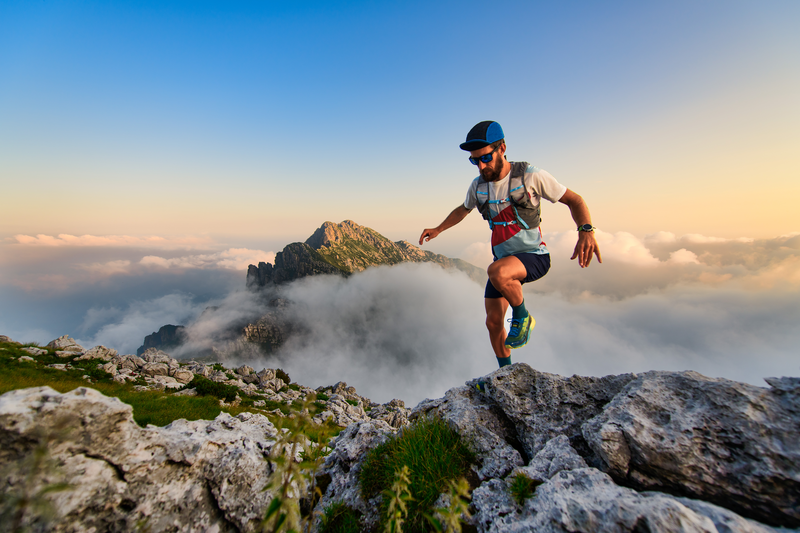
95% of researchers rate our articles as excellent or good
Learn more about the work of our research integrity team to safeguard the quality of each article we publish.
Find out more
REVIEW article
Front. For. Glob. Change , 03 April 2025
Sec. Forest Growth
Volume 8 - 2025 | https://doi.org/10.3389/ffgc.2025.1525461
This article is part of the Research Topic Forest Growth in a Changing Climate: Insights from Predictive Modeling and Adaptive Strategies View all 6 articles
Forests cover approximately 31% of the Earth’s land area. They serve as critical habitats for the majority of terrestrial organisms. Natural regeneration is the main method for renewing forests. This process not only drives forest development but also plays a crucial role in maintaining ecosystem productivity, stabilizing community structure and conserving biodiversity. Current studies indicate that the natural forest regeneration process is influenced by a multitude of environmental factors, including light availability, water resources, wind patterns, soil properties, geography features and groundcover. Light strongly influences processes such as photosynthetic efficiency, biomass allocation and photoinhibition in tree growth. Temperature plays an important role in forest regeneration by influencing seed germination, seedling development, and nutrient cycling in the soil. Water availability regulates the competition between trees and other vegetation. Wind plays a key role in seed dispersal, and with the recovery process following wind disturbances potentially extending for 30–50 years. Soil composition, both physical and chemical, as well as biological factors such as microorganisms, directly determine the trajectory and efficiency of forest ecosystem recovery. Geo-environmental factors such as altitude and topography further shape regeneration by modifying climatic conditions and hydrothermal conditions. In addition, the groundcover layer can promote seed germination while also posing challenges to regeneration through resource competition or by promoting the spread of pathogens and pests. Despite significant advances, several gaps remain in the research: (1) Research on the effects of wind speed on trees’ physiological properties, such as growth and root stability, is limited; (2) Most existing studies primarily focus on seed-based regeneration, with relatively little attention given to coppicing regeneration; (3) There is a scarcity predictive ecological models for coping with future climate change. Addressing these gaps requires more comprehensive studies on the impact of wind factors on the physiological and ecological characteristics of seedlings and young trees to break through the bottleneck associated with natural regeneration. Furthermore, in-depth studies are needed on emergent plants resilience and their adaptability under varying light, soil and climate conditions. A systematic comparison of coppicing regeneration with seed-dependent regeneration is suggested to understand the advantages and challenges associated with different regeneration methods.
Forests, covering about 31% of the world’s land area, are one of the most vital ecosystem on Earth (FAO, 2024). They store about 296 billion tonnes of carbon and provide habitats for most terrestrial species. As the mainstay of terrestrial ecosystems, forests play an indispensable role in mitigating climate change by acting as carbon sinks through photosynthesis (Heimann and Reichstein, 2008; Miles and Kapos, 2008). The ability of forest ecosystems to maintain their ecological functions depends on their capacity for natural regeneration, which is essential for maintaining long-term health and stability (Chazdon, 2008). Forest composition, structure and function are heavily shaped by environmental factors, including topography, soil, elevation and climate (Bond et al., 2005; Tesfaye et al., 2015).
Climatic conditions have varying degrees of influence at each stage of forest regeneration. For example, seed germination is closely linked to specific temperature requirements, with species varying in their optimal winter and spring temperature thresholds (Harrington and Gould, 2015). Wind speed plays a crucial role in seed dispersal, and wind speeds below 2.0 m/s do not allow seeds to travel far enough from the parent tree (Zang et al., 2007). Climate models predict that an annual temperature rising of 2°C, along with a prolongation of the dry season, could reduce annual photosynthesis by 5–11% and decrease transpiration by 5–8%. Conversely, an increase in CO2 concentration and elevated temperatures can increase annual photosynthesis by 56–59% and raise transpiration by 14% (Koskela, 2008). Young trees, with their shallow root systems, are particularly vulnerable to reduced water availability under climate change, as competition for water with groundcover intensifies (Thrippleton et al., 2016).
Global warming also significantly impacts on forest phenology, extending the longer growing season, especially in the Northern Hemisphere, where the start of the plant growing season has been advanced by about 8 days and the end delayed by 4 days (Thrippleton et al., 2016). In addition to these conclusions, the rich biodiversity of forest ecosystems is increasingly at risk due to ongoing climate change, which threatens to disrupt habitats, reduce population sizes, and even drive some species to extinction. For example, increased atmospheric CO2 concentrations and raised sea level had already caused widespread mortality among forest species along both the Atlantic and Pacific coasts (Mueller-Dombois, 1992). In a study by Ma et al. (2016) on broadleaf forests in central U.S. Their results showed the dominant tree species in the region will shift from being dominated by oaks (percentage of total number of species 22.5% Quercus serrata and 20.5% Quercus serrata) and hickories (15.4% Juglandaceae) to being dominated by maples (55.2–60.4% Sapindaceae) by 2,100, and the diversity of tree species will decrease by between 9.6 and 11.5% over the next 90 years. These climate changes will fundamentally affect the stability and functioning of forest ecosystems. Reduced precipitation, especially drought conditions, poses a severe threat to plant regeneration and species composition, as most plant species are highly susceptible to environmental changes during the seedling stage (Bond and Midgley, 2001; Toledo et al., 2011; Tuinenburg et al., 2022).
Natural regeneration, while being one of the primary mechanism for forest renewal, remains a significant challenge in forest management due to the complexity of abiotic and biotic factors involved (Bose et al., 2016; Lucas Borja, 2014). A modeling study by Petrie et al. (2023) predicted that future increases in temperature and reductions in precipitation may drastically alter species composition and diversity during the regeneration phase. Temperature, in particular, influences physiological activities and biochemical reactions in plants, with even small shifts in temperature significantly influencing seed germination and overall seedling growth (Tang, 1989). Xu (2007) found that 20°C was more conducive for breaking seeds dormancy in comparison with lower or higher temperature, highlighting the variation in dormancy performance under different conditions. Soil, as a provider of essential nutrients and water, plays a crucial role in determining whether the plant can access the necessary resources for survival and development. For example, Turczanski et al. (2021) found that ash tree saplings in plots with soil pH below 6.5 were less susceptible to damage compared to those in plots where pH exceeded 7.0. The regeneration stage offers the greatest potential for forests to adapt to new environmental conditions over the long term (Qiu et al., 2021) Thus, understanding the interactions between these environmental factors and how they influence forest regeneration is critical for promoting natural regenerations and ensuring healthy plant growth.
