- Key Laboratory of Inorganic Nonmetallic Crystalline and Energy Conversion Materials, College of Materials and Chemical Engineering, China Three Gorges University, Yichang, China
Covalent organic frameworks (COFs), one of a multitude of advanced mainstream materials in photocatalysts, are crystalline porous materials with tunable topology and high porosity and are connected by dynamic covalent bonds. We sought to achieve efficient photocatalytic yields, making the stability of the bond energy crucial. Stable bond energy will enable our products to be more economically efficient. At present, the main photocatalysts are reversible imine bonds and other 2D COFs, but irreversibly stable COFs are seldom involved. Therefore, this review surveys the relevant literature to explore the stability of irreversible bond energy, elaborating the synthesis schemes of irreversible bonds. Irreversibly connected bonds will improve the stability and durability of our synthesized catalyst in practical applications, so we seek to explore the stability of bond energy for our practical applications. In this review, we offer a detailed summary of the irreversible bonding pattern, explore the stability of COFs, and summarize classic highly crystalline ordered semiconductor COFs with irreversible bonding methods.
1 Introduction
The pioneering work in photocatalysis was published by Fujishima and Honda in 1972, in which photoelectrochemical cells utilized titanium dioxide as an N-type semiconductor to achieve the decomposition of water under ultraviolet radiation (Ghosh et al., 2022). Great efforts have been invested in such research, and many experiments on photocatalytic degradation have been conducted. In the photocatalytic process, there are three principal steps: (i) light absorption, (ii) electron–hole pair separation, and (iii) surface redox reactions (Gong et al., 2023). Superior photocatalytic performance depends on these steps. In recent years, many conventional photocatalysts, mostly inorganic semiconductor materials, have been widely used for various photocatalytic reactions due to their low expense, stability quality, and ease of modification. However, practical applications have been greatly hampered by the fact that conventional photocatalytic materials exhibit a narrow range of photoresponse, rapid compounding of solar energy, and poor electron–hole pair utilization efficiency (Xu et al., 2019). Therefore, preparation of novel catalysts with high stability and performance is of the highest priority in the field of photocatalysis.
Semiconductor polymer materials have had a profound impact on photocatalysis. In particular, the concept of covalent organic frameworks (COFs) has been much discussed in photocatalysis since 2005, when it was introduced by Cote et al. (2005). Despite the large amount of published research on COFs since, almost all COFs are still synthesized in two dimensions and based on reversible covalent bonds. The crystalline nature of COFs with imine structures, although excellent, inherently limits their stability, thereby weakening the chemical bonding-linked COFs. Their property of irreversibility makes the synthesized catalyst in the whole photoreaction process irreversible; in terms of dynamics and thermodynamics, the energy in the whole process is stable and cannot be reversed. This will reduce the bidirectional partial energy loss of the reversible process. Furthermore, the reversible bond thermodynamically conserves energy but is erratic. Labile bond energy will limit the electron–hole pairs on the catalyst surface after exposure to sunlight, and charge carrier mobility will also be affected. To ensure yield, the most critical factors for photocatalytic materials are inhibiting the recombination rate of carriers on the catalyst surface after exposure to sunlight and promoting the more excited charge generation. In addition, photocatalytic materials not only have surface redox reactions in the process of illumination but also experience partial self-decomposition, which greatly impacts the practical application of the catalyst. Reducing the reaction process using more stable bond energy would solve the diminished energy in the reaction process and promote a more effective yield. It has been found that irreversible bond COFs can improve the performance of the catalyst and reduce the extent of energy. The reversibility of covalent bonds indicates that the bond energy will be poorly stable solely due to reversibility, which results in the two-dimensional imine bonds not performing well as photocatalysts. To solve this instability problem, researchers from many disciplines consider it necessary to develop COFs with irreversible bonds and stable chemical properties. Although some crystal COFs formed by irreversible reactions have been reported (Daugherty et al., 2019), the method of converting reversible bonds into irreversible bonds is still very difficult because irreversible reactions generally produce non-crystalline polymers that exhibit different morphological characteristics; due to their amorphous nature, their structures are difficult to characterize (Zhai et al., 2019a). Therefore, it is a great challenge and opportunity to develop irreversible bonds in the yield of COFs, achieving the high stability of bonding energy that is anticipated in design. It will also guide the research field of COFs and expand new patterns in photocatalysis.
The most important factor in determining the properties of material iscatalytic efficiency (Kumar et al., 2019), which itself depends on the stability of the material. COFs have been increasingly utilized due to their pre-designed structure, higher specific surface area, and easy molecular functionalization as crystalline porous polymers (Ascherl et al., 2016). Most COFs that have been developed have two-dimensional (2D) structures (Sick et al., 2018), composed of reversible imine bonding. COFs are simultaneously transportable and have a higher specific surface area, enhanced available active sites, and extremely crystalline and porous structures (Nawaz et al., 2021). Their self-contained properties make COFs very promising for efficient energy transformation and environmental employability, with many studies proposing them for various applications in photocatalysis (Wang J. et al., 2017). However, COFs have drawbacks: their limitations under dispersion and the insolubility of microcrystalline powder in solvents (Wang K. et al., 2017). Stability of catalysts is thus one of the essential indicators of preferred performance. We here discuss the exploration of the stability of COF materials, the existing research on irreversible bonds, and the progress of some irreversible bonds in photocatalysis.
2 Covalent organic framework photocatalytic materials
Photocatalytic materials have recently undergone rapid development; organic photocatalysts have been especially popular in photocatalysis. Compared with traditional inorganic photocatalysts, organic photocatalysts are more versatile, more diverse in synthesis, and can also be tuned to different light-capture capabilities or band gaps. The production process is simpler, they are lighter in mass, and they are more eco-friendly than inorganic materials (Yan et al., 2013). Among the current mainstream photocatalytic materials, COF materials have seen a rapid development within the last decade due to their macroporous adjustable structure (Xin-Le et al., 2020). Especially widespread in photocatalysis are 2D COFs, with imine bonding being one of the most common bonding methods in existing studies of COFs (Lohse and Bein, 2018). However, after much research, imine bonds have been found to be generally unstable, often difficult to isolate, and prone to hydrolysis reactions, which, when hydrolyzed, generate aldehydes or ketones (Zhai et al., 2019b). Therefore imines, especially Schiff bases, can be used as carbonyl-protecting groups in synthesis. Imines are generally unstable and are prone to decomposition under alkaline conditions (Kandambeth et al., 2019). To address this, research is being directed to porous materials of COFs with relatively excellent stability. Among this research is Tang et al. (2021), who demonstrated in their groundbreaking work that the authentic potential of organic photocatalysts was detected when relatively efficient H2 was produced using graphitic carbon nitride loaded with Pt co-catalysts (Uribe-Romo et al., 2009). Cooper and colleagues have reported on conjugated microporous polymers (CMPs) (Cote et al., 2005), while Thomas and colleagues have reported on covalent triazine-based frameworks (CTFs) (Huang et al., 2022). COFs are a potential family of porous organic materials with periodic networks and highly organized topologies (Zhao et al., 2021). The effective conversion of unstable bonds into stable ones is an important goal for COFs in the development process, since stable irreversible bonds can improve the stability and crystallinity of the synthesized material. Current mainstream crystalline materials generally form reversible bonds through thermodynamically controlled reactions—a process also known as dynamic covalent chemistry (DCC) (Rowan et al., 2002). This uses a vast range of chemical factors to produce COF materials with high crystallinity and permanent porosity. They maintain the ideal connection of bonds in COFs so that the attached bonds are not thermally dissociated and do not recombine into other configurations or produce long-range ordered materials (Smith et al., 2016). Another aspect is the reversibility of chemical bonds in COFs that exert erratic bond energy. Transforming erratic reversible bonds into stable irreversible bonds while maintaining high crystallinity is a major challenge for researchers.
The synthesis of COF catalysts with irreversible and stable bond energy is of great significance for our subsequent practical catalyst application, which not only depends on the proportion of production at the time of application but also on whether the catalyst can be recycled often in the application process to reduce costs. Therefore, the synthesis of COF catalysts with irreversible and stable bond energy has profound significance to the study of stable bond energy for photocatalysts. We have therefore surveyed several research articles on the transformation of erratic reversible bonds into stable irreversible bonds based on imine bonds. We welcome criticism and correction of any problems or deficiencies here presented and will incorporate these into our research.
