- 1Department of Genetics, Ribeirão Preto Medical School, University of São Paulo, Ribeirão Preto, Brazil
- 2Department of Biochemistry and Molecular Biology, Institute of Biological Sciences, Federal University of Goiás, Goiânia, Brazil
- 3Department of Microbiology, Institute of Biological Sciences, Federal University of Minas Gerais, Belo Horizonte, Brazil
The growth and development of organisms depend on nutrient availability. Dermatophytes must sense nutrient levels and adapt to the host environment to colonize human and animal keratinized tissues. Owing to the clinical importance of the Trichophyton genus, this study compared the expression profile of genes involved in metabolism, cell cycle control, and proteases in two Trichophyton species, Trichophyton rubrum, and Trichophyton interdigitale, in response to nutrients and environmental pH. In addition, we evaluated the activity of enzymes in the tricarboxylic acid, glyoxylate, and methylcitrate cycles. Moreover, the effects of interruption of the transcription factor pacC on T. interdigitale in the same conditions as for the wild-type strain were determined. Our analyses revealed specific responses in each species to the nutritional and pH variation. An improved adaptation of T. interdigitale to keratin was observed, compared with that of T. rubrum. T. rubrum growth in buffered keratin media indicated pH 8.0 as an optimal pH condition for metabolic activity, which differed from that for T. interdigitale. Tricarboxylic acid components in T. rubrum showed increased enzymatic activity and transcript accumulation. In T. interdigitale, a higher activity of enzymes in glyoxylate and methylcitrate cycles was observed, with no direct correlation to the transcriptional profile. T. interdigitale fungal metabolism suggests the requirement of anaplerotic pathways in the late cultivation period. The identified differences between T. rubrum and T. interdigitale may represent determinants for adaptation to the host and the incidence of infection with each species.
Introduction
Nutrient sensing and signaling modulate cell growth in various organisms. The ability to use different nutrition sources is crucial in pathogenic fungi, given that they must acquire nutrients from different host tissues. Dermatophytes are fungi that use keratin as a nutrient source, thereby infecting different niches, such as human and animal tissues (skin, hair, and nails), consequently causing cutaneous diseases (Grumbt et al., 2011; Gnat et al., 2019). Trichophyton rubrum and Trichophyton interdigitale are anthropophilic and cosmopolitan dermatophytes. They cause the diseases tinea corporis and tinea pedis, and represent two major pathogens that cause tinea unguium (Mehul et al., 2019; Martinez-Rossi et al., 2021).
Data on the molecular aspects of nutrient acquisition and cellular growth in dermatophytes remain scarce. Different genes are expressed in response to in vitro, ex vivo, or in vivo infection models (Zaugg et al., 2008; Peres et al., 2016; Tran et al., 2016). Additionally, in vitro analysis have revealed that during the growth and development of Trichophyton in glycine, keratin, and lipids as a carbon source, the extracellular pH shifts from acidic to alkaline due to the secretion of ammonia (Ferreira-Nozawa et al., 2003; Maranhão et al., 2007, 2011; Silveira et al., 2010; Mendes et al., 2012) and urea (Martins et al., 2020). Furthermore, the alkalinization of the culture medium by T. interdigitale when grown in keratin upregulates genes encoding subtilisins and metalloproteases (Maranhão et al., 2007), whereas acidic conditions regulate the expression of genes associated with the glyoxylate cycle (GC) and heat shock proteins (Peres et al., 2010). It was concluded that extracellular pH changes are necessary for regulating gene expression as an efficient strategy for colonization, development, and maintenance in the host tissue (Martinez-Rossi et al., 2012, 2017).
The highly conserved signal transducer pathway involved in the adaptive response to pH comprises at least six pal genes (palA, palB, palC, palF, palH, and palI). In many filamentous fungi, this signaling cascade mediates the proteolytic processing of the transcription factor PacC, which is functional in acidic and alkaline environments (Martinez-Rossi et al., 2012; Rossi et al., 2013; Li et al., 2021). A ΔpacC strain of T. interdigitale (formerly T. rubrum), which contains the disrupted pacC gene, shows reduced growth in nail fragments and the decreased secretion of keratinolytic proteases, directly associating this transcription factor to the proteolytic activity of T. interdigitale H6 (Ferreira-Nozawa et al., 2006).
In this study, we assessed the expression profile of genes involved in metabolism (idh1, idh2, idhp, icl, and meicl), cell cycle control (mad2 and mad2B), and proteolytic activity (sub3 and sub5) in the Trichophyton species, T. rubrum and T. interdigitale along with the T. interdigitale pacC mutant strain, in response to nutrient source and environmental pH. In addition, we evaluated the enzymatic activity and phosphorylation to explore their influence on fungal development. We further explored the effects of pacC deletion on T. interdigitale adaptation to pH variation, highlighting the relevance of this transcription factor in dermatophyte adaptation. Considering the high incidence of infections caused by dermatophytes, which affects nearly 25% of the world's population (Martinez-Rossi et al., 2021), especially by Trichophyton species, we aimed to increase the knowledge of the molecular mechanisms of these pathogens associated with nutrient acquisition, growth, and survival.
Materials and Methods
Trichophyton Strains
The strains used in this study included T. rubrum strain CBS 118892 (Westerdijk Fungal Biodiversity Institute, formerly CBS-KNAW, Utrecht, Netherlands); T. interdigitale H6 strain (ATCC MYA-3108) isolated at the University Hospital of Ribeirão Preto Medical School, São Paulo University, Brazil, and the ΔpacC strain obtained from T. interdigitale H6, which carries a disrupted pacC gene (Ferreira-Nozawa et al., 2006). The H6 strain was previously classified as T. rubrum and reclassified as T. interdigitale (Persinoti et al., 2018).
Fungal Culture Conditions
The strains were cultured on malt extract agar (pH 5.7) at 28°C. Conidia suspensions were obtained by flooding 22-day-old plates with sterilized 0.9% NaCl, recovering the liquid, mixing by vortex, and filtering through glass wool. Conidia concentration was estimated by counting in the Neubauer chamber. For growth in the liquid medium, 1 × 107 conidia from each strain were inoculated into 50 ml of sabouraud dextrose broth (SDB; pH 5.7). The 96 h incubation was performed under continuous shaking at 100 rpm at 28°C. For unbuffered culture conditions, we individually transferred mycelia from a 96 h culture to 100 ml of minimal medium (MM) (Cove, 1966) at pH 5.0, supplemented with 2 mM KH2PO4 and the following carbon sources: 55 mM glucose, 50 mM glycine, 55 mM glucose plus 50 mM glycine, or 4 g/L powdered ox hull keratin. Cultures were incubated under continuous shaking at 100 rpm for 24, 48, 72, and 96 h. For buffered conditions, the SDB 96 h culture mycelia were transferred to MM adjusted with 50 mM sodium citrate to pH 5.0 or with 50 mM Tris–HCl to pH 8.0. Buffered cultures were incubated for 24 h under continuous shaking. After each culture period, the mycelia were aseptically filtered and the pH of the medium was measured. The mycelium was dried in sterilized filter paper, weighed, frozen in liquid nitrogen, and stored at −80°C until use.
RNA Isolation, cDNA Synthesis, and Quantitative Real-Time PCR (RT-qPCR)
Total RNA was extracted from frozen mycelium using TRIzol™ reagent (Invitrogen, Carlsbad, CA, USA) following the manufacturer's instructions. The purified RNA from each experimental condition was reverse transcribed to cDNA using the High-Capacity cDNA Reverse Transcription Kit (Applied Biosystems, Foster City, CA, USA) following the manufacturer's instructions. The specific primer pairs for each gene are listed in Table 1. Quantitative real-time PCR (RT-qPCR) was performed in a final volume of 12.5 μl, containing 2.5 μM of each primer, 1 × SYBR Green (PCR mix), and 50 ng cDNA. Amplification was performed in the StepOnePlus™ Real-Time PCR system (Applied Biosystems). The PCR cycle consisted of 2 min at 50°C, 10 min at 95°C, and 40 cycles of 95°C for 15 s, and 60°C for 1 min. The melting curve analysis was performed using the Dissociation Curves Software version 1.0 (Applied Biosystems) to exclude primer dimers and unspecific PCR products. We calculated the relative transcript quantity using the ΔΔCt method (Livak and Schmittgen, 2001), with glyceraldehyde-3-phosphate dehydrogenase (gapdh, TERG_04402) and beta-tubulin (β-tub, TERG_07904) as endogenous reference genes (Jacob et al., 2012).
Data normalization was performed using the GenEx 5 MultiD Analyses AB tool (www.multid.se), and the relative quantification was expressed as 2−ΔΔCt, converted to log2. The reference conditions for relative expression displayed the lowest expression in each analysis, converting the resulting values into log2. The statistical analysis of the RT-qPCR data was performed using one-way ANOVA followed by the Bonferroni post-test correction using GraphPad Prism v. 5.01 (GraphPad Software, La Jolla, CA, USA).