In summary, forests are essential to human well-being and global biodiversity. Restoring their ecological functions and biodiversity after disturbances is a core objective for achieving sustainable development. In recent years, significant progress has been made in understanding how natural forest regeneration responds to environmental factors, particularly in areas such as climate change, water availability, soil conditions, biodiversity, human activities, ecosystem services and ecosystem restoration (Bond and Midgley, 2001; Holl and Aide, 2011; Rodriguez-Garcia et al., 2011; Djiofack et al., 2024; Hishe et al., 2021). These studies provide a scientific basis for developing forest management strategies that address the challenges of climate change, while offering new perspectives on ecosystem restoration. This paper reviews recent advances in research on environmental factors affecting natural forest regeneration, aiming to deepen our understanding of forest regeneration mechanisms and promote sustainable forest management.
Natural forest regeneration refers to the process by which new forests emerge through the reproductive capacity of existing trees. Compared with artificial regeneration and artificially facilitated natural regeneration, natural regeneration has significant advantages, such as saving money on silvicultural requirements, requires less heavy equipment, and does not require a large investment in human and financial resources; could better preservation of forest biodiversity. In addition, natural regeneration has certain disadvantages, such as seedlings and saplings grow slowly, extending the regeneration period and it fosters the growth of secondary non-target tree species, potentially decreasing the yield of the target species in commercial forests. Additionally, it serves as a key strategy for achieving sustainable forest development (Chazdon, 2008).
An overview of natural forest regeneration pathways found, as Figure 1, natural forest regeneration is primarily occurring through two mechanisms: sexual reproduction (seed-based regeneration) and asexual reproduction (coppicing regeneration). In seed-based regeneration, forest renewal is achieved through seed dispersal and germination. This process requires adequate seed production, effective dispersal mechanisms, and favorable environmental conditions for seedling establishment. Seed dispersal and migration contribute to the expansion of species distributions, playing a vital role in the “colonization effect,” which helps establish new populations in previously unoccupied areas (Bond and Midgley, 2001). In contrast, coppice regeneration involves the forest regeneration from shoots or sprouts that emerge from the stumps or roots of felled trees. This process, often referred to as the “persistence effect,” allows plants to rapidly reoccupy space and resources following disturbances that reduce aboveground biomass. Coppicing enables species to maintain their niche in disturbed environments by quickly restoring their pre-disturbance condition (Bond and Midgley, 2001).
When forest ecosystems are predominantly governed by sexual reproduction (seed-based regeneration), the resultant resource allocation patterns and temporal gaps in canopy closure may create ecological niches conducive to invasive species colonization. This phenomenon can significantly alter species composition and structural complexity within disturbed communities through competitive exclusion mechanisms (Knapp et al., 2017). In contrast, plant communities characterized by robust asexual regeneration (coppicing) exhibit enhanced ecosystem stability, as evidenced by their superior autogenic regulation capacity and post-disturbance resilience (Bond and Midgley, 2003). These communities can also foster isolated population distributions and promote endemism. However, this resilience may be accompanied by a slower rate of species turnover and reduced overall species richness (Bond and Midgley, 2001). Studies have indicated that in the early stages of community succession, tree species that primarily regenerate through coppicing can improve habitat conditions following disturbances, creating opportunities for other species to establish and ultimately enhancing plant diversity (Caplat and Anand, 2009). The regeneration method used by woody plants directly affect the successional process of forests. For example, if pioneer species in a community regenerate primarily through coppicing, vegetation succession may slow. However, if coppicing species dominate later stages of succession, the process can accelerate. Thus, how forests naturally regenerate not only determines the population composition and the ecosystem stability but also affects their long-term successional dynamics and ecological balance (Feng, 2012). There is also a trade-off between the seed-based and coppice regeneration in forest ecosystems (Mc Carthy et al., 2014). with this balance being influenced by different disturbance regimes such as logging intensity (Escandon et al., 2020).
Most current studies focus on seed-based regeneration and the effects of various biotic and abiotic factors, including seed source, light, soil, moisture, temperature, animals, understory scrub layer, pathogenic fungi, and others (Li and Zhang, 2001; Zhang et al., 2023; Scariot, 2010; Zhang et al., 2017; Guariguata and Pinard, 1998). While research on seed-based regeneration is relatively comprehensive, it often isolate individual factors, making it challenging to identify the underlying mechanisms influencing natural seed regeneration. Future studies should emphasize a more systematic investigation into natural seed-based regeneration processes, considering the interactive effects of multiple factors. In contrast, Coppice regeneration is prevalent in forest ecosystems (Yan et al., 2005), and is a way for plants to cope with disturbances (Tredici, 2001). Second-growth forests are mostly formed after primary forests have been harvested to a certain extent, so coppice regeneration as a direct regeneration method is of great practical significance for second-growth forest management and management, as well as for the production of energy materials (Bellingham and Sparrow, 2000; Li et al., 2005). However, although coppice regeneration plays an important role in maintaining the stability of forest communities under disturbed or stressful conditions, studies on coppice regeneration have been limited to qualitative descriptions, with less attention paid to the processes and mechanisms, resulting in various aspects of coppice regeneration lagging behind seed regeneration (Klimesová and Klime, 2007) Further research is needed to fully comprehend the ecological roles of coppice regeneration and its implications for forest recovery, particularly in response to disturbances and changing environmental conditions.
Natural forest regeneration is influenced by a diverse array of environmental factors, including climate, soil, geography, and ground cover. These factors can act as barriers at various stages of the forest regeneration, particularly when their effects exceed or fall below certain threshold values. Understanding the interactions among these factors is crucial for promoting successful regeneration and ensuring the resilience of forest ecosystems. Currently, academic research on these influencing factors remains relatively limited, often focusing on individual factors or a select few, while lacking an comprehensive exploration of their combined effects. Therefore, further research on the synergistic interactions among these factors is urgently needed.
Light is a fundamental ecological factor influencing plant survival, growth, and spatial distribution. Changes in light availability can trigger adaptive responses in plants, leading to alterations in their morphology and photosynthetic characteristics (Zhou et al., 2017). These adaptive mechanisms enable plants to optimize their growth and survival under varying light conditions. Light availability affects key physiological processes, including photosynthetic efficiency, biomass partitioning, light-induced inhibition, protection mechanisms, and ecological adaptations. Consequently, fluctuations in light can shape plant responses through alterations in these dimensions, thereby affecting their growth trajectories, resource distribution, and survival strategies. For instance, Zhang et al. (2022) found that both full light and deep shade conditions limited the growth and photosynthetic efficiency of maple species (Acer mono and Acer pseudosieboldianum), and that only when properly shaded could they cause an increase in the content of, for example, antioxidant enzyme activity or osmoregulatory substances within the two species of maple and promote seedling regeneration. This highlights the complex role of light intensity on seedling development. Differences in the optimum light intensity for seedling growth among different tree species were also found, which is consistent with the findings of Zhu et al. (2024). Xue et al. investigated the growth and photosynthesis responses of Castanopsis hystrix under shading treatments of 0, 40, 60 and 80%. They found that the 60% shading treatment was the most effective in improving the growth and photosynthetic characteristics, promoting leaf organic matter production while limiting stem growth (Xue et al., 2023). In another study, Luo et al. examined the growth physiological changes of Horsfieldia hainanensis under full light conditions, and found that proper shading (67.5 and 45.7% of natural irradiance) promoted root development, increased chlorophyll content and photosynthesis, and eventually accumulated a large amount of biomass (Luo et al., 2023).
Solar radiation transfer is also an important factor that directly affects the tree growth rates. In the process of light gap treatment, excessive exposure to sunlight in forest gaps can result in higher seedling mortality, as direct solar radiation dries out and damages young plants (Santelices-Moya et al., 2024). Kitao et al. (2023) demonstrated that reducing direct solar radiation can enhance seedling survival and growth, suggesting that moderate shading can protect against photoinhibition. Additionally, canopy plays a significant role in regulating regeneration. Low canopy cover promotes forest regeneration and plant growth, but overly dense canopies may reduce light penetration, inhibiting growth and regeneration (Martinez Pastur et al., 2011). A higher canopy coverage increases seed production and hence regeneration density, but higher tree density also reduces solar radiation transmission rates, which reduces light available for plant growth and affects tree growth (Pastur et al., 2008), This indicates that there is a favorable range for natural forest regeneration between canopy coverage and tree density, which needs to be taken into account in forest regeneration management.