3 Post-synthetic modification
We have collated the defects of reversible bonds in some COF materials. Much research has demonstrated that COF materials already make a significant contribution to photocatalytic semiconductor materials. However, 2D topology is currently the most common application for COF materials in the field of synthetic COFs (Colson and Dichtel, 2013); 2D network structure with submarine bonds is also the most synthesized and widely used application area. However, synthetic imine bonds are also prone to hydrolysis and unstable performance due to their characteristics, which have resisted the widespread popularization of photocatalytic reduction, oxidation, and hydrolysis (Kandambeth et al., 2012). To solve this problem, many researchers have found that irreversible bonds can be obtained by pushing the reversible bonds backward with stronger bond energy and higher stability. This provides a broader platform for many scholars to solve the problem of the instability of reversible bonds and has made an important contribution to the widespread use of irreversible bonds in the field of photocatalysis for CO2 reduction and H2 and H2O2 production. In the following, we summarize findings on irreversible bonds in the synthesis and application of COFs.
3.1 A combination of reversible and irreversible pathways
COFs synthesized by submarine bonding undergo reversible reactions during synthesis (Suematsu et al., 1983) and usually also completely decompose under ambient humidity. Doping pyriridine 9c and alkyl 9a showed a minor improvement in hydrolytic stability. Nevertheless, these modifications reduce gas adsorption, although they improve hydrolytic stability to a lesser extent. Thus, the issue of COF instability is still a challenge and hinders the practical application of COFs.
This article reports for the first time the synthesis of two novel COFs (Figure 1), TpPa-1 and TpPa-2, using a combination of reversible and irreversible organic reactions (Huang et al., 2016). They are synthesized by reacting 1, 3, 5-triformylchlorophenol (Tp) with p-phenylenediamines (Pa-1) or 2, 5-dimethyl p-phenylenediamines (Pa-2), respectively.
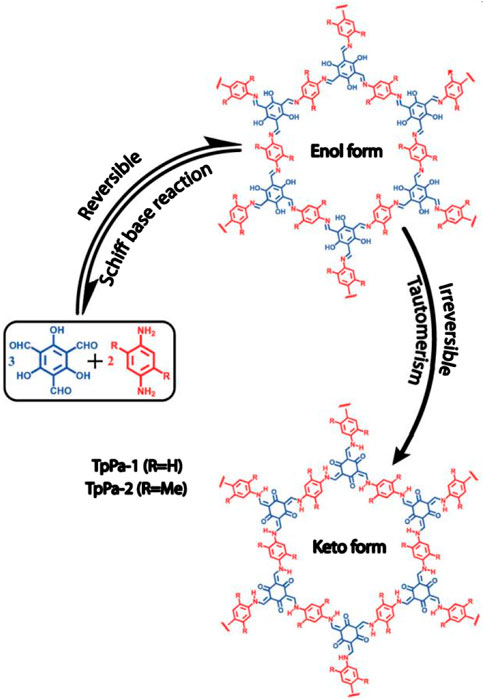
FIGURE 1. Schematic diagram of the reversible and irreversible combined reactions of 1,3,5 tricarboxyresorcinol with p-phenylenediamine and 2,5-dimethylenediamine, respectively, for the synthesis of TpPa-1 and TpPa-2. The whole reaction was divided into two steps: (1) reversible Schiff base reaction and (2) irreversible enol-keto interconversion isomerization.
First, the compound is polymerized, by using the reversible Schiff base reaction to form the crystalline skeleton, which then undergoes irreversible enolone tautomerization, thus enhancing the chemical stability of the overall structure of the catalyst. The crystallinity of the COF is not affected by the irreversible properties but only involves the bond movement and keeps the atoms in the same position. TPA-1 and TPA-2 showed good resistance to boiling water and acid treatment, and TPA-2 also showed preferred stability in basic media (9N sodium hydroxide). Under these conditions, both COFs maintained excellent crystallinity and excellent gas adsorption properties, while the stability in water is due to the irreversible enol-ketone tautomer generated. It is also found in simple N-salicylate anilines and is more stable.
Arylene and imine nitrogen (C-N) are stronger than phenolic oxygen (O-H). While the main factor in the single-substituted N-salicylate is still the aromatic nature, and the compounds can only exist as enols, basic imines can be changed to a ketone. Even after heating the sample to a very high temperature, the equilibrium is not destroyed and is considered irreversible. In contrast, the COF catalyst synthesized in this study is also very stable in an acidic environment due to the formation of enol bonds. Sharath Kandambeth demonstrated that, at very high pH, the secondary nitrogen underwent deprotonation, resulting in the reverse conversion of the keto to the enol form. Their hypothesis is that when treated with strong bases such as lithium diisopropylamide, the compound is deprotonated to form the structural chelation of keto-enol tautomerization.
To overcome base unsteadiness, two large methyl groups in TPA-2 are located near the secondary nitrogen center (CN), and the hydrolysis stability in water increases accordingly. The PXRD peak position of TPA-2 was retained after 7 days of NaOH treatment, although a slight decrease in peak intensity was observed. Sharath Kandambeth indicated the first new scheme for the synthesis of a COF for high acid- and alkali-stable crystallization.
In addition, the introduction of a large alkyl group near the nitrogen center slightly reduces the surface area of the prepared COF catalyst but partly solves the base stability problem; Sharath Kandambeth believes that increasing the length of the diamine ligand would improve the surface area and gas adsorption properties of these synthesized COFs. The sheet metals present in the material can be used as a carrier for doped nanoparticles, which are more conducive to catalysis. In this study, by increasing the length of the diamine ligand and doping a large alkyl group, the surface area and gas adsorption performance of the modified COF was greatly improved. Increasing the surface area, which is more conducive to the separation efficiency of charge carriers on the surface of COFs after illumination and is also more conducive to illumination, will also improve the yield. Compared with before and after modification, the COF yield of the irreversible stable bond formed after modification is higher, which also results in the desired performance improvement.
3.2 Stable aromatic benzoxazole linkage
Efficient conversion of unstable bonds to stable bonds is a major goal of COF chemistry, as it improves stability and preserves crystallinity. To make COFs stable even under alkaline conditions with irreversible bonds, Jeong-Min Seo went through previous studies. After synthesis, the cyclization bond was converted into the stable aromatic benzoxazole-linked BO-COF (Figure 2), forming a polymer with the chemical properties of a thickened aromatic ladder structure. Efficient postprocessing of the bond of synthesized unstable COFs to stable irreversible COFs (Stewart et al., 2017) can now significantly improve stability and crystallinity. Post-postprocessing strategies are widely used to synthesize stable thickened aromatic COFs (Li et al., 2018). The effective posttreatment conversion of the unstable reversible COF into a stable irreversible COF will improve the stability and crystallinity of the catalyst. This strategy is generally applicable to the synthesis of steady states.