Enzymatic Activity Assays
The mycelia obtained from T. rubrum CBS 118892 cultured in SDB for 96 h, and from cultures incubated for an additional 24 and 96 h in MM containing glucose, glycine, glucose plus glycine, or keratin, were collected for enzymatic assays. Furthermore, mycelia were collected from T. interdigitale H6 and pacC mutant strain of T. interdigitale in 96 h SDB cultures, and from 24 and 96 h cultures in MM supplemented with keratin. The mycelium was frozen and macerated in liquid nitrogen until a fine powder was obtained to analyze intracellular enzyme activities. The macerate was transferred to 50 ml Falcon tubes and cooled on ice. To 0.75 g of each macerated mycelium, 500 μl of Tris-HCl buffer (50 mM Tris-HCl, 2 mM MgCl2, 2 mM dithiothreitol, pH 8.0) was added. Samples were vortexed, dispensed in 2 ml tubes, and centrifuged for 30 min at 1,268 × g at 4°C. The supernatant (protein extract) was collected and kept on ice for use in the enzymatic assays. Proteins were quantified using Bradford reagent (Sigma-Aldrich, St. Louis, MO, USA) according to the manufacturer's instructions.
Intracellular Enzyme Assay
Isocitrate lyase (ICL) activity was determined using a phenylhydrazine-based assay (Ebel et al., 2006). The reaction was initiated by adding the isocitrate substrate and the glyoxylate phenylhydrazone product was determined at 324 nm (ε 16.8 mM−1 cm−1; Brock et al., 2001). Under the assessed conditions, one enzyme unit was defined as the amount of enzyme that produced 1 mmol of glyoxylate phenylhydrazone per minute. Specific activity was established as units per milligram (U mg−1) of protein used in the experiment.
Isocitrate lyase activity associated with protein dephosphorylation was measured at 96 h of cultivation. The assays were performed using FastAP™ Thermosensitive Alkaline Phosphatase (Thermo Fisher Scientific, Cleveland, OH, USA) by following the manufacturer's instructions. The supernatant (protein extract) was evaluated before and immediately after treatment with FastAP™.
The methylisocitrate lyase (MeICL) activity was determined based on the formation of phenylhydrazone pyruvate (Brock et al., 2001). The reaction was initiated with the addition of methylisocitrate substrate. The formation of phenylhydrazone pyruvate product was determined at 324 nm (ε 16.8 mM−1 cm−1). Under the analyzed conditions, one enzymatic activity unit was defined as the amount of enzyme that produced 1 mmol of phenylhydrazone pyruvate per minute. The specific activity was established as units per milligram (U mg−1) of protein used in the experiment.
The nicotinamide adenine dinucleotide (NAD+)-dependent isocitrate dehydrogenase (IDH; EC 1.1.1.41) activity was determined in the assay as nicotinamide adenine dinucleotide, reduced form (NADH) formation (Lin and Mcalister-Henn, 2002). The reaction was initiated with the addition of the protein extract. The product formation was determined at 340 nm, and 1 unit of enzymatic activity was defined as the formation of 1 mmol of NADH per minute under the experimental conditions. The specific activity was established as units per milligram (U mg−1) of protein used.
The NADP+-dependent isocitrate dehydrogenase (IDHP; EC 1.1.1.42) activity was determined in the assay as NADPH formation (Keys and Mcalister-Henn, 1990). The reaction was initiated with the addition of the protein extract. The product formation was determined at 340 nm, defining 1 unit of enzymatic activity as the formation of 1 mmol of NADPH per minute under experimental conditions. The specific activity was established as (U mg−1) of protein.
In silico Analysis of the DNA-Binding Consensus for the PacC Transcriptional Regulator
Bioinformatics data mining in the genome of T. interdigitale H6 was performed while searching for the binding site for PacC in the promoter region of the evaluated genes. We analyzed 1,000 nucleotides upstream of the start codon to locate the consensus for PacC protein, described as GCCA(A/G)G, and performed the same for the reverse and complementary sequence C(C/T)TGGC (Tilburn et al., 1995; Espeso and Penalva, 1996).
Results
Modulation of the Extracellular pH and Mycelial Growth
When Trichophyton species were cultured in unbuffered MM, the extracellular pH shifted from acidic to alkaline except in the case of glucose, in which the pH remained acidic during 96 h of culture (Figure 1A). A similar accumulation in mycelial mass was observed for T. rubrum CBS 118892 in both glycine and glucose during 96 h of cultivation. Notably, the combination of these nutrient sources enabled further accumulation of fungal mass after 72 h (glycine plus glucose, in purple) (Figure 1B). The mycelial mass developed during growth in keratin increased over time in all strains; the mycelial mass accumulation occurred independently of the pacC gene in T. interdigitale H6.
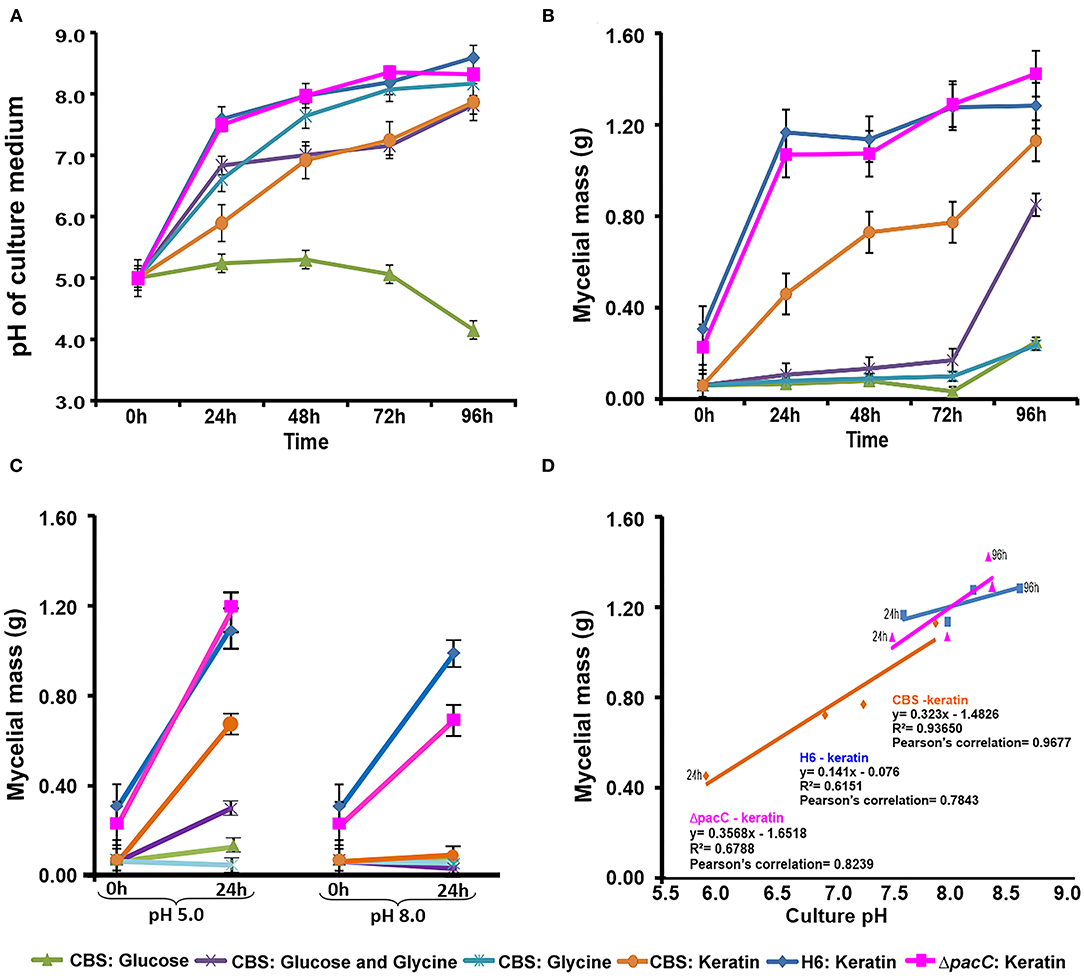
Figure 1. Time-dependent correlation between culture pH and mycelial mass. (A) Culture pH in unbuffered media (initial pH: 5.0). (B) Mycelial mass production in unbuffered media. (C) Mycelial mass production in buffered media. (D) Mycelial mass production and culture pH correlations. CBS, Trichophyton rubrum CBS 118892; H6, wild-type Trichophyton interdigitale; ΔpacC, Trichophyton interdigitale H6 strain carrying a disrupted pacC gene.
In buffered media (Figure 1C), alkaline pH suppressed the mycelial mass accumulation to a greater extent than in acidic pH. Only the growth of T. interdigitale H6 cultured in keratin was not inhibited by the alkaline conditions, independently of pacC expression. T. rubrum CBS 118892 showed a lower growth rate than that of T. interdigitale H6 in keratin at acidic pH, and the growth of T. rubrum CBS 118892 was inhibited in buffered (pH 8.0) medium (Figure 1D).
In silico Analysis of the PacC DNA-Binding Domain
Analyses of the promoter region revealed the PacC consensus in the sub5, icl, and meicl genes among the evaluated genes (idh1, idh2, icl, meicl, mad2, mad2B, sub3, and sub5). The sequencing quality of the idhp gene promoter region did not allow the analysis of 1,000 upstream base pairs.