All studies support that moderate shade (e.g., 60% natural light) can significantly promote photosynthesis and growth of seedlings by alleviating photoinhibition, regulating antioxidant enzyme activities and optimising biomass partitioning, whereas excessive shade (>80%) or full light can inhibit plant growth by exacerbating reactive oxygen species accumulation or photodamage (Zheng and Zheng, 2013). There are significant differences in the light environment requirements of different species and different life stages of the same species, which are closely related to genotypes, light signaling regulatory pathways and shade tolerance. For example, shade-tolerant species can adapt to low-light environments under shade via the photoreceptor-PIF-growth factor pathway, whereas light-loving plants require higher light intensities to maintain metabolic homeostasis (Liu et al., 2019). To summarise, light conditions profoundly affect plant growth and regeneration by influencing photosynthetic efficiency, biomass allocation, photoprotection mechanisms, and ecological adaptations. Effective management of light, especially through moderate shading, can optimise the plant-growing environment and promote healthy regeneration. Moreover, tailoring light management strategies to the specific needs of different tree species can significantly enhance regeneration outcomes.
Temperature is another fundamental factor affecting the plant natural regeneration, influencing seed germination, growth rates, flowering and fruit ripening, dormancy, and susceptibility to pests and diseases. Research has found that mean annual temperature is second only to tree species in influencing seedlings density in regenerating woodland. Higher temperatures generally increasing seedling emergence, development and growth, but can also decrease survival, with small changes in temperature (3°C) having a significant effect on the seed germination stage (Fisichelli et al., 2014). Increased temperatures generally promote the survival of tree seedlings, but excessively high temperatures may reduce survival, especially in competitive environments (De Lombaerde et al., 2020). Appropriate temperature conditions can facilitate seed germination, within a certain range, rising temperatures can boost germination rates. However, once temperatures exceed a critical threshold, germination rates decline sharply (Wang, 2015). Different species exhibit varying optimal temperature ranges for seed germination. For example, on the high plateau mountains of southern Gansu, seeds requiring dormancy break thrive at moderate temperatures (around 20°C, subtropical conditions), while higher temperatures (e.g., 30°C) primarily benefit non-dormant seeds (Xu, 2007).
However, extreme heat can negatively impact regeneration. For example, the probability of successful germination for seeds of pioneer species decreases with increasing temperatures, and drops to 0 percent after 42 days for aspen (Populus tremula), birch (Betula pendula) and larch (Larix decidua) at an air temperature of 40°C in humid condition. With the continuously increasing in air temperature, the window for natural regeneration may be reduced by half, decreasing from over three months to as little as three weeks for certain species (Tiebel et al., 2023).
According to the latest Intergovernmental Panel Climate Change (IPCC) report, global temperatures are currently 1.1°C above pre-industrial levels (Change IPCC Climate, 2022). Variations in annual temperatures can lead to differences in diversity in forest regeneration, and leading to decrease in seedling densities with increasing temperature (Khaine et al., 2018). The frequency and duration of extreme temperature events and heat waves are expected to increase, resulting in heightened thermal stress on trees. Excessive heat, particularly during extreme weather events, can expose trees to thermal stress, leading to physical damage such as cracking and growth abnormalities. These stresses often reduce regeneration rates and compromise the quality of tree recovery (Change IPCC Climate, 2014). The natural regeneration capacity of different tree species at the forest line varies depending on the species’ regeneration capacity and the temperature requirements for seed germination. For example, Juntunen and Neuvonen (2006) found that Norway spruce (Picea abies) seedlings always outnumbered and outgrew European red pine (Pinus sylvestris) seedlings near the forest line due to the nutritional regeneration capacity of spruce and the lower temperatures required for its seed maturation. Furthermore, tree species at the timberline respond more sensitively to climate warming relative to decrease in temperature. In the temperate alpine environment of central Spain, as global temperatures rise, timberlines may shift to higher elevations, altering the species composition of regenerating forests (Enríquez-de-Salamanca, 2022).
Studies have shown that soil temperature affects the dynamics of soil nutrients, which in turn affects the deepening of the root system in the growth of young trees and seedlings and improves their resilience, among other things, thus having a significant impact on the regeneration of natural forests. Optimal soil temperatures promote microbial metabolic activity, increasing available phosphorus concentration in the soil, which supports plant uptake and forest regeneration (He et al., 2019). At the same time, higher soil temperatures also stimulate microbial activity, accelerate processes such as denitrification, and increase N2O fluxes in the soil, collectively promoting natural regeneration. Therefore, soil temperature plays a crucial role in regulating microbial metabolism and soil nutrient dynamics, which in turn impact the forest regeneration rate (Zhang et al., 2021). Moreover, soil temperature has also been found to significantly influence soil CO2 fluxes, displaying a single-peak trend that aligns with seasonal climate changes, peaking around August (Liu S. et al., 2020). These findings demonstrate that soil temperature regulates the dynamics of the content of carbon and phosphorus in soil nutrients to influence physiological activities such as photosynthesis, and that it also influences the growth of the plant root system, which in turn influences the area of root-soil contact to affect nutrient uptake and utilisation, and ultimately the rate of natural regeneration.
Additionally, vegetation cover on the forest floor plays a crucial role in moderating soil temperature and its fluctuations. During summer, dense vegetation reduces the heat input to the soil by mitigating direct sunlight effects. In contrast, during winter, vegetation cover helps to maintain soil warmth and minimize temperature fluctuations by obstructing radiative cooling. At the same time, snow on vegetation has an insulating effect, and although snow has a lower temperature, it is effective in preventing surface temperatures from becoming too low (Voropay et al., 2022). This vegetative mechanism ensures that soil temperature remains within a more favorable range throughout different seasons, thereby supporting the natural forest regeneration.
The effects of warming on tree regeneration in high-and low-elevation forests show significant differences: at high elevations (e.g., Himalayas), warming promotes regeneration and treeline migration of cold-adapted species (e.g., Himalayan fir) by alleviating low-temperature constraints (at rates of up to 1.1 m/10 yr), whereas in low-elevation temperate forests high temperatures impede the regeneration and growth of cold-adapted species (e.g., Himalayan fir) through exacerbation of water stress or direct inhibition of seed physiological activity. Germination and growth of pioneer tree species (e.g., poplar). This difference may be due to the fact that cold-adapted species (cold-tolerant) are adapted to high altitude through temperature sensitivity, whereas pioneer species (drought-tolerant) are more susceptible to low altitude high temperatures due to moisture sensitivity or that low soil temperatures at high altitude may buffer the negative impacts of warming air, whereas the superimposed soil and air temperatures at low altitude exacerbate the high temperature stresses, although the synergistic effect has not yet been adequately quantified (Sigdel et al., 2024; Yang et al., 2022). Overall, temperature significantly influences natural forest regeneration. It affects not only the germination and growth rates in tree species but also the broader ecological balance within forest ecosystems. As climate change continues to alter thermal conditions worldwide, understanding and managing temperature-related challenges will be essential for successful forest regeneration and sustainable forest management.
The water factor is crucial for natural forest regeneration, as it supports critical physiological processes such as physiological processes, cell expansion, seed germination and photosynthesis. Photosynthesis, as the most important physiological process of plants, the water in the external environment will affect the process of plant water absorption, aeration, etc.; the water in the plant body will affect the process of plant photosynthesis in a variety of photosynthetic pigment synthesis, light reactions, etc., which in turn affects the forest regeneration (Li, 2012). Additionally, water availability influences the environmental adaptability and ecological distribution in plants, which in turn contributes to ecosystem stability and community regeneration (Tang, 1989).
The decrease in precipitation and prolonged summer drought represent significant factors limiting forest regeneration, with seedling density being highly dependent on annual average rainfall and maximum rainfall. In general, increases in annual average rainfall and maximum rainfall correlate with higher densities of seedlings and young trees (Khaine et al., 2018).
