- U.S. Geological Survey, Mississippi Cooperative Fish and Wildlife Research Unit, Mississippi State, MS, United States
Life-history traits of a species have been postulated as a factor in abundance and occupancy patterns. Understanding how traits contribute to the ubiquity and rarity of taxa can facilitate the development of effective conservation policy by establishing a connection between species requirements and resource. The goal was to evaluate fish assemblages in artificial lakes for evidence of the abundance-occupancy patterns reported in natural environments and, if evident, to explore if observed patterns of abundance and occupancy could be attributed to species traits. Fish abundance and occupancy were estimated over 1990–2018 in 22 artificial lakes impounded within the Tennessee River basin, USA. Consistent with reports for many other taxonomic groups in natural environments, there was a positive association amidst 114 fish species between abundance and occupancy in artificial lakes (R2 = 0.78). This result indicates that the fish assemblages that develop in these anthropized environments follow the fundamental abundance-occupancy patterns uncovered in natural environments, despite assemblages having been disfigured by the dramatic rearrangement of habitats brought by impoundment. Moreover, a redundancy analysis focusing mostly on reproductive and habitat traits adequately predicted abundance-occupancy patterns of fish assemblages in artificial lakes (R2 = 0.69). Species abundance-occupancy is influenced by the interplay between life-history traits and habitat availability, even in artificial lakes, and by extension, possibly other artificial ecosystems.
Introduction
Numerous taxa, including plants, spiders, insects, amphibians, fish, birds, and mammals, have been found to exhibit positive correlations between species abundance and the number of sites they occupy (Hanski et al., 1993; Brown, 1995; Gaston and Blackburn, 2000; Gaston et al., 2000; Ten Caten et al., 2022). This correlation suggests that widespread species are also apt to be more abundant at a specific location. Consequently, factors influencing the distribution of a species within a particular region may also influence the abundance of that species at a specific location within the region. Although this abundance-occupancy pattern appears to be widespread among plants and animals, the mechanisms underlying the pattern are not clear (Gaston et al., 1997; McGeoch and Gaston, 2002; Verberk et al., 2010).
Abundance-occupancy relationships have relevant implications to conservation, harvesting, invasions, and biodiversity monitoring, as reviewed by Gaston et al. (2000). Nevertheless, with few exceptions, the abundance-occupancy connections of freshwater fish are poorly studied. The subject is so infrequent in the literature on fish that it has not been included in compilation volumes on freshwater fish (e.g., Gido and Jackson, 2010; Ross, 2015; Matthews and Marsh-Matthews, 2017). Possible causes for this dearth of literature include an absence of sufficient data sets or an inability to prioritize the topic among freshwater ecologists. According to a recent review by Xia et al. (2022), only 11 investigations had considered the abundance-occupancy relationships of freshwater fish, all in streams and natural lakes of Europe and the Americas, with eight studies revealing a positive trend and three finding none. Considering these low numbers, in comparison to the hundreds to terrestrial studies, it is inconclusive if the principles of abundance-occupancy defined for terrestrial species (Gaston, 1996; Lawton, 1999) also apply to freshwater fish, and even less is known about whether these principles apply to anthropized hydrosystems. Given the relevancy of abundance-occupancy patterns, and the various threats fresh waters face (Dudgeon et al., 2006; Tickner et al., 2020), an improved understanding of abundance-occupancy patterns can help conservation efforts.
The fish assemblages that develop in artificial lakes created by impounding a stream are riverine in origin (Fernando and Holčík, 1982). These assemblages are distinct and the result of a restructuring of riverine communities by local extinction of some components of the riverine fish community and by drastic revisions in the abundance of most species retained (Anderson et al., 1995; Agostinho et al., 1999). Only the organisms that have adapted to the manmade environments will remain. Species with high plasticity in feeding, reproductive adaptations, habitat use, and in particular open-water planktivores and detritivores are likely to dominate (Gomes and Miranda, 2001).
In the Tennessee River, USA, the construction of artificial lakes has had a profound effect on the natural habitats of the river's native fish. Large uniform swaths of lentic open water stand out most, as do the extensive littoral and nearshore zones that border upland environments rather than natural floodplains of diverse vastness once flanking the river. Underneath these waters, substrates have been transformed from mostly heterogeneous particles of varying sizes to mostly homogeneous stagnant sediment. Only the upper reaches of lakes and confluent tributaries retain riverine attributes (Miranda et al., 2014; Smith et al., 2023). Several characteristics of water quality, starting with temperature and dissolved oxygen levels, have changed because of the seasonal stratification of water layers brought on by the increases in depth (Thornton et al., 1991). Although organic substrates like terrestrial or aquatic plants and woody debris persist nearshore, the substrates in littoral zones typically originate from flooded uplands and are not of river origin. Fishes that are ubiquitous across broad sections of eastern North America tend to be abundant and have flourished in these expanded anthropized hydrosystems, whereas fishes that are uncommon are symbols of the vanished environments.
Approximately 130 of the 259 fish species that have been identified in the basin have also been found in artificial lakes of the Tennessee River (Miranda et al., 2021a; Southeastern Fishes Council, 2021). This filtered group of species has varying degrees of lacustrine habitat suitability, but none are exclusively lacustrine, and many require habitats provided by tributaries to complete their life cycle, or at least their abundance in the artificial lake is enhanced by access to riverine habitats (Dos Santos et al., 2017; da Silva et al., 2019). Filtering and proliferation of selected species in artificial lakes generally has led to homogenized fish assemblages (Petesse and Petrere, 2012), which may indicate shifts in abundance-occupancy relationships.
Species traits have been proposed as a driver of abundance and occupancy patterns (Heino and Tolonen, 2018). Traits determine the range of environmental conditions in which a species is most likely to occur, and abundances at those locations are expected to be greatest when the set of traits best matches environmental conditions. Understanding how various sets of functional traits contribute to the ubiquity, restriction, or rarity of fishes facilitates the development of effective conservation policy by establishing a link between species needs and resource availability (McGill et al., 2006; Hurlbert and White, 2007).