Expression Profile of Genes Involved in Metabolic Pathways
The expression levels of genes associated with the tricarboxylic acid (TCA) cycle, idh1, idh2, and idhp, in T. rubrum CBS 118892 were relatively higher in 96 h cultures in unbuffered MM in the presence of glucose, and glucose plus glycine (Figure 2A). However, in glycine, these genes were upregulated after 72 h of cultivation. A concomitant increase in the expression levels of methylisocitrate lyase (meicl) and isocitrate lyase (icl) genes was observed at 72 h that lasted until 96 h.
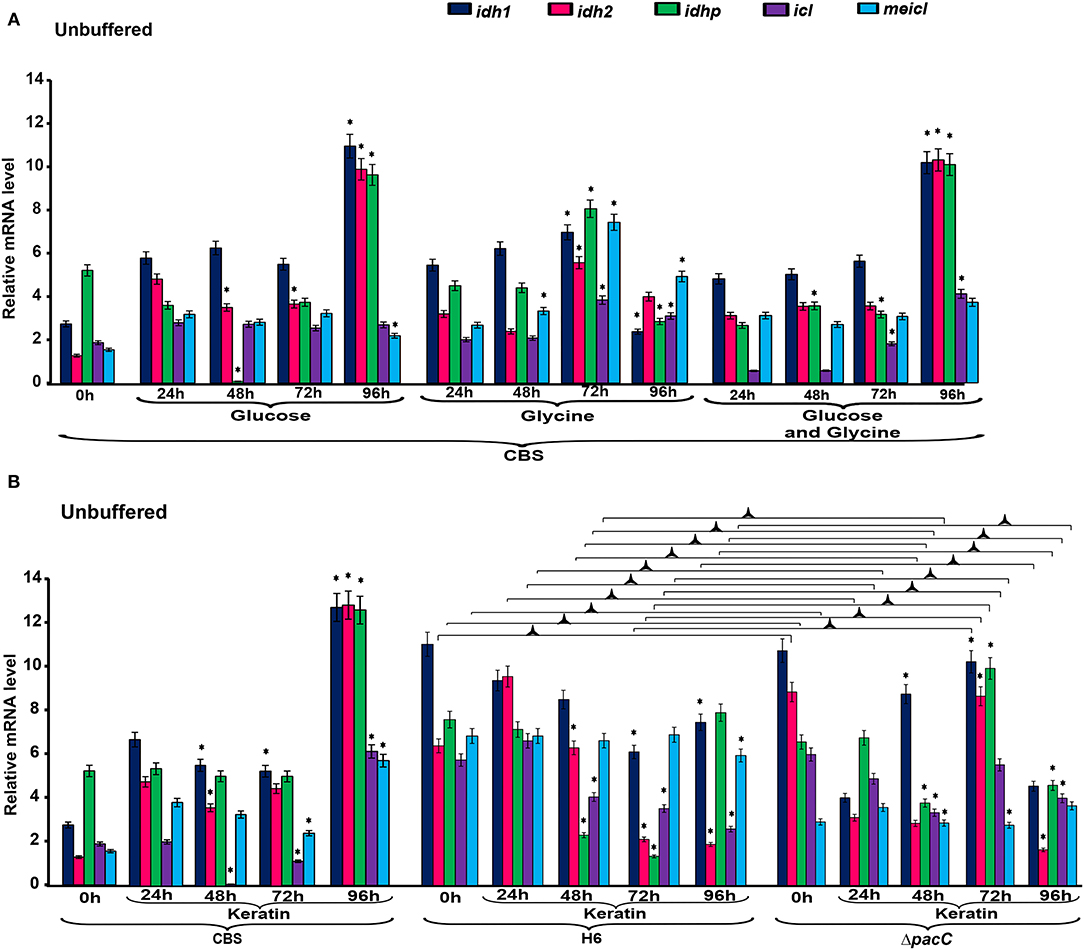
Figure 2. Differential expression of genes involved in metabolism under unbuffered conditions. (A) T. rubrum CBS 118892 grown in glucose, glycine or glucose plus glycine. (B) T. rubrum CBS 118892 and T. interdigitale strains grown in keratin medium. Expression of idh1, idh2, idhp, icl, and meicl genes was analyzed by RT-qPCR. The 0 h time point corresponds to 96 h cultures in Sabouraud dextrose broth. Significant differences (P < 0.05) in gene expression compared to that at 24 h for each nutritional source are indicated by *. The condition associated with the lowest expression was chosen as a reference for relative expression (idhp/T. rubrum in minimal medium containing glucose at 48 h). CBS, Trichophyton rubrum CBS 118892; H6, wild-type Trichophyton interdigitale; ΔpacC, Trichophyton interdigitale H6 strain carrying a disrupted pacC gene.
The expression of idh1, idh2, and idhp was induced in T. rubrum CBS 118892 cultivated in keratin-containing medium after 96 h of cultivation (Figure 2B). The deletion of pacC upregulated the same TCA cycle genes at 72 h, presenting a different pattern from that of the wild-type strain T. interdigitale H6. Under buffered conditions (Figure 3A), T. rubrum CBS 118892 gene expression was less affected during growth in glucose plus glycine compared with that in other evaluated conditions. The deletion of pacC directly influenced the TCA cycle gene expression: keratin exposure (pH 8.0) induced idh2 and idhp expression in the wild-type T. interdigitale H6 strain and induced idh1 expression in the T. interdigitale mutant ΔpacC strain (Figure 3B).
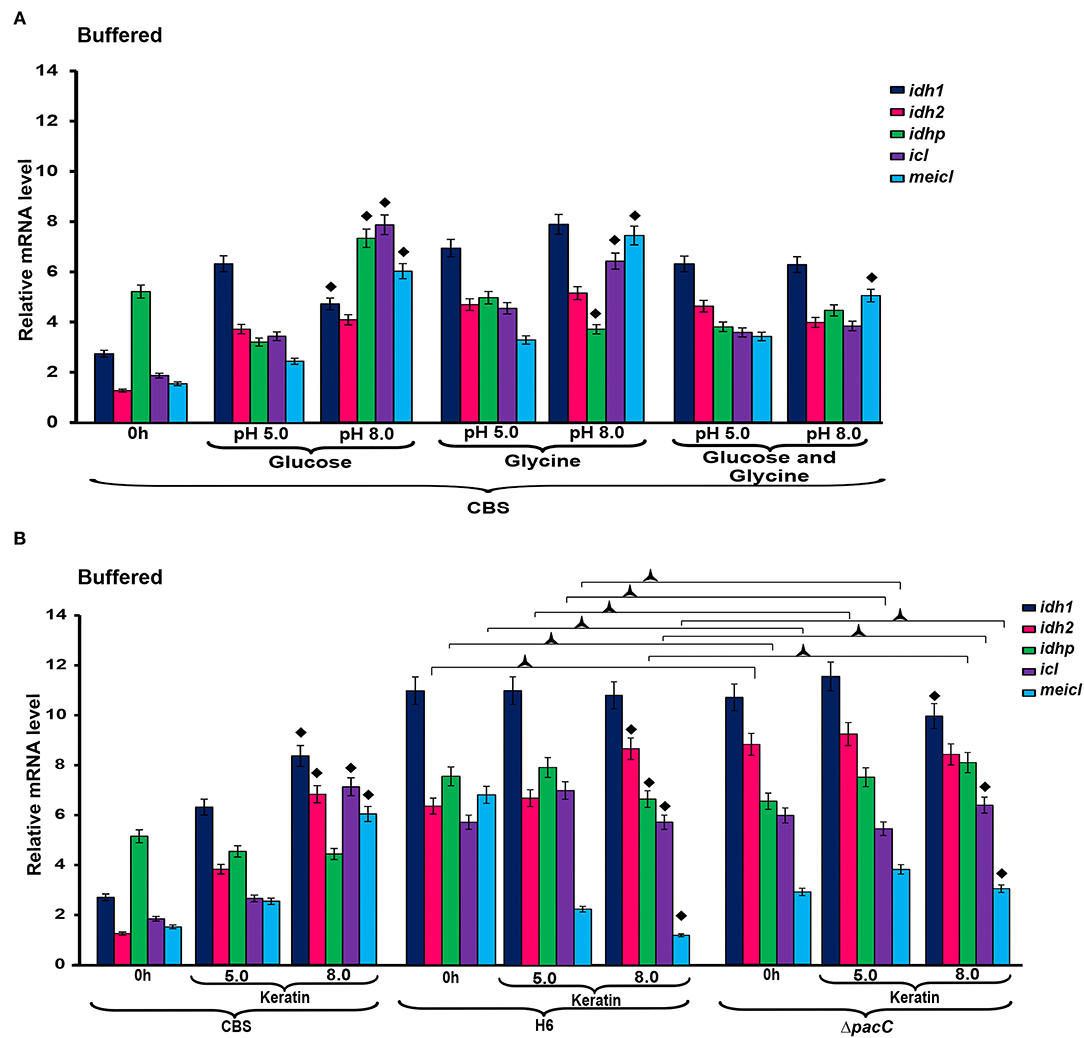
Figure 3. Differential expression of genes involved in metabolism under buffered conditions. (A) T. rubrum CBS 118892 grown in glucose, glycine or glucose plus glycine. (B) T. rubrum CBS 118892 and T. interdigitale strains grown in keratin medium. Expression of idh1, idh2, idhp, icl, and meicl genes was analyzed by RT-qPCR. The 0 h time point corresponds to 96 h cultures in Sabouraud dextrose broth. Significant differences (P < 0.05) in gene expression compared to that at 24 h of each nutritional source are indicated as ♦ for pH 8.0 compared to pH 5.0 of the respective nutritional source; ΔpacC strain compared to wild-type Trichophyton interdigitale H6 in the same condition is indicated as . The condition associated with the lowest expression was chosen as a reference for relative expression (idhp/T. rubrum in minimal medium containing glucose at 48 h). CBS, Trichophyton rubrum CBS 118892; H6, wild-type Trichophyton interdigitale; ΔpacC, Trichophyton interdigitale H6 strain carrying a disrupted pacC gene.
