- International Centre of Insect Physiology and Ecology (icipe), Nairobi, Kenya
Entomopathogenic fungi can cause substantial mortality in harmful insects. Before killing the insect, these pathogens start by negatively affecting the biological parameters of the host. Prior to our study, the information about how fungal exposure affects the biological parameters of the stable fly, Stomoxys calcitrans was still elusive. Therefore, we aimed to assess the infection of S. calcitrans with some Metarhizium anisopliae strains, and their impact on feeding, fecundity, fertility and other life-history traits of this fly. Among the 11 M. anisopliae strains screened, we identified ICIPE 30 as the most virulent strain against S. calcitrans. We observed that the infectivity of this strain was sex and age-dependent. Infected male S. calcitrans died earlier than their counterpart females. Older infected S. calcitrans died faster than infected young ones. Also, male and female S. calcitrans successfully transmitted ICIPE 30 conidia to their mates. We demonstrated that infection by ICIPE 30 extended the feeding time of S. calcitrans and consequently reduced the feeding probability of the fly and the amount of blood taken. Using a dual test oviposition bioassay, we determined that uninfected gravid female S. calcitrans avoided laying eggs on substrates amended with ICIPE 30 conidia. We showed that these conidia could lower the hatchability of the eggs deposited by gravid females. Using, a no-choice test, we showed that gravid female S. calcitrans infected with ICIPE 30 laid fewer eggs than uninfected females and those eggs hatched less. Using 11 strains of M. anisopliae and four high concentrations of ICIPE 30 conidia, we verified that S. calcitrans larvae were not susceptible to fungal infection. Further, we showed that though these larvae were tolerant to fungal infection, there was a significant effect on their fitness, with contaminated larvae having a small bodyweight coupled with longer developmental time as compared to uncontaminated larvae. Our study provides detailed information on how fungal infection affects the biology of S. calcitrans and the potential of using M. anisopliae ICIPE 30 as a biopesticide to reduce the fly population. Such knowledge can assist in developing fungal-based control strategies against this harmful fly.
Introduction
Metarhizium anisopliae (Hypocreales: Clavicipitaceae) is a ubiquitous entomopathogenic fungus infecting a wide range of insect hosts and used for biological control (Brunner-Mendoza et al., 2019). Its mode of action involves attachment to the host's cuticle, germination, epicuticle penetration and dissemination inside the insect body as hyphae (Ortiz-Urquiza and Keyhani, 2013). This infection mechanism is facilitated by a group of enzymes including hydrolases, proteases, chitinases and lipases (Brunner-Mendoza et al., 2019). When proliferating inside insect tissues, blastospores produce toxic molecules (e.g., destruxins) that induce pathogenesis, paralysis, cellular alterations and dysfunction of the middle intestine, malpighian tubules, and muscle tissues (Samuels et al., 1988). These cascades of events ultimately provoke insect death 3–7 days after infection (Mondal et al., 2016). It has been demonstrated that the speed with which an insect succumbs from fungal infection could depend on the fungal strain (Valero-Jiménez et al., 2014), insect species, insect sex, and age (Maniania and Odulaja, 1998). However, before the death occurs, several pre-lethal reactions including the reduction in development, feeding propensity, and reproduction can be observed in infected insects.
Several laboratory and field trials have demonstrated the lethal and pre-lethal effects of M. anisopliae infection in insects. In blood-feeding insects, this fungus is known to cause high mortality in immature and adult stages. For instance, M. anisopliae has proven to reduce survival of different larval stages of Aedes aegypti, Anopheles stephensi, and Culex quinquefasciatus (Diptera: Culicidae) (Greenfield et al., 2015; Ravindran et al., 2016). In Phlebotomus papatasi (Diptera: Psychodidae), M. anisopliae reduces adult emergence when applied to larval food (Zayed et al., 2013; Alkhaibari et al., 2017). Adults of the tsetse fly, Glossina morsitans (Diptera: Glossinidae) that emerge from M. anisopliae-infected pupae suffer from high mortality (Kaaya and Munyinyi, 1995). Maniania (2002) found that in the field M. anisopliae can reduce the population of Glossina spp. by 82.4%. Laboratory-based bioassay revealed high mortality in Anopheles gambiae and C. quinquefasciatus owing to M. anisopliae infection (Scholte et al., 2003). In Meccus pallidipennis (Hemiptera: Reduviidae), a vector of Trypanosoma cruzi, Flores-Villegas et al. (2016) observed that individuals treated with M. anisopliae died sooner than untreated individuals. In addition to this lethal effect, infection by M. anisopliae is also known to induce pre-lethal effects on hematophagous insects. This has been shown in An. gambiae (Scholte et al., 2003) and Ae. aegyptis (Paula et al., 2011); where a reduction of feeding and reproduction was reported after exposure to M. anisopliae. Here, we studied the infection of M. anisopliae in the stable fly, Stomoxys calcitrans (Diptera: Muscidae) and its consequences on the feeding, fecundity, fertility, and life-history traits of this fly.
S. calcitrans is a cosmopolitan blood-feeding dipteran involved in the mechanical transmission of viruses (e.g., West Nile fever virus, Rift Valley fever virus), bacteria (e.g., Bacillus anthracis, Pasteurella multocida), protozoa (e.g., Trypanosoma evansi, Besnoitia besnoit), and helminths (e.g., Habronema microstoma, Dirofilaria repens) in various hosts including cattle, camels, horses, dogs, and humans (Baldacchino et al., 2013). During its high infestation periods, S. calcitrans can induce a reduction of 40–60% in milk yield and 19% in cattle weight gain (Walker, 1990; Carn, 1996) as a result of the nuisance caused to livestock. In cattle industries, losses attributed to S. calcitrans are estimated to be around $2.2 billion annually (Taylor et al., 2012). As all holometabolous insects, the development of S. calcitrans goes through an egg, three larval instars (with the size and morphology varying across the three instars (see Friesen et al., 2015), pupae and adult stages. The development of S. calcitrans occurs in herbivorous dung (Baleba et al., 2019) and rotting organic matter such as silage, hay, grass clippings, and garden compost (Cook et al., 2018).
The use of the entomopathogenic fungus M. anisopliae as a control agent against S. calcitrans has already been investigated in various studies; with results showing the high susceptibility of adults, but not larvae (Moraes et al., 2008). The M. anisopliae strain Ma135 was reported to kill more than 90% of S. calcitrans adults (López-Sánchez et al., 2012). When aspersed on dairy cattle, Cruz-Vazquez et al. (2015) established that the M. anisopliae strain Ma134 reduce populations of S. calcitrans by 73%. To our knowledge, there are no studies in the literature addressing the pre-lethal effect of M. anisopliae infection in S. calcitrans or the influence of the age or sex of the fly. Moreover, there is no evidence of the horizontal transmission of M. anisopliae conidia in S. calcitrans. Thus, our main aim was to study the lethal and pre-lethal effect of M. anisopliae infection in S. calcitrans. Specifically, we tested whether: (1) infection of S. calcitrans by M. anisopliae conidia would be sex- and age-dependent; (2) M. anisopliae conidia would be horizontally transmitted in S. calcitrans; (3) M. anisopliae infection would negatively impact the feeding propensity, fecundity and fertility of S. calcitrans; (4) there would be a trade-off between the tolerance of S. calcitrans larvae to M. anisopliae infection and their larval life-history traits.
Materials and Methods
M. anisopliae Strain Culture
The eleven strains of M. anisopliae used in our experiment were obtained from the icipe's Arthropod Germplasm Center (Mweke et al., 2018; Akutse et al., 2020). The strains were cultured on Sabouraud Dextrose Agar (SDA) medium using 90-mm Petri dishes and maintained in the darkness at 25°C. Two weeks after the start of the culture, we harvested conidia of each strain by scraping the surface of the sporulated cultures using a sterile spatula. We suspended conidia of the different strains in 10 ml of distilled water with 0.05% Triton X-100 in universal bottles containing 3–5 glass beads (3 mm in diameter per bottle) each. The mixture was then vortexed for 5 min at 700 rpm to homogenize the suspension. Using an improved Neubauer haemocytometer under the light microscope, we determined the conidia concentration of each strain suspension following the protocol described by Lacey (2012). Before each bioassay, we tested the ability of conidia to germinate by spreading 100 μl of each strain suspension (titrated at 3 × 106 conidia ml−1) on SDA plate. We sealed the inoculated plates with Parafilm membrane and incubated them in complete darkness at 25°C. At 18 h post-incubation, we flooded the plates with lactophenol aniline cotton blue to stop the germination process and stain the spore to ease their visibility for counting. Following this, we determined the number of conidia that germinated by counting 100 randomly selected conidia beneath each coverslip under a light microscope (400×). Conidium was considered as germinated if the length of its germ-tube was at least twice its diameter (Lacey, 2012). For each strain, we used five plates as replicates.
S. calcitrans Colony
Individuals of S. calcitrans used in all our experiment were obtained from the “International Center of Insect Physiology and Ecology (icipe)” Animal Rearing and Quarantine Unit (ARCU) in Nairobi, Kenya (1° 13′ 12″ S, 36° 52′ 48″ E; ≈ 1,600 m above sea level) colony. This colony was established and maintained as described in Baleba et al. (2019). Briefly, wild individuals of S. calcitrans were captured at icipe campus using Vavoua traps (Laveissière and Grebaut, 1990), maintained inside a cage (75 cm × 60 cm × 45 cm) and fed twice per day (800 and 1,600 h) on defibrinated bovine blood poured on moistened cotton to initiate reproduction. Once gravid females were obtained, we exposed them to rabbit dung (fermented in a plastic bag for 1 week) placed in plastic containers (21.5 cm × 14.5 cm × 7.4 cm) for oviposition. After 24 h, we transferred the exposed containers to another cage (75 cm × 60 cm × 45 cm), and we monitored the development of the larval and pupal stages until adult emergence. We fed emerged adults with bovine blood and repeated the previously described above. We reared all the insects and performed our experiments in a laboratory under buffered conditions of 25 ± 5°C 65 ± 5% relative humidity, and 12L:12D photoperiod.
Effect of M. anisopliae Infection on the Survival of S. calcitrans Adults
We determined the pathogenicity and virulence of 11 strains of M. anisopliae on S. calcitrans adults following the contamination protocol used by Wamiti et al. (2018) on Glossina fuscipes fuscipes (Diptera: Glossinidae). In this protocol, the contamination device (Supplementary Figure 1A) is comprised of a cylindrical plastic tube (95 mm × 48 mm) which has an inner part covered by a velvet carpet material impregnated with fungal dried conidia. Adult flies were gently introduced into the contamination device and allowed to pick conidia. After this period of exposure, flies were gently removed, and transferred in another cylindrical plastic tube free of conidia. In all our experiment, we used 0.1 g of conidia evenly spread on the velvet carpet, and exposed flies to conidia for 10 min. After transferring the fungus-exposed flies in a clean cylindrical plastic tube, we provided them with blood and recorded the number of dead flies daily for 7 days. We removed cadavers found inside the plastic tube using sterilized forceps and incubated them in Petri dishes containing moistened filter paper to assess the outgrowth of the applied fungal conidia (Supplementary Figure 1B). We used fungus-free flies as control. For each treatment, we used 10 flies and replicated the experiment five times.
Effect of Sex and Age on M. anisopliae ICIPE 30 Infectivity in S. calcitrans
We used newly emerged (24 h old) males and females [differentiated based on the size of the two compound eyes that are smaller and more widely separated in females (dioptic) than in males (holoptic)] to see whether the pathogenicity of M. anisopliae varied between the sex of S. calcitrans. In the earlier experiment, we identified ICIPE 30 as the most virulent M. anisopliae strain against S. calcitrans (see results section). Here, we used this strain (0.1 g of dried conidia) to contaminate 10 males and 10 females following the protocol previously described above (Wamiti et al., 2018). As a control, we used unexposed males and females. For the age effect bioassay, we used only 10 exposed female flies (to account for any bias resulting from sex effect) of 1, 7, and 14 days old. The control groups consisted of fungus-free flies of 1, 7, and 14 days old. In both bioassays, we provided each group with bovine blood and recorded individual mortalities daily for 7 days. To confirm whether the death of the flies was caused by M. anispoliae ICIPE 30 infection, we placed dead flies in Petri dishes (9 cm) containing moistened filter paper to initiate fungal sporulation on the cadaver surfaces. We replicated each experiment five times.