Summer precipitation, in particular, plays a crucial role in the regeneration process. For example, De Frutos et al. (2022) monitored tree regeneration in a mountainous region of southern Spain over a 7-year period and found that September rainfall positively affects seedling survival during dry summers. Similarly, Calama et al. (2017) studied the germination growth of Pinus sylvestris seeds sown in different sowing seasons (March–June in spring and September–November in autumn), found that seedlings sprouted from spring sowing had a survival rate of 17% after 90 days of growth, compared to 42% for seedlings sown in autumn, suggesting that Pinus sylvestris regeneration is highly dependent on summer rainfall, as spring germination. This indicates that Pinus sylvestris regeneration is highly dependent on summer rainfall, as spring germinated seedlings are more likely to die during summer droughts, but the difference in seedling survival between sowing seasons was not significant after the first summer (Fernandes et al., 2017).
In addition, the rainfall variability in other seasons can also affect natural forest regeneration. Prolonged periods without precipitation in spring can impede the timely germination of pioneer tree seeds, potentially leading to an accelerated loss of seed germination capacity in pioneer tree species (Tiebel et al., 2023). In semi-arid and Mediterranean climates, limited water availability directly constrains forest regeneration and growth. Insufficient soil moisture during drought conditions impairs trees’ ability to perform normal photosynthesis and other physiological processes, thus inhibiting growth and regeneration (Enríquez-de-Salamanca, 2022). Toledo et al. (2011) reported that tree growth in Bolivian lowland forests was lowest during the dry season, with regeneration rates increasing as water availability improved. Furthermore, different species with varying rainfall requirements also significantly influence species variety and diversity in forest regeneration, some species are distributed in a wide range of rainfall areas, while others are adapted to specific areas. For example, tree species such as Xylia dolabriformis and Tectona grandis show differential regeneration rates depending on annual rainfall, with the former performing better in regions receiving between 843 and 2035 mm annually (Khaine et al., 2018).
As climate change intensifies, arid regions will face even greater challenges to forest regeneration. Future net primary productivity (NPP) for tree species in arid areas of China is predicted to decline significantly, while NPP in humid areas is expected to rise (Ding et al., 2019). Changes in hydrological conditions pose a serious threat to the regenerative capacity of forest ecosystems, while natural forest regeneration has a corresponding impact on hydrological conditions. Pietsch et al. (2003) simulation of the oak model in a river floodplain ecosystem found that in trees with rooting depths less than 0.5 m experience significant hindrances to water absorption due to continuous declines in groundwater levels, further obstructing regeneration. In the early stage of forest regeneration, increased tree density may lead to higher evaporation rates and reduced water availability, causing further declines in forest productivity (Inbar et al., 2022). Arid soils severely limit the growth and regeneration of trees and grassland vegetation. Under normal conditions, small-leaved poplar trees can grow to more than 15 metres (Sun et al., 1998). Different tree species have different soil water use strategies and trade-offs during regeneration. Conifers typically conserve water, whereas deciduous species use water more efficiently for photosynthesis and growth, leading to faster soil moisture depletion (Niemczyk et al., 2023). Water conditions also influence competitive dynamics between species. For instance, wet soil conditions can enhance the competitiveness of Betula platyphylla, while persistent flooding may reduce its growth rate relative to competitors like Eupatorium sp. (Kitao et al., 2022).
Similarly, the effect of the water factor on vegetation regeneration varies significantly across climatic zones: in the Mediterranean climate zone, summer precipitation plays a key role in pine regeneration, whereas tropical rainforest regeneration is more dependent on the total annual precipitation than on seasonal distribution. This may be due to different vegetation water use strategies, soil water holding capacity and canopy retention effects in the study area (Qi et al., 2024; Jiang et al., 2020; Liu et al., 1994). In summary, water availability is a critical factor in forest regeneration, affecting not only physiological processes but also species competition and ecosystem stability. As water resources become scarcer due to climate change, forest management strategies must adapt to ensure sustainable regeneration.
Natural regeneration processes, including seed production, dispersal, and seedling establishment, exhibit considerable uncertainty, with wind factors significantly influencing these stages. Wind is a driving force in plant ecology and evolution. Outside the tropics, most forests are occupied by plants whose seeds, pollen or spores are wind-dispersed. For example, in United States forests, wind-borne or wind-dispersed trees account for an estimated 95% of the basal area of the forest and 75% of the tree species, whereas in tropical forests between 3 and 30% of the tree species are wind-dispersed and between 1 and 10% are wind-borne. Wind-dependent plants include almost all conifers, grasses, ferns and mosses, and a few flowering plants (Regal, 1982; Ollerton et al., 2011; Howe and Smallwood, 1982).
More than 90% of seeds in seed rain are wind-dispersed, but wind speeds below 1.6 to 2.0 m/s often insufficient to carry seeds over long distances, reducing the effectiveness of seed dispersal and, consequently, naturally regenerating (Zang et al., 2007). However, this limitation has a relatively minor effect on overall forest regeneration compared to other factors.
On a larger scale, windstorms can have profound and long-lasting impacts on forest structure and regeneration. Following medium and large-scale storm disturbances, the recovery process can be slow and dynamic, often taking 30 to 50 years for the canopy to fully close and for species composition to stabilize. These disturbances can lead to shifts in dominant species, as wind-damaged forests may create openings that allow more light-demanding species to establish and grow, thereby altering forest composition and structure over time (Ganzhorn et al., 1999).
Soil particle composition, microbial communities, nutrients availability and pH are important for maintaining natural plant regeneration, and they interact to provide a healthy soil environment for plant growth and play an essential role in determining the pattern and success of forest regeneration.
Soil particle composition ranks among the most important soil physical properties, directly affecting soil moisture transport, soil erosion, accumulation, and physicochemical processes, thereby affecting the distribution pattern of vegetation renewal (Bayat et al., 2015). Soil particle size plays a crucial role in determining the type and rate of forest vegetation restoration. A study of soil properties in three forest types: mixed, pure and economic forests showed that soils with a high clay or chalk content tend to support the regeneration in mixed and pure forests, while finer sandy soils are more suitable for the development of economic forests (economic forests is a forest for the production of dried and fresh fruits, edible oils, beverages, spices, industrial raw materials and medicinal herbs, etc., and is an important part of the forest resource) (Li, 2018). Soil texture also affects regeneration dynamics differently between forest types: broadleaf forests on clay-rich soils typically show better understorey recovery, whereas coniferous forests regenerate more effectively on coarser, well-drained soils (Danilov et al., 2020).
Soil particle size can also influence essential nutrients availability for forest regeneration. For example, soils with higher clay content generally have higher carbon content and carbon-to-nitrogen ratio, both of which are positively correlated with increased soil moisture, while nitrogen and phosphorus availability may decrease (Azaryan et al., 2022). These nutrient dynamics significantly shape the recovery and growth of forest ecosystems.
Soil microbial communities play crucial ecological and physiological roles in natural forest regeneration. These microorganisms contribute to forest regeneration by improving the soil’s physical and chemical properties, thus increasing its overall suitability for plant growth (Sofo et al., 2014; Sofo et al., 2012). This process involves an increase in the modularity of the symbiotic network among soil bacterial communities and the enrichment of bacteria involved in key metabolic pathways, positively influencing forest regeneration. These effects primarily link to microbial interactions with soil nutrients, height, litter thickness, and herbaceous plant diversity (Liu J. B. et al., 2020; Massaccesi et al., 2020). For example, de Medeiros et al. (2017) demonstrated that after 17 years of natural regeneration in secondary forests, changes in plant diversity and composition promoted an increase in soil microbial biomass. In turn, and microbial communities released soil nutrients, contributing to the vegetation development and forest regeneration rate (de Medeiros et al., 2017). This highlights the important influence of plant diversity on soil microbial communities and their ecological processes. As forest communities regenerate, the complexity of soil bacterial communities tends to decrease. During the regeneration of larch (Larix gmelinii var. principis-rupprechtii), the bacterial community was found to be more active and influential on ecological processes than fungal community (Niu et al., 2022), suggesting that bacteria play a key role in forest regeneration. In addition, natural forest regeneration promotes the growth of mycorrhizal fungi. Nogueira et al. (2016) indicated that these mycorrhizal fungi significantly influenced the accumulation of soil organic carbon and carbon pools, playing an important role in the ecological succession of plant species. Further studies in Puerto Rican woodlands have also shown that natural regeneration occurring after agriculture activity leads to variations in the functional potential of soil microorganisms with stand age (Pressler et al., 2020).
