum enhance the growth of cultivated cotton (Gossypium hirsutum) and negatively affect survival of the cotton bollworm (Helicoverpa zea). Biological Control 89, 53–60. doi: 10.1016/J.BIOCONTROL.2015.03.010
Lourenção A. L., de Moraes A. R. A., Godoy I. J., Ambrosano G. M. B. (2007). Efeito da infestação de Enneothrips flavens moulton sobre o desenvolvimento de cultivares de amendoim. Bragantia 66, 623–636. doi: 10.1590/S0006-87052007000400013
Mantzoukas S., Chondrogiannis C., Grammatikopoulos G. (2015). Effects of three endophytic entomopathogens on sweet sorghum and on the larvae of the stalk borer Sesamia nonagrioides. entomol. Exp. Appl. 154, 78–87. doi: 10.1111/eea.12262
Mantzoukas S., Eliopoulos P. A. (2020). Endophytic entomopathogenic fungi: A valuable biological control tool against plant pests. Appl. Sci. 10, 360. doi: 10.3390/app10010360
Mantzoukas S., Kitsiou F., Natsiopoulos D., Eliopoulos P. A. (2022). Entomopathogenic fungi: Interactions and applications. Encyclopedia 2, 646–656. doi: 10.3390/encyclopedia2020044
Mascarin G. M., Lopes R. B., Delalibera Í. Jr., Fernandes É. K. K., Luz C., Faria M. (2019). Current status and perspectives of fungal entomopathogens used for microbial control of arthropod pests in Brazil. J. Invertebr. Pathol. 165, 46–53. doi: 10.1016/j.jip.2018.01.001
Mc Coy C. W., Samson R. A., Boucies D. G. (1988). “Entomogenous fungi”. In: Handbook of Natural Pesticides, Vol. V, Microbial Insecticides. Ed. Ignoffa C. M., Mandava N. B. (Boca Raton, FL: CRC Press), 296, 151–236.
McGee P. A. (2002). Reduced growth and deterrence from feeding of the insect pest Helicoverpa armigera associated with fungal endophytes from cotton. Aust. J. Exp. Agric. 42, 995–999. doi: 10.1071/EA01124
Mendiburu F. d. (2021) Agricolae: Statistical procedures for agricultural research. Available at: https://CRAN.R-project.org/package=agricolae (Accessed March 15, 2022).
Michelotto M. D., Godoy I. J., Fávero A. P., Carrega W. C., Finoto E. L. (2012). Occurrence of enneothrips flavens moulton and Stegasta bosquella (Chambers) and its effects on agronomic traits of wild arachis accessions. Biosci. J. 29, 115–124.
Michelotto M. D., Santos J. F., Godoy I. J., Busoli A. C. (2015). “Resistência a pragas e doenças em amendoim,” in Tópicos em entomologia agrícola, vol. VIII. (Jaboticabal - SP,Brazil: Multipress). 105–116.
Moscardi F., Souza M. L., Castro M. E. B., Moscardi M. L., Szewczyk B. (2011). “Baculovirus pesticides: present state and future perspectives,” in Pichtel. microbes and microbial technology. Eds. Ahmad I., Ahmad F. (New York: Springer), 415–445.
Oliveira D. G. P., Pauli G., Mascarin G. M., Delalibera I. (2015). A protocol for determination of conidial viability of the fungal entomopathogens Beauveria bassiana and Metarhizium anisopliae from commercial products. J. Microbiol. Methods 119, 44–52. doi: 10.1016/j.mimet.2015.09.021
Parsa S., Ortiz V., Vega F. E. (2013). Establishing fungal entomopathogens as endophytes: towards endophytic biological control. J. Vis. Exp. 74, 50360. doi: 10.3791/50360
Peña-peña A. J., Santillán-galicia M. T., Hernández-lópez J., Guzmán-Franco A. W. (2015). Metarhizium pingshaense applied as a seed treatment induces fungal infection in larvae of the white grub Anomala cincta. J. Invertebr. Pathol. 130, 9–12. doi: 10.1016/j.jip.2015.06.010
Pinto J., Powell S., Peterson R., Rosalen D., Fernandes O. (2020). Detection of defoliation injury in peanut with hyperspectral proximal remote sensing. Remote Sens. 12, 3828. doi: 10.3390/rs12223828
Pirotta M. Z., Silva F. M., Michelotto M. D., Fávero A. P., Godoy I. J., Unêda-travisoli S. H. (2017). Resistance to Enneothrips flavens moulton and genetic parameters estimation in interspecific genotypes of peanut. Acta Sci. Agron. 39, 339–348. doi: 10.4025/actasciagron.v39i3.32776
Powell W. A., Klingeman W. E., Ownley B. H., Gwinn K. D. (2009). Evidence of endophytic beauveria bassiana in seed treated tomato plants acting as a systemic entomopathogen to larval Helicoverpa zea (Lepidoptera : Noctuidae). J. Entomol. Sci. 44, 391–396. doi: 10.18474/0749-8004-44.4.391
Powell W. A., Klingeman W. E., Ownley B. H., Gwinn K. D., Dee M., Flanagan P. C. (2007). Endophytic Beauveria bassiana in tomato yields mycosis in tomato fruitworm larvae. HortScience 42, 933.
