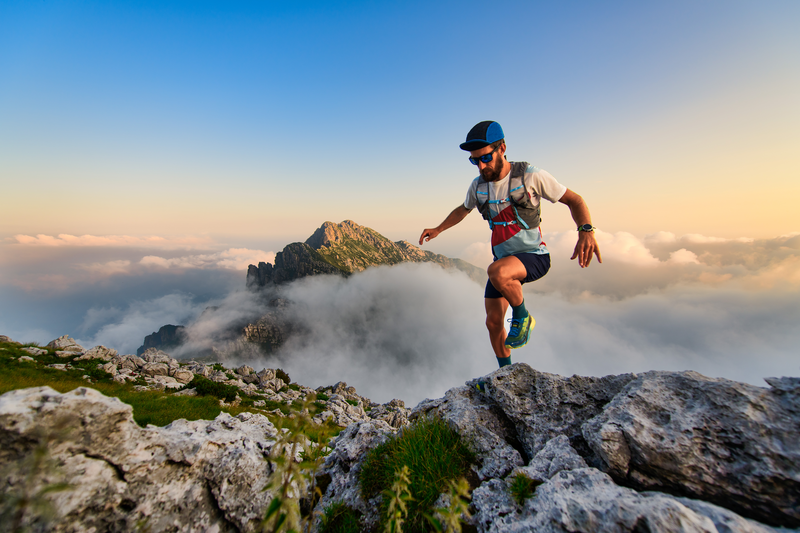
94% of researchers rate our articles as excellent or good
Learn more about the work of our research integrity team to safeguard the quality of each article we publish.
Find out more
ORIGINAL RESEARCH article
Front. For. Glob. Change
Sec. Forest Management
Volume 8 - 2025 | doi: 10.3389/ffgc.2025.1529552
The final, formatted version of the article will be published soon.
You have multiple emails registered with Frontiers:
Please enter your email address:
If you already have an account, please login
You don't have a Frontiers account ? You can register here
The reconstruction of climate factor fields is essential for understanding large-scale climate change. The study utilized climate data from 29 meteorological stations in North China to analyze the spatial distribution of the maximum temperatures in July in the region. It reconstructed the maximum temperature series for July from 1845 to 2002 using 30 tree-ring width chronologies from the area. The findings reveal several key points: (1) July maximum temperatures in North China exhibit four distinct spatial variation types, categorized as Region 1 (West), Region 2 (East), Region 3 (South), and Region 4 (North). (2) Temperature is identified as the primary limiting factor for the radial growth of trees in the study area, with July maximum temperature exerting the most significant influence. (3) A sliding analysis indicates the presence of two relatively cold periods and two relatively warm periods within the reconstructed series, highlighting a prolonged cold phase during the mid to late 20th century. (4) The July maximum temperature series across different regions demonstrates that temperature fluctuations in Region 4 (North) are less pronounced compared to the other three regions; an upward trend in temperatures is observed across all four regions. (5) Morlet wavelet analysis and cross-wavelet analysis of the reconstructed series suggest that the El Niño-Southern Oscillation (ENSO), Sunspot, and the Pacific Decadal Oscillation (PDO) are the primary factors influencing July maximum temperatures in the study area.
Keywords: Tree-ring, Historical Temperature, EOF, PPR, North China
Received: 17 Nov 2024; Accepted: 10 Mar 2025.
Copyright: © 2025 Liu, Li, Wang, Han, Guo, Zhao, Zhao and Du. This is an open-access article distributed under the terms of the Creative Commons Attribution License (CC BY). The use, distribution or reproduction in other forums is permitted, provided the original author(s) or licensor are credited and that the original publication in this journal is cited, in accordance with accepted academic practice. No use, distribution or reproduction is permitted which does not comply with these terms.
* Correspondence:
Shuheng Li, Key Laboratory of Earth Surface System and Environmental Carrying Capacity, College of Urban and Environmental Science, Northwest University, Xi'an, 710127, China
Disclaimer: All claims expressed in this article are solely those of the authors and do not necessarily represent those of their affiliated organizations, or those of the publisher, the editors and the reviewers. Any product that may be evaluated in this article or claim that may be made by its manufacturer is not guaranteed or endorsed by the publisher.
Research integrity at Frontiers
Learn more about the work of our research integrity team to safeguard the quality of each article we publish.