Nutrient availability in soils, particularly nitrogen and phosphorus, directly impacts forest regeneration (Ricklefs et al., 1999). As forests regenerate, nitrogen and phosphorus levels typically increase with species diversity and structural complexity. Over the past several decades, research has shown that the increased nitrogen deposition, combined with global warming, has led to phosphorus limitation in subtropical forests. Under such conditions, plants tend to improve phosphorus cycling efficiency, reducing phosphorus content in litter (Kitayama et al., 2000; See et al., 2015). This leads to more phosphorus being released back into the soil through decomposition. Xiong et al. (2023) found that nitrogen limitation became more pronounced during the regeneration of Phoebe bournei forests, underscoring the importance of nutrient management for successful regeneration. In general, ammonia nitrogen and soil organic matter, among other variables related to soil properties, had a large contribution to natural regeneration (Wang et al., 2016), and Zhao et al. (2023) used SEM analysis found that TN (total nitrogen) greatly contributed to natural regeneration compared with other soil properties, TN (p < 0.05) was negatively correlated with plant regeneration, high levels of TN can lead to reduced resistance of tree seedlings, thinning of leaf thickness, etc., thus limiting the natural regeneration of plants. Though high levels TN would limit natural regeneration, but seedling growth required the consumption of a large proportion of TN (Xu et al., 2018). In sites with high fertility, nutrient input increases with stand density during natural regeneration. In sites with lower fertility, nutrient input increases with tree species richness and the proportion of nitrogen fixing tree species, indicating that the relationship between plant community renewal effect and nutrient cycling largely depends on site conditions (Machado et al., 2021).
Soil pH represents another key factor influencing forest regeneration. In tropical and temperate regions, neutral soils with pH values between 6.0 and 7.5 generally provide optimal conditions for natural regeneration, as nutrients are more readily available in this range (Horneck et al., 2011). Loeb and Mao (2021) found that higher pH levels can sometimes hinder regeneration, particularly for species such as Acer rubrum and Fraxinus americana, which are sensitive to elevated pH. In contrast, other studies, such as Kassa et al. (2020), have shown that neutral pH conditions promote richer nutrient availability and improve plant regeneration. These differences suggest that soil pH effects may vary depending on species-specific responses and the broader ecosystem context. The difference in results may due to species variations, as Robert E. Loeb focusing on a few broadleaf species, while Kassa et al. came up with generalised patterns for most tree species in the study area. Both community diversity and tree species growth were significantly and positively correlated with soil pH, indicating that soil acidity and/or alkalinity can regulate plant community diversity and the natural regeneration process in forests (Xiong et al., 2023). Soil acidity and alkalinity have different effects on the growth status across various different tree species. Soil pH closely relates to the regeneration of broad-leaved trees, but has little correlation with coniferous forests (Danilov et al., 2020). Marek Malicki and Rem et al. (2018) in Czerwona Woda River ValleyintheStołowe Mountains National Park found that soil pH affects density at all ages and heights in a Norway spruce where located in a low altitude region, and there was a negative correlation between soil pH and plant age-hight classess, that is, tree seedlings grow more under lower soil pH conditions (Dyderski et al., 2018).
In conclusion, soil properties, including particle size, microbial communities, nutrient content, and pH, play a fundamental role in shaping natural forest regeneration. Understanding how these factors interact can provide valuable insights for developing effective forest management strategies, particularly in the context of changing environmental conditions.
Geo-environmental factors such as altitude, slope, and topography are indirect ecological factors that play a key role in the natural forest regeneration by modifying climatic conditions, hydrology, soil properties and microclimatic environments. These factors play a crucial role in determining the distribution, growth, and survival of plant populations during the natural regeneration process.
Topographic heterogeneity significantly impacts early forest regeneration. This process involves the combined effects of environmental filtering and resource competition among plants. Slope orientation and gradient affect the amount of solar radiation, soil moisture, and nutrient retention, which in turn influence seedling survival and growth rates. The effect of topography on regeneration varies between species, with deciduous species being more sensitive to topographic changes than evergreen species. For instance, the mortality rate of deciduous species is estimated to be influenced by topographic factors by as much as 65%, while for evergreen species, this figure is only 30% (Zhang et al., 2023). In addition, slope gradient also affects soil depth and nutrient retention. Steeper slopes often having shallower soils that store fewer nutrients and are more prone to erosion, creating unfavorable conditions for seed storage and germination, which reduces seedling survival (Pham et al., 2022). Slope orientation plays an essential role in determining how much solar radiation the forest floor receives (Maciel-Nájera et al., 2020). Zhao et al. (2023) found that the northwest-facing slopes received more solar radiation than the west-facing slopes, leading to a higher density of seedling regeneration in the former. This suggests that slope orientation can significantly influence regeneration patterns based on the light requirements of tree species.
Terrain creates contrasting microclimates, especially between arid north and south slopes. Principe et al. (2019) found that areas with higher potential solar radiation, such as south-facing slopes, had significantly lower tree age and density due to the limiting effects of intense sunlight and higher temperatures on seedling germination and sapling development. Additionally, terrain can also partially affect soil nutrient accumulation and retention. For example, total nitrogen levels tend to increase with slope position, while phosphorus availability may decrease as slope steepness increases (Liang and Wei, 2020).
Altitude is another critical geo-environmental factor influencing forest regeneration by affecting temperature, moisture, and soil nutrient composition. Trees at higher altitudes typically experience lower temperatures, which can slow down growth rates and limit regeneration success. Orman et al. (2023) found that the Norway spruce (Picea abies (L.) H. Karst.) saplings at high-altitude had a thicker, more elongated crown compared to their lower altitude samples, reflecting structural adaptations to the cooler and more challenging climate at higher elevations. With the increase of altitude, the species diversity often decreases, Sigdel et al. (2024) modelling of tree lines in the central Himalayas showed that the fir moved upward at a faster rate than the birch, while the birch replenishment was drastically reduced. Thus, fir is likely to outcompete birch at the mixed treeline and is expected to be the dominant treeline species in the Himalayan region, leading to changes in species composition near the treeline (Sigdel et al., 2024), and altitude has varying degrees of impact on the availability of soil nutrients. The availability of key soil nutrients, such as soil organic carbon, nitrogen, phosphorus, potassium, and zinc increases with altitude, while pH and available iron decrease (Wani et al., 2022). Additionally, tree seeds differed significantly across the altitudinal gradient, with seeds from lower altitudes showing higher germination rates but longer germination times. In contrast, seeds from higher altitudes exhibit lower germination rates, suggesting that seeds of Ephedra saxatilis from lower altitudes have a survival advantage over seeds from medium to high altitudes (Wardle, 1970). However, studies suggest that altitude gradients less than 300 meters may not significantly impact vegetation development or regeneration due to minimal changes in temperature and moisture conditions (Liu et al., 2012). This finding indicates that the effects of altitude on regeneration are species-specific and vary depending on the microclimatic conditions and the broader geographical context.
In summary, geo-environmental factors such as slope, altitude, and topography have a comprehensive impact on the forest regeneration. These factors alter climatic conditions, hydrological processes, and soil nutrient availability, thereby shaping the distribution, growth, and survival of tree species.
Ground layer can be divided into live forest ground layer and dead forest ground layer according to its biological activity, with live forest ground layer referring to organisms such as dwarf herbs, lichens, mosses, etc. that live in forest environments, and dead forest ground layer referring to branches, leaves, flowers and fruits that fall to the ground after the forest plants have withered and faded and can also be referred to as forest litter (Facelli and Facelli, 1993). Ground layer plays a crucial role in natural forest regeneration. It can either facilitate or inhibit the regeneration process by influencing seed germination, seedling survival, and growth. The effects of the ground layer are complex and often double-edged, depending on factors such as the thickness, composition, and moisture retention properties of the cover.