Horizontal Transmission of M. anisopliae ICIPE 30 Conidia by S. calcitrans
Before testing whether M. anisopliae ICIPE 30-exposed males and females could transmit conidia to their conspecific mates, we aimed to determine whether the number of conidia carried by S. calcitrans individuals could vary between sex and across time. To do so, we chilled 5 males and 5 females (2 days old) in ice for 2–3 min to induce a coma. Using fine sterilized forceps, we gently placed the immobilized flies inside the cylindrical plastic tube (on top of velvet carpet containing 0.1 g of ICIPE 30). After recovered from the coma, we allowed the flies to walk on conidia for 30 min, then individually introduced them inside universal bottles containing 2–5 glass beads and 1 ml of sterile distilled water with 0.05% Triton X-100. The bottles with the exposed flies were thereafter vortexed for 5 min (to remove conidia from the insect's body) and estimated the number of conidia carried by each individual using the Neubauer haemocytometer. To assess how the number of conidia carried by each individual be across time, we transferred the exposed male and female flies in a cleaned cage (15 cm × 15 cm × 20 cm), waited for 2, 4, 6, and 8 h before proceeding with the conidia quantification as previously described.
With a slight modification, we followed the protocol described by Maniania et al. (2013) to perform the horizontal transmission assay. We contaminated 5 males (donors) with 0.1 g of M. anisopliae ICIPE 30 conidia for 10 min then transferred them into another clean cage (15 cm × 15 cm × 20 cm). Four hours after this process, we transferred these males inside a clean cylindrical plastic tube and paired them with 5 fungus-free females (receivers). We use the same protocol to pair fungus-exposed females (donors) with fungus-free males (receivers). We considered fungus-free males and females as control. In all the treatments, we provided our flies with blood and recorded their mortality daily for 7 days. To confirm whether the dead in both sexes was induced by M. anisopliae infection, we placed separately the dead bodies of male flies in Petri dishes (9 cm) containing moistened filter paper to later assess fungal growth on the cadaver surfaces. We used five replicates in all the bioassays.
Impact of M. anisopliae ICIPE 30 Infection on the Feeding Propensity of S. calcitrans
Here, using the previous contamination device, we exposed female S. calcitrans (2 days old) with the M. anisopliae strain ICIPE 30. To determine the effect of this fungal infection on the feeding propensity of S. calcitrans, we recorded three parameters, namely (1) the feeding duration, (2) the proportion of blood-fed, and (3) the amount of blood consumed. We determined the feeding duration by recording the time taken by an individual fly to get engorged after inserting its proboscis into the blood source (Supplementary Figure 2B). The proportion of blood-fed corresponded to the number of flies (in a group of 10 individuals) that managed to take blood after 60 s of their exposure to the blood exposition. The amount of blood consumed per fly was estimated as the difference in their weight, after (Supplementary Figure 2C) and before (Supplementary Figure 2A) the blood meal. As a control, we used fungus-free individuals. We collected all the data 2, 3, and 4 days after fungal infection. The feeding duration and the amount of blood consumed data were obtained from 30 fungus-exposed and fungus-free female flies; while the proportion of blood-fed data were from 5 groups of 10 individuals each.
Influence of M. anisopliae ICIPE 30 on Gravid Female S. calcitrans Reproduction Traits
To elucidate whether M. anisopliae ICIPE 30 could affect the reproduction of S. calcitrans, we used (1) egg-laying decision, (2) fecundity and (3) fertility as proxies. To test the effect of M. anisopliae ICIPE 30 on S. calcitrans egg-laying decision, we conducted two oviposition choice bioassays (Supplementary Figure 3A). In the first bioassay, we exposed 10 gravid female S. calcitrans to two Petri dishes (Diameter: 5.5 cm) containing each, only 50 g of rabbit dung to see whether they will lay the same number of eggs on both Petri dishes. For the second bioassay, we presented rabbit dung supplemented with 0.1 g of M. anisopliae conidia (strain ICIPE 30) and rabbit dung only (control) to 10 gravid female S. calcitrans to see whether these females will select either substrate preferentially. In both bioassays, we used 10 replicates, and for each replicate, we counted the number of eggs laid on each substrate after 24 h and determined their ability to hatch 5 days after egg deposition (by counting the number of larvae found on each substrate).
To assess the effect of M. anisopliae ICIPE 30 on the fecundity (number of eggs laid) and fertility (number of eggs hatched) of S. calcitrans, we performed two no-choice oviposition bioassays (Supplementary Figures 3Bi,ii). To do so, following the previously described protocol, we exposed 30 females (4 days old) to M. anisopliae strain ICIPE 30 and transferred them individually inside cages (15 cm × 15 cm × 20 cm) containing 2 males to allow mating. We supplied these flies with blood daily and once females become gravid, we provided them with a Petri dish containing rabbit dung for oviposition. As a control, we used fungus-free gravid female S. calcitrans. We recorded the number of eggs laid on each substrate daily until the female succumbs from fungal infection. To assess the fertility of eggs laid by infected and uninfected gravid female S. calcitrans, we determined their hatchability by counting the number of larvae found on each substrate 5 days after the egg deposition.
Trade-Offs Between M. anisopliae ICIPE 30 Infection Tolerance and Life-History Traits in S. calcitrans Larvae
It has been reported previously that S. calcitrans larvae are not susceptible to M. anisopliae infection (Moraes et al., 2008). To test this, we infected second larval instar of this fly with the same 11 strains of M. anisopliae as described above. For each strain, we sprayed 10 larvae (placed on a Petri dish) with 10 ml of suspension at the concentration of 2 × 108 conidia ml−1 using a Burgerjon's spray tower (Burgerjon, 1956). After spraying, we transferred the contaminated larvae in transparent plastic cups of 200 ml prior containing 50 g of rabbit dung. As a control group, we used larvae treated with sterile distilled water containing 0.05% Triton X-100. We recorded the number of dead larvae daily until pupation. We carried out all the treatments in five times. We observed that most infected S. calcitrans larvae (90 %) managed to reach the pupal stage. Therefore, in a subsequent bioassay, we aimed to challenge these larvae with higher concentrations of M. anisopliae. As previously described, we contaminated 10 S. calcitrans larvae with four increasing conidia concentrations (3 × 108, 4 × 108, 5 × 108, and 6 × 108 conidia ml−1) of the strain ICIPE 30 and recorded the number of dead larvae daily until pupation.
To test whether the tolerance to M. anisopliae ICIPE 30 infection in S. calcitrans larvae could impact their fitness parameters, we contaminated 10 individuals of each S. calcitrans larval instar (L1, L2, and L3) with 10 ml of ICIPE 30 concentrated at 2 × 108 conidia ml−1. It is indicated that life stages that undergo metamorphosis (occasioning the change in size and morphology) should be treated independently when studying their responses to biotic stresses (McCormick and Gagliano, 2009; Kingsolver et al., 2011; Ezeakacha and Yee, 2019). We followed the contaminated larvae daily until the adult stage by recording the following life-history fitness parameters: (1) pupation time, (2) larval weight, (3) pupation rate, (4) pupal weight, (5) emergence percentage, (6) emergence time, and (7) adult weight. Larval, pupal, and adult weight data were collected as described in Baleba et al. (2020). For the weight parameter, we weighed all the larvae individually, as well as pupae and adults that emerged from contaminated larvae. We recorded larval weight 2, 4, and 6 days after fungal contamination in the individuals from L1 and L2 instars. While in individuals from the L3 instar (close to the pupal stage), we recorded weight only 2 days after contamination. As a control, we used L1, L2, and L3 individuals sprayed with sterile distilled water containing 0.05% Triton X-100. We replicated this experiment five times.
Data Analysis
We conducted all the statistical analysis in the R environment for statistical computing (version 3.6.3) (R Core Team., 2020) and grouped all the graphs in Adobe Illustrator CC 2017(version 21.0). Before conduct the analysis, we subjected mortality data to Abbot's correction (Abbot, 1925).
For the bioassay aiming to study the effect of the 11 strains of M. anisopliae on the S. calcitrans survival, we performed Kaplan–Meier survival analysis with the Mantel–Cox log-rank chi-squared test using the R package “survival” (Therneau, 2015) to see how the survival of S. calcitrans adults varied as a result to exposure to the different fungus strains. Owing to the normal distribution (Shapiro–Wilk test: P > 0.05) and the homoscedasticity (Bartlett's test: P > 0.05) of the median lethal time data, we ran the analysis of variance (ANOVA) followed by the Student–Neuman–Keuls (SNK) post-hoc multiple comparison tests to see how this parameter varied across the 11 strains. For the same reason, we performed the ANOVA followed by the SNK post-hoc tests to compare the proportion of alive S. calcitrans (at 7th day of our bioassay) across the 11 strains.
In the experiment testing the effect of sex and age on M. anisopliae infectivity, we used the Kaplan–Meier survival analysis with the Mantel–Cox log-rank chi-squared test to elucidate how these factors affected the infectivity of M. anisopliae. We employed the unpaired t-test to compare the median lethal time between the sexes of S. calcitrans. To determine whether this parameter could vary across the three ages of S. calcitrans (1, 7, and 14 days), we performed the ANOVA followed by the SNK post-hoc tests. Using the same analysis, we compared the number of alive S. calcitrans (at 7th day of our bioassay) across the sex and the ages.
For the experiment aiming to test whether, in S. calcitrans, M. anisopliae conidia could be transferred from one sex to another, we used the Kaplan–Meier survival analysis with the Mantel–Cox log-rank chi-squared test to see whether the survival of the fungus-donor, fungus-receiver, and fungus-free (control) S. calcitrans could significantly vary. We ran the unpaired t-test to compare the median lethal time between fungus-donor and fungus-receiver flies. We performed the ANOVA followed by the SNK post-hoc tests to compare the number of fungus-donor, fungus-receiver, and fungus-free S. calcitrans that were still alive at the end of our experiment (7th day).
In the feeding propensity test, we used the unpaired Wilcoxon test to compare the feeding time of infected and uninfected flies. Owing to the binary nature of the feeding proportion data (engorged vs. not engorged) we performed a generalized linear model (GLM) with binomial distribution followed by the analysis of deviance (with the chi-squared test) to see how the proportion of blood-fed flies varied between infected and uninfected flies. We executed the unpaired t-test to compare the amount of blood taken by infected and uninfected flies.
For the experiment testing the effect of M. anisopliae on S. calcitrans reproduction, we used the paired t-test to compare the number of eggs laid by gravid females S. calcitrans on the two Petri dishes containing only rabbit dung. We used the same statistical analysis to compare the number of eggs laid by these females on Petri dishes with and without M. anisopliae ICIPE 30 dried conidia. The Egg hatchability data were binary (hatched vs. unhatched); therefore, we used a GLM with binomial distribution and analysis of deviance (with chi-squared test) to see how this parameter varied between substrates with and without conidia. To compare the number of eggs laid by infected and uninfected gravid females S. calcitrans, we used an unpaired t-test. We compared the hatchability the eggs produced by these females, using a GLM with binomial distribution and analysis of deviance (with chi-squared test).
For the data from the bioassay testing the effect of M. anisopliae on the survival of S. calcitrans larvae, we performed the ANOVA test to compare the proportion of larvae that pupated across the different M. anisopliae strains and the ICIPE 30 concentrations. In the experiment testing the effect of M. anisopliae infection on the life-history parameters of three different larval instars of S. calcitrans, we subjected data from pupation time, larval weight, pupal weight, emergence time, and adult weight to the normality and homogeneity tests. In case the data of a particular parameter were normally distributed (Shapiro–Wilk test: P > 0.05) and their variances were homogeneous (Bartlett's test: P > 0.05), we used the unpaired t-test to see how this parameter varied between infected and uninfected larvae. When these two assumptions were not fulfilled, we used the unpaired Wilcoxon test. We analyzed the pupation and emergence percentage data using a GLM with binomial distribution and analysis of deviance (with chi-squared test).
Statistical significance was noted at P < 0.05 and its strength was represented with asterisks (*P < 0.05; **P < 0.01; ***P < 0.001, and ****P < 0.001).