Changes in abundance-occupancy patterns due to habitat disturbances caused by human activity have been studied infrequently, and never in freshwater environments. In artificial marine substrates vertebrate and invertebrate occupants increased the abundance of high occupancy species while decreased the abundance of low occupancy species, changing the slope of the abundance-occupancy relationship and undermining biodiversity (Sanabria-Fernandez et al., 2018). In British birds, habitat modifications decoupled abundance-occupancy as suggested by an increased scatter that decreased strength in abundance-occupancy relationships (Webb et al., 2007). Conversely, in arthropod communities in the Azores, there was no meaningful relationship between deviation from the abundance-occupancy pattern and habitat disturbances (Rigal et al., 2013). Research results are conflicting about whether the abundance-occupancy relationship is affected by human activity. Habitat disturbances may simply reconfigure species assemblages without necessarily altering abundance-occupancy patterns, or they may effectively alter patterns. To address these questions, I examined the fish assemblages of artificial lakes to test if positive abundance-occupancy relationships hold true even in these highly altered, pervasive environments. If so, I investigated whether the observed patterns of species abundance and occupancy could be anticipated based on traits expected to thrive in the habitats that proliferate in artificial lakes.
Methods
Abundance-occupancy descriptions of fish assemblages in artificial lakes in the Tennessee River basin were used in this study. This river is one of the most diverse basins in North America (Miranda et al., 2021b). The basin begins in the Appalachian Mountains and flows westward for 1,500 km. The river drains 106,000 km2 and discharges an average of 1,926 m3s−1 into the Ohio River at its confluence. In the Tennessee River basin, there are 44 manmade lakes that are bigger than 100 hectares; 22 of the larger lakes have been more closely monitored due to their significance and are included in this analysis (Figure 1). The sample lakes range in elevation from 110 m above mean sea level at Kentucky Lake to 585 m at Chatuge Lake in the Hiwassee River and 597 m at Watauga Lake in the Holston River.
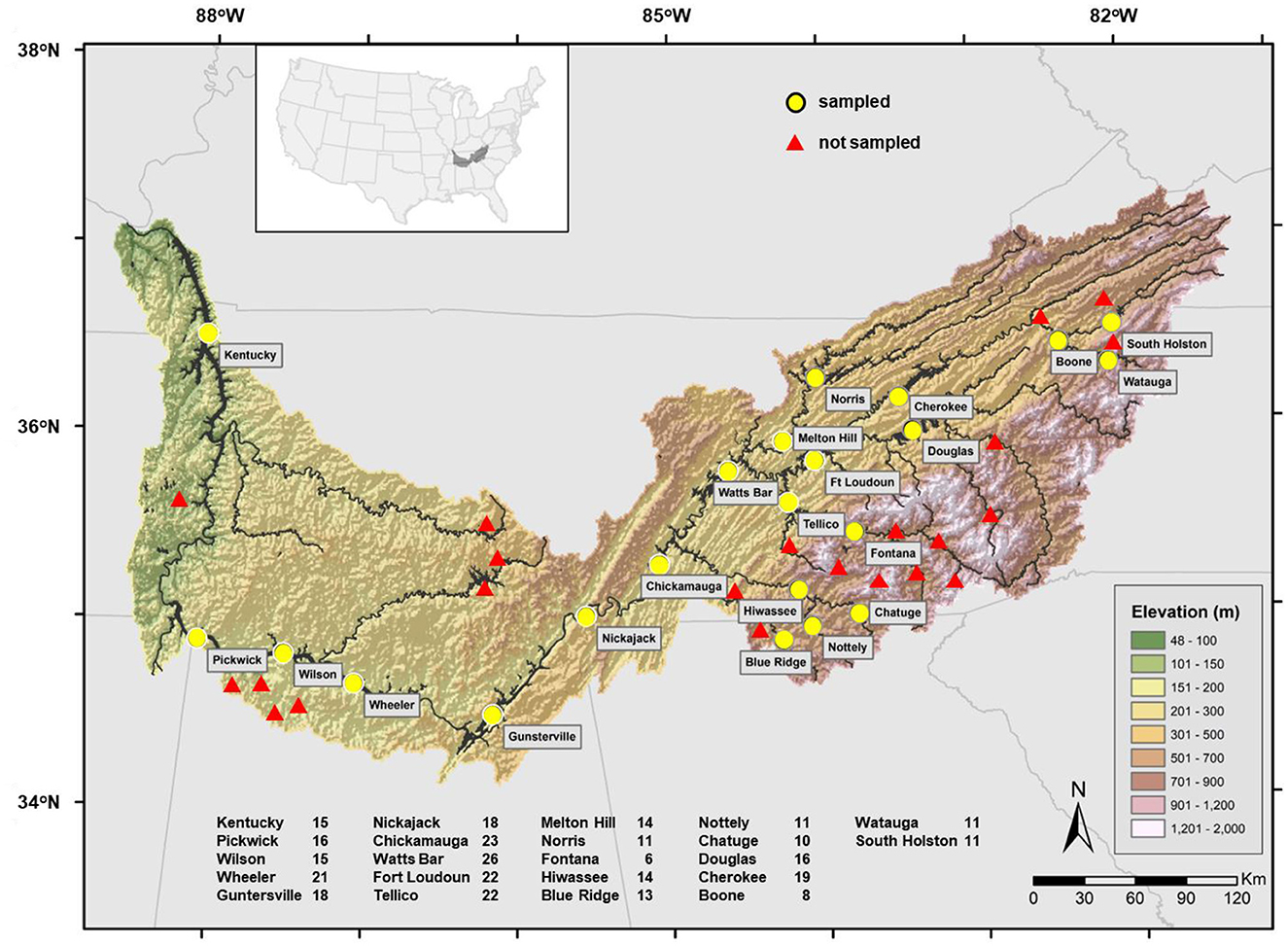
Figure 1. The Tennessee River basin in the United States showing the distribution of 44 artificial lakes impounded on the mainstem and tributaries. Only lakes sampled are named. The numbers next to each lake on the lower end represent the total number of years each lake was sampled in 1990–2018.
Fish assemblages were evaluated through boat electrofishing by the Tennessee Valley Authority during surveys conducted in fall, 1990-2018. Electrofishing was performed using a system comparable to that described by Miranda and Boxrucker (2009) for standardized electrofishing in standing waters (60 Hz pulsed DC; 8-12 peak A depending on conductivity). Fish were collected within transects dispersed throughout each lake and randomly selected without replacement. In total, 10-90 (median 30) samples (each averaging 750 s in time and spanning about 300-m in length at a depth <3 m) were collected per lake per year, but not all lakes were sampled every year (Figure 1). Every fish collected was identified and counted before release or disposal. Reportedly, electrofishing adequately reflects the largescale differences in fish assemblages expected over the Tennessee River basin (Miranda et al., 2021a).