The forest surface litter layer is a key factor in regeneration process. Litter provides essential benefits such as shading, moisture retaining, insulation and protection from mechanical damage. However, it can also pose challenges through its potential to chemically inhibit seedling growth, block sunlight, or harbor pathogens and pests (Asplund et al., 2018; Calviño-Cancela et al., 2018). Increased litter thickness enhances water-holding capacity, stabilizes temperatures, and creates favorable conditions for seed germination by reducing soil moisture evaporation (Petrou and Milios, 2020). In southeastern Tibet, Wang R. H. et al. (2024) found a positive correlation between litter thickness and the presence of fir seedlings, juvenile trees, and small trees, indicating that a sufficiently thick litter layer supports regeneration. However, excessive litter can impede seedling establishment by limiting light availability, particularly in the early germination and seedling development. In some cases, seeds may perish in the litter layer as their roots can not penetrate the soil and access nutrients (Zhao et al., 2023; Lu et al., 2021). Moreover, large amounts of litter may physically block seeds from contacting the soil or create a chemical environment that is toxic to seedling growth, as decomposed litter releases compounds that inhibit germination (Willis et al., 2021). This dual role of the litter layer underscores the need for balanced management of ground layer conditions to support regeneration, for example, natural regeneration of forests is promoted through the introduction of decaying organisms such as earthworms and beetles to promote the natural decomposition of dead wood and reduce its inhibitory effect on seedling growth, and the moderate clearing of excessively thick layers of dead wood at specific times to improve light conditions and reduce anthropogenic disturbances such as the harbouring of pathogens and pests.
Mosses are another important part of the ground layer, playing a significant role in regulating the forest floor’s water and nutrient cycles, which are essential for seed germination. The impact of mosses on forest regeneration largely depends on the thickness and water content of the moss layer (Wheeler et al., 2011). Research has shown that the thickness and coverage of the moss layer are critical for water vapor exchange and can positively influence seedling emergence due to their ability to retain moisture (Wang R. H. et al., 2024). In addition, the inherent water retention capacity of the moss layer provides important hydration that promotes seed germination and seedling growth (Zamfir, 2000). However, while mosses promote the germination of seedlings, they can hinder later stages of seedling growth, particularly when the moss layer is too thick. Dense moss layers may prevent adequate water infiltration into the soil and restrict the availability of nutrients to developing saplings, resulting in high mortality rates (Ali et al., 2019). Thus, the relationship between moss thickness and regeneration is thus complex: a moderate moss cover enhances moisture conditions for germination, but excessive moss growth can create microhabitats that are too dry for seedlings, limiting their survival. The litter layer and the moss layer seem to have similar roles, and a comprehensive analysis may lead to more accurate conclusions, while it should be possible to integrate these two components in forest management, for example, by moderately controlling the thickness of the litter and moss layer, the water retention capacity of the soil, the supply of nutrients and the conditions for germination of seeds can be optimised; the appropriate removal of litter and moss to reduce the number of pathogens and pests harbouring, etc.
Shrubs also play a multifaceted role in the regeneration process. Shrub cover can positively correlate with the number of saplings and regeneration potential, as shrubs often provide a buffer against environmental extremes and protect seedlings from desiccation. For example, Wang Z. Z. et al. (2024) found that higher shrubs cover was associated with improved regeneration potential in multi-species forests, indicating that shrub cover can serve as a useful indicator of forest community regeneration potential. However, the effects of shrubs can vary depending on their dominance and diversity. In some cases, species like tamarisk (Tamarix spp.) can dominate a habitat, reducing overall regeneration success by competing with tree seedlings for resources (Acácio et al., 2024). While a diverse range of diverse shrub species may enhance seedling survival by providing a more favorable microclimate, overly dominant shrubs can block sunlight and suppress regeneration. Bannister et al. (2023) showed that in temperate rainforest ecosystems, the regeneration rate of canopy species significantly increased to more than 81% five years after cutting down understorey bamboo vegetation (e.g., moso bamboo), which was dominated by seed regeneration, and the total natural regeneration increased by 55.5% (p < 0.05; incremental increase of >1 plant/m2-yr). This restoration effect stemmed from the release of light, soil nutrients and spatial resources after the removal of understory vegetation, which accelerated the natural recovery process of degraded ecosystems (Ruggirello et al., 2023). This reflects that shrubs play dual roles in forest regeneration, and their effects are highly dependent on species characteristics, ecosystem context and management strategies: in diverse multi-species forests, native shrubs significantly enhance seedling survival and regeneration potential by moderating microclimate (reduction and resource conservation) (Parra and Moreno, 2018), while invasive dominant shrubs inhibit tree regeneration in arid zones by grabbing water via deep roots and releasing chemosensory substances, and forming a mono-dominant community (Cao et al., 2020). In contrast, Bannister et al. (2023) showed that in degraded temperate rainforests caused by anthropogenic disturbances, active removal of competing bamboo vegetation released light and nutrients, activated the soil seed bank, and increased the regeneration rate of canopy species by 81%.
Meanwhile, the interaction between shrubs and trees is often species-specific, and some shrubs can serve as ecological filters that influence the succession direction. For instance, Psychotria shrubs can buffer ecological succession in forest understories, supporting natural regeneration even in high-density conditions (da Silva et al., 2021). This suggests that the influence of shrubs on natural tree regeneration also varies among forest species. There is often overlap between shrub and tree ecological niches, which can significantly influence tree growth. Additional, ecological niche differentiation between shrubs and trees promotes diversity and allows coexistence. Under adequate light conditions, a suitable shrub layer cover is favourable to seedling growth. However, under limited light conditions, shrub cover may further inhibit light availability, hampering seedling growth (Lu et al., 2021). For example, Michael B. Walters et al. found the shrub layer of crowberry (Empetrum spp.) significantly inhibited the growth of tree seedlings below 2 meters due to light competition, but this negative effect disappeared once the seedlings grew taller (Walters et al., 2020).
In conclusion, the ground layer is a critical component of the natural forest regeneration, with both positive and negative effects on seedling germination and growth. While the litter and moss layers promote moisture retention and protection, they may also create physical or chemical barriers to seedling development. Similarly, shrub cover can either facilitate or hinder regeneration, depending on species composition and light availability. Effective management of the ground cover layer is essential to balance its benefits and challenges, ensuring successful forest regeneration.
In recent years, due to climate change, anthropogenic activities and biological invasions, insect pest have been frequent in forests, especially in fir (Abies fabri) and Chinese pine (Pinus tabuliformis), serious impediments to forest regeneration and restoration (Fiala and Holusa, 2022; Xu et al., 2023). Insect pests are a type of animal disturbance that can cause a series of abnormal morphological, organisational or physiological-ecological changes in a forest tree, leading to stunted growth, reduced yield and quality, or even causing the death of the tree or the entire stand and ecological degradation (Xu et al., 2002). Since planted forests are mostly concentrated pure forests, they can provide a large number of consistent food sources and ideal habitats for pests and diseases; moreover, the low biodiversity, simple food chain and food web structure of planted forests are not conducive to the habitation of natural enemies, and their low systemic resistance makes them highly susceptible to large-scale pests and diseases, therefore, natural regeneration of planted forests will be subject to more adverse impacts than that of natural forests. In northern China, large areas of pure plantation poplar forests are commonly attacked by stem-boring and leaf-feeding pests due to their monoculture and low biodiversity (Zhang and Zhang, 2004). However, insect pest also occurs in natural forests, thus affecting the regeneration of forest trees. For example, Lieffers et al. (2024) through an in-depth survey of 33 small plots heavily infested with the insect in Alberta, Canada. In forest regeneration after infestation by mountain pine beetle (MPB, Dendroctonus ponderosae), the quality of tree regeneration was poor in the last 6–9 years, and the original dominance of the number and quality of pine seedlings were low (only 42% of the plots were found with seedlings). The dominant species of the forest may be gradually transformed during the regeneration (Lieffers et al., 2024). There are also deciduous insects (caterpillars, leaf wasp larvae, etc.) that can significantly reduce acorn production, resulting in constraints on the natural regeneration of oaks in the forests (Eötvös et al., 2023).