Intracellular Enzyme Activity
Analysis of the intracellular enzyme activity, such as the TCA cycle components IDH and IDHP, GC component ICL, and methylcitratecycle (MC) component MeICL (Table 2) revealed that ICL in T. rubrum CBS 118892 and T. interdigitale ΔpacC strains showed the highest specific activity in SDB medium. In contrast, the highest IDH activity was observed in T. interdigitale wild-type H6, when cultured in keratin. ICL activity was the highest in SDB compared with that in other cultivation conditions in T. rubrum CBS 118892, T. interdigitale H6, and in the T. interdigitale ΔpacC strains. T. rubrum CBS 118892 cultured in glucose plus glycine showed a high IDH and IDHP activities at 96 h.
Trichophyton interdigitale wild-type H6 strain showed high IDHP activity when cultured in keratin. This activity was dependent on the pacC gene. At 24 h, IDHP activity in T. interdigitale H6 strain was similar to that in T. rubrum CBS 118892; however, wild-type T. interdigitale H6 showed an increased IDHP activity compared with that of T. rubrum CBS 118892 at 96 h. The T. interdigitale ΔpacC strain showed higher IDH and ICL activities than those of the wild-type T. interdigitale H6 strain.
We investigated the potential effect of phosphorylation on ICL activity (Table 3). An increase in ICL activity was observed in dephosphorylated protein extracts. Although in the untreated samples, ICL activity was higher in the T. interdigitale ΔpacC mutant than that in the wild-type T. interdigitale H6 strain, no significant difference was observed in the activity of treated protein extracts.
The enzymatic activity presented in a heat map (Figure 4A) highlights two groups, one with the TCA enzymes IDH and IDHP, and another with the enzymes from anaplerotic pathways (ICL from GC, and MeICL from MC). The enzymatic activity of T. rubrum CBS 118892 grown in glucose, comparing 96 h with the 24 h time points, is presented in Figure 4B. This profile was also observed for the T. interdigitale mutant ΔpacC grown in keratin. The enzymatic activity in T. rubrum CBS 118892 in response to glycine and to glucose plus glycine is presented in Figures 4C,D, respectively. This late profile was similar to that of the enzymatic activity in wild-type T. interdigitale H6 in response to keratin.
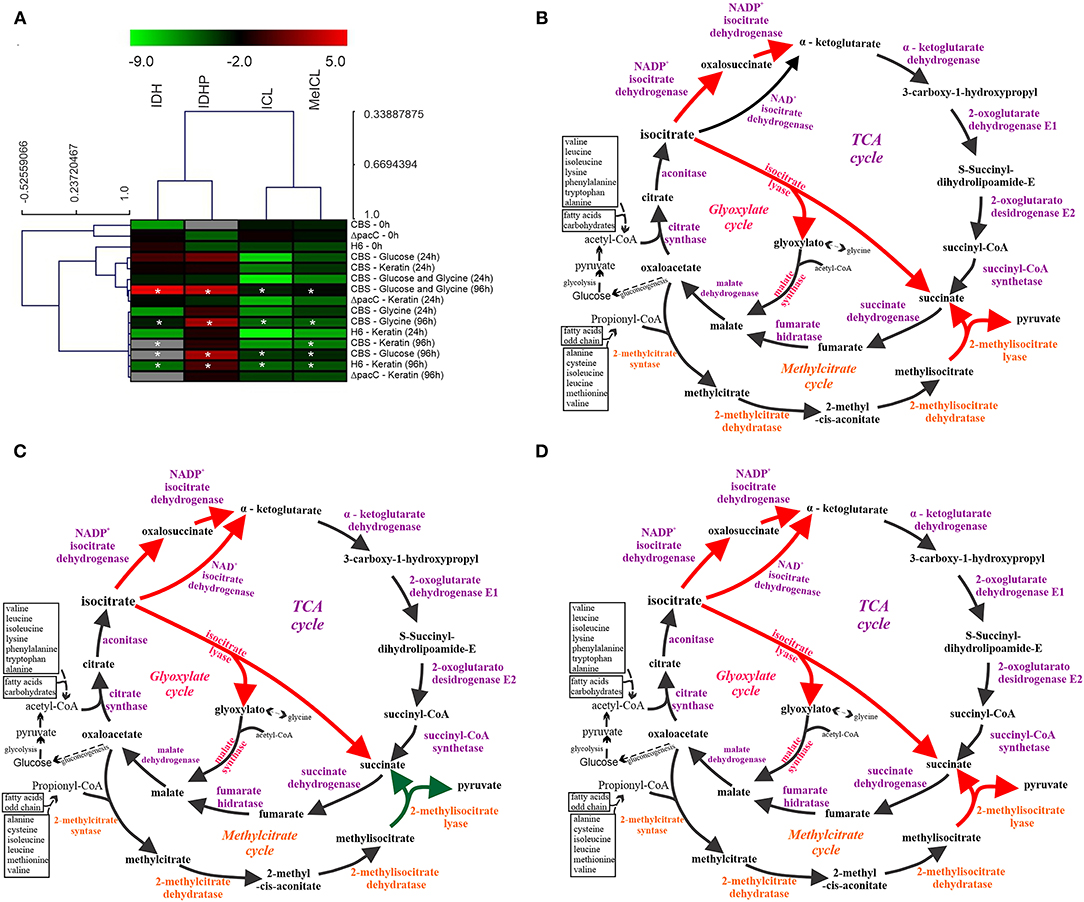
Figure 4. Schematic representation of TCA, GC, and MC integration in response to nutritional source in Trichophyton spp. (A) Heat map of the enzymatic activities represented as values in log2. Hierarchical clustering was performed using Pearson correlation. In gray, no enzymatic activity detected. Values are color-coded according to the top color bar. Asterisks (*) indicate significant differences in 96 h activity compared to that at 24 h in the same nutritional condition. (B) Schematic representation of the metabolic activity of Trichophyton rubrum grown in glucose and of the Trichophyton interdigitale H6 mutant ΔpacC strain grown in keratin. (C) T. rubrum grown in glycine. (D) T. rubrum grown in glucose plus glycine, and wild-type T. interdigitale H6 grown in keratin. Enzymes marked in orange are exclusive of the MC and those exclusive of GC are marked in pink. Dashed black arrows indicate routes that have been simplified. Red arrows indicate higher enzyme activity than that marked using green arrows (according to the heatmap). TCA, tricarboxylic acid cycle; GC, glyoxylate cycle; MC, methylcitrate cycle; CBS, Trichophyton rubrum CBS 118892; H6, wild-type Trichophyton interdigitale; ΔpacC, Trichophyton interdigitale H6 strain carrying a disrupted pacC gene.
Transcriptional Profile of Genes Involved in Mitosis Control
To evaluate the mechanisms via which nutritional source and pH influence cell cycle-associated genes in Trichophyton species, we assessed the transcriptional profile of mad2 and mad2B genes. The gene mad2B was upregulated in T. rubrum CBS 118892 at 96 h in the unbuffered media. The gene mad2 was similarly upregulated in T. rubrum CBS 118892 at 96 h except in response to glycine, in which an earlier transcript accumulation at 72 h was observed (Figure 5A). Furthermore, both genes were upregulated in buffered-medium (pH 8.0), suggesting an expression in response to pH in T. rubrum CBS 118892 (Figure 5B). In unbuffered medium, mad2 in the wild-type T. interdigitale H6 was downregulated at 48 h, as observed in the T. interdigitale mutant ΔpacC strain. However, the downregulation was prolonged in the mutant strain to a different extent from that in the wild-type T. interdigitale H6 strain (Figure 5C). The transcriptional profile observed in the buffered medium also highlights the effects of pacC on the mad2 gene (Figure 5D).