Qayyum M. A., Wakil W., Arif M. J., Sahi S. T., Dunlap C. A. (2015). Infection of Helicoverpa armigera by endophytic beauveria bassiana colonizing tomato plants. Biol. Control 90, 200–207. doi: 10.1016/j.biocontrol.2015.04.005
Ramírez-Rodríguez D., Sánchez-Peña S. (2016). Endophytic Beauveria bassiana in zea mays: pathogenicity against larvae of fall armyworm, Spodoptera frugiperda. Southwest. Entomol. 41, 875–878. doi: 10.3958/059.041.0330
R Core Team (2020) R: A language and environment for statistical computing (Vienna, Austria: R Foundation for Statistical Computing). Available at: https://www.R-project.org/ (Accessed March 15, 2022).
Reddy N. P., Ali Khan A. P., Devi U. K., Sharma H. C., Reineke A. (2009). Treatment of millet crop plant (Sorghum bicolor) with the entomopathogenic fungus (Beauveria bassiana) to combat infestation by the stem borer, Chilo partellus swinhoe (Lepidoptera: Pyralidae). J. Asia-Pacif. Entomol. 12, 221–226. doi: 10.1016/j.aspen.2009.06.001
Resquín-Romero G., Garrido-Jurado I., Delso C., Ríos-Moreno A., Quesada-Moraga E. (2016). Transient endophytic colonizations of plants improve the outcome of foliar applications of mycoinsecticides against chewing insects. J. Invertebr. Pathol. 136, 23–31. doi: 10.1016/j.jip.2016.03.003
Russo M. L., Pelizza S. A., Vianna M. F., Allegrucci N., Cabello M. N., Toledo A. V., et al. (2019). Effect of endophytic entomopathogenic fungi on soybean glycine max (L.) merr. growth and yield. J. King Saud Univ. 31, 728–736. doi: 10.1016/j.jksus.2018.04.008
Sánchez-Rodríguez A. R., Raya-Díaz S., Zamarreño Á. M., García-Mina J. M., Del Campillo M. C., Quesada-Moraga E. (2018). An endophytic Beauveria bassiana strain increases spike production in bread and durum wheat plants and effectively controls cotton leafworm (Spodoptera littoralis) larvae. Biol. Control. 116, 90–102. doi: 10.1016/j.biocontrol.2017.01.012
Santos R.C.d., Freire R. M. M., Lima L. M. (2013). O Agronegócio do amendoim no brasil (Brasília, DF: Embrapa).
Sasan R. K., Bidochka M. J. (2012). The insect-pathogenic fungus Metarhizium robertsii (Clavicipitaceae) is also an endophyte that stimulates plant root development. Am. J. Bot. 99, 101–107. doi: 10.3732/ajb.1100136
Senthilraja G., Anand T., Durairaj C., Raguchander T., Samiyappan R. (2010). Chitin-based bioformulation of Beauveria bassiana and Pseudomonas fluorescens for improved control of leafminer and collar rot in groundnut. Crop Prot. 29, 1003–1010. doi: 10.1016/j.cropro.2010.06.002
Senthilraja G., Anand T., Kennedy J., Raguchander T., Samiyappan R. (2013). Plant growth promoting rhizobacteria (PGPR) and entomopathogenic fungus bioformulation enhance the expression of defense enzymes and pathogenesis-related proteins in groundnut plants against leafminer insect and collar rot pathogen. Physiol. Mol. Plant Pathol. 82, 10–19. doi: 10.1016/j.pmpp.2012.12.002
Shrivastava G., Ownley B. H., Augé R. M., Toler H., Dee M., Vu A., et al. (2015). Colonizaton by arbuscular mycorrhizal and endophytic fungi enhanced terpene production in tomato plants and their defense against a herbivorous insect. Symbiosis 65, 65–74. doi: 10.1007/s13199-015-0319-1
Silva A. C. L., Silva G. A., Abib P. H. N. (2020). Endophytic colonization of tomato plants by the entomopathogenic fungus Beauveria bassiana for controlling the south American tomato pinworm, Tuta absoluta. CABI Agric. Biosci. 1, 3. doi: 10.1186/s43170-020-00002-x
Tefera T., Vidal S. (2009). Effect of inoculation method and plant growth medium on endophytic colonization of sorghum by the entomopathogenic fungus beauveria bassiana. BioControl 54, 663–669. doi: 10.1007/s10526-009-9216-y
USDA (2021) Oilseeds and products annual. foreign agricultural service. Available at: https://apps.fas.usda.gov/newgainapi/api/Report/DownloadReportByFileName?fileName=Oilseeds%20and%20Products%20Annual_Brasilia_Brazil_04-01-2021 (Accessed January 10, 2022).