Results
Effect of M. anisopliae Infection on the Survival of S. calcitrans Adults
All the 11 strains of M. anisopliae used in our study possessed a germination percentage above 90% (Figure 1A). As time progressed, the proportion of S. calcitrans surviving from M. anisopliae infection reduced with a significant difference across the strains (Figure 1B; log-rank test, χ2 = 50.3, df = 11, P < 0.0001). The median lethal time [Figure 1C; One-way ANOVA: F(10−44) = 7.79, P < 0.0001] and the proportion of alive S. calcitrans after the 7 days post-infection [Figure 1D; One-way ANOVA: F(11−47) = 17.5, P < 0.0001] significantly differed across the 11 strains of M. anisopliae. Of all the S. calcitrans individuals infected with 11 strains of M. anisopliae, only those infected with the strain ICIPE 30 had simultaneously, lower median lethal time (Figure 1C) and lower proportion of alive individuals (20%) at the end of our experiment (Figure 1D). Although at the end of our experiment, the proportion of alive S. calcitrans infected with the strain ICIPE 7 was similar to that of S. calcitrans infected with the strain ICIPE 30 (Figure 1D), the strain ICIPE 7 took the longest time (>5 days) to kill half individuals of S. calcitrans as compared to the strain ICIPE 30 (<4 days) (Figure 1C). Therefore, we considered the strain ICIPE 30 as the most potent and virulent for S. calcitrans.
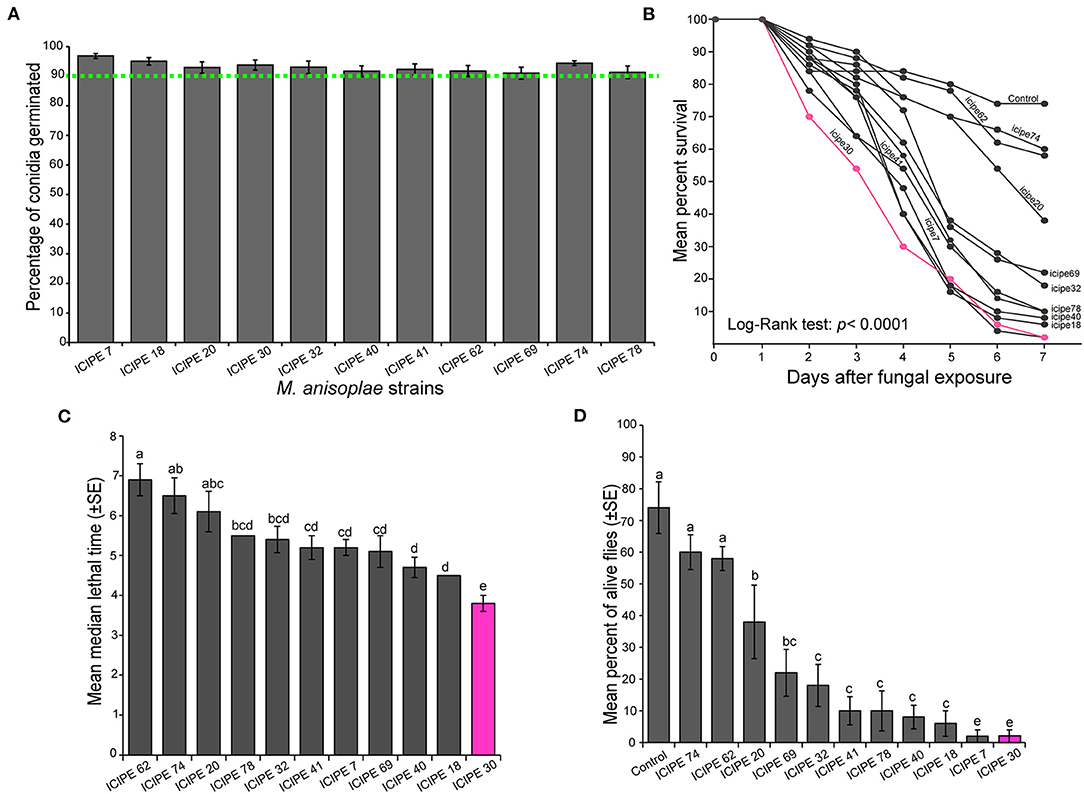
Figure 1. Virulence of different Metarhizium anisopliae strains toward Stomoxys calcitrans. (A) Bar chart showing the mean percentage germination of the 11 M. anisopliae strains used in all our experiment. (B) Kaplan–Meier curve showing survivorship over time in the adult of S. calcitrans across the different M. anisopliae strains (Mantel–Cox log-rank χ2 test, P < 0.05, n = 5). (C) Bar chart illustrating the mean median lethal time (LT50) in S. calcitrans adults infected by the different M. anisopliae strains. (D) Bar chart depicting the mean percentage of S. calcitrans adults alive 7 days after fungal infection, across the different M. anisopliae strains (ANOVA followed by SNK post-hoc test; p < 0.05, n = 5). Error bars indicate the standard error of the mean. Bars with different lowercase letters are significantly different from each other.
Effect of S. calcitrans Sex and Age on M. anisopliae ICIPE 30 Infectivity
In both sex of S. calcitrans, the survival over time of uninfected (males and females) and infected (males and females) individuals significantly varied (Figure 2Ai; log-rank test, χ2 = 11.8, df = 3, P = 0.008). But the pairwise comparison using the log-rank test revealed that the survival of infected males and infected females over time did not significantly differ (P = 0.26). This was also true for uninfected males and uninfected females (P = 0.39). The mean median lethal time of infected female S. calcitrans was significantly higher than that of infected males (Figure 2Aii; U = 22, P = 0.04). At the end of our experiment, infected flies of both sexes had a significantly lower proportion of alive individuals as compared to that uninfected flies [Figure 2Aiii; One-way ANOVA: F(3−16) = 27.27, P < 0.0001]. But this proportion was similar between infected males and females; and uninfected males and females.
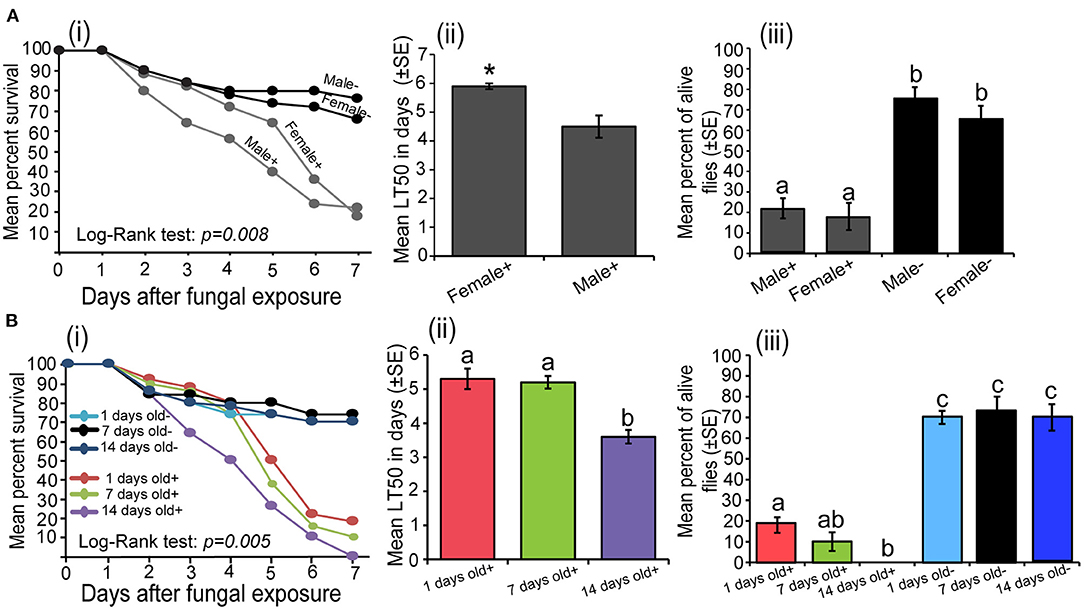
Figure 2. (A) Influence of the Stomoxys calcitrans sex on Metarhizium anisopliae ICIPE 30 infectivity: (i) Kaplan–Meier curve showing survivorship over time of infected (males and females) and uninfected (males and females) S. calcitrans (Mantel–Cox log-rank χ2 test, P < 0.05, n = 5), (ii) Bar chart illustrating the mean median lethal time (LT50) between infected males and females of S. calcitrans (unpaired t-test, *p < 0.05, n = 5), (iii) Bar chart depicting the mean percentages of individual alive across the sex of infected and uninfected S. calcitrans the 7th day of our bioassay (ANOVA followed by SNK post-hoc test; p < 0.05, n = 5). (B) Influence of the S. calcitrans age on M. anisopliae ICIPE 30 infectivity: (i) Kaplan–Meier curve showing survivorship over time of infected (1, 7, and 14 days old) and uninfected (1, 7, and 14 days old) S. calcitrans (Mantel–Cox log-rank χ2 test, P < 0.05, n = 5), (ii) Bar chart illustrating the mean median lethal time (LT50) across the age of infected S. calcitrans (ANOVA followed by SNK post-hoc test; p < 0.05, n = 5), (iii) Bar chart showing the mean percentages of individual alive across the age of infected and uninfected S. calcitrans at the 7th day of our bioassay (ANOVA followed by SNK post-hoc test; p < 0.05, n = 5). The signs + and – denote infected and uninfected individuals, respectively. Error bars indicate the standard error of the mean. Bars with different lowercase letters are significantly different from each other.
The survival of S. calcitrans over time significantly changed across the different age of flies (log-rank test, χ2 = 16.9, df = 5, P = 0.005); with 14-days old infected possessing the lower survival rate (Figure 2Bi). As compared to 1 and 7 days old, 14-days old infected flies had a smaller median lethal time [Figure 2Bii; One-way ANOVA: F(2−12) = 16.65, P < 0.001]. Regardless of the age, the proportion of alive flies obtained 7 days after contamination was significantly lower in infected flies and as compared to uninfected flies [Figure 2Biii; One-way ANOVA: F(5−24) = 59.39, P < 0.0001]. In infected flies, this proportion was significantly lower in 14 days old flies followed by 7 and 1-day old flies (Figure 2Biii).
Horizontal Transmission of M. anisopliae ICIPE 30 Conidia by S. calcitrans
The amount of M. anisopliae ICIPE 30 conidia carried by S. calcitrans significantly varied across time (P < 0.0001) with no variations between sex (P = 0.051). This amount was higher directly after the contamination process; but 2 h later, it drastically dropped with no significant change even 8 h after the fly's contamination (Figure 3A). We found that 4 h after exposure to M. anisopliae ICIPE 30 conidia, fungus-exposed flies (donors) were still able to contaminate fungus-free flies (receivers). When we contaminated S. calcitrans males (donors) and associated them with fungus-free females (receivers), the survival of these flies over time significantly reduced as compared to those of males and females maintained uncontaminated throughout the bioassay (Figure 3Bi; log-rank test, χ2 = 26.2, df = 3, P < 0.0001). The median lethal time of male donors was significantly lower as compared to that of female receivers (Figure 3Bii; t = 3.6, df = 6, P = 0.013). Both male donors and female receivers had reduced proportion of alive individuals at the 7th-day post-contamination as compared to males and females maintained uncontaminated [Figure 3Biii; One-way ANOVA: F(3−14) = 16.29, P < 0.0001]. We obtained the same result pattern when we contaminated females (donors) and associated them with fungus-free males (receivers). The survival of female donors and male receivers significantly reduced over time as compared to the survival of uncontaminated males and females (Figure 3Ci; log-rank test, χ2 = 16.2, df = 3, P < 0.001). Female donors had a lower mean median lethal time as compared to that of male receivers (Figure 3Cii, U = 2, P = 0.026). The proportion of female donors and male receivers alive at the end of our experiment was significantly lower than those of uncontaminated males and females [Figure 3Ciii; One-way ANOVA: F(3−17) = 10.2, P < 0.001].