Abundance and occupancy were estimated for each species across the 22 artificial lakes. Abundance was indexed as catch per hour of electrofishing, calculated as the total catch for each species divided by the total electrofishing hours, with totals pooled over years, over lakes, but including only lakes in which the species was detected. Because zero values contribute more and more to the mean catch per hour as occupancy decreases, this computation of mean based on detections-only lakes avoids artifactually positive correlations between abundance and occupancy, and explicitly addresses the hypothesis that site abundance and site occupancy are coupled (Gaston, 1996). A species' occupancy was calculated as the percentage of the 22 lakes where it was found, considering all available years pooled to maximize number of samples and, by extension, species detectability. Pooling of all available samples over all sampling years sacrificed annual variation in favor of a more robust estimate of occupancy. Following Gaston (1994) the distribution of species occupancies was informally classified according to quartiles: widespread (occupying more than 75% of the 22 lakes), restricted (occupying 25–75% of the lakes), and rare (occupying less than 25% of the lakes). This classification was used solely for the purpose of summarizing results; it was not incorporated into any analysis.
Simple least-squares regression analysis was applied to test if fish species followed the abundance-occupancy patterns previously reported in natural environments. Catch per hour for each of the species detected in the 22 lakes combined (dependent variable; loge transformed) was regressed on the proportion of the 22 lakes occupied by the species (predictor variable). Body size and local population density has generally been found to be negatively related among animal populations (Brown, 1995). Therefore, maximum total length of each species (Frimpong and Angermeier, 2009) was included as a covariate to remove effects fish size may have on abundance-occupancy patterns.
Species traits were retrieved from the FishTraits database assembled by Frimpong and Angermeier (2009). The database includes 58 binary (0/1) variables representing three general types of traits: feeding, reproductive, and habitat traits. Feeding traits included eight strategies ranging from algae to detritus to fish. The 25 reproductive features ranged from minimal parental care (open-substratum broadcast spawning) to hiding eggs on certain substrates (brood hiders), building nests and watching over young (substrate choosers and nest guarders), and bearing live offspring (bearers). Habitat traits included 25 strategies that can be separated into substrates (e.g., sand, gravel, woody debris; total of 10 traits) and macrohabitats (e.g., lacustrine, small rivers, lowlands; total of 15 traits). Due to missing data, descriptions from FishBase (Froese and Pauly, 2010) were used to fill in the reproductive features for the slabrock darter Etheostoma smithi and saddleback darter Percina vigil. Silver carp Hypophthalmichthys molitrix, an invasive species was excluded from all analyses because, toward the end of the sampling period, it had just recently started to colonize the Tennessee River basin and had neither saturated their environment nor spread beyond a few lakes.
Multivariate analyses were used to evaluate the association between abundance-occupancy and various metrics defining the trophic, reproductive, and habitat traits of fishes. Distance-based redundancy analysis (dbRDA; Legendre and Anderson, 1999) assessed the relationship between the dependent matrix consisting of abundance and occupancy for each of the N fish species detected in the 22 reservoirs, and the independent matrix consisting of the 58 binary fish traits for each of the N species. The dbRDA is equivalent to multiple regression, as it models the relation of the explanatory variables (i.e., traits) to a similarity matrix constructed with the set of dependent variables (i.e., abundance and occupancy). The similarity matrix representing the dependent variable was constructed with a Euclidean distance after log-transforming catch per effort to reduce the skew and rescaling to zero mean and unit variance. The dbRDA retained variables using a stepwise selection procedure that added or removed predictive traits to the model on the basis of the Akaike's information criterion. The selection stopped when no improvements on the selection criterion could be made by either adding or deleting a trait (Anderson et al., 2008, pp 134–135). The R2 statistic was computed to interpret the explanatory power of the traits included in the dbRDA. The F-statistic was computed as an overall test of the dbRDA by comparing the resulting model to a null model. The F test is based on the null hypothesis that the observed strength of the relationship is not larger than the value that would be obtained if the set of dependent and independent variables were unrelated. The distLM procedure in PRIMER-E software, v7 (Clarke and Gorley, 2015) was applied to conduct the dbRDA and model testing.
Results
Overall, 9,624 boat electrofishing samples were collected in the 22 artificial lakes over the course of the study. A mean of 15 years (range 6–26 years) was sampled in each lake. The average number of boat electrofishing transects per lake was 437 (range, 45–1,472 transects). The average number of boat electrofishing transects per lake square kilometer was 7.4 (range 1.4–21.0). While larger lakes received greater sampling effort, lake surface area was directly linked to the amount of effort assigned (Spearman rank correlation, r = 0.85). This sampling effort produced 1.33 million fish representing 115 species including one regularly stocked hybrid (striped bass, Morone saxatilis X white bass, M. chrysops).
Abundance increased with occupancy (Figure 2). The linear regression model fit to the loge abundance—occupancy plot, with the interaction of loge of maximum total length and loge abundance as a covariate, produced an R2 of 0.78, suggesting a robust pattern and statistically significant relationship (F = 188.5; df = 112; P < 0.01). The significant positive occupancy term (t = 9.0, P < 0.01) indicated abundance increased with occupancy (Table 1). The significant negative interaction term (t = −4.1, P < 0.01) indicated that while abundance increased with occupancy, it increased more quickly for smaller species. In all, 20 species were considered widespread, 43 restricted, and 51 rare. Five species were collected in all 22 lakes, and 25 species were identified only in one lake. These results suggest that abundance increased with occupancy, and vice versa, but the rate of increase depended on species size. Furthermore, widespread species were in the minority, and most species were either rare or restricted in distribution.