Some natural disasters such as earthquakes, tsunamis, volcanic eruptions, and snowstorms, although their frequency is low, can have serious impacts on regeneration when they occur. The density of young regenerated trees is negatively correlated with the basal area of surviving trees after a storm and positively correlated with the mortality of canopy trees. Canopy trees that survive storm disturbances protect understory seedlings and therefore play an important role in delaying the regeneration process (Cerioni et al., 2022). In addition, Cao et al. (2023)’s study on the renewal of Chinese gugertree (Schima superba Gardner & Champ.) after freezing disaster in Jianglangshan Nature Reserve, China, found that the mortality rate of tree sprouts increased every year for six years after being affected by freezing disaster, and the mortality rate of sprouts that sprouted earlier was relatively low, which may mean that the renewal of new sprouts that sprouted earlier. This may imply that early bud regeneration is more effective and that forest regeneration can be facilitated in forest management by retaining early vigorous buds and pruning late buds (Cao et al., 2023). And in areas with frequent snowstorms, trees also have different mechanisms to promote renewal and recovery from forest damage. A study by Nakano and Sakio (2018) in an area of heavy snowfall on Sado Island, Japan, found that young Japanese wingnut (Pterocarya rhoifolia) trees that had become established were often damaged by snow pressure, but also produced some newsprouting stems. These results suggest that in areas of heavy snowfall, water huckleberries can maintain their population size through sprouting, thus allowing the trees to recover from snow damage (Nakano and Sakio, 2018).
In recent years, significant advancements in research methodologies have greatly enhanced our understanding of the factors influencing natural forest regeneration, these methods for studying forest regeneration have been used primarily to monitor and inventory. Innovations have enabled researchers to collect data more efficiently and accurately, providing deeper insights into complex processes governing forest recovery. Several key methodological approaches have emerged, including remote sensing technologies, genetic analysis, and climate modeling.
One impactful innovation in forest regeneration research is the use of Unmanned Aerial Vehicles (UAV) for aerial surveys. UAVs equipped with high-resolution cameras allow for rapid data collection across large areas, significantly reducing the time and resources required for traditional field surveys. For example, Kitao et al. (2023) utilized UAVs to assess the survival of Abies sachalinensis seedlings in response to varying levels of solar radiation. By capturing wide-area imagery, UAVs provide detailed information on seedling distribution and canopy structure, enabling researchers to analyze regeneration patterns at a landscape scale (Kitao et al., 2023).
Light Detection and Ranging (Lidar) technology has also become a valuable tool in forest regeneration research. The innovation of Lidar technology lies in its ability to provide detailed three-dimensional data on forest landscapes efficiently and accurately, offering robust technical support for scientific research and forest management. This technology allows researchers to quantify canopy structure changes with high accuracy, providing crucial information for understanding how light conditions affect seedling establishment and growth (Markgraf et al., 2020). By integrating LiDAR with other remote sensing tools, forest managers can better monitor regeneration progress and develop tailored management strategies to promote natural recovery.
High temperature accelerate the aging of seeds and reduces their viability, while modern scholars have used genetic analysis methods such as genomics and molecular ecology, to study the mitochondria and peroxisomes in seed cells, as well as their genetic characteristics, to explore the causes of aging and thus make corresponding strategies for tree reproduction (Becerra-Vázquez et al., 2018; Kijowska-Oberc et al., 2021).
General circulation models (GCMs), which represent physical processes in the atmosphere, oceans and land, are the basis for weather and climate prediction. These models are fundamental for estimating the long-term effects of climate change on forest regeneration. Recent advancements in machine learning have further improved climate modeling capabilities. For instance, in 2024, Google researchers developed NeuralGCM, a machine learning model that surpasses traditional climate prediction models in accuracy and efficiency. This innovation holds great promise for reducing uncertainty in long-term climate forecasts, assisting researchers in anticipating extreme weather events and their impacts on forest ecosystems (Kochkov et al., 2024).
Remote sensing technologies, such as high-resolution satellite imagery and Geographic Information Systems (GIS), have revolutionized the study of terrain, soil, and climatic factors in forest regeneration. By analyzing long-term satellite data, researchers can monitor changes in forest cover and assess the effects of slope, altitude, and aspect on regeneration rates (He et al., 2018). Other scholars have used Digital Elevation altitude Models (DEMs) to access fine-scale terrain data, enabling researchers to study the effects of slope gradient and orientation on tree growth and forest recovery. These tools are particularly valuable for tracking regeneration over time and across different topographical conditions (Zhou et al., 2020).
In summary, recent advancements in research methodologies have greatly enhanced our ability to study natural forest regeneration. The integration of technologies such as UAVs, LiDAR, genomics, and climate modeling has expanded our understanding of the complex ecological processes involved in forest recovery. These technologies not only improve data collection, but also provide new opportunities for developing sustainable forest management strategies. Future research should continue to utilise these advanced techniques and explore new technologies to address the challenges posed by climate change and environmental degradation.
Current study has revealed that various ecological factors affecting natural regeneration, such as light, temperature, moisture, soil, and geography. These factors interact in complex ways, exerting integrated and stage-specific effects on tree growth. Forest communities are affected by the interactions between the factors at every growth and development stage. Understanding the intricate interactions between these environmental factors is essential for forest management. While the focus of this paper has primarily been on the effects of these factors on natural regeneration, several key considerations for forest operators emerge from the findings:
(1) Forest managers need to closely monitor and, where appropriate, artificially interfere with the natural regeneration of forests, for example, by regulating temperature conditions and ensuring that soil pH and nutrient levels remain within appropriate ranges to mitigate the adverse effects of extreme temperatures on forest regeneration. In addition, soil management is critical to ensure that soil pH and nutrient levels remain within appropriate ranges to support the growth of a wide range of tree species. Particularly in the case of temperature extremes, a balance must be struck between optimising plant growth and preventing adverse effects of temperature on soil and plants.
(2) When designing forest management strategies, the different responses of different tree species to climate change and environmental stresses should be taken into account. Selecting climate-resilient species and developing species-specific management practices can enhance the resilience of forest ecosystems to climate change.
(3) Long-term monitoring and environmental data collection should be strengthened so that management measures can be adjusted in a timely manner to cope with the uncertainties and challenges posed by climate change.
(4) According to the climatic and environmental conditions of each region, it is necessary to cultivation and promotion of natural regeneration of tree species suitable for survival in these environmental conditions are selected through measures such as nurturing. This approach enhances forests’ roles in improving the terrain, conserving water, and supporting biodiversity. For example, the introduction of drought-tolerant species and adjustments to management practices are necessary to cope with the challenges of climate change. The selection of appropriate species and management methods is crucial for ensuring the long-term sustainability of forest ecosystems.
This review provides a comprehensive examination of the environmental factors affecting natural forest regeneration, highlighting the importance of integrating these factors into forest management strategies. While substantial progress has been made in understanding how these factors interact to shape regeneration processes, there are still gaps in the research. For example, relatively few studies have explored the impact of wind speed on regeneration, and most research has focused on seed-based regeneration, with less attention given to coppicing regeneration. Additionally, there is a lack of predictive ecological models that can forecast how forest regeneration will respond to future climate change. At the same time, this study did not focus on a particular study area to review the natural regeneration of forests, and future articles can focus on a particular temperature zone or region to conduct more detailed studies, which will be more conducive to the development of forest regeneration research.
To effectively manage and promote forest regeneration, it is crucial to consider factors such as light, temperature, moisture, soil and geography in an integrated manner, interactions between multiple factors cannot be ignored, and recent studies such as Wang et al. (2022) quantified the interactive effects of light-water-nutrients on seedling survival through structural equation modelling, and found that the negative effect of light intensity was enhanced by 200% under drought conditions. This confirmed the non-linear relationship between the factors. System dynamics modelling or network analysis can be introduced in future studies to reveal more about the synergistic effects of environmental factors and their nonlinear effects on forest regeneration. Meanwhile, bibliometric analyses showed that the research hotspots gradually shifted to epigenetic and transgenerational adaptation mechanisms from 2012 to 2021. For example, the proportion of studies on the regulation of seed germination by DNA methylation increased from 5 to 18% (Yan et al., 2024), and the exploration of the influence mechanisms of these factors and the influence of genes behind the species should be increased in the future as well, and more attention should be paid to this transgenerational transmission mechanism of gene–environment interactions.