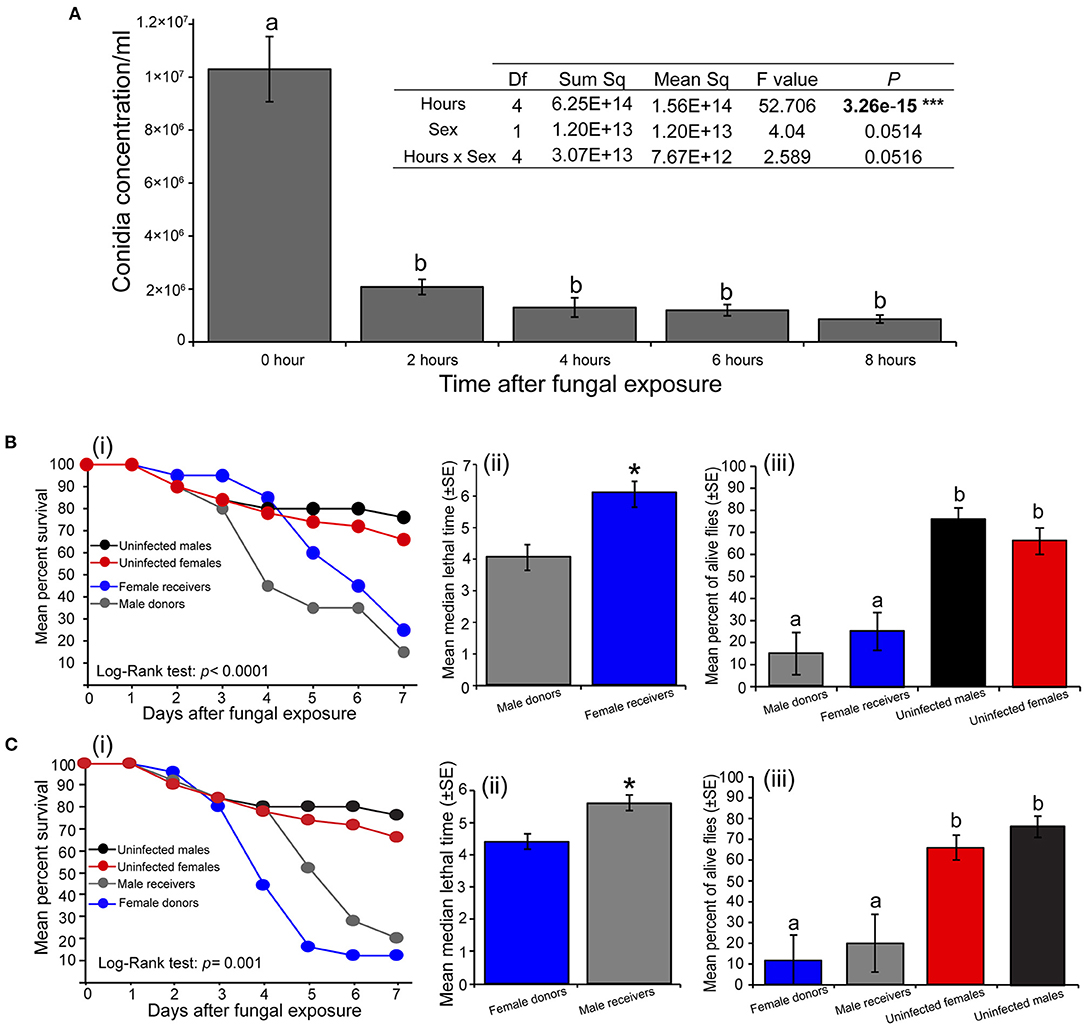
Figure 3. Horizontal transmission of Metarhizium anisopliae conidia in Stomoxys calcitrans. (A) Bar chart showing the variation across the time of conidia load in S. calcitrans (one-way ANOVA followed by SNK post-hoc test; P < 0.05, ***P < 0.001, n = 10). (B) Transmission of M. anisopliae ICIPE 30 conidia from S. calcitrans males (donors) to females (receivers): (i) Kaplan-Meier curve showing survivorship over time of S. calcitrans male donors and female receivers (Mantel-Cox log-rank χ2 test, P < 0.05, n = 5); (ii) Bar chart illustrating the mean median lethal time (LT50) of male donors and female receivers (unpaired t-test, *P < 0.05, n = 5); (iii) Bar chart illustrating the mean percentage of S. calcitrans male donors and female receivers alive the 7th day of our bioassay (one-way ANOVA followed by SNK post-hoc test; P < 0.05, n = 5). (C) Transmission of M. anisopliae ICIPE 30 conidia from S. calcitrans females (donors) to males (receivers): (i) Kaplan–Meier curve showing survivorship over time of S. calcitrans female donors and male receivers (Mantel–Cox log-rank χ2 test, P < 0.05, n = 5); (ii) Bar chart illustrating the mean median lethal time (LT50) of female donors and male receivers (unpaired t-test, *P < 0.05, n = 5); (iii) Bar chart depicting the mean percentage of S. calcitrans female donors and male receivers alive the 7th day of our bioassay (ANOVA followed by SNK post-hoc test; P < 0.05, n = 5). Error bars indicate the standard error of the mean. Letters above error bars indicate a significant difference across the treatment.
Effect of M. anisopliae ICIPE 30 Infection on the Feeding Propensity of S. calcitrans
Infection of S. calcitrans by M. anisopliae ICIPE 30 significantly altered its feeding propensity. Fourty-eight (48) hours after fungal exposure, the time taken by S. calcitrans to get engorged did not vary between infected and uninfected flies (t = 0.06, df = 67.7, P = 0.95). But, 72 (t = 2.18, df = 65.5, P = 0.032) and 96 (U = 466, P < 0.001) h after the conidia exposure occurred, infected flies took significantly more time to get engorged as compared to uninfected flies (Figure 4A). Sixty (60) seconds after blood exposure, 48-h infected flies and uninfected flies had the same proportion flies that managed to take blood (Figure 4Bii; GLM, χ2 = 19.92, df = 1, P = 0.50). Nonetheless, this proportion significantly reduced in 72-h (GLM, χ2 = 9.5, df = 1, P = 0.042) and 96-h (GLM, χ2 = 25.53, df = 1, P = 0.004) infected flies (Figure 4B). The amount of blood taken by infected flies at 48 (t = 4.60, df = 61.5, P < 0.0001), 72 (t = 3.71, df = 66, P < 0.001), and 96 (t = 2.9, df = 39.4, P < 0.01) h after fungal exposure was significantly lower as compared to that of uninfected flies (Figure 4C).
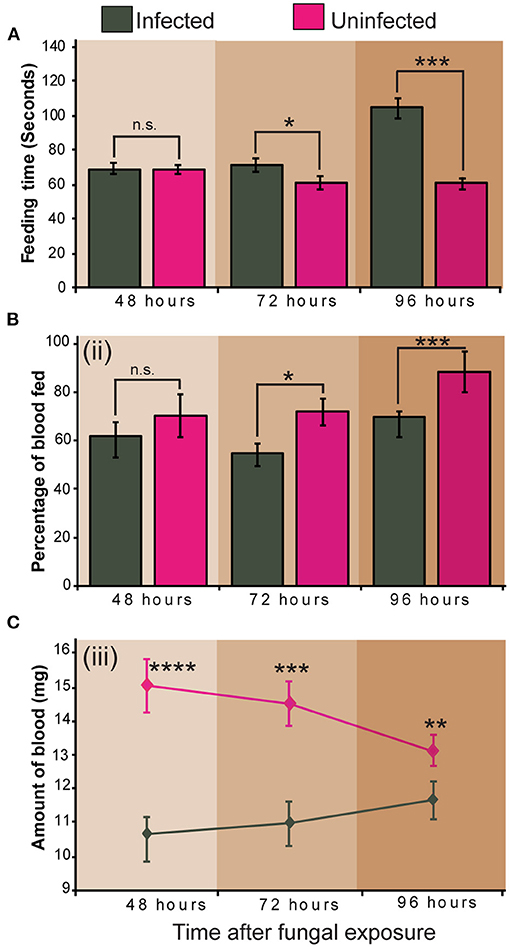
Figure 4. Impact of Metarhizium anisopliae infection on Stomoxys calcitrans feeding propensity. (A) Bar charts showing the mean feeding duration across time in infected and uninfected S. calcitrans; (B) Bar charts illustrating the mean percentage of infected and uninfected S. calcitrans individuals that managed to get engorged in 60 s across time; (C) Line graph showing the change of the amount of blood taken by infected and uninfected S. calcitrans across time. Error bars indicate the standard error of the mean. Asterisks indicate that mean between infected and uninfected individuals differed significantly (unpaired t-test: *P < 0.05; **P < 0.01, ***P < 0.001, ****P < 0.0001, n = 30). “n.s” indicates non-significant difference (P > 0.05).
Influence of M. anisopliae ICIPE 30 on S. calcitrans Female's Reproduction Traits
In our dual-test oviposition bioassay, when we presented two fungus-free substrates to S. calcitrans gravid females, they laid the same number of eggs on both substrates (Figure 5Ai, t = 0.83, df = 17.9, P = 0.41). However, when we added dried conidia of M. anisopliae on one of the substrates, these females laid significantly few numbers of eggs on the fungal-embedded substrate (Figure 5Aii; U = 0, P < 0.01). Consequently, the proportion of eggs deposited on the substrate with dried conidia that hatched was significantly less than that of eggs laid on the substrate without dried conidia (Figure 5Aiii, GLM; χ2 = 23.07, df = 1, P < 0.0001). In the no-choice bioassay, S. calcitrans gravid females infected with M. anisopliae ICIPE 30 laid a significantly fewer number of eggs than uninfected S. calcitrans gravid females (Figure 5Bi; t = 5.08, df = 11.84, P < 0.001). Also, the hatchability of eggs laid by infected females was significantly lower than that of eggs deposited by uninfected females (Figure 5Bii, GLM; χ2 = 26.63, df = 1, P < 0.0001).
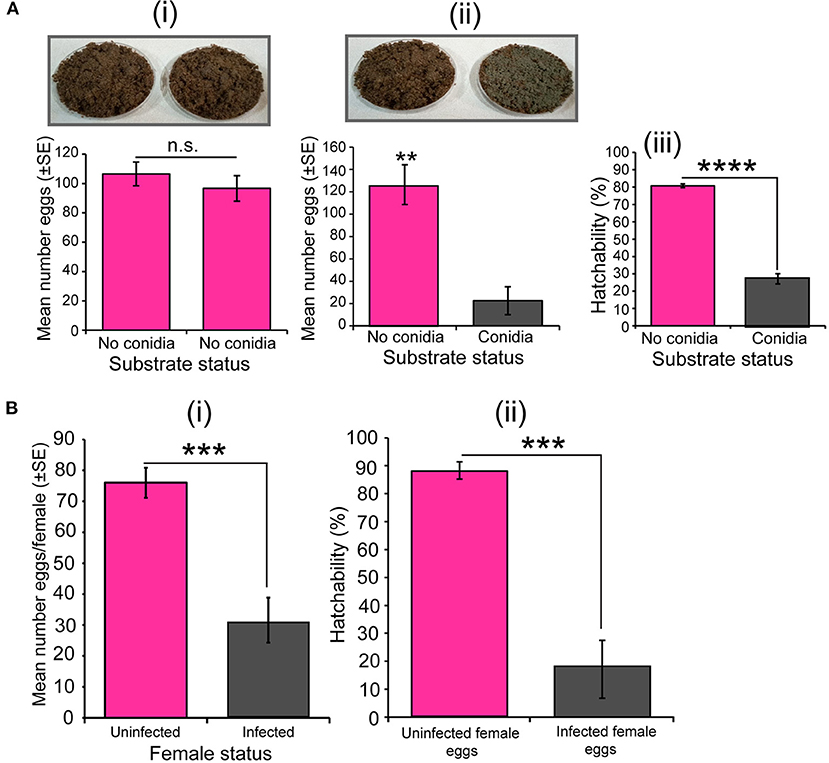
Figure 5. Effect of Metarhizium anisopliae on the oviposition decision, fecundity, and egg hatchability of Stomoxys calcitrans. (A) Egg-laying decision bioassay with uninfected gravid female S. calcitrans: (i) Bar chart showing the mean number of eggs laid by S. calcitrans on substrates without dried conidia of M. anisopliae ICIPE 30 (paired t-test, n = 10); (ii) Bar chart showing the mean number of eggs laid by S. calcitrans on substrates with and without dried conidia of M. anisopliae ICIPE 30 (paired t-test: p > 0.05, n = 10); (iii) Bar chart illustrating the proportion of eggs hatched from eggs laid of substrates with and without dried conidia of M. anisopliae ICIPE 30 (GLM with binomial distribution followed by the analysis of deviance test, n = 10). (B) Egg-laying assay with single uninfected and infected gravid female S. calcitrans. (i) Bar chart illustrating the mean number of eggs laid by uninfected and infected gravid females of S. calcitrans (unpaired t-test, n = 30); (ii) Bar chart showing the mean proportion of eggs that hatched from eggs laid by uninfected and infected of S. calcitrans (GLM with binomial distribution followed by the analysis of deviance test). Errors bar on each bar chart indicates the standard error of the mean. **P < 0.01, ***P < 0.001, and ****P < 0.0001.