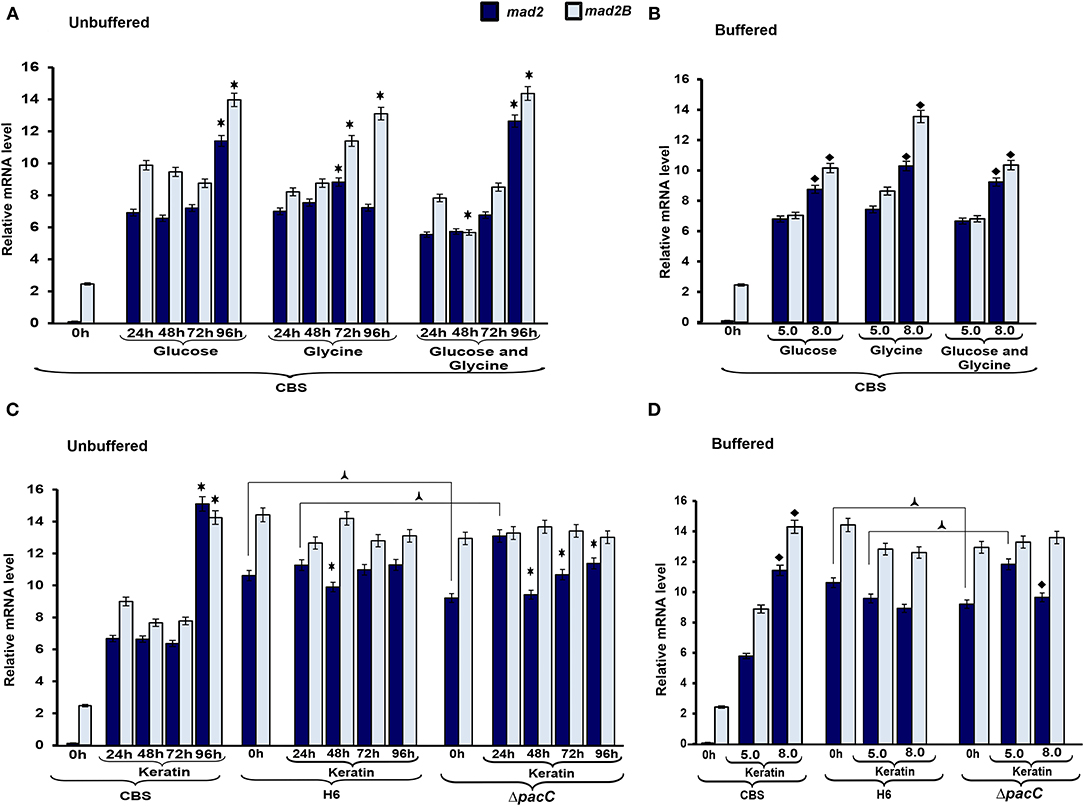
Figure 5. Expression profile of genes associated with cell cycle control. Expression of the genes mad2 and mad2B was analyzed during growth in unbuffered (A,C) and buffered (B,D) culture media. The 0 h condition corresponds to mycelia from 96 h Sabouraud dextrose broth cultures. Data represent median values from three independent experiments. Significant differences (P < 0.05) in gene expression were compared to that at 24 h of each nutritional source (*A,C), pH 8.0 compared to pH 5.0 of the respective nutritional source (♦B,D), Trichophyton interdigitale H6 mutant ΔpacC strain compared to wild-type T. interdigitale H6 in the respective conditions ( C,D). The condition associated with the lowest expression was chosen as a reference for relative expression (mad2/Trichophyton rubrum in Sabouraud dextrose broth). CBS, Trichophyton rubrum CBS 118892; H6, wild-type Trichophyton interdigitale; ΔpacC, Trichophyton interdigitale H6 strain carrying a disrupted pacC gene.
Transcriptional Profile of Endoproteases
To evaluate the mechanisms via which nutritional source and pH influence protease production in Trichophyton species, we assessed the transcriptional profile of subtilisin endoproteases encoded by sub3 and sub5. The overall profile in T. rubrum CBS 118892 reveals the regulation of sub3 and sub5 genes over time in response to the culture media (Figure 6). In response to pH, the transcripts of both genes accumulated at pH 8.0 in T. rubrum CBS 118892, except for sub3 at pH 5.0 in response to keratin. Moreover, the wild-type T. interdigitale H6 inversely repressed the transcriptional accumulation of sub5, compared with that in T. rubrum CBS 118892. Although also repressed, the T. interdigitale ΔpacC strain showed a considerably higher repression at 96 h, whereas the wild-type T. interdigitale H6 strain showed a higher repression at 72 h (Figure 6C). Both T. interdigitale wild-type H6 and the mutant T. interdigitale ΔpacC strains were responsive to buffered pH.
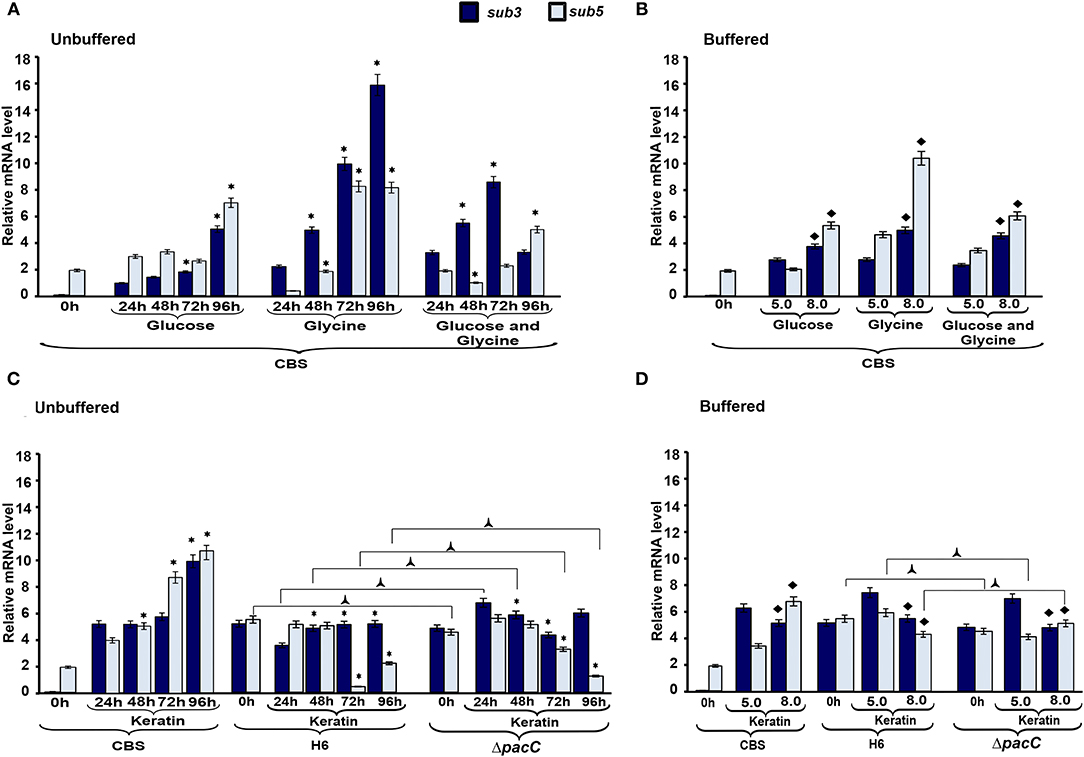
Figure 6. Expression profile of protease genes. The expression of sub3 and sub5 genes in Trichophyton rubrum CBS 118892 and Trichophyton interdigitale H6 was analyzed during growth in unbuffered (A,C) and buffered (C,D) culture medium. Time 0 h indicates Sabouraud dextrose broth cultures at 96 h. Data represent median values from three independent experiments. Significant differences (P < 0.05) in gene expression were compared to that at 24 h of each nutritional source (*A,C), pH 8.0 compared to pH 5.0 of the respective nutritional source (♦B,D), and T. interdigitale H6 mutant ΔpacC compared to wild-type T. interdigitale H6 in the respective conditions (C,D). The condition associated with the lowest expression was chosen as a reference for relative expression (sub3/T. rubrum in Sabouraud dextrose broth). CBS, Trichophyton rubrum CBS 118892; H6, wild-type Trichophyton interdigitale; ΔpacC, Trichophyton interdigitale H6 strain carrying a disrupted pacC gene.
Discussion
Fungi contain advanced complex signal transduction networks allowing their adaptation to pH fluctuation, triggering the expression of several genes to facilitate their survival in a broad range of environmental conditions (Rossi et al., 2013; Martins et al., 2019a,b). The pH shift and nutritional source are of great importance in maintaining dermatophyte growth (Martinez-Rossi et al., 2017). The hydrolysis of skin proteins releases amino acids, such as glycine, promoting the modulation of the extracellular pH from acidic to alkaline conditions. This contributes to host tissue damage and favors dermatophyte colonization (Maranhão et al., 2007; Silveira et al., 2010). However, data on the molecular aspects of nutrient acquisition and cellular growth in dermatophytes remain scarce.
Here, we evaluated pH modulation in response to nutrient variation in two Trichophyton species, T. rubrum CBS 118892 and T. interdigitale H6, and in the T. interdigitale pacC mutant strain. It is well-documented that these pathogens' in vitro growth depends on the initial culture pH. Our results show the correlation between the nutritional source and pH variation in fungus development. In T.rubrum CBS 118892, the alkalinization was glucose-repressible, as previously observed for T. interdigitale H6 (Maranhão et al., 2007; Mendes et al., 2012) and Candida albicans (Vylkova et al., 2011). This trend occurs independently of pacC (Mendes et al., 2012). Furthermore, the extracellular alkalinization and growth of the wild-type T. interdigitale H6 were not affected by the disruption of the pacC gene during growth in keratin as a nutritional source, as previously reported (Silveira et al., 2010).
The nutrient source influenced the growth rate of T. rubrum CBS 118892 in buffered conditions. After shifting from SDB to keratin-containing culture media, T. rubrum CBS 118892 mycelial mass accumulation was highly impacted by the buffered (pH 8.0) condition (Figure 1C). At 24 h, the pH 8.0 media inhibited T. rubrum CBS 118892 growth compared with the growth in unbuffered media at the same time point (Figures 1B,C). Conversely, the mycelial mass accumulated in buffered pH 5.0 was higher than that in the unbuffered media at 24 h. In this buffered condition, the mycelial mass was similar to the mass observed at 48 h, reflecting a good condition for T. rubrum CBS 118892 mycelial mass accumulation.