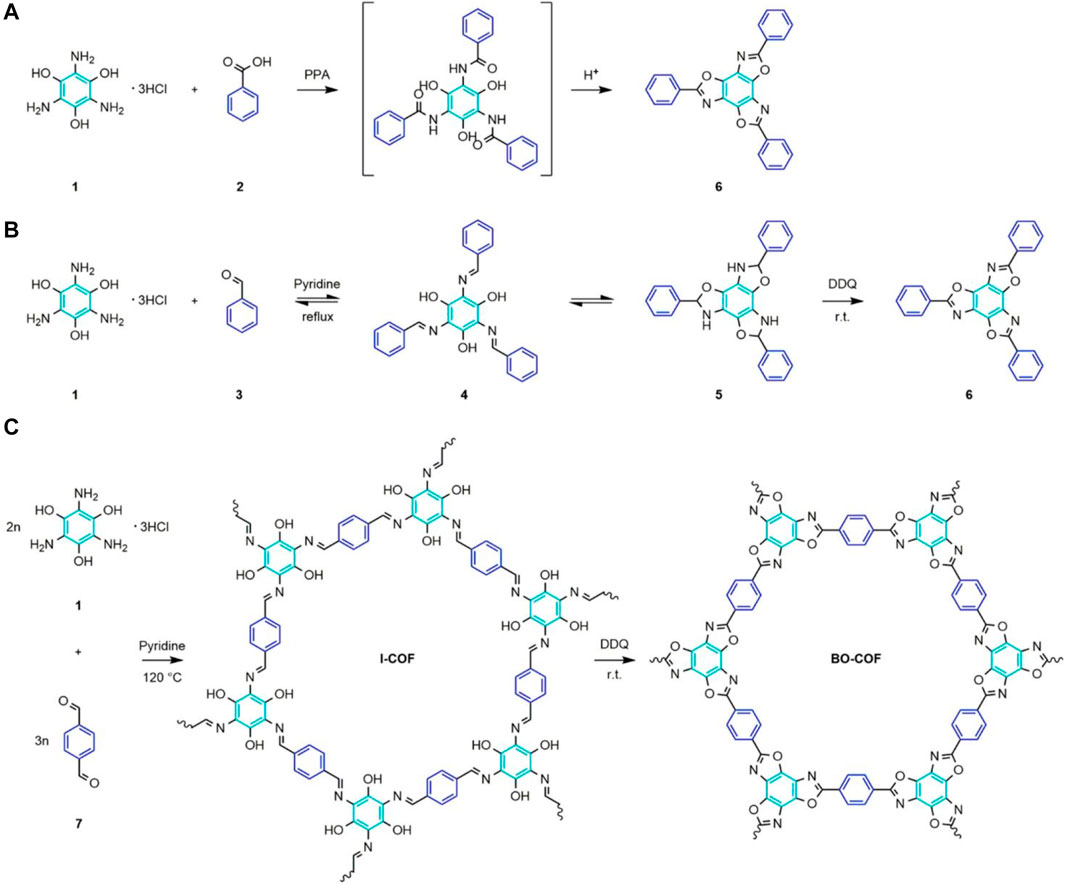
FIGURE 2. (A) One-step irreversible condensation of TABT (1) with benzoic acid (2) in the PPA medium to form benzoxazole compound (6), forming a benzoxazole ring. (B) Mimicking the reaction of TABT (1) with benzaldehyde (3) to generate reversible imine compound (4) and its reciprocal isomeric intermediate benzoxazole structure (5), and oxidative cyclization with DDQ to finally transform to benzoxazole compound (6). (C) I-COF was synthesized by the reaction of TABT (1) and TPA (7), followed by oxidative cyclization of I-COF with DDQ to produce BO-COF.
In the course of their study, researchers found that an alternative was to convert the unstable bonding of imine bonds into another stable bond through postprocessing. However, this will make the utility of the catalyst in practice not ideal, so a more reliable method was developed to improve its utility. Constructed a stable new bonds to need a stable connecting rod to support first (So et al., 1998). The COFs in this report were crystallized by an irreversible cascade of reactions involving continuous cyanide migration, closed-loop reactions, and oxidation reactions (Pyles et al., 2018). Bond energies present in acids and bases are also irreversible, with high chemical stability, allowing them to undergo post-synthetic modification under harsh conditions to install pre-designed functions. We emphasize that functionalized COFs are specialized carriers for super proton conduction. Nevertheless, most COFs are synthesized via covalent binding, which is reversible. The synthesized catalyst will have crystal properties, but its stability will be impaired, making the structure and bond energy of the catalyst unstable. It is thus necessary to develop new irreversible stable bond energy. Although some irreversible bonds have been reported, current mainstream research still focuses on two-dimensional reversible bonds. This is attributed to the fact that irreversible reactions during synthesis cause noncrystalline polymers to also exhibit different functions, and their amorphous properties make it difficult to characterize their structure. Therefore, the development of irreversible bonding chemistry in the field of COFs is promising but is nevertheless time- and energy-consuming.
Organic molecules are connected to the extended structures by covalent bonds to form linear one-dimensional (1D), two-dimensional (2D), and three-dimensional (3D) polymer structures. In general, polymers with porous 2D and 3D networks form amorphous materials. This creates a lot of uncertainty in the characterization process. The synthesized catalyst’s crystallinity is also related to performance, which can limit its practical application. Hemodynamic reactions can be achieved that form reversible bonds. Until now, all mainstream syntheses of stable bonds use this postprocessing method, with the synthetic catalyst material having very high crystallinity and long-term porosity. For example, if the bond in COFs is not as desired, the synthesized COF material can be thermally dissociated into the desired long-range ordered material. The key to any new method of building a COF is selecting stable connections so that the catalysts synthesized will have better and more stable performance. In this study, linear aromatic polymers were chosen to find stable bonds. Aromatic heterocyclic polymers, including polybenzoxazole (PBO), form irreversible aromatic rings during reaction formation, showing high stability. Typically, benzoxazole bonds are formed by irreversible processes, such as the formation of aromatic rings between o-aminophen [Ph- (amino) -OH] and benzoic acid (PH-COOH) at high temperatures in the presence of acidic catalysts. The thermodynamic energy gain after the formation of the aromatic oxazole ring by double condensation (2H2O per reaction) prevented the reverse reaction (Scheme 2A). Another scheme for synthetic benzoxazole bonds is the oxidative cyclization of the phenol Schiff base resulting from the condensation of 2-aminophenol and aldehyde. However, COF formation with high crystallization properties is a reversible process. It has been reported that benzoxazole-linked COFs can be synthesized in a one-pot method. This scheme would kinetically cause a “defect correction” at an ordered position to rapidly form the oxazole ring through an irreversible oxidation reaction, thereby minimizing the reverse reaction. Moreover, a post-modification method of imine bonds exchanging via a heterojunction has also been reported. The direct transformation adopted in this study works. The reversible imine-linked bonds are cyclized through oxidation and then transformed into irreversible benzoxazole-linked bonds. The resultant monomer, BO-COF, used a two-step reaction sequence (Scheme 2C) of the formation and oxidation of imine bonds. First, 1,3,5-tri amino 2,4,5-benzyl enol (TABT) and para benzaldehyde (TPA) were reacted in methanol and medium methanol for 3 days to obtain I-COF.
In summary, compared to the one-pot strategy, the COF synthesized by Jeong-Min Seo can maintain the original topology, crystallinity, and porosity along with improved thermal and chemical stability. They focused on improving stability by applying the stepwise reaction to fix the ordered structure of the frame before conversion into a stable benzoxazole bond. In this COF article, the strategy for synthesizing stable bonds is introduced. To a large extent, the post-synthetic modification method introduced provides reference significance for modifying COF with stable bond energy based on the synthesized COF and obtaining higher performance. It provides guidance for further study and synthesizes modification strategies to improve catalyst stability.
3.3 Benzofuran-linked
The article proposes the first critical step of how cyanide shifts into a COF cascade (Peng-Lai et al., 2019). Yan Su investigated the combination of 2,5-dihydroxyphenylglydialdehyde (DHPA) or 2,4,6-trihydroxybenzene-1,3,5-tricarboxaldehyde (THTA) -base with 2,2,2- (benzene 1,3,5-triplet) -tri acetonitrile (BTTA), or 2′(1,4-benzene) acetonitrile (PDA) to synthesize two organic frames with high crystallinity and porosity. The first was to create a major large frame through reversible reactions, resulting in irreversible heteroaromatic cyano-substituted benzofuran bonds through continuous cyanide migration, ring closure, and oxidation reactions (Zhang et al., 2016). By extending the synthesis of COFs through irreversible reactions, the crystallinity, porosity, and feasibility of remodification are further extended to a stable chemical bond framework. In particular, Yan Su emphasizes that the special carrier of super proton conduction is the result of acid hydration.
The initial target was to synthesize molecular analogs of cyanogen-substituted phenyldifuran bonds to determine the basic properties of the cyanogen-substituted phenyldifuran ring. It is worth noting that dividing the reported copper salt-catalyzed benzofuran reaction, synthesized benzofuran with high yield through a transition metal-free catalyst process—excluding the potential factors for the functional design of COFs—moreover found that the slow evaporation of chloroform solution produces crystals.
Yan Su emphasized that the acid-lyzed COFs have special carriers of super proton conduction. The initial goal was to synthesize cyanosubstituted benzofuran-linked molecular analogs to determine the fundamental properties of cyanosubstituted benzofuran rings. Two kinds of crystalline and porous COFs with new benzofuran bonds were synthesized by irreversible cascade reaction. The resulting benzofuran bond has high stability properties and introduces new functions in the COFs modified after synthesis. Yan Su demonstrated that acidified covalent organic frame materials show superproton conductivity, further improving their practicality. This provides a new idea for the construction of irreversible and stable COFs. In this study, a two-dimensional COF was synthesized by connecting hydroxybenzaldehyde and acetonitrile components to form a cyano-substituted benzofuran bond (As shown in Figure 3). The catalysts thus formed have irreversibility, have high chemical stability in acids and bases, and are post-synthetic and modified under harsh conditions before the installation of pre-designed functions onto the synthetic skeleton. While irreversible bonds have made recent progress, the mainstream synthetic COFs are still based on reversible covalent bonds with crystal properties; however, reversible bonds have limited stability and are fragile in terms of chemical properties. The way to solve the bottleneck is to take new material steps to make the catalyst functionally long-range ordered, which will make the synthesized COF under stable control in terms of bond energy. In currently reported crystal COFs, stabilizing the synthesis of irreversible bonds is still uncommon. Irreversible reactions usually produce amorphous polymers with different functions, and structural characterization is difficult to some extent due to their amorphous nature. Under alkaline conditions, GSCOF-1 and-2 were obtained under oxygen atmosphere and at K-110°C in high yields (88% and 92%, respectively). The reaction step plays a crucial role in forming an ordered backbone structure. Moreover, oxygen is necessary for the reaction; those products without oxygen were impure.