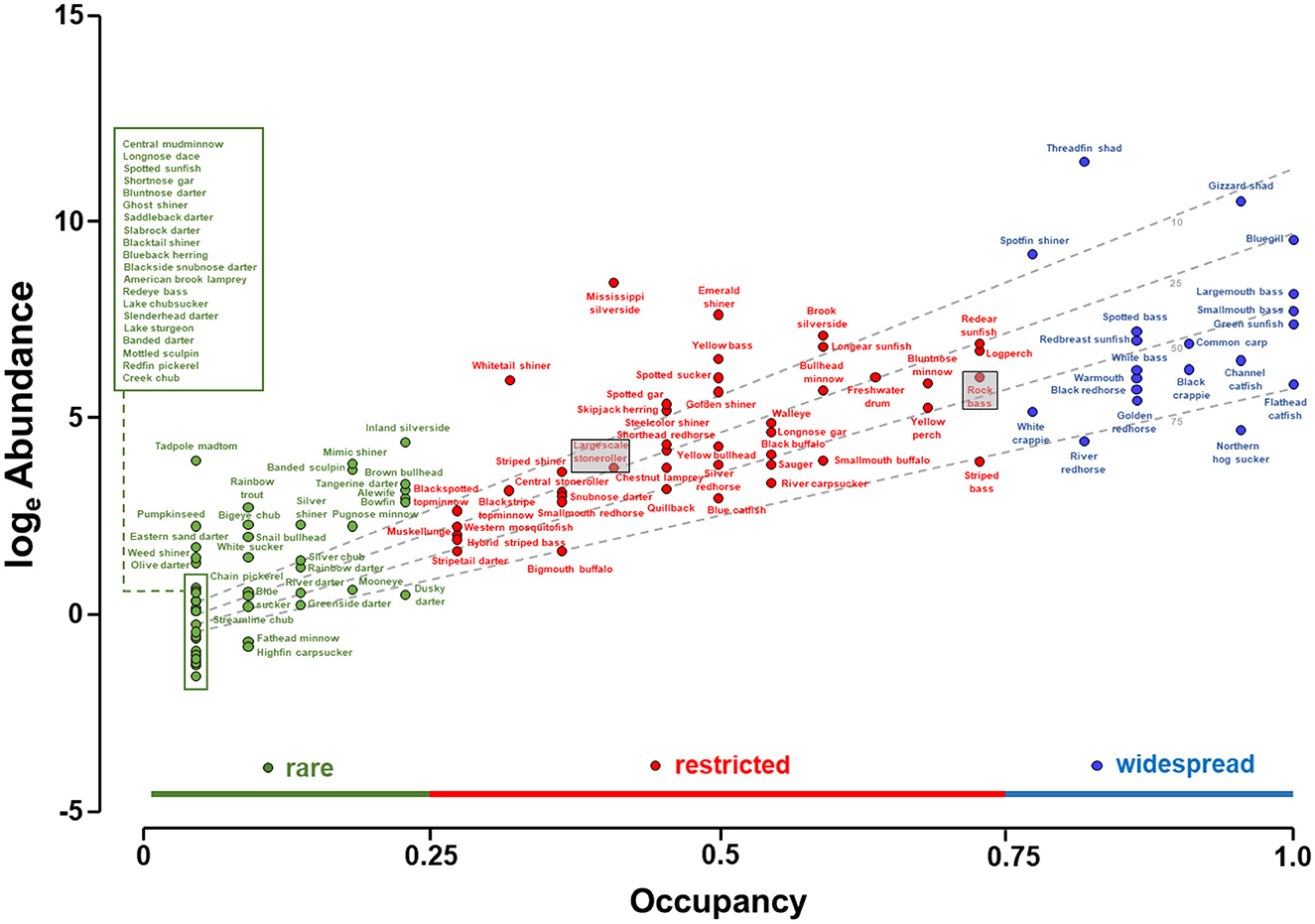
Figure 2. Abundance-occupancy relationship among fish species in artificial lakes of the Tennessee River basin. Abundance was indexed as catch per hour of electrofishing, calculated as the total catch for each species divided by the total electrofishing hours, with totals pooled over years, over lakes, but including only lakes in which the species was detected. Occupancy is the proportion of the 22 lakes in which the species was found considering all available years. The distribution of species occupancies was informally divided into three groups: widespread (occupying more than 0.75 of the 22 lakes), restricted (0.25–0.75), and rare (<0.25). The dashed lines identify the predicted abundance for species with 10 (top dashed line), 25, 50, and 75 (bottom dashed line) cm total length. Boxes are used to denote the placements of the largescale stoneroller and rock bass in relation to their placement in Figure 3B.

Table 1. Least-squares regression model applied to test if fish species in artificial lakes followed a positive abundance-occupancy pattern.
Abundance and occupancy of the species were predicted by species traits (Figure 3). The vectors represent the extent and direction of correlations between abundance and occupancy relative to the two axes. Axis 1 (dbRDA1) separated species mostly by occupancy whereas axis 2 (dbRDA2) separated them by abundance. The smaller than 90-degree angle between the occupancy and abundance vectors reflects the correlation between these variables illustrated in Figure 2. Axis 1, mostly correlated with occupancy, was the most influential axis accounting for 94.2% of the variability explained by the traits, and 64.3% of the total variation. Overall, both axes together accounted for 69% of the total variability in abundance and occupancy accounted by the traits and the model was statistically significant (F = 8.9; df = 28, 112; P < 0.01). Although the traits predicted abundance and occupancy reasonably well, the observed sequence in Figure 2 did not precisely correspond to the predicted sequence in Figure 3. These discrepancies may be the result of prediction error. Alternatively, they may reflect the possibility that some species in anthropized hydrosystems are over- or under-performing in terms of abundance and occupancy in relation to their trait capacity.