Fitness Cost Associated With the Tolerance of M. anisopliae Infection in S. calcitrans Larvae
As demonstrated in other studies, our study showed that S. calcitrans larvae are not susceptible to infection with M. anisopliae strains used. All the M. anisopliae strains-contaminated larvae and uncontaminated larvae (control) had similar pupation percentage [F(11−48) = 0.42, P = 0.94]. For each treatment, about 90% of larvae reached the pupal stage (Figure 6Ai). Even at high conidia concentrations, the pupation percentage of contaminated larvae did not reduce [Figure 6Aii; F(4−20) = 0.62, P = 0.65]. Even though the high proportion of contaminated larvae did not succumb from M. anisopliae exposure (since some managed to pupate), we demonstrated a negative impact of this contamination on some of their fitness parameters.
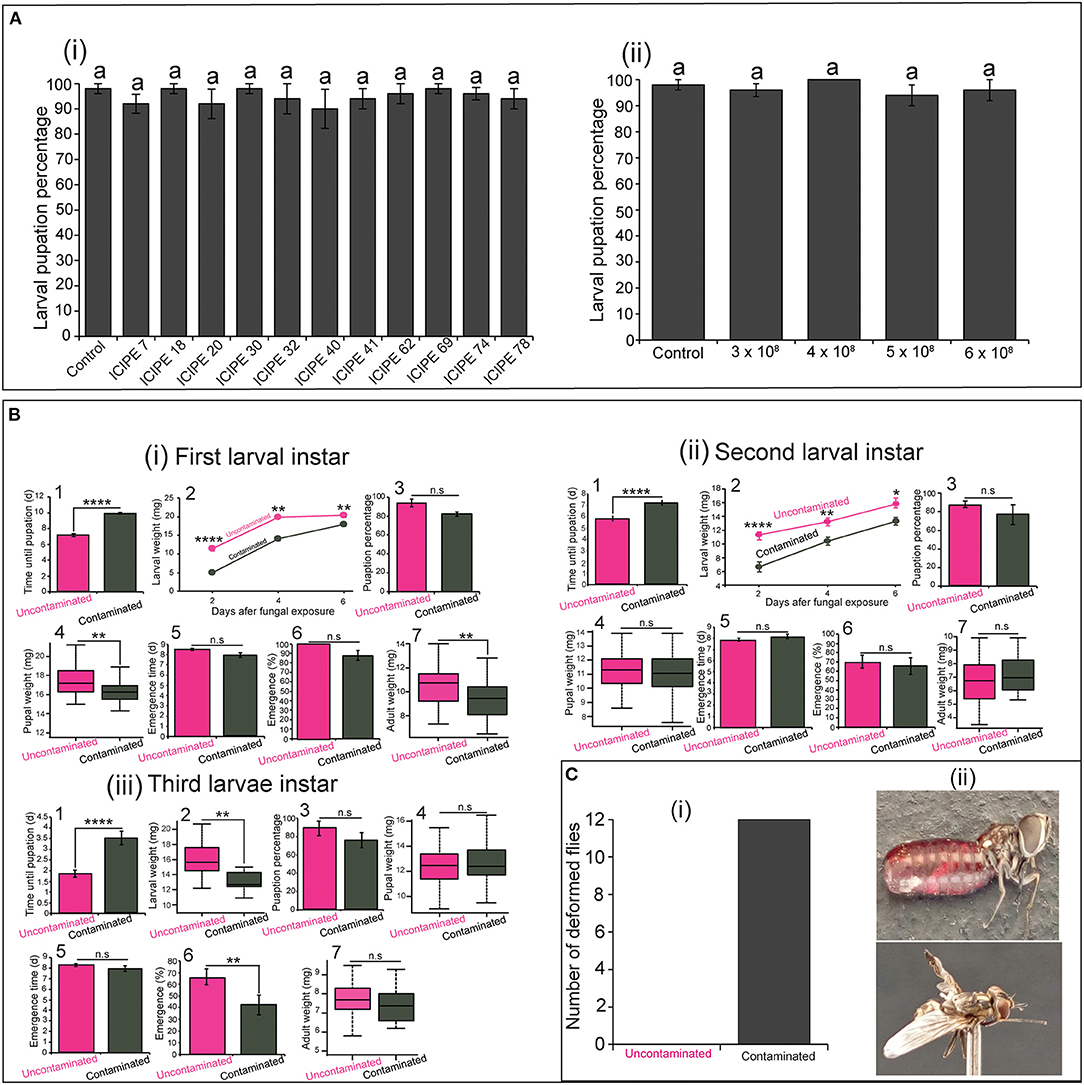
Figure 6. Influence of Metarhizium anisopliae infection on the fitness parameters of the three larval instars of Stomoxys calcitrans. (A) Bar chart showing the mean pupation percentage of S. calcitrans larvae contaminated by the 11 strains of M. anisopliae (i) and the increasing concentrations of the M. anisopliae strain ICIPE 30 (ii) (ANOVA test; n = 5). (B) Fitness parameters of uncontaminated and contaminated first (i), second (ii), and third (iii) larval instar of S. calcitrans: (1) Bar charts depicting the mean pupation time in each uncontaminated and contaminated larval instar of S. calcitrans (unpaired Mann–Whitney test); (2) Line graphs showing the variation of weight in each uncontaminated and contaminated larval instar of S. calcitrans after 2, 4, and 6 days of infection (unpaired t-test test or unpaired Mann–Whitney test); (3) Bar charts illustrating the mean pupation percentage of each uncontaminated and contaminated larval instar of S. calcitrans (GLM with binomial distribution followed by the analysis of deviance test, n = 5); (4) Box plots showing the pupal weight obtained from each uncontaminated and contaminated larval instar of S. calcitrans (unpaired t-test); (5) Bart charts illustrating the mean emergence time of S. calcitrans adults developed from each uncontaminated and contaminated larval instars (unpaired Mann–Whitney test); (6) Bar charts depicting the emergence percentage of S. calcitrans adults developed from each uncontaminated and contaminated larval instars (GLM with binomial distribution followed by the analysis of deviance test, n = 5); (7) Box plots showing the weight of S. calcitrans adults developed from each uncontaminated and contaminated larval instars (unpaired t-test). (C) Histogram (i) showing the total number of deformed S. calcitrans adults (ii; Original Photo: Steve B. S. Baleba) emerged from contaminated larvae. On each bar chart and line graph, error bars indicate the standard error of the mean. Each box plots shows the median (bold horizontal lines) and whiskers the interquartile range. n.s (non-significant difference): P > 0.05; significant difference: *P < 0.05, **P < 0.01, and ****P < 0.0001.
We observed that the pupation time was significantly longer in contaminated first (Figure 6Bi1: U = 70, P < 0.0001), second (Figure 6Bii1: U = 255.5, P < 0.0001), and third (Figure 6Biii1, U = 346, P < 0.0001) larval instars of S. calcitrans as compared to that of their corresponding control (uncontaminated larvae). Also, the weight of contaminated first (Figure 6Bi2: Day 2: t = 8.45, df = 14.4, P < 0.0001; Day 4: U = 317, P = 0.002; Day 6: t = 3.2, df = 17.98, P = 0.005), second (Figure 6Bii2: Day 2: t = 5.5, df = 14.6, P < 0.0001, Day 4: t = 3.5, df = 18, P = 0.002; Day 6: t = 2.4, df = 15.9, P = 0.026), and third (Figure 6Biii2; t = 2.97, df = 15.4, P = 0.009) larval instar of S. calcitrans was smaller than those of uncontaminated instars. The pupation percentage did not significantly change between contaminated and uncontaminated individuals from the first (Figure 6Bi3; GLM; χ2 = 3.55, df = 1, P = 0.06), second (Figure 6Bii3; GLM; χ2 = 3.25, df = 1, P = 0.071), and third (Figure 6Biii3; GLM; χ2 = 2.48, df = 1, P = 0.11) larval instars. The pupal weight was significantly reduced in contaminated individuals developed from the first larval instars (Figure 6Bi4: t = 3.5, df = 80, P < 0.001). However, pupae formed from the contaminated second (Figure 6Bii4: t = −0.73, df = 37.66, P = 0.46), and third (Figure 6Biii4: t = −0.89, df = 65.51, P = 0.37) larval instars had similar weight with those developed from uncontaminated second and third larval instars respectively. Pupae developed from contaminated and uncontaminated first (Figure 6Bi5: U = 1002.5, P = 0.12), second (Figure 6Bii5: U = 229.5, P = 0.16), and third (Figure 6Biii5: U = 262.5, P = 0.32) larval instars formed at the same time. The proportion of adults obtained from contaminated and uncontaminated first (Figure 6Bi6: GLM; χ2 = 1.46, df = 1, P = 0.22) and second (Figure 6Bii6: GLM; χ2 = 0.002, df = 1, P = 0.97) larval instars was not significantly different; but in the third larval instar, this proportion was significantly higher in uncontaminated larvae (Figure 6Biii6: GLM; χ2 = 7.04, df = 1, P = 0.008). Adult obtained from contaminated first larval instars had a significant reduced weight as compared to those emerged from uncontaminated first larval instars (Figure 6Bi7: t = −3.27, df = 68.8, P = 0.002). While those obtained from contaminated and uncontaminated second (Figure 6Bii7: t = −1.06, df = 44.2, P = 0.29) and third (Figure 6Biii7: t = 1.27, df = 29.45, P = 0.21) larval instars had the same weight. Independently to the larval instars, we numbered 12 deformed adults from contaminated larvae and no deformed adults from uncontaminated larvae (Figure 6C).
Discussion
Our results demonstrate that M. anisopliae infection significantly reduces the survival, feeding propensity, fecundity, and fertility of S. calcitrans. Also, these findings show that there is a fitness cost associated with the tolerance of S. calcitrans larvae to M. anisopliae infection.
All the 11 strains of M. anisopliae studied killed S. calcitrans adults; although the speed and the rate at which this occurred varied significantly among the strains. Several studies have demonstrated the implication of morphological and physiological characteristics of fungal strains on this virulence variation. These characteristics include hyphal growth rate, conidial viability, conidia production, conidia size, enzyme secretion among other factors (Liu et al., 2003; Quesada-Moraga and Vey, 2003; Talaei -Hassanloui et al., 2006). In our study, M. anisopliae strain ICIPE 30 rapidly killed (<4 days) half of S. calcitrans individuals and appeared to be the most virulent strain. The virulence of this strain has previously been demonstrated in other haematophagous dipterans including Glossina morsitans morsitans (Maniania and Odulaja, 1998), An. gambiae (Mnyone et al., 2009, 2011) and Ae. aegypti (Jemberie et al., 2018). At this stage, there is a need to study the genetic, molecular and physiological mechanisms mediating the virulence of ICIPE 30. Niassy et al. (2013) started by characterizing the chitinase genes (chi2 and chi4) of this strain responsible for the secretion of enzymes that digest the insect cuticle. The overexpression of such genes in Metarhizium fungus using bioengineering methods could increase their virulence and this need to be investigated further. For instance, the genetically engineered M. anisopliae in which the cat1 gene has been overexpressed tolerate more exogenous hydrogen peroxide; resulting in the acceleration of its germination and the increase of its virulence (Morales Hernandez et al., 2010).