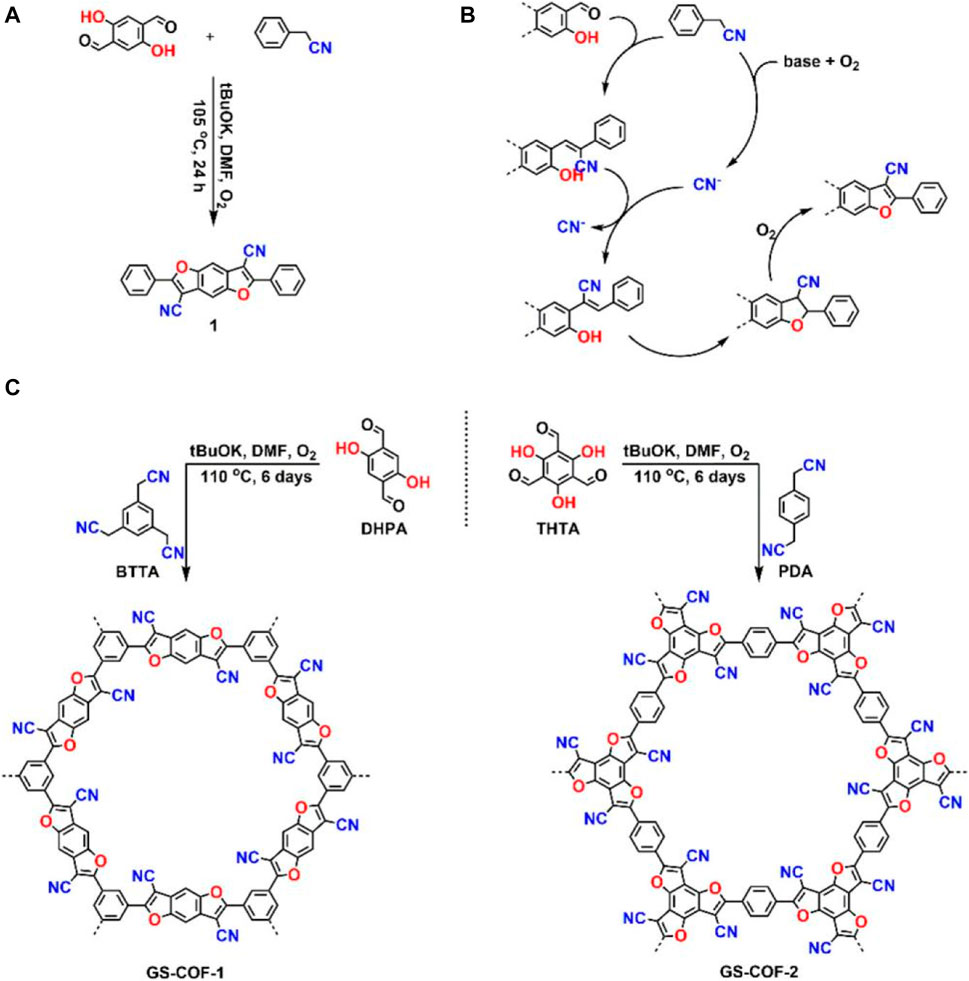
FIGURE 3. (A) Synthesis of molecular analogs. (B) Proposed mechanism of benzofuran bond formation. (C) Synthesis of GS-COF-1 and -2.
In conclusion, two COFs with crystalline and porous novel benzofuran bonds were synthesized using irreversible rearrangement. The resulting bonds allow, due to high stability factors, the introduction of new functional groups in COFs under harsh conditions. This strategy provides a new method for constructing special properties not easily available in a conventional COF. Yan Su designed their ideas in advance and synthesized the COF for stable performance to insure against various unexpected conditions that occur during synthesis. The synthesized COF was designed in advance to ensure that the overall synthesis process can develop according to prior direction. Compare with the two articles mentioned above, this article provides a broader concept for the subsequent synthesis of stable COF. Designing in advance and mastering the general idea of synthesizing COF ensured that an ideal and expected state could be reached.
4 Direct synthesis
4.1 Irreversible amide-linked
Designing high-capacity, fast kinetic, stable, selective gold recovery adsorbents is challenging but also economically and environmentally important. In this article, JNU-1 COF with an irreversible bond was constructed, and the resultant catalyst was found to be useful for gold recovery (Qian et al., 2020). The exchange of 4,4′-biphenyl (BA) with trobenphthalyl chloride, synthesized in a parent covalent organic frame TzBA consisting of 4,4′,4″-(1,3,5-triazine-2,4,6-triyl) triphenylamine (Tz) and BA. JNU-1 has high stability, good selectivity, kinetic feasibility, and strong adsorption capacity. After extensive calculations, Hai Long Qian showed that the excellent performance of JNU-1 in the gold recovery process is caused by the formation of hydrogen bond C (N) -H and the coordination interaction of Cl and O with Au (Figure 4).
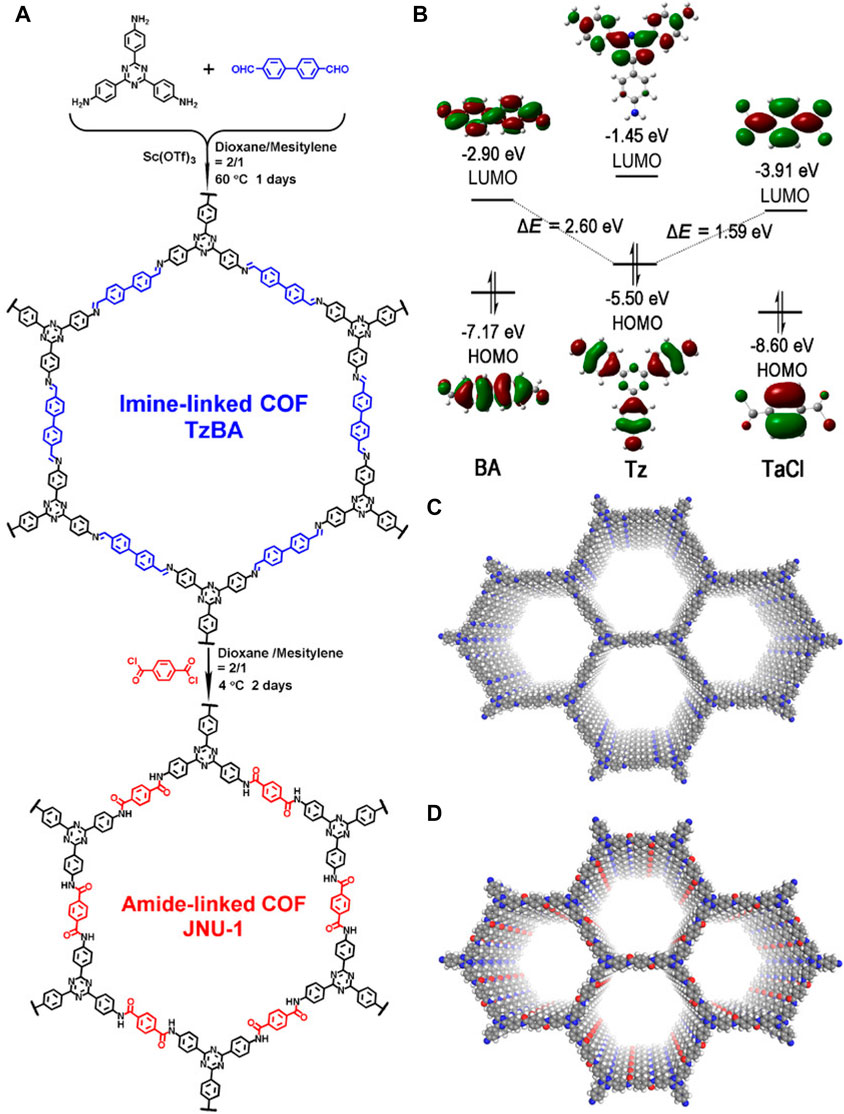
FIGURE 4. (A) Design of the BBE strategy for JNU-1 synthesis. (B) Computational diagram of the HOMO-LUMO interaction of Tz with BA and TaCl. (C) Space-filling model of TzBA. (D) Space-filling model of JNU-1 (gray, C; blue, N; A, red; white, H).