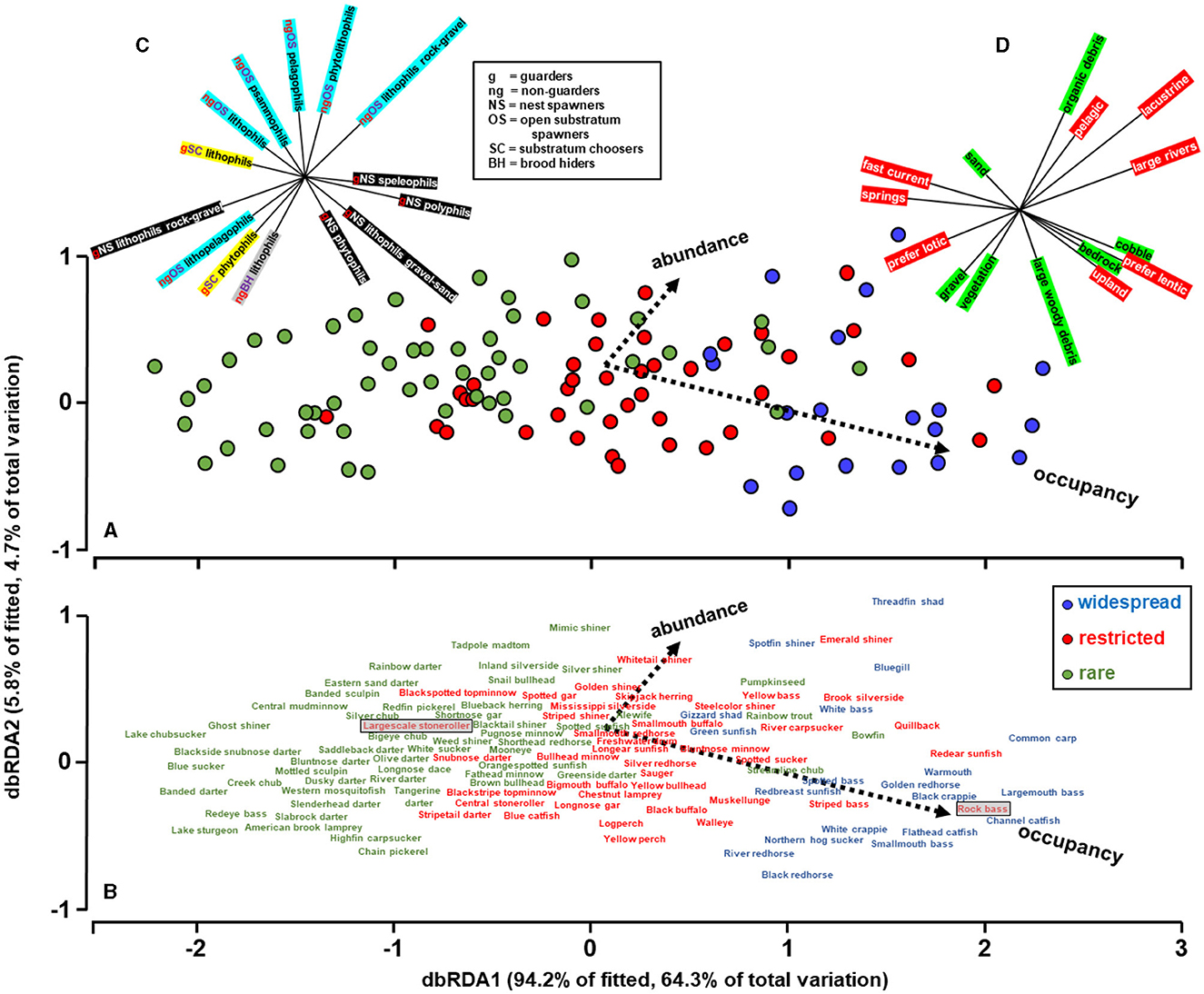
Figure 3. Predictions of species abundance and occupancy distance based on stepwise distance-based redundancy analysis between the two dependent variables (i.e., abundance and occupancy), and multiple explanatory variables (i.e., fish traits). (A, B) are equivalent; (B) provides the common names of the species represented by the circles in (A). Color coding of species represents the widespread, restricted, and rare groups identified in Figure 2. The x-axis reflects principally occupancy and most of the variability, and the y-axis principally abundance. (C, D) identify the reproductive and habitat traits, respectively, retained by the stepwise selection procedure and the vectors identify their relative correlation with the x and y axes. In (C), color coding helps visually separate guarders nest spawners (black), from guarders substratum choosers (yellow), and non-guarders open-substrate spawners (light blue). (D), color coding helps separate substrate choices (green) from macrohabitat choices (red). The two shaded boxes in (B) are used to denote the placements of the largescale stoneroller and rock bass in relation to their placement in Figure 2.
The stepwise selection retained none of the feeding traits, 14 of the reproductive traits, and 15 of the habitat traits. The vectors associated with the reproductive traits (Figure 3C) suggest that nest-spawning guarder species have the highest occupancies and are widespread in the artificial lakes, non-guarders open-substratum species tend to have the highest abundances, and rare and restricted species tend to require specialized substrates predominantly of lithic qualities. The vectors associated with habitat traits (Figure 3D) suggest that species that are rare in artificial lakes are primarily associated with flowing water environments or specialized environments such as sand, gravel, and vegetation that are scarce within most artificial lakes but accessible in incoming tributaries. Widespread species, on the other hand, are predominantly lacustrine and pelagic, preferring lentic habitats with large and small organic detritus and the rocky outcrops that characterize the Tennessee River.
Discussion
This study examined previously unexplored relationships between the ecology of fish species and the anthropized hydrosystems they inhabit. Consistent with reports for many other taxa (reviewed by Gaston and Blackburn, 2000; Gaston et al., 2000), there was a positive correlation between abundance and occupancy. Amidst 114 fish species, the invasive silver carp excluded, the association was strong, with an R2 value of 0.78. These findings are in line with those of other studies in natural freshwater environments (Tales et al., 2004; Faulks et al., 2015; Miranda and Killgore, 2019; Xia et al., 2022). Furthermore, the slope of the abundance-occupancy relationship decreased with body size. This inverse relation between size and abundance suggests that large-bodied fish live at lower abundances than small-bodied ones, a pattern that has been reported in various other animal populations (Blackburn et al., 1993). Therefore, the abundance-occupancy relations that develop in fish assemblages occupying artificial lakes seem to follow the fundamental patterns uncovered for various terrestrial and aquatic species, despite assemblages having been disfigured by the rearrangement of habitats brought on by impoundment.
The artificial freshwater ecosystems in the Tennessee River have restructured fish assemblages and now reflect the altered aquatic conditions. Less than 5% of the species were detected in all lakes, and <20% were labeled as widespread. In line with the habitats available in these newly formed artificial freshwater environments, the widespread species were usually nest-spawning guarders, but the most abundant widespread species were usually non-guarders, open-substratum, pelagic species. The widespread species include several species of the families Clupeidae and Centrarchidae, which can thrive in both lotic and lentic environments (Taylor et al., 2001). These widespread species, including one introduction (i.e., redbreast sunfish Lepomis auritus) from a neighboring watershed, occupy extensive sections of eastern North America. As such, they may occupy broad niches in terms of breadth of habitat, diet, and environmental tolerance (Slatyer et al., 2013). According to an analysis by Giam and Olden (2018), because of their adaptations to seek refuge in non-glaciated lakes during the last glacial maximum and recolonize northward when glacial sheets receded, fishes of North America that inhabit lacustrine environments have wider range sizes. Conversely, rare and restricted species represented 45% and 37% of all species, respectively. These include many species predominantly of the families Percidae, Cyprinidae, and Catostomidae. Species in these families require specialized habitats, mostly fluid environments with stony, sandy, and vegetated substrates and depend on lotic conditions to reproduce (Etnier and Starnes, 1993). Some of the species classified as restricted are confined to the Tennessee River basin or small sections of eastern North America (e.g., whitetail shiner Cyprinella galactura, snubnose darter Etheostoma simoterum, Mississippi silverside Menidia audens). Correspondingly, many of the species classified as rare have narrowly restricted distributions. Possibly owing to the asymmetrical expansion of a small medley of lacustrine-like habitats, leading to an asymmetrical selection of life-history traits, the widespread species are in the minority, and the rare and restricted species are in the majority.