We demonstrated that the M. anisopliae infectivity could be influenced by the sex and the age of S. calcitrans individuals. As compared to female S. calcitrans, M. anisopliae ICIPE 30 killed faster 50 % of male S. calcitrans (Figure 2Aii). This may indicate that there are differences in the innate immunity of male S. calcitrans compared to females. We speculate that female S. calcitrans produce more vigorous cellular and humoral immune reactions against M. anisopliae blastospore. Using Drosophila melanogaster (Diptera: Drosophilidae) and Beauveria bassiana (Hypocreales: Cordycipitaceae), Shahrestani et al. (2018) demonstrated the existence of sexual dimorphism in the immune response of insects to fungal infection. Our results contrast with those of Maniania and Odulaja (1998) who showed that females of G. m. morsitans and G. m. centralis succumb first to ICIPE 30 infection. We attribute this difference to the dissimilarity of the insect species used in our respective studies. Nonetheless, our finding is supported by other studies involving different strains of M. anisopliae and fungal species. Kaaya (1989) showed that males of G. m. morsitans infected with M. anisopliae strains 35-79, 82-82, and 100-82 were more susceptible to the infection than females. In Musca domestica (Diptera: Muscidae), males infected with Entomophthora muscae (Entomophthorales: Entomophthoraceae) died significantly earlier than females (Mullens, 1985). In addition to this effect of sex, we also found a significant effect of the S. calcitrans age on M. anisopliae ICIPE 30 infectivity. Fourteen-days old female S. calcitrans infected with ICIPE 30 died relatively sooner than females of 1 and 7-days old. This increase of mortality with the age in infected S. calcitrans has already been observed in other blood-feeding insects including adult tsetse flies (Maniania and Odulaja, 1998) and An. gambiae (Mnyone et al., 2011). The reduction of the immune response to M. anisopliae infection could explain why older female S. calcitrans died faster. In general, the immune system of animals weakens as they become older. For instance, the melanization which is an immediate immune response to pathogens in arthropods is reduced in old Ae. aegypti individuals (Christensen et al., 1986). Enzymes such as phenoloxidases which play a key role in insect immune system decline with the insect age (González-Santoyo and Córdoba-Aguilar, 2012). In Ae. aegypti, the high mortality observed in old individuals after their infection with Escherichia coli is associated to decrease in the number of hemocytes in their hemolymph (Hillyer et al., 2005). Our results would be explained better by further studies investigating the change that undergoes the immune system of S. calcitrans with the age.
We showed that the number of conidia attached to S. calcitrans cuticle reduced drastically after the exposure (~75% within 2 h) (Figure 3A). This result could be associated with the fact that, after the fungal exposure, S. calcitrans exhibit active grooming behavior, with flies trying to clean as much as possible all their body parts. The conidia found on S. calcitrans 8 h after exposure could be from the areas hard to reach (e.g., back of thorax and abdomen) during the active grooming. This reduction of the conidia load over time has also been found in Ceratitis cosyra (Diptera: Tephritidae) (Dimbi et al., 2013). We demonstrated that in S. calcitrans, 4 h after M. anisopliae contamination, conidia of this fungus can still be transmitted horizontally from one sex to another. The survival of females that received ICIPE 30 conidia from contaminated males was significantly lower than that of uncontaminated females (Figure 3B). We obtained a similar result with males that received conidia from contaminated females (Figure 3C). Horizontal transmission of fungal conidia is also found in other blood-feeding insect species including G. morsitans (Maniania et al., 2013), Triatoma infestans (Hemiptera: Rediviidae) (Forlani et al., 2011), An. gambiae (Scholte et al., 2004) and Ae. aegyptis (Diptera: Culicidae) (García-Munguía et al., 2011). We hypothesize that the horizontal transmission of M. anisopliae conidia in S. calcitrans occurs during courtship events including wing extension and vibration, abdomen drumming and curving, or proboscis extension. Also, the fact that S. calcitrans repeatedly mate in a day could increase the probability of an infected individual to transmit conidia to several uninfected mates. This result is important as it demonstrates that ICIPE 30 could be used in an auto-dissemination control strategy to suppress the fly populations. In the field conditions, the incorporation of this fungal strain into trapping devices that massively catch and release S. calcitrans individuals would assist in spreading the fungus inoculum in the environment where the population density of the fly is high. The auto-dissemination control strategy has shown its effectiveness in other control system involving Aedes albopictus (Diptera: Culicidae) (Unlu et al., 2017) and this need to be validated under field condition in S. calcitrans.
We elucidated that M. anisopliae ICIPE 30 infection significantly impacted the feeding propensity of S. calcitrans. Our results showed that compared to uninfected controls, infected S. calcitrans took more time to consume blood. Also, these individuals were less likely to feed and when they did, they consumed a small amount of blood. For vector control purpose, reduction in feeding propensity is particularly important since pathogens they carry are transmitted during the blood meal. Pre-lethal reduction in feeding due to fungal infection has been shown in An. stephensi (Blanford et al., 2011, 2012), Ae. aegyptis (Darbro et al., 2012), and An. gambiae (Scholte et al., 2006). Studies indicate that this reduction in feeding is related to the fact that, individuals infected with fungi fail to locate potential blood sources owing to the reduction of the olfactory sensitivity occasioned by the infection. For example, George et al. (2011) showed that B. bassiana and Metarhizium acridum fungal spores, as well as inducing sublethal effects in An. stephensi, also reduce the responsiveness of its olfactory neurons. The reduction of feeding in infected S. calcitrans could also be due to the antifeedant activity of the secondary metabolites produced by the M. anisopliae blastopores, and the toxicity activity of these metabolites on the tissues of the insect midgut. For instance, Amiri et al. (1999) demonstrated that the destruxins produced by M. anisopliae have an antifeedant effect against larvae of Plutella xylostella (Lepidoptera: Plutellidae) and Phaedon cochleariae (Coleoptera: Chrysomelidae). Also, Skrobek and Butt (2005) showed that these molecules exhibit a cytotoxicity activity in Spodoptera frugiperda (Lepidoptera: Noctuidae) cells.
Our results also revealed that gravid females S. calcitrans differentiated substrates treated with M. anisopliae ICIPE 30 from substrates without this fungus. These females laid fewer eggs on treated substrates compared to the untreated ones (Figure 5Aii). Using Metarhizium brunneum, Machtinger et al. (2016) also found this fungus-induced oviposition avoidance in S. calcitrans. We showed that eggs laid on substrates with fungus had lower hatchability (Figure 5Aiii). This could explain why these substrates were avoided by gravid females S. calcitrans. In our previous studies, we showed that gravid females S. calcitrans were able to avoid substrates that could harm their progeny (Baleba et al., 2019, 2020). As the egg-laying decision of S. calcitrans is guided by olfactory cues (Baleba et al., 2019), we suggest that the avoidance of substrates treated with M. anisopliae by gravid females S. calcitrans could be mediated by chemical volatiles produced by M. anisopliae. Studies conducted on the termites Macrotermes michaelseni (Isoptera: Termitidae) (Mburu et al., 2011) and Coptotermes formosanus Shiraki (Isoptera: Rhinotermitidae) (Hussain et al., 2010) have already demonstrated the repellency of volatiles emitted by M. anisopliae conidia. Our results open new research avenues in identifying repellent odourant molecules from M. anisopliae that may be used to control S. calcitrans and reduce the spread of diseases that they transmit.
We found a reduction of eggs production in gravid females S. calcitrans infected with M. anisopliae. Also, we showed that the hatchability of eggs produced by these females was significantly low. These results suggest that once infected by M. anisopliae, S. calcitrans females are less likely to produce viable progeny for the next generation; contributing therefore to their population reduction. In An. gambiae, females infected with M. anisopliae laid fewer eggs (Scholte et al., 2006). Using B. bassiana, García-Munguía et al. (2011) also obtained a reduction of fecundity in infected females Ae. aegytis. The introduction of the entomopathogenic fungus, Aspergillus parasiticus (Eurotiales: Trichocomaceae) into natural populations of An. gambiae, Culex fatigans, and Ae. aegypti significantly reduced the fecundity and fertility of females that become infected (Nnakumusana, 1985). In our study, we hypothesize that the observed reduction of fecundity and fertility could be the immediate effect of the reduction of blood intake observed in M. anisopliae infected S. calcitrans. It has been shown that ovarian development, egg maturation and fertility heavily depend on the amount of blood taken by the insect (Gonzales and Hansen, 2016). Moreover, the reduction of fecundity and fertility in infected S. calcitrans could be associated with the effect of M. anisopliae on the maturation of S. calcitrans eggs. For example, Sánchez-Roblero et al. (2012) demonstrated that B. bassiana delay the maturation of Anastrepha ludens (Diptera: Tephritidae) eggs, resulting to the reduction the quantity of their mature basal oocytes and ultimately the number of eggs laid.
Also, we demonstrated that there is a fitness cost associated with the tolerance of S. calcitrans larvae to M. anisopliae infection. As Moraes et al. (2008), we found that S. calcitrans larvae were not susceptible to M. anisopliae infection. About 90% of the larvae contaminated with the 11 strains of M. anisopliae (Figure 6Ai) and those contaminated with high concentrations of ICIPE 30 (Figure 6Aii) reached the pupal stage. Moraes et al. (2014) demonstrated the existence of antifungal activity of S. calcitrans larvae against entomopathogenic fungal infection. The authors found that macerated solution of S. calcitrans larvae reduces the growth of B. bassiana; suggesting that these larvae produce robust anti-fungal substances protecting them from infection. When subjected to high-performance liquid chromatography (HPLC) analysis, new peaks in the chromatogram that could represent the antifungal molecules were obtained from the solution of macerated B. bassiana infected larvae as compared to the control group (Moraes et al., 2014). Earlier, a peptide affecting microorganism growth (stomoxyn) was identified in the exterior midgut of S. calcitrans (Boulanger et al., 2002). We recommend further studies to characterize molecules secreted by fungal infected S. calcitrans larvae and their protective role against pathogens.
Furthermore, we found that the tolerance of S. calcitrans larvae to M. anisopliae infection compromised some of their life-history traits. For instance, even though the majority of contaminated individuals from each larval instar pupated, their pupation period was longer than that of uncontaminated individuals. Also, these contaminated larvae were smaller, and in some contaminated larval instars such as the first and the third instars, adults had small weight and emergence percentage. Moreover, we obtained deformed adults from contaminated larvae even though their number was reduced. Vogelweith et al. (2017) explained that to optimize their response to pathogen invasion, individuals generally balance between investing in their immune system and other life-history traits. Thus, we argue that during a fungal infection in S. calcitrans larvae, certain fitness traits (e.g. developmental speed and weight gain) are suppressed in favor of immune reactions that will enhance the response against the infection.
In summary, our study examined the previously unexplored lethal and pre-lethal effect of M. anisopliae infection in S. calcitrans. We identified a potent and virulent M. anisopliae strain for S. calcitrans (ICIPE 30) that could be developed as biopesticide to manage the fly. We showed that the infectivity of this strain against S. calcitrans could be sex and age-dependent. Also, we proved that males and females of S. calcitrans contaminated with ICIPE 30 can horizontally transmit conidia of this fungal strain to their conspecific mates. Our results demonstrated that M. anisopliae ICIPE 30 infection reduces the feeding propensity, fecundity and fertility of S. calcitrans adults. We showed that the tolerance of S. calcitrans larvae to M. anisopliae infection has a fitness cost in these larvae. Taken together, our work provides detailed insights into the consequence of fungal infection of S. calcitrans, demonstrating the potentiality of the use of entomopathogenic fungi in controlling this important vector of various pathogens of human and veterinary significance. We recommend further chemical, molecular, and physiological studies that would additionally explain or elucidate our results.
Data Availability Statement
The raw data supporting the conclusions of this article will be made available by the authors, without undue reservation.
Author Contributions
SB: study conceptualization, experimental design, data collection, analysis and interpretation, and first manuscript draft preparation. AA: assistance in media preparation, fungus culture, germination test, and manuscript proofreading. MG and KA: advice in experimental design and manuscript proofreading. SS: manuscript proofreading. DM: study conceptualization, fund acquisition, advise in experimental design, supervision, and manuscript proofreading. All authors contributed to the article and approved the submitted version.
Funding
This work received financial support from the German Federal Ministry for Economic Cooperation and Development (BMZ) commissioned and administered through the Deutsche Gesellschaft für Internationale Zusammenarbeit (GIZ) Fund for International Agricultural Research (FIA), grant number: 81235250. We also gratefully acknowledge the financial support for this research by the following organizations and agencies: UK's Foreign, Commonwealth and Development Office (FCDO); the Swedish International Development Cooperation Agency (Sida); the Swiss Agency for Development and Cooperation (SDC); the Federal Democratic Republic of Ethiopia; and the Government of the Republic of Kenya. The views expressed herein do not necessarily reflect the official opinion of the donors.