Gold is an important precious metal from an economic and environmental perspective, but, from the point of view of energy used in its extraction, numerous scholars have turned their attention to recycling gold from secondary resources such as electrical and electronic equipment waste. There are many techniques for recovering gold from aqueous solutions, including adsorption, precipitation, solvent extraction, and ion exchange. The porous adsorption method has attracted wide attention in recent years due to its ecological friendliness, lower process cost, and simple operation. At present, developing high-performing adsorbents is required to meet the conditions of gold recovery. Most of these adsorbents undergo lengthy kinetic processes, taking hours or even days to reach equilibrium. In addition, effective recovery of gold depends on the selected adsorbent. The design of a stable adsorbent with large capacity and fast dynamics for the rapid extraction of gold is a difficult issue for researchers. Stability is central in the application of COFs. The formation of most typical COFs is based on dynamic covalent chemistry. The chemical bonds formed during synthesis are both reversible and irreversible. However, the overall focus is on the crystallization of the resultant monomer, which is also an inherent limiting factor for the stability of COFs. Only few researchers have intensively explored this possibility. Polyaryl ether COFs were synthesized by an irreversible nucleophilic aromatic substitution reaction. The preparation conditions for irreversible benzoxazole and amide-linked COFs should ensure chemical transformation at high temperatures and high pressure. However, the existing synthetic, stable, and irreversible key applications are not yet widely available. The synthetic COF-based catalyst, which has a block strategy, redesigns bonds and functional groups on its synthesized amide bonds to confer irreversible properties, thus improving its stability.
Many researchers found that preparing COFs based on irreversible bonds is effective for obtaining stable COFs, but it is extremely difficult to synthesize COFs with irreversible properties. The BBE synthesis strategy expanded a good idea for the development of irreversible and stable bond energy. However, it was never designed to prepare irreversible COFs. The highlight of this article lies in the use of this strategy to synthesize and design highly stable COFs for irreversible connections (Figure 4A). The irreversible amide bonds with high gold selectivity were chosen as effective connections of COF materials. Tz was first condensed with BA to prepare the crystalline parent COF-TzBA, and the highly nucleotropic benzoyl chloride (TaCl) was selected to exchange the BA block of TzBA to form JNU-1, which is more conducive to the chemical reaction in the frontier track theory.
Finally, Hai Long Qian demonstrate that the BBE synthesis strategy for the preparation and design of a highly stable COF is feasible and can be used for ultra-fast and selective gold recovery. The introduction of irreversible bonds in covalent organic frame structures is a promising method for preparing stable covalent organic frames, although traditional methods make it difficult to obtain stable covalent organic frames based on irreversible bonds. Here, the example of amide-linked JNU-1 demonstrates the great potential of the BBE strategy for preparing highly stable irreversible COFs. The irreversibility and selectivity of amide bonds to gold is a prerequisite for designing a highly stable JNU-1 for efficient gold recovery. The proton–amide bond in the ordered COF network provides a high boost in JNU-1 at both the kinetic level and in selectivity and adsorption through the backbone hydrogen interaction of] C-H with [AuCl4], and the coordination of Cl with Au and O. This is more favorable to the catalyst than sunlight in the redox reaction process, increasing the electron-hole pairs of the catalyst on the surface and thus further improving the performance of the catalyst. Therefore, transforming the reversible bond into an irreversible bond is more promising.
4.2 Crystalline dioxin-linked
Triangles 2,3,6,7,10,11-hexahydroxytribenzene (HHTP) and linear tetrafluorophthalonitrile (TFPN) or 2,3,5,6-tetrafluoron-4-pyridine nitrile (TFPC) are connected by dioxin bonds to form two-dimensional COFs called COF-316 and COF-318 (Zhang B. et al., 2018). In the process of synthetic COF-based photocatalysts, the common synthesis method is a condensation reaction, but the traditional synthesis method of COF is generally reversible from the perspective of bond energy, and the performance is unstable. We seek to investigate the synthesis of stable and irreversible bonds. Therefore, the reactions used in this report are based on nucleophilic aromatic substitution reactions that proved to be bond-energy irreversible. Bing Zhang showed that using nitrile substituents in synthesis to enhance the reactivity between monomers makes the construction of planar units easier for further reaction. The resulting COF material has a framework with high chemical stability in both acid and base. In this article, COFs are reduced in crystal form to determine the structure of COF materials at the atomic scale, which Bing Zhang believes will ultimately advance the current rapid growth of irreversible bonds in the area of COF materials. COF-316 requires extreme conditions for post-synthetic modification and covalently installs a function not otherwise available. The network chemistry of COFs represents the practice of organic chemistry in two and three dimensions, going beyond discrete molecules (0D) and polymers (1D). Implementing COFs in crystal form is an important component in determining their structure at the atomic scale and ultimately advancing the current rapidly evolving field. The formation of conventional reversible covalent bonds is required for the crystallization of COFs, but this aspect inherently limits their high stability because it makes these materials chemically fragile. To take COF chemistry beyond this conventional dichotomy so that irreversible bonds can also produce crystallization, Bing Zhang in this article hypothesize that it is necessary to develop junction chemistry to provide further potential in our original synthesis. Strategies such as tautomerization and junction transformation have been used to enable easily hydrolyzed backbones to improve their stability. Specifically, in the presence of the bases, the strong electron-withdrawing substituents of the synthetic monomer on the building unit make them susceptible to nucleophilic attack by the hydroxyl functional group of HHTP (Figure 5C). From two consecutive aromatic nucleophilic substitution reactions, the closed-loop generates an irreversible heteroaromatic dioxin-linked bond. The COFs synthesized in the article succeeded in extending the range of mesh synthesis to irreversibly connected bonds in this respect. The initial goal was to synthesize a dioxin-linked molecular analog (Figure 5A) to determine the planarity of the dioxin-linked ring. The acetonitrile solution was slowly evaporated to generate the crystals. Single-crystal X-ray diffraction confirmed that the 1,4-dioxin ring was coplanar with the gel benzene ring. Bing Zhang attempted to synthesize COFs by using the two monomers they selected as linear and triangular linker reactions. The nitrile group of the TFPN monomer has strong electron-withdrawing properties that can enhance the electrophilicity of the CF bond and thus increase the reactivity to the HHTP monomer. Many practices were applied to screening various synthetic conditions, including linkers, solvent mixture, temperature, and reaction time on the synthesized COF materials. In the presence of stoichiometry, a crystal framework called COF-316 was successfully synthesized. It is assumed that the stiffness of building components and the strong directionality of the link influence the order of improving the structure. Moreover, the nitrile group is essential for the reactivity of the nucleophilic substituents, and it was found that the weaker electron-withdrawing groups, such as trifluoromethyl and aldehyde substituents, failed to produce a complete reaction.