Vakili N. G. (1990). “Biocontrol of stalk rot in corn,” in Proceedings of the Forty-fourth Annual Corn and Sorghum Industry Research Conference, Chicago, IL. Washington, DC, December 6–7, 1989, American Seed Trade Association. 87–105.
Vega F. E. (2018). The use of fungal entomopathogens as endophytes in biological control: a review. Mycologia 110, 4–30. doi: 10.1080/00275514.2017.1418578
Vega F. E., Posada F., Aime M. C., Pava-Ripoll M., Infante F., Rehner S. A. (2008). Entomopathogenic fungal endophytes. Biol. Control 46, 72–82. doi: 10.1016/j.biocontrol.2008.01.008
Vidal S., Jaber L. R. (2015). Entomopathogenic fungi as endophytes: plant–endophyte–herbivore interactions and prospects for use in biological control. Curr. Sci. 109, 46–54. doi: 10.1038/jstor.org/stable/24905690
Virk S. S., Prostko E. P., Kemerait R. C., Abney M. R., Rains G. C., Powell C. T., et al. (2021). On-farm evaluation of nozzle types for peanut pest management using commercial sprayers. Peanut Science. 48, 87–96. doi: 10.3146/PS21-2.1
Wickham H. (2016) ggplot2: Elegant graphics for data analysis (New York: Springer-Verlag). Available at: https://ggplot2.tidyverse.org (Accessed March 15, 2022).
Keywords: biological control (CB), entomopathogen, groundnut (A. hypogaea L.), hypocreales, Lepidoptera
Citation: Vinha FB, Rojas LAC, Ramos Sales C, Monteiro Lima NS, Nascimento JD, De Carvalho LAL and Lemos EGDM (2023) Negative effects on the development of Chrysodeixis includens and Spodoptera cosmioides fed by peanut plants inoculated with entomopathogenic fungi. Front. Fungal Bio. 3:968528. doi: 10.3389/ffunb.2022.968528
Received: 14 June 2022; Accepted: 15 December 2022;
Published: 26 January 2023.
Edited by:
Maria Bonatelli, Helmholtz Association of German Research Centres (HZ), GermanyReviewed by:
Almudena Ortiz-Urquiza, University of Nottingham, United KingdomCopyright © 2023 Vinha, Rojas, Ramos Sales, Monteiro Lima, Nascimento, De Carvalho and Lemos. This is an open-access article distributed under the terms of the Creative Commons Attribution License (CC BY). The use, distribution or reproduction in other forums is permitted, provided the original author(s) and the copyright owner(s) are credited and that the original publication in this journal is cited, in accordance with accepted academic practice. No use, distribution or reproduction is permitted which does not comply with these terms.
*Correspondence: Fernando Belezini Vinha, ZmVybmFuZG9iZXZpQGhvdG1haWwuY29t; Eliana Gertrudes De Macedo Lemos, ZWxpYW5hLmxlbW9zQHVuZXNwLmJy
Disclaimer: All claims expressed in this article are solely those of the authors and do not necessarily represent those of their affiliated organizations, or those of the publisher, the editors and the reviewers. Any product that may be evaluated in this article or claim that may be made by its manufacturer is not guaranteed or endorsed by the publisher.
Research integrity at Frontiers
Learn more about the work of our research integrity team to safeguard the quality of each article we publish.