In unbuffered media, both glucose and glycine impaired T. rubrum CBS 118892 mycelial mass accumulation over time. The association of glucose plus glycine induced mycelial mass accumulation only after 72 h of cultivation, suggesting a late adaptation to the environment created by the combination of sugar and amino acid. T. interdigitale H6 mycelial mass was slightly affected comparing keratin-containing media buffered in pH 5.0 and pH 8.0. The growth profile was not affected in buffered culture media compared with that in the unbuffered keratin-containing media at 24 h; this indicated that independent of buffering the pH in culture media, T. interdigitale H6 development was not impaired. In contrast, T. rubrum CBS 118892 was more sensitive to pH fluctuation than the T. interdigitale H6 strain during growth in keratin, reflecting a different adaptation process between species. Overall, a positive correlation was observed between pH and mycelial mass, indicating the influence of extracellular pH in dermatophyte growth.
To determine the influence of the nutritional conditions and pH variation on gene expression in the two Trichophyton species, we analyzed the transcriptional profiles of genes involved in the TCA cycle (idh1, idh2, and idhp), GC (icl), MC (meicl), cell cycle control (mad2 and mad2B), and keratin degradation (sub3 and sub5). In T. rubrum CBS 118892, under unbuffered conditions, the TCA-related genes idh1, idh2, and idhp were induced at 96 h, with a slightly lower induction observed in glycine than that in glucose and glucose plus glycine (Figure 2). An increased regulation of TCA-related genes was observed compared with the regulation of GC and MC genes icl and meicl. Both the TCA cycle-related IDHs and GC-related ICL enzymes are dependent on isocitrate as a substrate. IDH enzymes oxidatively decarboxylate isocitrate into α-ketoglutarate, whereas ICL forms glyoxylate and succinate in the glyoxysomes (Kornberg, 1966). MeICL catalyzes the formation of succinate and pyruvate via the cleavage of 2-methylisocitrate in the last reaction of the MC (Luttik et al., 2000). Our results suggest a preference in T. rubrum CBS 118892 for TCA cycle activation instead of a bypass to the GC supporting the fate of isocitrate. In the presence of glycine, the transcript accumulation observed at 72 h shows the TCA cycle activation together with MeICL gene expression, suggesting the combined activation of the anaplerotic pathway. This induction persisted until 96 h. This pattern was observed at 96 h of cultivation in keratin-containing media.
In the presence of keratin under unbuffered conditions (Figure 2B), T. interdigitale H6 showed a pacC-dependent activation of the TCA-related genes at 72 h. The regulation of TCA cycle genes occurred only at 96 h. The lack of transcription factor induced an earlier activation of TCA modulation compared with that in the wild-type T. interdigitale H6 strain. The icl and meicl genes showed an opposite pattern of modulation in unbuffered keratin media at 72 and 96 h of cultivation: at 72 h, icl was repressed whereas meicl was not differentially expressed in the wild-type T. interdigitale H6 strain. In the same condition, icl was not differentially expressed, whereas meicl was repressed in the pacC mutant strain of T. interdigitale H6. The PacC DNA-binding consensus sequence in the promoter region of both genes indicated a PacC-dependent regulation. This regulation was not observed in cultures in buffered media which supports the role of pH fluctuation in pacC activity (Figure 3B). In buffered pH, glucose plus glycine affected gene modulation in T. rubrum CBS 118892 to a less extent between pH 5.0 and 8.0 conditions (Figure 3A). T. rubrum CBS 118892 growth in buffered keratin-containing media suggests that pH 8.0 may represent an optimal condition for metabolic activity. This pattern was not observed in T. interdigitale H6 (Figure 3B).
The enzymatic assays revealed an overall increased activity at 96 h compared with that at 24 h (Figures 4B–D). Only in T. rubrum CBS 118892, an opposite pattern was observed for the MC enzyme MeICL when grown in glycine or keratin. Moreover, PacC regulates metabolic activity, given the reduced IDHP activity and increased ICL and MeICL activities in the T. interdigitale H6 mutant strain, compared with those in the wild-type T. interdigitale H6 (Table 2). During T. rubrum CBS 118892 growth in glucose for 96 h, IDHP activity increased by ~3-fold, ICL activity increased by 16-fold, and MeICL activity increased by 2-fold. The activity of TCA-related enzymes increased in T. rubrum CBS 118892, whereas higher GC and MC activities were observed in T. interdigitale H6, suggesting that fungal metabolism requires anaplerotic pathways in the late growth stages. T. rubrum CBS 118892 growth in glucose plus glycine for 96 h was showed IDHP, ICL, and MeICL activities that were similar to the sum of the respective enzyme activities at 96 h of growth in glucose or glycine. The enzymatic profile differs from the transcript accumulation pattern observed at the 96 h time point.
One of the mechanisms to control the molecular flow between TCA and GC involves regulating the active forms of IDH and ICL via phosphorylation. In prokaryotes, such as Escherichia coli, the phosphorylation of ICL enzyme results in its activation (Robertson et al., 1988); in contrast, in eukaryotes, such as Saccharomyces cerevisiae (Lopez-Boado et al., 1988) and Paracoccidioides brasiliensis (Cruz et al., 2011), phosphorylation reduces ICL activity, leading to inactivation. In our analyses, we observed a time-dependent increase in ICL activity (Table 2). A significant difference in ICL activity was observed between T. interdigitale H6 mutant strain ΔpacC and wild-type T. interdigitale H6 strains cultured in keratin at 96 h. However, no significant difference was observed in ICL activity between T. interdigitale H6 mutant strain ΔpacC and the wild-type T. interdigitale H6 strain cultured in the same conditions after in vitro dephosphorylation (Table 3). These results indicate a role of the PacC transcription factor upon ICL phosphorylation. In Aspergillus fumigatus, phosphorylation does not affect the catalytic activity of ICL (Ebel et al., 2006). P. brasiliensis shows a reversible mechanism of inactivation/activation via phosphorylation of ICL, regulated by the carbon source, in a rapid adaptation to changes in environmental conditions (Cruz et al., 2011). This mechanism may also occur in T. rubrum and T. interdigitale.
The effects of nutrient-sensing in growth and cell cycle progression have been extensively evaluated, mainly in yeasts (Busti et al., 2010; Gutteridge et al., 2010; Yanagida et al., 2011; Alberghina et al., 2012). To evaluate the mechanisms via which nutritional source and pH influence cell cycle-associated genes in Trichophyton species, we assessed the transcriptional profile of mad2 and mad2B genes. Both participate in inhibiting the anaphase-promoting complex (Chen and Fang, 2001; Bhat et al., 2015). We observed that in unbuffered conditions, the expression of both genes was induced in the latest time points evaluated (Figure 5). Buffering the media promoted the accumulation of transcripts in T. rubrum CBS 118892 at pH 8.0 to a different extent from that in the wild-type T. interdigitale H6. In T. rubrum CBS 118892, growth in the unbuffered medium for 96 h resulted in the upregulation of mad2 and mad2B, except in glycine. In this latest condition, similar to the TCA cycle genes, the induction was observed earlier at 72 h. Under buffered (pH 8.0) conditions, mad2 and mad2B expression is induced compared with that at pH 5.0. As the overall growth of T. rubrum CBS 118892 was inhibited at pH 8.0 (Figure 1), the gene expression profile suggests that when fungi cannot modulate environmental pH, they trigger the regulation of cell cycle control. Moreover, in T. interdigitale, the pacC deletion affected the transcription of mad2 at pH 5.0 in buffered medium, and at pH 5.0 under unbuffered conditions, both at 24 h of cultivation (Figures 5C,D). Although the mad2 gene does not present the consensus for PacC, our results indicate that this transcription factor is important in regulating the earlier transcription of mad2. The mad2 gene has been previously considered to be differentially expressed at pH 5.0, but not at pH 8.0, based on SSH analysis after 1 h cultivation in MM supplemented with glucose, sodium nitrate, and inorganic phosphate (Silveira et al., 2010).
The most important nutritional source for the growth of dermatophytes involves keratinous substrates. The breakdown of these molecules is promoted by secretory proteases, which have specificity for different substrates (Chen et al., 2010; Tran et al., 2016). Here, we analyzed the transcript accumulation of two subtilisin endoproteases encoded by sub3 and sub5. The higher expression of sub3 and sub5 genes in T. rubrum CBS 118892 was observed in glycine or keratin-containing media, in response to amino acid and protein sources (Figure 6). The metabolism of amino acids, such as glycine, released from skin proteins, results in the secretion of ammonia and shifts the ambient pH from acidic to alkaline (Maranhão et al., 2007; Silveira et al., 2010; Mendes et al., 2012; Martinez-Rossi et al., 2017). A glycine-rich environment is well-tolerated by T. rubrum CBS 118892, thereby enhancing sub3 and sub5 transcript accumulation. Moreover, the upregulation in glucose in 96 h cultures suggests that these genes may also be required for the later stages of development in a non-protein medium. Previously, the expression of genes coding for metalloproteases and subtilisins has been reported to remain relatively stable during T. rubrum growth in glucose, whereas they are upregulated in culture media containing collagen, elastin, keratin salt, or human skin (Leng et al., 2009). In unbuffered keratin media, the proteolytic activity of T. rubrum CBS 118892 increased over time, whereas the overall proteolytic profile of T. interdigitale H6 mutant strain ΔpacC showed a decreased activity over time. The same pattern was not observed in the wild-type T. interdigitale H6 strain reflecting PacC-associated regulation. Under buffered conditions, both sub3 and sub5 genes showed increased transcript accumulation in T. rubrum CBS 118892 under alkaline condition, except in keratin-containing media. Both T. rubrum CBS 118892 and T. interdigitale H6 repress sub3 transcript accumulation in keratin, independently of pacC. The transcript accumulation of sub5, which presents the consensus for PacC, increased in T. rubrum CBS 118892 and ΔpacC mutant of T. interdigitale H6 strains; whereas, it reduced in wild-type T. interdigitale H6, confirming a PacC-dependent modulation.