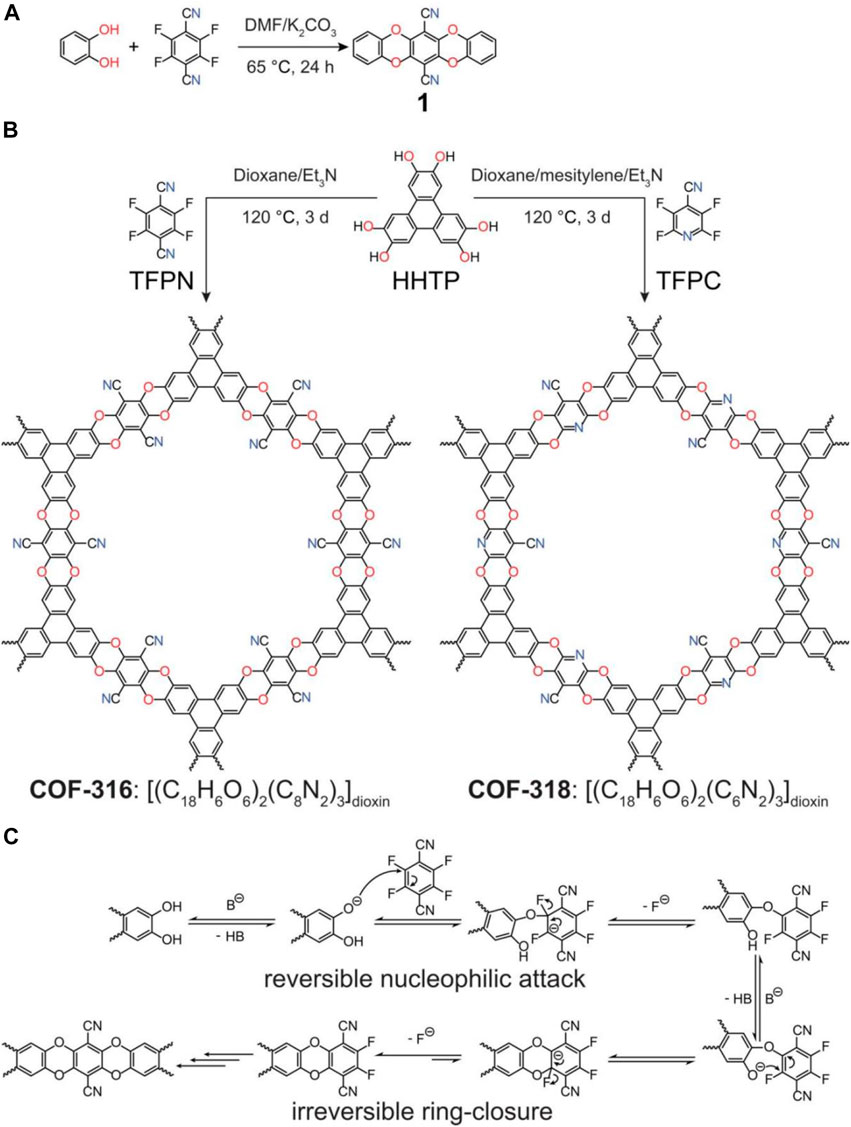
FIGURE 5. (A) Synthesis of molecular analogs (B) of COF-316 and -318. (C) Proposed mechanism of dioxin linkage formation.
Both COF-316 and COF-318 are yellow microcrystalline powders that are insoluble in common organic solvents such as acetone, alcohol, dichloromethane, tetrahydrofuran, N, and Nd-dimethylformamide. The surface area of COF-318 was increased after acid treatment. Bing Zhang speculated that the acid reacts with the remaining pyridine-containing oligomer in the pore and slowly alters from the covalent organic frame synthesis. During stability testing, the nitrile on the main chain of COF-316 was partially converted to an amide under strong alkaline conditions (6M sodium hydroxide). The COF reaction range was limited to 21 h under extreme conditions and was used for post-synthetic modification.
In conclusion, Bing Zhang synthesized two crystalline porous COFs with novel dioxin bonds using irreversible nucleophilic aromatic substitution reactions. The resulting dioxin bonds are irreversible at the chemical level, and the resulting COF materials were highly stable, making two new functional groups covalently introduced into COFs by post-synthetic modification under extreme conditions. The new path has been designed and built to enhance performance.
4.3 Successive reversible and irreversible chemical reactions
COFs are crystalline porous materials connected by dynamic covalent bonds. Dynamic chemistry enables the initial amorphous network to be transformed into a porous and crystalline COF. Although dynamic chemistry has been used to achieve the conversion between diverse types of COFs, including their conversion from 2D to 3D and the insertion of disparate connection types, the conversion of linear polymers to COFs has not been reported. Here, this study demonstrated a method for converting linear immune-linked polymers into keto-linked COFs by replacing the joint strategy (Zhu et al., 2021). The irreversible tautomer generates ketone bonds via a dynamic chemical reaction that replaces the linker in the linear polymer. The possible time-dependent transition of linear polymers to a COF is analyzed to understand the transition and substitution mechanism. This work demonstrated an alternative route for the preparation of covalent organic frames, providing a potential approach for its synthesis by sequential reversible and irreversible chemistry and solution treatment of the linear polymer conversion into the desired covalent organic frame structure. By selecting the appropriate monomer, the structure, porosity, and topology could be precisely controlled to achieve control of the monomer properties. After the synthesis of covalent organic polymers or COFs, linkers are substituted to produce new crystalline COFs. For example, Zhao and colleagues developed a connectome replacement method to produce COFs with three different kinds of pores (Qian et al., 2017). Subsequently, they reported a linker substitution strategy to achieve the conversion of imide COF to imide COFs and the imide COF conversion to imide COFs. Linker substitution has been shown to produce COFs with ameliorated crystallinity and hierarchical structure. Dichtel and colleagues significantly improved the crystallinity of β-ketamine-linked COFs through the monomer exchange of feminine-linked COFs (Zhang G. et al., 2018). Recently, Han and colleagues achieved structural conversion between large-hole 3D-COF and small-hole 3D-COF, as well as dimensional conversion from 3D-COF to 2D-COF through synthetic connectome exchange (Li et al., 2019). Yan and colleagues also used the linker exchange strategy to synthesize two amino-functionalized imino-COFs that were unavailable through alternative synthetic pathways. These studies demonstrate the value of joint exchange strategies in synthesizing new COFs and providing alternative synthetic routes for producing target COFs. The combination of reversible and irreversible chemistry has recently been used to construct ultra-stable and functional COFs. Banerjee and colleagues reported a series of ultra-stable COFs, first producing imine COFs with enol functional groups and then tautomerism to form ketones, resulting in COFs with excellent stability of both acids and bases. The first reversible reaction step achieves error correction and crystallization, and irreversible tautomerism converts the enol form into the keto form of the more stable COF. In the second stage, Banerjee and colleagues further produced a series of beta-ketamine COFs using an organic terra-clay process and synthesized porous films of this type of COF through self-assembly of COF nanospheres (Karak et al., 2017). In this study, a novel strategy for converting linear imino polymers into two-dimensional COFs through sequential reversible and irreversible chemical reactions was developed. A linear imino-linked polymer (LP-1 and LP-2, Figure 6) was first synthesized. A connector replacement reaction was performed based on the synthesis of the linear polymer using 2,4,6-trimethylchlorophenol (TPG), assuming that the junction group will be replaced on the linear polymer, and the resultant COF frame material generated a stable ketone group (Figure 6). It was also found that the transformed COFs had higher crystallinity and surface area than COFs directly synthesized by small molecule reagents. In this paper, it is demonstrated that the successful conversion of linear polymers into a COF provides one-dimensional linear polymer flooding with new solution treatments that can then be converted into a crystalline COF.
In summary, this study demonstrated the sequential reversible and irreversible chemical transformation of 1D linear polymers to 2D COFs. The strategy was to replace the linker with monomeric TPG, producing the final covalent organic frame product from TPG with improved crystallinity and greater porosity. The enol-type intermediates rapidly undergo tautomeric changes after displacement, forming a more stable keto bond in the final product. The highlight of their research is the use of fully soluble linear polymers to produce what can be treated in solution and converted into a COF. This work provides insights into the dynamic nature of COFs and provides a potential new approach.