The differences in abundance and occupancy among species was effectively explained by traits, with traits accounting for 69% of the observed variability. These results show that a species' occupancy, and to a lesser extent abundance, are influenced by the interplay between its life-history traits and the availability of its environment, even in artificial lakes, and by extension, presumably other anthropized hydrosystems. Noteworthy, traits predicted that some species would perform better than observed, while others would perform worse than observed (more below). These disparities may simply reflect collection gear selectivity, imprecision of trait assignment for some species, or possibly that additional traits may be needed to accurately characterize species. I am confident that occupancy was estimated adequately by electrofishing owing to the exhaustive multi-year sampling effort. However, I am less confident in the adequacy of the abundance estimates, for two reasons: first, the abundance of many species is known to fluctuate hugely over years and second, electrofishing tends to favor larger individuals who live in nearshore areas (Dolan and Miranda, 2003; Reynolds and Kolz, 2012). In accordance, the dbRDA model prioritized occupancy over abundance. Alternatively, the disparities may point to the intriguing possibility that some species are in fact performing below or above their trait's potential. For instance, species such as the largescale stoneroller Campostoma oligolepis seemed to outperform its traits, as traits predicted that largescale stoneroller should have a lower occupancy than observed (see position of largescale stoneroller, shaded, in Figure 2 vs. Figure 3). The largescale stoneroller is a popular baitfish and conceivably their occupancy has been expanded by bait-bucket releases. Conversely, rock bass Ambloplites rupestris were predicted by the dbRDA to have higher occupancy than manifested. The rock bass in the Tennessee River is at the southern edge of their geographic range, which may influence their survival. Observed abundance-occupancy patterns and those expected patterns based on traits can lend new insight into fish ecology.
In these artificial lakes the variation in abundance and occupancy was accounted for by mostly two sets of life-history traits: habitat and reproductive. Feeding traits were not retained by the stepwise selection dbRDA. This omission suggests that there was no definitive relationship between feeding patterns and abundance and occupancy. The feeding traits of fishes that dominate these artificial lakes are not diverse. During their life history, over 95% of the fish species feed on some combination of aquatic and terrestrial invertebrates including zooplankton, insects, microcrustaceans, annelids, and mollusks, but also on larval fishes (Frimpong and Angermeier, 2010). Other diets are possible including mostly algae or phytoplankton, macrophytes, detritus, and larger fishes, crayfishes, and alike. Conceivably, the rather homogeneous dietary needs of fishes in these artificial lakes limit the potential of feeding traits to make distinctions in abundance-occupancy relationships.
Conclusions
Starting in the 1920s, and over several decades, the artificial lakes in the Tennessee River basin were built to aid navigation, control floods, and generate electric power. Overall, these changes have reduced the river system's environmental variability and complexity (Peipoch et al., 2015) and transformed its fish assemblages. As the original objectives for building artificial lakes are still essential to society, it is unlikely that the dams will be torn down in the near future to address the more contemporary goal of restoring riverine ecosystems and natural fish assemblages. However, it is reasonable to predict that the structures will eventually cease to serve societal needs and in time be dismantled. In the interim, it is important to sustain rare and restricted species by preserving diverse habitat and reproductive traits. Conservation efforts targeted at preserving rare and restricted species in artificial lakes could aim at prioritizing conservation of novel habitats. Removal of silt from inlets and backwaters, and enhancement of the remaining lithic and flowing habitats could encourage diverse life history traits and provide sustaining conditions for many species. Furthermore, unimpounded or mildly impounded mainstem reaches and tributaries can provide access to the habitats needed to maintain diverse traits. Managing the quality of inflows from catchments, which is made easier by the smaller scale of tributaries; maintaining wood delivery and stream shading; preserving suitable instream habitats and flows; and preserving access to functional floodplains and associated backwaters could be conservation priorities.
Data availability statement
The original contributions presented in the study are included in the article/supplementary material, further inquiries can be directed to the corresponding author.
Ethics statement
Ethical approval was not required for the study involving animals in accordance with the local legislation and institutional requirements because i did not collect the dataset. It was collected by a government agency and made available to me.
Author contributions
LM: Conceptualization, Formal analysis, Methodology, Visualization, Writing—original draft, Writing—review and editing.
Funding
The author(s) declare that no financial support was received for the research, authorship, and/or publication of this article.
Acknowledgments
I thank Kurt Lakin and the Tennessee Valley Authority for facilitating access to their dataset. Jane Rogosch and two reviewers provided constructive reviews. Carolina Nascimento helped draft the figures. The Mississippi Cooperative Fish and Wildlife Research Unit is sponsored by the Mississippi Department of Wildlife, Fisheries, and Parks, Mississippi State University, the U.S. Fish and Wildlife Service, and the U.S. Geological Survey. Any use of trade, firm, or product names is for descriptive purposes only and does not imply endorsement by the U.S. Government. Fish were handled in accordance with American Fisheries Society guidelines for the use of fishes in research https://fisheries.org/policy-media/science-guidelines/guidelines-for-the-use-of-fishes-in-research/.
Conflict of interest
The author declares that the research was conducted in the absence of any commercial or financial relationships that could be construed as a potential conflict of interest.
The author LM declared that he was an editorial board member of Frontiers, at the time of submission. This had no impact on the peer review process and the final decision.
Publisher's note
All claims expressed in this article are solely those of the authors and do not necessarily represent those of their affiliated organizations, or those of the publisher, the editors and the reviewers. Any product that may be evaluated in this article, or claim that may be made by its manufacturer, is not guaranteed or endorsed by the publisher.
References
Agostinho, A. A., Miranda, L. E., Bini, L, M., Gomes, L. C., Thomaz, S. M., and Suzuki, H. I. (1999). “Patterns of colonization in Neotropical reservoirs, and prognoses on aging,” in Theoretical Reservoir Ecology and its Applications, eds Tundisi, J. G., Straškraba, M. (Leiden, The Netherlands: Backhuys Publishers), pp. 227–265.