Our data show that although dermatophytes are strikingly similar in genetic content (Martinez et al., 2012), the mechanisms for adaptation to environmental conditions and the transcriptional responses to nutritional sources and pH are distinct. Also, strains recently isolated from patients may show different responses concerning the enzymatic activity profile (Gnat et al., 2018). These differences may represent determinants for the incidence of infection with each species.
Data Availability Statement
The original contributions presented in the study are included in the article/supplementary materials, further inquiries can be directed to the corresponding author/s.
Author Contributions
AC and AR designed the project. AC, RS, NP, GT, and NM performed the experiments. AC, RS, MM, NP, NM-R, and AR wrote the manuscript. AR and NM-R supervised the study and prepared the manuscript. All authors participated in data analysis, in the critical revision of the manuscript, and approved the final version.
Funding
This work was supported by research grants from the Brazilian Funding Agencies FAPESP (Proc. No. 2019/22596-9 and Fellowship Nos. 2018/11319-1 to MM, 2009/08411-4 to NP, and 2009/15426-8 to GT), CNPq (Proc. Nos. 305797/2017-4 and 304989/2017-7), CAPES (Finance Code 001), and FAEPA of the HCFMRP-USP.
Conflict of Interest
The authors declare that the research was conducted in the absence of any commercial or financial relationships that could be construed as a potential conflict of interest.
Publisher's Note
All claims expressed in this article are solely those of the authors and do not necessarily represent those of their affiliated organizations, or those of the publisher, the editors and the reviewers. Any product that may be evaluated in this article, or claim that may be made by its manufacturer, is not guaranteed or endorsed by the publisher.
Acknowledgments
The authors thank S. H. Castrechini, V. M. Oliveira, M. Mazucato, M. D. Martins for technical assistance and P. R. Sanches for bioinformatics analyses. We also thank Matthias Brock (Leibniz Institute for Natural Product Research and Infection Biology, Hans Knoell Institute, Jena - Germany) for kindly donating the reagent methylisocitrate.
References
Alberghina, L., Mavelli, G., Drovandi, G., Palumbo, P., Pessina, S., Tripodi, F., et al. (2012). Cell growth and cell cycle in Saccharomyces cerevisiae: basic regulatory design and protein-protein interaction network. Biotechnol. Adv. 30, 52–72. doi: 10.1016/j.biotechadv.2011.07.010
Bhat, A., Wu, Z., Maher, V. M., Mccormick, J. J., and Xiao, W. (2015). Rev7/Mad2B plays a critical role in the assembly of a functional mitotic spindle. Cell Cycle 14, 3929–3938. doi: 10.1080/15384101.2015.1120922
Brock, M., Darley, D., Textor, S., and Buckel, W. (2001). 2-Methylisocitrate lyases from the bacterium Escherichia coli and the filamentous fungus Aspergillus nidulans- Characterization and comparison of both enzymes. Eur. J. Biochem. 268, 3577–3586. doi: 10.1046/j.1432-1327.2001.02262.x
Busti, S., Coccetti, P., Alberghina, L., and Vanoni, M. (2010). Glucose signaling-mediated coordination of cell growth and cell cycle in Saccharomyces cerevisiae. Sensors 10, 6195–6240. doi: 10.3390/s100606195
Chen, J., and Fang, G. (2001). MAD2B is an inhibitor of the anaphase-promoting complex. Genes Dev. 15, 1765–1770. doi: 10.1101/gad.898701
Chen, J., Yi, J. L., Liu, L., Yin, S. C., Chen, R. Z., Li, M. R., et al. (2010). Substrate adaptation of Trichophyton rubrum secreted endoproteases. Microb. Pathog. 48, 57–61. doi: 10.1016/j.micpath.2009.12.001
Cove, D. J.. (1966). The induction and repression of nitrate reductase in the fungus Aspergillus nidulans. Biochim. Biophys. Acta 113, 51–56. doi: 10.1016/S0926-6593(66)80120-0
Cruz, A. H. D., Brock, M., Zambuzzi-Carvalho, P. F., Santos-Silva, L. K., Troian, R. F., Goes, A. M., et al. (2011). Phosphorylation is the major mechanism regulating isocitrate lyase activity in Paracoccidioides brasiliensis yeast cells. FEBS J. 278, 2318–2332. doi: 10.1111/j.1742-4658.2011.08150.x
Ebel, F., Schwienbacher, M., Beyer, J., Heesemann, J., Brakhage, A. A., and Brock, M. (2006). Analysis of the regulation, expression, and localisation of the isocitrate lyase from Aspergillus fumigatus, a potential target for antifungal drug development. Fungal Genet. Biol. 43, 476–489. doi: 10.1016/j.fgb.2006.01.015
Espeso, E. A., and Penalva, M. A. (1996). Three binding sites for the Aspergillus nidulans PacC zinc-finger transcription factor are necessary and sufficient for regulation by ambient pH of the isopenicillin N synthase gene promoter. J. Biol. Chem. 271, 28825–28830. doi: 10.1074/jbc.271.46.28825
Ferreira-Nozawa, M. S., Nozawa, S. R., Martinez-Rossi, N. M., and Rossi, A. (2003). The dermatophyte Trichophyton rubrum secretes an EDTA-sensitive alkaline phosphatase on high-phosphate medium. Braz. J. Microbiol. 34, 161–164. doi: 10.1590/S1517-83822003000200014
Ferreira-Nozawa, M. S., Silveira, H. C. S., Ono, C. J., Fachin, A. L., Ross, A., and Martinez-Rossi, N. M. (2006). The pH signaling transcription factor PacC mediates the growth of Trichophyton rubrum on human nail in vitro. Med. Mycol. J. 44, 641–645. doi: 10.1080/13693780600876553
Gnat, S., Lagowski, D., Nowakiewicz, A., and Zieba, P. (2018). Phenotypic characterization of enzymatic activity of clinical dermatophyte isolates from animals with and without skin lesions and humans. J. Appl. Microbiol. 125, 700–709. doi: 10.1111/jam.13921
Gnat, S., Lagowski, D., Nowakiewicz, A., and Zieba, P. (2019). The host range of dermatophytes, it is at all possible? Phenotypic evaluation of the keratinolytic activity of Trichophyton verrucosum clinical isolates. Mycoses 62, 274–283. doi: 10.1111/myc.12876
Grumbt, M., Monod, M., and Staib, P. (2011). Genetic advances in dermatophytes. FEMS Microbiol. Lett. 320, 79–86. doi: 10.1111/j.1574-6968.2011.02276.x
Gutteridge, A., Pir, P., Castrillo, J. I., Charles, P. D., Lilley, K. S., and Oliver, S. G. (2010). Nutrient control of eukaryote cell growth: a systems biology study in yeast. BMC Biol. 8, 68. doi: 10.1186/1741-7007-8-68
Jacob, T. R., Peres, N. T. A., Persinoti, G. F., Silva, L. G., Mazucato, M., Rossi, A., et al. (2012). rpb2 is a reliable reference gene for quantitative gene expression analysis in the dermatophyte Trichophyton rubrum. Med. Mycol. J. 50, 368–377. doi: 10.3109/13693786.2011.616230
Keys, D. A., and Mcalister-Henn, L. (1990). Subunit structure, expression, and function of NAD(H)-specific isocitrate dehydrogenase in Saccharomyces cerevisiae. J. Bacteriol. 172, 4280–4287. doi: 10.1128/jb.172.8.4280-4287.1990
Kornberg, H. L.. (1966). The role and control of the glyoxylate cycle in Escherichia coli. Biochem. J. 99, 1–11. doi: 10.1042/bj0990001
Leng, W., Liu, T., Wang, J., Li, R., and Jin, Q. (2009). Expression dynamics of secreted protease genes in Trichophyton rubrum induced by key host's proteinaceous components. Med. Mycol. 47, 759–765. doi: 10.3109/13693780802524522
Li, B., Chen, Y., and Tian, S. (2021). Function of pH-dependent transcription factor PacC in regulating development, pathogenicity, and mycotoxin biosynthesis of phytopathogenic fungi. FEBS J. doi: 10.1111/febs.15808
Lin, A. P., and Mcalister-Henn, L. (2002). Isocitrate binding at two functionally distinct sites in yeast NAD+-specific isocitrate dehydrogenase. J. Biol. Chem. 277, 22475–22483. doi: 10.1074/jbc.M202534200