5 Prospects
We have here focused on the synthesis of partial irreversible stable bonds without distinguishing high or low performance. The synthesis of irreversible stable bonds introduced two methods which are post-synthetic modification and direct synthesis. We selected six studies to briefly introduce these two methods for better understanding. Whether discussing a post-synthetic modification or a direct synthetic strategy, these six studies are essential for the construction of irreversible and stable bonds. The field of metal-free organic photocatalysts has grown from the initial titanium dioxide to a variety of composite materials., The synthesis and preparation of efficiently stable catalysts is a major problem for the future of COF photocatalysts. COF materials, as the most promising porous materials in the area of photocatalysis, not only have large porosity and specific surface area but can also be combined with other materials. There are thus infinite possibilities for COF matrix composites, such as metal matrix composites and ceramic matrix composites. However, the COF matrix composite is also like the COF material itself, forming new bonds through bonds between organic molecules; we expect that the formed bonds cannot be easily destroyed. Therefore, the stability of the material is crucial to the synthesis of COF-based materials, which represents the production capacity and efficiency of the catalyst synthesized in mass production processing and also affects actual production applications. Energy is a future development problem; how to use the existing resources to create a higher value of energy is the direction and goal. With abundant solar energy resources, it is feasible to use photocatalysts to solve some of China’s energy problems. As a new force for solving China’s future energy shortage, photocatalytic materials must solve the problems of semiconductor catalyst materials. For example, the route of photocatalytic H2O2 production depends on the type of photocatalyst and reaction conditions, which mainly comprises three pathways: one-step two-electron ORR, two-step single-electron ORR, and two-channel pathway. In the process of H2O2 production, the yield is related to the selectivity of the catalyst. The low efficiency of H2O2 production by photocatalysis is mainly caused by the small range of sunlight absorption of the photocatalyst, the easy recombination of electron-hole pairs, many side reactions, and the poor selectivity of H2O2. The most vital factor affecting photocatalyst yield which causes the low selectivity of hydrogen peroxide is the location of the catalyst’s energy band. In the six studies we selected, the authors modified some of the previous catalysts or synthesized new catalysts. The reversible bond energy of the original catalyst has been improved so that the problem of low selectivity can be reduced as much as possible during the use of the catalyst. Improving productivity is of great research interest. In the above six studies, whether it is a post-synthetic modification or direct synthesis, the existing problems and defects of the originally synthesized catalyst are solved from different aspects. The specific surface area can be increased by changing the length of the skeleton, which can improve the performance of the catalyst, or the performance can be changed by inhibiting the recombination of photogenerated carriers and promoting separation efficiency. In practical applications, the reversible unstable bond will have a great impact on the cyclability and stability of the catalyst itself, and the reversible bond will destroy the structure of the catalyst itself in the actual production process, which cannot meet our requirements. To improve stability in irreversible stable bonds, the catalyst may be recycled many times in the actual production process. This will reduce the large cost of the synthesis process, reduce some economic loss, and use energy more efficiency.
Many scholars study whether bond energy is reversible in order to explore the impact of COF material synthesis on the performance of the catalyst and to explore a long-term sustainable field of energy materials. Similarly, COF-based materials will be very important to the field of photocatalytic materials for a long time. At present, COF-based materials have a set of their systems in the field of photocatalytic materials, including synthesis methods (solvothermal, microwave-assisted thermal, ionic synthesis, etc.), synthesis strategies (direct synthesis and post-synthetic modification, etc.,), and applications after synthesis (H2O2 production, H2, CO reduction, etc.). All have their own higher performance characteristics distinct from other materials. Since TiO2 was first discovered in the field of photocatalysts, many researchers have explored resin, g-C3N4, CTF, and a series of catalysts with higher photocatalytic properties in this field. COF-based photocatalysis has ushered in a leap of development, but many scholars also continue to use trial and error in exploring of COF-based materials. There are many factors that affect the production, application, and yield of photocatalysts, such as the stability of bond energy, porosity, and temperature. In the synthesis process, the stability of the bond energy plays a key role, directly affecting the efficiency of the synthesized catalyst in the production application. It is important to determine whether the band position of the catalyst is between the conduction band and the valence band in our practical application; the stability of the catalyst performance is equally important. The development of irreversible bonds is now a trend in the field of COF, and more stable catalysts are also quite favorable for practical industrial applications of catalytic dose generation. The purpose of this review is to provide a basis for COF-based photocatalytic material bond energy stability for the study COF materials. As this material is used as a catalyst in a wide range of applications, stable bonds have a higher yield and better performance for catalyst production applications (As shown in Figure 7). A clearer understanding of more stable irreversible bonds in the field of photocatalysis research will aid the current development trend of COF materials. Irreversible bonds in the field of photocatalytic COF will also promote future research.
Author contributions
FM: writing–original draft. LY: conceptualization, funding acquisition, supervision, and writing–review and editing.
Funding
The author(s) declare that financial support was received for the research, authorship, and/or publication of this article. This work is supported by the Hubei Provincial Natural Science Foundation of China (No. 2022CFA065) and the 111 Project (D20015).
Conflict of interest
The authors declare that the research was conducted in the absence of any commercial or financial relationships that could be construed as a potential conflict of interest.
The author(s) declared that they were an editorial board member of Frontiers at the time of submission. This had no impact on the peer review process and the final decision.
Publisher’s note
All claims expressed in this article are solely those of the authors and do not necessarily represent those of their affiliated organizations, or those of the publisher, the editors, and the reviewers. Any product that may be evaluated in this article, or claim that may be made by its manufacturer, is not guaranteed or endorsed by the publisher.
References
Ascherl, L., Sick, T. J. T., Margraf, J. T., Lapidus, S. H., Calik, M., Hettstedt, C., et al. (2016). Molecular docking sites designed for the generation of highly crystalline covalent organic frameworks. Nat. Chem. 8, 310–316. doi:10.1038/nchem.2444
Colson, J. W., and Dichtel, W. R. (2013). Rationally synthesized two-dimensional polymers. Nat. Chem. 5 (6), 453–465. doi:10.1038/nchem.1628
Cote, A. P., Benin, A. I., Ockwig, N. W., O’Keeffe, M., Matzger, A. J., and Yaghi, O. M. (2005). Porous, crystalline, covalent organic frameworks. Science 310, 1166–1170. doi:10.1126/science.1120411
Daugherty, M. C., Edon, V., Li, R. L., Evans, A. M., Chavez, A. D., and Dichtel, W. R. (2019). Improved synthesis of β-ketoenamine-linked covalent organic frameworks via monomer exchange reactions. Chem. Commun. 00, 1–3. doi:10.1039/x0xx00000x
Ghosh, S., Tsutsui, Y., Kawaguchi, T., Matsuda, W., Nagano, S., Suzuki, K., et al. (2022). Band-like transport of charge carriers in oriented two-dimensional conjugated covalent organic frameworks. Chem. Mat. 34, 736–745. doi:10.1021/acs.chemmater.1c03533
Gong, Y. N., Guan, X., and Jiang, H. L. (2023). Covalent organic frameworks for photocatalysis: synthesis, structural features, fundamentals and performance. Coord. Chem. Rev. 475, 214889. doi:10.1016/j.ccr.2022.214889