Anderson, A. A., Hubbs, C., Winemiller, K., and Edwards, R. J. (1995). Texas freshwater fish assemblages following three decades of environmental change. Southwest Nat. 1995, 314–321.
Anderson, M., Gorley, R., and Clarke, K. P. (2008). PERMANOVA+ for PRIMER: Guide to Software and Statistical Methods. Plymouth: PRIMER-E Ltd.
Blackburn, T. M., Brown, V. K., Doube, B. M., Greenwood, J. J. D., Lawton, J. H., and Stork, N. E. (1993). The relationship between abundance and body size in natural animal assemblages. J. Anim. Ecol. 62, 519–528. doi: 10.2307/5201
Clarke, K. R., and Gorley, R. N. (2015). PRIMER v7: User Manual/Tutorial (1st ed.). Plymouth: PRIMER-E Ltd.
da Silva, P. S., Miranda, L. E., Makrakis, S., de Assumpção, L., Pinheiro-Dias, J. H., and Cavicchioli-Makrakis, M. (2019). Tributaries as biodiversity preserves: an ichthyoplankton perspective from the severely impounded Upper Paraná River. Aquat. Conserv. 29, 258–269. doi: 10.1002/aqc.3037
Dolan, C. R., and Miranda, L. E. (2003). Immobilization thresholds of electrofishing relative to fish size. Trans. Am. Fish. Soc. 132, 969–976. doi: 10.1577/T02-055
Dos Santos, N. C. L., de Santana, H. S., Ortega, J. C. G., Días, R. M., Fernandes-Stegmann, L., da Silva-Araújo, I. M., et al. (2017). Environmental filters predict the trait composition of fish communities in reservoir cascades. Hydrobiologia 802, 245–253. doi: 10.1007/s10750-017-3274-4
Dudgeon, D., Arthington, A. H., Gessner, M. O., Kawabata, Z. I., Knowler, D. J., Lévêque, C., et al. (2006). Freshwater biodiversity: importance, threats, status and conservation challenges. Biol. Rev. 81, 163–182. doi: 10.1017/S1464793105006950
Etnier, D. A., and Starnes, W. C. (1993). The Fishes of Tennessee. Knoxville, TN: University of Tennessee Press.
Faulks, L., Svanbäck, R., Ragnarsson-Stabo, H., Eklöv, P., and Östman, Ö. (2015). Intraspecific niche variation drives abundance-occupancy relationships in freshwater fish communities. Am. Nat. 186, 272–283. doi: 10.1086/682004
Fernando, C. H., and Holčík, J. (1982). The nature of fish communities: a factor influencing the fishery potential and yields of tropical lakes and reservoirs. Hydrobiologia 97, 127–140. doi: 10.1007/BF00011966
Frimpong, E. A., and Angermeier, P. L. (2009). Fish traits: a database of ecological and life-history traits of freshwater fishes of the United States. Fisheries 34, 487–495. doi: 10.1577/1548-8446-34.10.487
Frimpong, E. A., and Angermeier, P. L. (2010). Trait-based approaches in the analysis of stream fish communities. Am. Fish Soc. Symp. 73, 109–136.
Froese, R., and Pauly, D. (2010). FishBase. Burlington, MA: World Wide Web electronic publication. Available online at: http://www.fishbase.org, version (accessed 02/2022).
Gaston, K. J. (1996). The multiple forms of the interspecific abundance-distribution relationship. Oikos 76, 11–220. doi: 10.2307/3546192
Gaston, K. J., and Blackburn, T. M. (2000). Pattern and process in macroecology. Oxford: Blackwell Science.
Gaston, K. J., Blackburn, T. M., Greenwood, J. J., Gregory, R. D., Quinn, R. M., and Lawton, J. H. (2000). Abundance–occupancy relationships. J. Appl. Ecol. 37, 39–59. doi: 10.1046/j.1365-2664.2000.00485.x
Gaston, K. J., Blackburn, T. M., and Lawton, J. H. (1997). Interspecific abundance-range size relationships: an appraisal of mechanisms. J. Anim. Ecol. 66, 579–601. doi: 10.2307/5951
Giam, X., and Olden, J. D. (2018). Drivers and interrelationships among multiple dimensions of rarity for freshwater fishes. Ecography 41, 331–344. doi: 10.1111/ecog.02946
Gido, K. B., and Jackson, D. A. (Eds.). (2010). Community Ecology of Stream Fishes: Concepts, Approaches, and Techniques. Bethesda, MD: American Fisheries Society Symposium, p. 73.
Gomes, L. C., and Miranda, L. E. (2001). Riverine characteristics dictate composition of fish assemblages and limit fisheries in reservoirs of the Upper Paraná River Basin. Regul River 17:67–76.
Hanski, I., Kouki, J., and Halkka, A. (1993). “Three explanations of the positive relationship between distribution and abundance of species,” in Species Diversity in Ecological Communities: Historical and Geographical Perspectives, eds Ricklefs, R., Schluter, D. (Chicago: University of Chicago Press), p. 108–116.
Heino, J., and Tolonen, K. T. (2018). Ecological niche features override biological traits and taxonomic relatedness as predictors of occupancy and abundance in lake littoral macroinvertebrates. Ecography 41, 2092–2103. doi: 10.1111/ecog.03968
Hurlbert, A. H., and White, E. P. (2007). Ecological correlates of geographical range occupancy in North American birds. Glob. Ecol. Biogeogr. 16, 764–773. doi: 10.1111/j.1466-8238.2007.00335.x
Legendre, P., and Anderson, M. J. (1999). Distance-based redundancy analysis: testing multispecies responses in multifactorial ecological experiments. Ecol. Monogr. 69, 1–24. doi: 10.1890/0012-9615(1999)0690001:DBRATM2.0.CO
Matthews, W. J., and Marsh-Matthews, E. (2017). Stream Fish Community Dynamics: A Critical Synthesis. Baltimore, MD: John Hopkins University Press.