Livak, K. J., and Schmittgen, T. D. (2001). Analysis of relative gene expression data using real-time quantitative PCR and the 2−ΔΔCT method. Methods 25, 402–408. doi: 10.1006/meth.2001.1262
Lopez-Boado, Y. S., Herrero, P., Fernandez, T., Fernandez, R., and Moreno, F. (1988). Glucose-stimulated phosphorylation of yeast isocitrate lyase in vivo. J. Gen. Microbiol. 134, 2499–2505. doi: 10.1099/00221287-134-9-2499
Luttik, M. A. H., Kotter, P., Salomons, F. A., Van Der Klei, I. J., Van Dijken, J. P., and Pronk, J. T. (2000). The Saccharomyces cerevisiaeICL2 gene encodes a mitochondrial 2-methylisocitrate lyase involved in propionyl-coenzyme A metabolism. J. Bacteriol. 182, 7007–7013. doi: 10.1128/JB.182.24.7007-7013.2000
Maranhão, F. C. A., Paiao, F. G., and Martinez-Rossi, N. M. (2007). Isolation of transcripts over-expressed in human pathogen Trichophyton rubrum during growth in keratin. Microb. Pathog. 43, 166–172. doi: 10.1016/j.micpath.2007.05.006
Maranhão, F. C. A., Silveira, H. C. S., Rossi, A., and Martinez-Rossi, N. M. (2011). Isolation of transcripts overexpressed in the human pathogen Trichophyton rubrum grown in lipid as carbon source. Can. J. Microbiol. 57, 333–338. doi: 10.1139/w11-011
Martinez, D. A., Oliver, B. G., Graser, Y., Goldberg, J. M., Li, W. J., Martinez-Rossi, N. M., et al. (2012). Comparative genome analysis of Trichophyton rubrum and related dermatophytes reveals candidate genes involved in infection. mBio 3:12. doi: 10.1128/mBio.00259-12
Martinez-Rossi, N. M., Peres, N. T. A., Bitencourt, T. A., Martins, M. P., and Rossi, A. (2021). State-of-the-Art dermatophyte infections: epidemiology aspects, pathophysiology, and resistance mechanisms. J. Fungi 7:80629. doi: 10.3390/jof7080629
Martinez-Rossi, N. M., Peres, N. T. A., and Rossi, A. (2017). Pathogenesis of dermatophytosis: sensing the host tissue. Mycopathologia 182, 215–227. doi: 10.1007/s11046-016-0057-9
Martinez-Rossi, N. M., Persinoti, G. F., Peres, N. T., and Rossi, A. (2012). Role of pH in the pathogenesis of dermatophytoses. Mycoses 55, 381–387. doi: 10.1111/j.1439-0507.2011.02162.x
Martins, M. P., Martinez-Rossi, N. M., Sanches, P. R., Gomes, E. V., Bertolini, M. C., Pedersoli, W. R., et al. (2019a). The pH signaling transcription factor PAC-3 regulates metabolic and developmental processes in pathogenic fungi. Front. Microbiol. 10, 2076. doi: 10.3389/fmicb.2019.02076
Martins, M. P., Martinez-Rossi, N. M., Sanches, P. R., and Rossi, A. (2019b). The PAC-3 transcription factor critically regulates phenotype-associated genes in Neurospora crassa. Genet. Mol. Biol. 43, e20190374. doi: 10.1590/1678-4685-gmb-2019-0374
Martins, M. P., Rossi, A., Sanches, P. R., Bortolossi, J. C., and Martinez-Rossi, N. M. (2020). Comprehensive analysis of the dermatophyte Trichophyton rubrum transcriptional profile reveals dynamic metabolic modulation. Biochem. J. 477, 873–885. doi: 10.1042/BCJ20190868
Mehul, B., De Coi, N., Grundt, P., Genette, A., Voegel, J. J., and Monod, M. (2019). Detection of Trichophyton rubrum and Trichophyton interdigitale in onychomycosis using monoclonal antibodies against Sub6 (Tri r 2). Mycoses 62, 32–40. doi: 10.1111/myc.12843
Mendes, N. S., Trevisan, G. L., Cruz, A. H. S., Santos, R. S., Peres, N. T. A., Martinez-Rossi, N. M., et al. (2012). Transcription of N- and O-linked mannosyltransferase genes is modulated by the pacC gene in the human dermatophyte Trichophyton rubrum. FEBS Open Bio 2, 294–297. doi: 10.1016/j.fob.2012.09.005
Peres, N. T., Silva, L. G., Santos Rda, S., Jacob, T. R., Persinoti, G. F., Rocha, L. B., et al. (2016). In vitro and ex vivo infection models help assess the molecular aspects of the interaction of Trichophyton rubrum with the host milieu. Med. Mycol. J. 54, 420–427. doi: 10.1093/mmy/myv113
Peres, N. T. A., Sanches, P. R., Falcao, J. P., Silveira, H. C. S., Paiao, F. G., Maranhao, F. C. A., et al. (2010). Transcriptional profiling reveals the expression of novel genes in response to various stimuli in the human dermatophyte Trichophyton rubrum. BMC Microbiol. 10, 39. doi: 10.1186/1471-2180-10-39
Persinoti, G. F., Martinez, D. A., Li, W. J., Dogen, A., Billmyre, R. B., Averette, A., et al. (2018). Whole-genome analysis illustrates global clonal population structure of the ubiquitous dermatophyte pathogen Trichophyton rubrum. Genetics 208, 1657–1669. doi: 10.1534/genetics.117.300573
Robertson, E. F., Hoyt, J. C., and Reeves, H. C. (1988). Evidence of histidine phosphorylation in isocitrate lyase from Escherichia coli. J. Biol. Chem. 263, 2477–2482. doi: 10.1016/S0021-9258(18)69231-2
Rossi, A., Cruz, A. H. S., Santos, R. S., Silva, P. M., Silva, E. M., Mendes, N. S., et al. (2013). Ambient pH sensing in filamentous fungi: pitfalls in elucidating regulatory hierarchical signaling networks. IUBMB Life 65, 930–935. doi: 10.1002/iub.1217
Silveira, H. C. S., Gras, D. E., Cazzaniga, R. A., Sanches, P. R., Rossi, A., and Martinez-Rossi, N. M. (2010). Transcriptional profiling reveals genes in the human pathogen Trichophyton rubrum that are expressed in response to pH signaling. Microb. Pathog. 48, 91–96. doi: 10.1016/j.micpath.2009.10.006
Tilburn, J., Sarkar, S., Widdick, D. A., Espeso, E. A., Orejas, M., Mungroo, J., et al. (1995). The Aspergillus Pacc Zinc-Finger transcription factor mediates regulation of both acid-expressed and alkaline-expressed genes by ambient pH. EMBO J. 14, 779–790. doi: 10.1002/j.1460-2075.1995.tb07056.x
Tran, V. D. T., De Coi, N., Feuermann, M., Schmid-Siegert, E., Bagut, E. T., Mignon, B., et al. (2016). RNA sequencing-based genome reannotation of the dermatophyte Arthroderma benhamiae and characterization of its secretome and whole gene expression profile during infection. mSystems 1, 16. doi: 10.1128/mSystems.00036-16
Vylkova, S., Carman, A. J., Danhof, H. A., Collette, J. R., Zhou, H., and Lorenz, M. C. (2011). The fungal pathogen Candida albicans autoinduces hyphal morphogenesis by raising extracellular pH. mBio 2, e00055–e00011. doi: 10.1128/mBio.00055-11
Yanagida, M., Ikai, N., Shimanuki, M., and Sajiki, K. (2011). Nutrient limitations alter cell division control and chromosome segregation through growth-related kinases and phosphatases. Philos. Trans. R. Soc. Lond. B Biol. Sci. 366, 3508–3520. doi: 10.1098/rstb.2011.0124
Keywords: Trichophyton, tricarboxylic acid cycle, glyoxylate cycle, methylcitrate cycle, cellular cycle, subtilisin
Citation: Cruz AHS, Santos RS, Martins MP, Peres NTA, Trevisan GL, Mendes NS, Martinez-Rossi NM and Rossi A (2022) Relevance of Nutrient-Sensing in the Pathogenesis of Trichophyton rubrum and Trichophyton interdigitale. Front. Fungal Biol. 3:858968. doi: 10.3389/ffunb.2022.858968
Received: 20 January 2022; Accepted: 03 March 2022;
Published: 31 March 2022.
Edited by:
Tamás Papp, University of Szeged, HungaryReviewed by:
Everardo López-Romero, University of Guanajuato, MexicoGustavo Alexis Niño-Vega, University of Guanajuato, Mexico
Sebastian Gnat, University of Life Sciences of Lublin, Poland
Copyright © 2022 Cruz, Santos, Martins, Peres, Trevisan, Mendes, Martinez-Rossi and Rossi. This is an open-access article distributed under the terms of the Creative Commons Attribution License (CC BY). The use, distribution or reproduction in other forums is permitted, provided the original author(s) and the copyright owner(s) are credited and that the original publication in this journal is cited, in accordance with accepted academic practice. No use, distribution or reproduction is permitted which does not comply with these terms.
*Correspondence: Nilce M. Martinez-Rossi, bm1tcm9zc2lAdXNwLmJy