Conflict of Interest
The authors declare that the research was conducted in the absence of any commercial or financial relationships that could be construed as a potential conflict of interest.
Acknowledgments
The authors are grateful for the technical assistance from Jane Wanjiru Kimemia, Levi Odhiambo Ombura, and Joseck Esikuri Otiwi.
Supplementary Material
The Supplementary Material for this article can be found online at: https://www.frontiersin.org/articles/10.3389/ffunb.2021.637817/full#supplementary-material
Supplementary Figure 1. (A) Cylindrical plastic tube used as the contamination device. (B) Uninfected (i) and infected (ii) adults of S. calcitrans (Original Photo: Steve B.S. Baleba).
Supplementary Figure 2. Individual of S. calcitrans before (A), during (B) and after the blood meal (C) (Original Photo: Steve B. S. Baleba).
Supplementary Figure 3. (A) Experimental setup where 10 gravid females were allowed to choose substrates with and without dried conidia of M. anisopliae ICIPE 30; (B) No choice oviposition bioassay setup using single uninfected (i) and infected (ii) female S. calcitrans.
References
Abbot, W. A. (1925). Method of computing the effectiveness of an insecticide. J. Econ. Entomol. 18, 265–267. doi: 10.1093/jee/18.2.265a
Akutse, K. S., Subramanian, S., Maniania, N. K., Dubois, T., and Ekesi, S. (2020). Biopesticide research and product development in Africa for sustainable agriculture and food security - experiences from the International Centre of Insect Physiology and Ecology (icipe). Front. Sustain. Food Syst. 4:563016. doi: 10.3389/fsufs.2020.563016
Alkhaibari, A. M., Carolino, A. T., Bull, J. C., Samuels, R. I., and Butt, T. M. (2017). Differential pathogenicity of Metarhizium blastospores and conidia against larvae of three mosquito species. J. Med. Entomol. 54, 696–704. doi: 10.1093/jme/tjw223
Amiri, B., Ibrahim, L., and Butt, T. M. (1999). Antifeedant properties of destruxins and their potential use with the entomogenous fungus Metarhizium anisopliae for improved control of crucifer pests. Biocontrol Sci. Technol. 9, 487–498. doi: 10.1080/09583159929451
Baldacchino, F., Muenworn, V., Desquesnes, M., Desoli, F., Charoenviriyaphap, T., and Duvallet, G. (2013). Transmission of pathogens by Stomoxys flies (Diptera, Muscidae): a review. Parasite 20:26. doi: 10.1051/parasite/2013026
Baleba, S. B. S., Torto, B., Masiga, D., Getahun, M. N., and Weldon, C. W. (2020). Stable flies, Stomoxys calcitrans L. (Diptera: Muscidae), improve offspring fitness by avoiding oviposition substrates with competitors or Parasites. Front. Ecol. Evol. 8:5. doi: 10.3389/fevo.2020.00005
Baleba, S. B. S., Torto, B., Masiga, D., Weldon, C. W., and Getahun, M. N. (2019). Egg-laying decisions based on olfactory cues enhance offspring fitness in Stomoxys calcitrans L. (Diptera: Muscidae). Sci. Rep. 9:3850. doi: 10.1038/s41598-019-40479-9
Blanford, S., Jenkins, N. E., Read, A. F., and Thomas, M. B. (2012). Evaluating the lethal and pre-lethal effects of a range of fungi against adult Anopheles stephensi mosquitoes. Malar. J. 11, 365. doi: 10.1186/1475-2875-11-365
Blanford, S., Shi, W., Christian, R., Marden, J. H., Koekemoer, L. L., Brooke, B. D., et al. (2011). Lethal and pre-lethal effects of a fungal biopesticide contribute to substantial and rapid control of malaria vectors. PLoS One 6:e23591. doi: 10.1371/journal.pone.0023591
Boulanger, N., Munks, R. J. L., Hamilton, J. V., Vovelle, F., Brun, R., Lehane, M. J., et al. (2002). Epithelial innate immunity: a novel antimicrobial peptide with antiparasitic activity in the blood-sucking insect Stomoxys calcitrans. J. Biol. Chem. 277, 49921–49926. doi: 10.1074/jbc.M206296200
Brunner-Mendoza, C., Reyes-Montes, M., del, R., Moonjely, S., Bidochka, M. J., and Toriello, C. (2019). A review on the genus Metarhizium as an entomopathogenic microbial biocontrol agent with emphasis on its use and utility in Mexico. Biocontrol Sci. Technol. 29, 83–102. doi: 10.1080/09583157.2018.1531111
Burgerjon, A. (1956). Pulvérisation de poudrage au laboratoire par des préparations pathogènes insecticides. Ann. Epiphyties 4, 677–688.
Carn, V. M. (1996). The role of dipterous insects in the mechanical transmission of animal viruses. Br. Vet. J. 152, 377–393. doi: 10.1016/S0007-1935(96)80033-9
Christensen, B. M., LaFond, M. M., and Christensen, L. A. (1986). Defense reactions of mosquitoes to filarial worms: effect of host age on the immune response to Dirofilaria immitis microfilariae. J. Parasitol. 72:212. doi: 10.2307/3281593
Cook, D. F., Telfer, D. V., Lindsey, J. B., and Deyl, R. A. (2018). Substrates across horticultural and livestock industries that support the development of stable fly, Stomoxys calcitrans (Diptera: Muscidae): substrates that support stable fly. Aust. Entomol. 57, 344–348. doi: 10.1111/aen.12282
Cruz-Vazquez, C., Carvajal Márquez, J., Lezama-Gutiérrez, R., Vitela-Mendoza, I., and Ramos-Parra, M. (2015). Efficacy of the entomopathogenic fungi Metarhizium anisopliae in the control of infestation by stable flies Stomoxys calcitrans (L.), under natural infestation conditions. Vet. Parasitol. 212, 350–355. doi: 10.1016/j.vetpar.2015.07.003
Darbro, J. M., Ritchie, S. A., Thomas, M. B., Johnson, P. H., Ryan, P. A., and Kay, B. H. (2012). Effects of Beauveria bassiana on survival, blood-feeding success, and fecundity of Aedes aegypti in laboratory and semi-field conditions. Am. J. Trop. Med. Hyg. 86, 656–664. doi: 10.4269/ajtmh.2012.11-0455
Dimbi, S., Maniania, N., and Ekesi, S. (2013). Horizontal transmission of Metarhizium anisopliae in fruit flies and effect of fungal infection on egg laying and fertility. Insects 4, 206–216. doi: 10.3390/insects4020206
Ezeakacha, N. F., and Yee, D. A. (2019). The role of temperature in affecting carry-over effects and larval competition in the globally invasive mosquito Aedes albopictus. Parasit. Vectors 12:123. doi: 10.1186/s13071-019-3391-1
Flores-Villegas, A. L., Cabrera-Bravo, M., Toriello, C., Bucio-Torres, M. I., Salazar-Schettino, P. M., and Córdoba-Aguilar, A. (2016). Survival and immune response of the Chagas vector Meccus pallidipennis (Hemiptera: Reduviidae) against two entomopathogenic fungi, Metarhizium anisopliae and Isaria fumosorosea. Parasit. Vectors 9:176. doi: 10.1186/s13071-016-1453-1
Forlani, L., Pedrini, N., and Juarez, M. P. (2011). Contribution of the horizontal transmission of the entomopathogenic fungus Beauveria bassiana to the overall performance of a fungal powder formulation against Triatoma infestans. Res. Rep. Trop. Med. 2, 135–140. doi: 10.2147/RRTM.S22961
Friesen, K., Chen, H., Zhu, J., and Taylor, D. B. (2015). External morphology of stable fly (Diptera: Muscidae) larvae. J. Med. Entomol. 52, 626–637. doi: 10.1093/jme/tjv052
García-Munguía, A. M., Garza-Hernández, J. A., Rebollar-Tellez, E. A., Rodríguez-Pérez, M. A., and Reyes-Villanueva, F. (2011). Transmission of Beauveria bassiana from male to female Aedes aegypti mosquitoes. Parasit. Vectors 4:24. doi: 10.1186/1756-3305-4-24
George, J., Blanford, S., Domingue, M. J., Thomas, M. B., Read, A. F., and Baker, T. C. (2011). Reduction in host-finding behaviour in fungus-infected mosquitoes is correlated with reduction in olfactory receptor neuron responsiveness. Malar. J. 10:219. doi: 10.1186/1475-2875-10-219
Gonzales, K., and Hansen, I. (2016). Artificial diets for mosquitoes. IJERPH 13:1267. doi: 10.3390/ijerph13121267
González-Santoyo, I., and Córdoba-Aguilar, A. (2012). Phenoloxidase: a key component of the insect immune system. Entomol. Exp. Appl. 142, 1–16. doi: 10.1111/j.1570-7458.2011.01187.x
Greenfield, B. P. J., Peace, A., Evans, H., Dudley, E., Ansari, M. A., and Butt, T. M. (2015). Identification of Metarhizium strains highly efficacious against Aedes, Anopheles and Culex larvae. Biocontrol Sci. Technol. 25, 487–502. doi: 10.1080/09583157.2014.989813
Hillyer, J. F., Schmidt, S. L., Fuchs, J. F., Boyle, J. P., and Christensen, B. M. (2005). Age-associated mortality in immune challenged mosquitoes (Aedes aegypti) correlates with a decrease in haemocyte numbers. Cell. Microbiol. 7, 39–51. doi: 10.1111/j.1462-5822.2004.00430.x
Hussain, A., Tian, M.-Y., He, Y.-R., Bland, J. M., and Gu, W.-X. (2010). Behavioral and electrophysiological responses of Coptotermes formosanus Shiraki towards entomopathogenic fungal volatiles. Biol. Control 55, 166–173. doi: 10.1016/j.biocontrol.2010.08.009
Jemberie, W., Hill, S., Rännbäck, L.-M., Ondiaka, S., Raja, N., and Ignell, R. (2018). Evaluation of entomopathogenic fungi Metarhizium anisopliae against dengue virus mosquitoes Aedes aegypti (Diptera: Culicidae). Entomology. 43, 7–18.
Kaaya, G. P. (1989). Glossina morsitans morsitans: mortalities caused in adults by experimental infection with entomopathogenic fungi. Acta Trop. 46, 107–114. doi: 10.1016/0001-706X(89)90004-1
Kaaya, G. P., and Munyinyi, D. M. (1995). Biocontrol potential of the entomogenous fungi Beauveria bassiana and Metarhizium anisopliae for tsetse flies (Glossina spp.) at developmental sites. J. Inverteb. Pathol. 66, 237–241. doi: 10.1006/jipa.1995.1095
Kingsolver, J. G., Arthur Woods, H., Buckley, L. B., Potter, K. A., MacLean, H. J., and Higgins, J. K. (2011). Complex life cycles and the responses of insects to climate change. Integ. Comp. Biol. 51, 719–732. doi: 10.1093/icb/icr015
Lacey, L. A. ed. (2012). Manual of Techniques in Invertebrate Pathology, 2nd ed. Oxford; New York, NY: Academic Press imprint of Elsevier Science.
Laveissière, C., and Grebaut, P. (1990). The trapping of tsetse flies (Diptera: Glossinidae). Improvement of a model: the Vavoua trap. Trop. Med. Parasitol. 41, 185–192.