McGeoch, M. A., and Gaston, K. J. (2002). Occupancy frequency distributions: patterns, artefacts and mechanisms. Biol. Rev. 77, 311–331. doi: 10.1017/S1464793101005887
McGill, B. J., Enquist, B. J., Weiher, E., and Westoby, M. (2006). Rebuilding community ecology from functional traits. Trends Ecol. Evol. 21, 178–185. doi: 10.1016/j.tree.2006.02.002
Miranda, L. E., and Boxrucker, J. (2009). “Warmwater fish in large standing waters,” in Standard Methods for Sampling North American Freshwater Fishes, eds Bonar SA, Hubert WA, and Willis DW (Bethesda, MD: American Fisheries Society), pp. 29–42.
Miranda, L. E., Coppola, G., Hatcher, H. R., Jargowsky, M. B., Moran, Z. S., and Rhodes, M. C. (2021a). A bird's-eye view of reservoirs in the Mississippi Basin tips a need for large-scale coordination. Fish Fish 22, 128–140. doi: 10.1111/faf.12509
Miranda, L. E., Faucheux, N. M., and Lakin, K. M. (2021b). Fishing gear performance nearshore is substantiated by spatial analyses. Rev. Fish Biol. Fish. 31, 977–987. doi: 10.1007/s11160-021-09683-7
Miranda, L. E., and Killgore, K. J. (2019). Abundance–occupancy patterns in a riverine fish assemblage. Freshw. Biol. 64, 2221–2233. doi: 10.1111/fwb.13408
Miranda, L. E., Wigen, S. L., and Dagel, J. D. (2014). Reservoir floodplains support distinct fish assemblages. River Res. Appl. 30, 338–346. doi: 10.1002/rra.2641
Peipoch, M., Brauns, M., Hauer, F. R., Weitere, M., and Valett, H. M. (2015). Ecological simplification: human influences on riverscape complexity. BioScience 65, 1057–1065. doi: 10.1093/biosci/biv120
Petesse, M. L., and Petrere Jr, M. (2012). Tendency towards homogenization in fish assemblages in the cascade reservoir system of the Tietê river basin, Brazil. Ecol. Eng. 48, 109–116. doi: 10.1016/j.ecoleng.2011.06.033
Reynolds, J. B., and Kolz, L. (2012). “Electrofishing,” in Fisheries Techniques, 3rd edition, eds Zale AV, Parrish DL, and Sutton TM (Bethesda, MD: American Fisheries Society), pp. 305–361.
Rigal, F., Whittaker, R. J., Triantis, K. A., and Borges, P. A. V. (2013). Integration of non-indigenous species within the interspecific abundance–occupancy relationship. Acta. Oecol. 48, 69–75. doi: 10.1016/j.actao.2013.02.003
Ross, S. T. (2015). Ecology of North American Freshwater Fishes. Oakland, CA: University of California Press.
Sanabria-Fernandez, J. A., Lazzari, N., Riera, R., and Becerro, M. A. (2018). Building up marine biodiversity loss: artificial substrates hold lower number and abundance of low occupancy benthic and sessile species. Mar. Environ. Res. 140, 190–199. doi: 10.1016/j.marenvres.2018.06.010
Slatyer, R. A., Hirst, M., and Sexton, J. P. (2013). Niche breadth predicts geographical range size: a general ecological pattern. Ecol. Lett. 16, 1104–1114. doi: 10.1111/ele.12140
Smith, N. G., Buckmeier, D. L., Fleming, B. P., Grubh, A. R., Homer Jr, M. D., and Robertson, S. M. (2023). Spatial variability in the fish assemblage of a large river–reservoir ecosystem. N Am. J. Fish. Manag. 43, 313–326. doi: 10.1002/nafm.10804
Southeastern Fishes Council. (2021). Updated List of Fishes in the Southeastern United States. Available online at: https://sfc8.wildapricot.org/Fishes. (accessed 2021-10-20).
Tales, E., Keith, P., and Oberdorff, T. (2004). Density-range size relationship in French riverine fishes. Oecologia 138, 360–370. doi: 10.1007/s00442-003-1430-1
Taylor, C. M., Holder, T. L., Fiorillo, R. A., Williams, L. R., Thomas, R. B., and Warren, M. L Jr. (2001). Consequences of stream impoundment on fish communities in a small North American drainage. Regul River 17, 687–698. doi: 10.1002/rrr.629
Ten Caten, C., Holian, L., and Dallas, T. (2022). Weak but consistent abundance–occupancy relationships across taxa, space and time. Glob. Ecol. Biogeogr. 31, 968–977. doi: 10.1111/geb.13472
Thornton, K. W., Kimmel, B. L., and Payne, F. E. (1991). Reservoir Limnology: Ecological Perspectives. New York, NY: Wiley.
Tickner, D., Opperman, J. J., Abell, R., Acreman, M., Arthington, A. H., Bunn, S. E., et al. (2020). Bending the curve of global freshwater biodiversity loss: an emergency recovery plan. BioScience 70, 330–342. doi: 10.1093/biosci/biaa002
Verberk, W. C., Van Der Velde, G., and Esselink, H. (2010). Explaining abundance–occupancy relationships in specialists and generalists: a case study on aquatic macroinvertebrates in standing waters. J. Anim. Ecol. 79, 589–601. doi: 10.1111/j.1365-2656.2010.01660.x
Webb, T. J., Noble, D., and Freckleton, R. P. (2007). Abundance-occupancy dynamics in a human dominated environment: linking interspecific and intraspecific trends in British farmland and woodland birds. J. Anim. Ecol. 7, 123–134. doi: 10.1111/j.1365-2656.2006.01192.x
Keywords: rarity, commonness, functional traits, metacommunity, freshwater fishes, reservoirs, dams
Citation: Miranda LE (2023) Fish life-history traits predict abundance-occupancy patterns in artificial lakes. Front. Freshw. Sci. 1:1270939. doi: 10.3389/ffwsc.2023.1270939
Received: 01 August 2023; Accepted: 26 September 2023;
Published: 17 October 2023.
Edited by:
Gerry Closs, University of Otago, New ZealandReviewed by:
Nicolas PECH, UMR 1467 Recover Aix-Marseille University—INRAE, FranceAdam Brysiewicz, Institute of Technology and Life Sciences, Poland
Copyright © 2023 Miranda. This is an open-access article distributed under the terms of the Creative Commons Attribution License (CC BY). The use, distribution or reproduction in other forums is permitted, provided the original author(s) and the copyright owner(s) are credited and that the original publication in this journal is cited, in accordance with accepted academic practice. No use, distribution or reproduction is permitted which does not comply with these terms.
*Correspondence: L. E. Miranda, smiranda@usgs.gov