Huang, G., Lin, G., Niu, Q., Bi, J., and Wu, L. (2022). Covalent triazine-based frameworks confining cobalt single atoms for photocatalytic CO2 reduction and hydrogen production. J. Mater. Sci. Technol. 116, 41–49. doi:10.1016/j.jmst.2021.11.035
Huang, N., Wang, P., and Jiang, D. (2016). Covalent organic frameworks: a materials platform for structural and functional designs. Nat. Rev. Mat. 1, 16068. doi:10.1038/natrevmats.2016.68
Kandambeth, S., Dey, K., and Banerjee, R. (2019). Covalent organic frameworks: chemistry beyond the structure. J. Am. Chem. Soc. 141, 1807–1822. doi:10.1021/jacs.8b10334
Kandambeth, S., Mallick, A., Lukose, B., Mane, M. V., Heine, T., and Banerjee, R. (2012). Construction of crystalline 2D covalent organic frameworks with remarkable chemical (acid/base) stability via a Combined reversible and irreversible route. J. Am. Chem. Soc. 134 (38), 19524–19527. doi:10.1021/ja308278w
Karak, S., Kandambeth, S., Biswal, B. P., Sasmal, H. S., Kumar, S., Pachfule, P., et al. (2017). Constructing ultraporous covalent organic frameworks in seconds via an organic terracotta process. J. Am. Chem. Soc. 139, 1856–1862. doi:10.1021/jacs.6b08815
Kumar, S., Maivizhikannan, V., Drews, J., and Krishnan, V. (2019). Fabrication of nanoheterostructures of boron-doped ZnO-MoS2 with enhanced photostability and photocatalytic activity for environmental remediation applications. Vacuum 163, 88–98. doi:10.1016/j.vacuum.2019.02.001
Li, X., Zhang, C., Cai, S., Lei, X., Altoe, V., Hong, F., et al. (2018). Facile transformation of imine covalent organic frameworks into ultrastable crystalline porous aromatic frameworks. Nat. Commun. 9, 2998. doi:10.1038/s41467-018-05462-4
Li, Z., Ding, X., Feng, Y., Feng, W., and Han, B. H. (2019). Structural and dimensional transformations between covalent organic frameworks via linker exchange. Macromolecules 52, 1257–1265. doi:10.1021/acs.macromol.8b01814
Lohse, M. S., and Bein, T. (2018). Covalent organic frameworks: structures, synthesis, and applications. Adv. Funct. Mater. 28 (1-71), 1705553. doi:10.1002/adfm.201705553
Nawaz, A., Goudarzi, S., Asghari., M. A., Pichiah, S., Selopal., G., Rosei, F., et al. (2021). Review of hybrid 1D/2D photocatalysts for light-harvesting applications. ACS Appl. Nano Mat. 4, 11323–11352. doi:10.1021/acsanm.1c01014
Peng-Lai, W., San-Yuan, D., Zhi-Cong, Z., Zhi-Peng, W., and Wei, W. (2019). Robust covalent organic frameworks via multicomponent reactions. J. Am. Chem. Soc. 141, 45, 18004. doi:10.1021/jacs.9b10625
Pyles, D. A., Coldren, W. H., Eder, G., Hadad, C. M., and Mcgrier, P. L. (2018). Mechanistic investigations into the cyclization and crystallization of benzobisoxazole-linked two-dimensional covalent organic frameworks. Chem. Sci. 9, 6417–6423. doi:10.1039/C8SC01683F
Qian, C., Qi, Q. Y., Jiang, G. F., Cui, F. Z., Tian, Y., and Zhao, X. (2017). Toward covalent organic frameworks bearing three different kinds of pores: the strategy for construction and COF-to-COF transformation via heterogeneous linker exchange. J. Am. Chem. Soc. 139, 6736–6743. doi:10.1021/jacs.7b02303
Qian, H., Meng, F., Yang, C., and Yan, X. (2020). Irreversible amide-linked covalent organic framework for selective and ultrafast gold recovery. Angew. Chem. Int. Ed. 59, 17607–17613. doi:10.1002/anie.202006535
Rowan, S. J., Cantrill, S. J., Cousins, G. R. L., Sanders, J. K. M., and Stoddart, J. F. (2002). Dynamic covalent chemistry. Angew. Chem. Int. Ed. 41, 898–952. doi:10.1002/1521-3773(20020315)41:6<898::aid-anie898>3.0.co;2-e
Seo, J. M., Noh, H. J., Hu, Y. J., and Baek, J. B. (2019). Converting unstable imine-linked network into stable aromatic benzoxazole-linked one via post-oxidative cyclization. J. Am. Chem. Soc. 141 (30), 1–6. doi:10.1021/jacs.9b05244
Sick, T. A. G., Hufnagel, A. G., Kampmann, J., Kondofersky, I., Calik, M., Rotter, J. M., et al. (2018). Oriented films of conjugated 2D covalent organic frameworks as photocathodes for water splitting. J. Am. Chem. Soc. 140, 2085–2092. doi:10.1021/jacs.7b06081
Smith, B. J., Overholts, A. C., Hwang, N., and Dichtel, W. R. (2016). Insight into the crystallization of amorphous imine-linked polymer networks to 2D covalent organic frameworks. Chem. Commun. 52, 3690–3693. doi:10.1039/c5cc10221a
So, Y. H., Heeschen, J. P., Bell, B., Bonk, P., Briggs, M., and DeCaire, R. (1998). Study of the mechanism for poly(p-phenylene)benzoxazole polymerizationa remarkable reaction pathway to make rigid-rod polymers. Macromolecules 31 (16), 5229–5239. doi:10.1021/ma980467i
Stewart, D., Antypov, D., Dyer, M. S., Pitcher, M. J., Katsoulidis, A. P., Chater, P. A., et al. (2017). Stable and ordered amide frameworks synthesised under reversible conditions which facilitate error checking. Nat. Commun. 8, 1102. doi:10.1038/s41467-017-01423-5
Suematsu, K., Nakamura, K., and Takeda, J. (1983). Polyimine, a C=N double bond containing polymers: synthesis and properties. Polym. J. 15, 71–79. doi:10.1295/polymj.15.71
Tang, D., Shao, C., Jiang, S., Sun, C., and Song, S. (2021). Graphitic C2N3: an allotrope of g-C3N4 containing active azide pentagons as metal-free photocatalyst for abundant H2 bubble evolution. Acs. Nano. 15 (4), 7208–7215. doi:10.1021/acsnano.1c00477
Uribe-Romo, F. J., Hunt, J. R., Furukawa, H., Klock, C., O’Keeffe, M., and Yaghi, O. M. (2009). A crystalline imine-linked 3-D porous covalent organic framework. J. Am. Chem. Soc. 131, 4570–4571. doi:10.1021/ja8096256
Wang, J., Li, J., Gao, M., and Zhang, X. (2017a). Self-assembling covalent organic frameworks functionalized magnetic graphene hydrophilic biocomposite as an ultrasensitive matrix for N-linked glycopeptide recognition. J. Name 00, 1–3. doi:10.1039/c7nr02932b
Wang, K., Yang, L., Wang, X., Guo, L., Cheng, G., Zhang, C., et al. (2017b). Covalent triazine frameworks via a low temperature polycondensation approach. Angew. Chem. Int. Ed. 56, 14149–14153. doi:10.1002/anie.201708548
Xin-Le, L., Song-Liang, C., Bing, S., Chong-Qing, Y., Jian, Z., and Yi, L. (2020). Chemically robust covalent organic frameworks: progress and perspective. Matter 3 (5), 2590–2393. doi:10.1038/s41467-018-05462-4
Xu, C., Ravi Anusuyadevi, P., Aymonier, C., Luque, R., and Marre, S. (2019). Nanostructured materials for photocatalysis. Chem. Soc. Rev. 48 (14), 3868–3902. doi:10.1039/c9cs00102f
Yan, H., Wang, X., Yao, M., and Yao, X. (2013). Band structure design of semiconductors for enhanced photocatalytic activity: the case of TiO2. Prog. Nat. Sci. Mater. Int. 23 (4), 402–407. doi:10.1016/j.pnsc.2013.06.002
Zhai, Y., Liu, G., Jin, F., Zhang, Y., Gong, X., Miao, Z., et al. (2019a). Construction of covalent-organic frameworks (COFs) from amorphous covalent organic polymers via linkage replacement. Angew. Chem. Int. Ed. 58, 17679–17683. doi:10.1002/anie.201911231
Zhai, Y., Liu, G., Jin, F., Zhang, Y., Gong, X., Miao, Z., et al. (2019b). Construction of covalent-organic frameworks (COFs) from amorphous covalent organic polymers via linkage replacement. Angew. Chem. 131 (49), 17843–17847. doi:10.1002/ange.201911231
Zhang, B., Wei, M., Mao, H., Pei, X., Alshmimri, S. A., Reimer., J. A., et al. (2018a). Crystalline dioxin-linked covalent organic frameworks from irreversible reactions. J. Am. Chem. Soc. 140, 12715–12719. doi:10.1021/jacs.8b08374
Zhang, G., Tsujimoto, M., Packwood, D., Duong, N. T., Nishiyama, Y., Kadota, K., et al. (2018b). Construction of a hierarchical architecture of covalent organic frameworks via a postsynthetic approach. J. Am. Chem. Soc. 140 (7), 2602–2609. doi:10.1021/jacs.7b12350
Zhang, L., Peng, Z., Wen, Q., Li, X., Lu, P., Wang, Y., et al. (2016). Copper-catalyzed preparation of 2-Aryl-3-cyanobenzofurans with bright blue photoluminescence. Org. Lett. 18, 728–731. doi:10.1021/acs.orglett.5b03704
Zhao, Z., Zheng, Y., Wang, C., Zhang, S., Song, J., Li, Y., et al. (2021). Fabrication of robust covalent organic frameworks for enhanced visible-light -driven H2 evolution. ACS Catal. 11 (4), 2098–2107. doi:10.1021/acscatal.0c04820
Keywords: covalent organic frameworks, irreversible bond, photocatalysis, stability, activity
Citation: Ma F and Ye L (2024) Irreversible bonds for higher stability of COF materials in photocatalytic reactions. Front. Fuels. 1:1320326. doi: 10.3389/ffuel.2023.1320326
Received: 12 October 2023; Accepted: 20 December 2023;
Published: 05 February 2024.
Edited by:
Xin Ying Kong, Nanyang Technological University, SingaporeReviewed by:
Boon-Junn Ng, Xiamen University, MalaysiaXing’An Dong, Chongqing Normal University, China
Copyright © 2024 Ma and Ye. This is an open-access article distributed under the terms of the Creative Commons Attribution License (CC BY). The use, distribution or reproduction in other forums is permitted, provided the original author(s) and the copyright owner(s) are credited and that the original publication in this journal is cited, in accordance with accepted academic practice. No use, distribution or reproduction is permitted which does not comply with these terms.
*Correspondence: Liqun Ye, lqye@ctgu.edu.cn