Liu, H., Skinner, M., Brownbridge, M., and Parker, B. L. (2003). Characterization of Beauveria bassiana and Metarhizium anisopliae isolates for management of tarnished plant bug, Lygus lineolaris (Hemiptera: Miridae). J. Inverteb. Pathol. 82, 139–147. doi: 10.1016/S0022-2011(03)00018-1
López-Sánchez, J., Cruz-Vázquez, C., Lezama-Gutiérrez, R., and Ramos-Parra, M. (2012). Effect of entomopathogenic fungi upon adults of Stomoxys calcitrans and Musca domestica (Diptera: Muscidae). Biocontrol Sci. Technol. 22, 969–973. doi: 10.1080/09583157.2012.699026
Machtinger, E. T., Weeks, E. N. I., and Geden, C. J. (2016). Oviposition deterrence and immature survival of filth flies (Diptera: Muscidae) when exposed to commercial fungal products. J. Insect Sci. 16:54. doi: 10.1093/jisesa/iew032
Maniania, N. K. (2002). A low-cost contamination device for infecting adult tsetse flies, Glossina spp., with the entomopathogenic fungus Metarhizium anisopliae in the field. Biocontrol Sci. Technol. 12, 59–66. doi: 10.1080/09583150120110662
Maniania, N. K., and Odulaja, A. (1998). Effect of species, age, and sex of tsetse on response to infection by Metarhizium anisopliae. BioControl 43, 311–323. doi: 10.1023/A:1009939711555
Maniania, N. K., Okech, M. A., Adino, J. O., Opere, J. O., and Ekesi, S. (2013). Transfer of inoculum of Metarhizium anisopliae between adult Glossina morsitans morsitans and effects of fungal infection on blood feeding and mating behaviors. J. Pest Sci. 86, 285–292. doi: 10.1007/s10340-012-0473-7
Mburu, D. M., Ndung'u, M. W., Maniania, N. K., and Hassanali, A. (2011). Comparison of volatile blends and gene sequences of two isolates of Metarhizium anisopliae of different virulence and repellency toward the termite Macrotermes michaelseni. J. Exp. Biol. 214, 956–962. doi: 10.1242/jeb.050419
McCormick, M. I., and Gagliano, M. (2009). “Carry-over effects-the importance of a goodstart,” in Proceedings of the 11th International Coral Reef Symposium (Florida: Nova South Eastern University), 305–310.
Mnyone, L. L., Kirby, M. J., Lwetoijera, D. W., Mpingwa, M. W., Knols, B. G., Takken, W., et al. (2009). Infection of the malaria mosquito, Anopheles gambiae, with two species of entomopathogenic fungi: effects of concentration, co-formulation, exposure time and persistence. Malar. J. 8:309. doi: 10.1186/1475-2875-8-309
Mnyone, L. L., Kirby, M. J., Mpingwa, M. W., Lwetoijera, D. W., Knols, B. G. J., Takken, W., et al. (2011). Infection of Anopheles gambiae mosquitoes with entomopathogenic fungi: effect of host age and blood-feeding status. Parasitol Res. 108, 317–322. doi: 10.1007/s00436-010-2064-y
Mondal, S., Baksi, S., Koris, A., and Vatai, G. (2016). Journey of enzymes in entomopathogenic fungi. Pac. Sci. Rev. A 18, 85–99. doi: 10.1016/j.psra.2016.10.001
Moraes, A. P. R., Angelo, I., da, C., Fernandes, É. K. K., Bittencourt, V. R. E. P., and Bittencourt, A. J. (2008). Virulence of Metarhizium anisopliae to eggs and immature stages of Stomoxys calcitrans. Ann N Y Acad Sci. 1149, 384–387. doi: 10.1196/annals.1428.008
Moraes, A. P. R., Videira, S. S., Bittencourt, V. R. E. P., and Bittencourt, A. J. (2014). Antifungal activity of Stenotrophomonas maltophilia in Stomoxys calcitrans larvae. Rev. Bras. Parasitol. Vet. 23, 194–199. doi: 10.1590/S1984-29612014037
Morales Hernandez, C. E., Padilla Guerrero, I. E., Gonzalez Hernandez, G. A., Salazar Solis, E., and Torres Guzman, J. C. (2010). Catalase overexpression reduces the germination time and increases the pathogenicity of the fungus Metarhizium anisopliae. Appl. Microbiol. Biotechnol. 87, 1033–1044. doi: 10.1007/s00253-010-2517-3
Mullens, B. A. (1985). Host age, sex, and pathogen exposure level as factors in the susceptibility of Musca domestica to Entomophthora muscae. Entomol. Exp. Appl. 37, 33–39. doi: 10.1111/j.1570-7458.1985.tb03449.x
Mweke, A., Ulrichs, C., Nana, P., Akutse, K. S., Fiaboe, K. K. M., Maniania, N. K., et al. (2018). Evaluation of the entomopathogenic fungi Metarhizium anisopliae, Beauveria bassiana and Isaria sp. for the Management of Aphis craccivora (Hemiptera: Aphididdae). J. Econ. Entomol. 111, 1587–1594. doi: 10.1093/jee/toy135
Niassy, S., Subramanian, S., Ekesi, S., Bargul, J. L., Villinger, J., and Maniania, N. K. (2013). Use of Metarhizium anisopliae chitinase genes for genotyping and virulence characterization. BioMed Res. Int. 2013:1–9. doi: 10.1155/2013/465213
Nnakumusana, E. S. (1985). Laboratory infection of mosquito larvae by entomopathogenic fungi with particular reference to Aspergillus parasiticus and its effects on fecundity and longevity of mosquitoes exposed to sporal infections in larval stages. Curr. Sci. 54, 1221–1228.
Ortiz-Urquiza, A., and Keyhani, N. (2013). Action on the surface: entomopathogenic fungi versus the insect cuticle. Insects 4, 357–374. doi: 10.3390/insects4030357
Paula, A. R., Carolino, A. T., Silva, C. P., and Samuels, R. I. (2011). Susceptibility of adult female Aedes aegypti (Diptera: Culicidae) to the entomopathogenic fungus Metarhizium anisopliae is modified following blood feeding. Parasit. Vectors 4:91. doi: 10.1186/1756-3305-4-91
Quesada-Moraga, E., and Vey, A. (2003). Intra-specific variation in virulence and in vitro production of macromolecular toxins active against locust among Beauveria bassiana strains and effects of in vivo and in vitro passage on these factors. Biocontrol Sci. Technol. 13, 323–340. doi: 10.1080/0958315031000110346
R Core Team. (2020). R: A Language and Environment for Statistical Computing. R Foundation for Statistical Computing, Vienna, Austria. Available online at: https://www.R-project.org/ (accessed July, 2020).
Ravindran, K., Akutse, K. S., Sivaramakrishnan, S., and Wang, L. (2016). Determination and characterization of destruxin production in Metarhizium anisopliae Tk6 and formulations for Aedes aegypti mosquitoes control at the field level. Toxicon 120, 89–96. doi: 10.1016/j.toxicon.2016.07.016
Samuels, R. I., Charnley, A. K., and Reynolds, S. E. (1988). The role of destruxins in the pathogenicity of 3 strains of Metarhizium anisopliae for the tobacco hornworm Manduca sexta. Mycopathologia 104, 51–58. doi: 10.1007/BF00437924
Sánchez-Roblero, D., Huerta-Palacios, G., Valle, J., Gómez, J., and Toledo, J. (2012). Effect of Beauveria bassiana on the ovarian development and reproductive potential of Anastrepha ludens (Diptera: Tephritidae). Biocontrol Sci. Technol. 22, 1075–1091. doi: 10.1080/09583157.2012.713090
Scholte, E.-J., Knols, B. G., and Takken, W. (2004). Autodissemination of the entomopathogenic fungus Metarhizium anisopliae amongst adults of the malaria vector Anopheles gambiae. Malar. J. 3:45. doi: 10.1186/1475-2875-3-45
Scholte, E.-J., Knols, B. G. J., and Takken, W. (2006). Infection of the malaria mosquito Anopheles gambiae with the entomopathogenic fungus Metarhizium anisopliae reduces blood feeding and fecundity. J. Inverteb. Pathol.91, 43–49. doi: 10.1016/j.jip.2005.10.006
Scholte, E.-J., Njiru, B. N., Smallegange, R. C., Takken, W., and Knols, B. G. (2003). Infection of malaria (Anopheles gambiae s.s.) and filariasis (Culex quinquefasciatus) vectors with the entomopathogenic fungus Metarhizium anisopliae. Malar. J. 2:29. doi: 10.1186/1475-2875-2-29
Shahrestani, P., Chambers, M., Vandenberg, J., Garcia, K., Malaret, G., Chowdhury, P., et al. (2018). Sexual dimorphism in Drosophila melanogaster survival of Beauveria bassiana infection depends on core immune signaling. Sci. Rep. 8:12501. doi: 10.1038/s41598-018-30527-1
Skrobek, A., and Butt, T. M. (2005). Toxicity testing of destruxins and crude extracts from the insect-pathogenic fungus Metarhizium anisopliae. FEMS Microbiol. Lett. 251, 23–28. doi: 10.1016/j.femsle.2005.07.029
Talaei -Hassanloui, R., Kharazi-Pakdel, A., Goettel, M., and Mozaffari, J. (2006). Variation in virulence of Beauveria bassiana isolates and its relatedness to some morphological characteristics. Biocontrol Sci. Technol. 16, 525–534. doi: 10.1080/09583150500532758
Taylor, D. B., Moon, R. D., and Mark, D. R. (2012). Economic impact of stable flies (Diptera: Muscidae) on dairy and beef cattle production. J. Med. Entomol. 49, 198–209. doi: 10.1603/ME10050
Therneau, T. (2015). A Package for Survival Analysis in S. Version 2.38. Available online at: https://CRAN.R-project.org/package=survival (accessed July, 2020).
Unlu, I., Suman, D. S., Wang, Y., Klingler, K., Faraji, A., and Gaugler, R. (2017). Effectiveness of autodissemination stations containing pyriproxyfen in reducing immature Aedes albopictus populations. Parasit. Vectors 10:139. doi: 10.1186/s13071-017-2034-7
Valero-Jiménez, C. A., Debets, A. J., van Kan, J. A., Schoustra, S. E., Takken, W., Zwaan, B. J., et al. (2014). Natural variation in virulence of the entomopathogenic fungus Beauveria bassiana against malaria mosquitoes. Malar. J. 13:479. doi: 10.1186/1475-2875-13-479
Vogelweith, F., Körner, M., Foitzik, S., and Meunier, J. (2017). Age, pathogen exposure, but not maternal care shape offspring immunity in an insect with facultative family life. BMC Evol. Biol. 17:69. doi: 10.1186/s12862-017-0926-y
Walker, A. R. (1990). “Disease caused by arthropods,” in Handbook on Animal Diseases in the Tropics, 4th edn, eds M. M. H. Sewell, and D. W. Brocklesby (London: Bailliere Tindall), 44.
Wamiti, L. G., Khamis, F. M., Abd-alla, A. M. M., Ombura, F. L. O., Akutse, K. S., Subramanian, S., et al. (2018). Metarhizium anisopliae infection reduces Trypanosoma congolense reproduction in Glossina fuscipes fuscipes and its ability to acquire or transmit the parasite. BMC Microbiol. 18:142. doi: 10.1186/s12866-018-1277-6
Keywords: Metarhizium anisopliae, Stomoxys calcitrans, biological parameters, pre-lethal effects, ICIPE 30
Citation: Baleba SBS, Agbessenou A, Getahun MN, Akutse KS, Subramanian S and Masiga D (2021) Infection of the Stable Fly, Stomoxys calcitrans, L. 1758 (Diptera: Muscidae) by the Entomopathogenic Fungi Metarhizium anisopliae (Hypocreales: Clavicipitaceae) Negatively Affects Its Survival, Feeding Propensity, Fecundity, Fertility, and Fitness Parameters. Front. Fungal Biol. 2:637817. doi: 10.3389/ffunb.2021.637817
Received: 04 December 2020; Accepted: 02 February 2021;
Published: 24 February 2021.
Edited by:
Nicolas Pedrini, National University of La Plata, ArgentinaReviewed by:
Avelino José Bittencourt, Universidade Federal Rural Do Rio de Janeiro, BrazilJuscelino Rodrigues, Universidade Federal de Goiás, Brazil
Copyright © 2021 Baleba, Agbessenou, Getahun, Akutse, Subramanian and Masiga. This is an open-access article distributed under the terms of the Creative Commons Attribution License (CC BY). The use, distribution or reproduction in other forums is permitted, provided the original author(s) and the copyright owner(s) are credited and that the original publication in this journal is cited, in accordance with accepted academic practice. No use, distribution or reproduction is permitted which does not comply with these terms.
*Correspondence: Steve B. S. Baleba, sbernardsteve@gmail.com; Daniel Masiga, dmasiga@icipe.org
†Present address: Steve B. S. Baleba, Department of Evolutionary Neuroethology, Max Planck Institute for Chemical Ecology, Jena, Germany