In addition, it is also necessary to expand the scale of natural regeneration research from populations to communities and entire ecosystems. As global climate conditions continuously change, regeneration patterns obtained today may no longer be applicable to future forestry development. For example, in the central Himalayan treeline, fir is advancing upwards more rapidly than birch, and birch regeneration has declined significantly. This suggests that fir is expected to surpass birch as the dominant species along the treeline in the central Himalayas, which will lead to alterations in the species assemblage near the treeline (Sigdel et al., 2024). Therefore, climate models should be established to anticipate these changes and give appropriate response strategies in advance. In the context of global change, this integrated understanding is essential for the development of sustainable forest management and ecological restoration strategies.
JL: Writing – original draft, Writing – review & editing.
The author(s) declare that no financial support was received for the research and/or publication of this article.
The author declares that the research was conducted in the absence of any commercial or financial relationships that could be construed as a potential conflict of interest.
The author(s) declare that no Gen AI was used in the creation of this manuscript.
All claims expressed in this article are solely those of the authors and do not necessarily represent those of their affiliated organizations, or those of the publisher, the editors and the reviewers. Any product that may be evaluated in this article, or claim that may be made by its manufacturer, is not guaranteed or endorsed by the publisher.
Acácio, V., Dias, F. S., Ferreira, M., Rodriguez-González, P. M., Matias, H., and Caldeira, M. C. (2024). Effects of shrub species dominance and diversity on oak seedling survival in Mediterranean woodlands: the interplay of abiotic conditions and plant traits. For. Ecol. Manag. 555:121713. doi: 10.1016/j.foreco.2024.121713
Ali, A., Dai, D., Akhtar, K., Teng, M., Yan, Z., Urbina-Cardona, N., et al. (2019). Response of understory vegetation, tree regeneration, and soil quality to manipulated stand density in a Pinus massoniana plantation. Glob. Ecol. Conserv. 20:e00775. doi: 10.1016/j.gecco.2019.e00775
Asplund, J., Hustoft, E., Nybakken, L., Ohlson, M., and Lie, M. H. (2018). Litter impair spruce seedling emergence in beech forests: a litter manipulation experiment. Scand. J. For. Res. 33, 332–337. doi: 10.1080/02827581.2017.1388440
Azaryan, M., Vajari, K. A., and Amanzadeh, B. (2022). Soil properties and fine root morphological traits in relation to soil particle-size fractions in a broad-leaved beech (Fagus orientalis Lipsky) forest. Acta Oecologica-Int. J. Ecol. 117:103852. doi: 10.1016/j.actao.2022.103852
Bayat, H., Rastgo, M., Zadeh, M. M., and Vereecken, H. (2015). Particle size distribution models, their characteristics and fitting capability. J. Hydrol. 529, 872–889. doi: 10.1016/j.jhydrol.2015.08.067
Becerra-Vázquez, A. G., Sánchez-Nieto, S., Coates, R., Flores-Ortiz, C. M., and Orozco-Segovia, A. (2018). Seed longevity of five tropical species from south-eastern Mexico: changes in seed germination during storage. Tropical Conserv. Sci. 11, 1–17. doi: 10.1177/1940082918779489
Bellingham, P. J., and Sparrow, A. D. (2000). Resprouting as a life history strategy in Woody Plant communities. Oikos 89, 409–416. doi: 10.1034/j.1600-0706.2000.890224.x
Bond, W. J., and Midgley, J. J. (2001). Ecology of sprouting in woody plants: the persistence niche. Trends in ecology and evolution. Trends Ecol. Evol. 16, 45–51. doi: 10.1016/S0169-5347(00)02033-4
Bond, W. J., and Midgley, J. J. (2003). Evolution of functional traits in plants || the evolutionary ecology of sprouting in Woody plants. Int. J. Plant Sci. 164, S103–S114. doi: 10.1086/374191
Bond, W. J., Woodward, F. I., and Midgley, G. F. (2005). The global distribution of ecosystems in a world without fire. New Phytol. 165, 525–538. doi: 10.1111/j.1469-8137.2004.01252.x
Bose, A. K., Weiskittel, A., Wagner, R. G., and Kuehne, C. (2016). Assessing the factors influencing natural regeneration patterns in the diverse, multi-cohort, and managed forests of Maine, USA. J. Veg. Sci. 27, 1140–1150. doi: 10.1111/jvs.12433
Calviño-Cancela, M., Lorenzo, P., and González, L. (2018). Fire increases Eucalyptus globulus seedling recruitment in forested habitats: effects of litter, shade and burnt soil on seedling emergence and survival. For. Ecol. Manag. 409, 826–834. doi: 10.1016/j.foreco.2017.12.018
Cao, J. Y., Liu, J. F., Yuan, Q., Xu, D. Y., Fan, H., Chen, H., et al. (2020). Traits of shrubs in forests and bushes reveal different life strategies. Chin. J. Plant Ecol. 44, 715–729. doi: 10.17521/cjpe.2020.0024
Cao, Y. H., Zhou, B. Z., and Wang, X. M. (2023). A 6-year study on the mortality dynamics of sprouts germinated on Schima superba after a severe ice storm in southern China. Front. Plant Sci. 14. doi: 10.3389/fpls.2023.1178007
Caplat, P., and Anand, M. (2009). Effects of disturbance frequency, species traits and resprouting on directional succession in an individual-based model of forest dynamics. J. Ecol. 97, 1028–1036. doi: 10.1111/j.1365-2745.2009.01541.x
Cerioni, M., Fidej, G., Diaci, J., and Nagel, T. A. (2022). Dynamics and drivers of post-windthrow recovery in managed mixed mountain forests of Slovenia. Eur. J. For. Res. 141, 821–832. doi: 10.1007/s10342-022-01475-3
Change IPCC Climate (2022). “Change, climate change” in Impacts, adaptation and vulnerability (New York: Cambridge University Press), 2022.
Chazdon, R. L. (2008). Beyond deforestation: restoring forests and ecosystem services on degraded lands. Science 320, 1458–1460. doi: 10.1126/science.1155365
C.o. W.G.I.C.t. the, F.A.R.o. the, and I.P.o.C (2014). “Change, climate change” in Mitigation of climate change (New York: Cambridge University Press), 2014.
da Silva, J. C. C., Rovedder, A. P. M., Capitani, L. C., Schenato, R. B., Neuenschwander, F., Peccatti, A., et al. (2021). Does the high density of Psychotria shrubs in the understory influence the natural regeneration of trees? Ecol. Eng. 172:106401. doi: 10.1016/j.ecoleng.2021.106401
Danilov, D. A., Anisimova, I. M., Belyaeva, N. V., and Kazi, I. A. (2020). Post-fire restoration of tree species in various soil conditions after surface fires zone. Earth Environ. Sci. 574:012019. doi: 10.1088/1755-1315/574/1/012019
De Frutos, S., Bravo-Fernández, J. A., Roig-Gómez, S., Del Río, M., and Ruiz-Peinado, R. (2022). Natural regeneration and species diversification after seed-tree method cutting in a maritime pine reforestation. Iforest-Biogeosci. Forestry 15, 500–508. doi: 10.3832/ifor4088-015
De Lombaerde, E., Blondeel, H., Baeten, L., Landuyt, D., Perring, M. P., Depauw, L., et al. (2020). Light, temperature and understorey cover predominantly affect early life stages of tree seedlings in a multifactorial mesocosm experiment. For. Ecol. Manag. 461:117907. doi: 10.1016/j.foreco.2020.117907
de Medeiros, E. V., Duda, G. P., dos Santos, L. A. R., Lima, J. R. D., de Almeida-Cortéz, J. S., Hammecker, C., et al. (2017). Soil organic carbon, microbial biomass and enzyme activities responses to natural regeneration in a tropical dry region in Northeast Brazil. Catena 151, 137–146. doi: 10.1016/j.catena.2016.12.012
Ding, Y., Liang, S., and Peng, S. (2019). Climate change affects Forest productivity in a typical climate transition region of China. Sustain. For. 11:2856. doi: 10.3390/su11102856
Djiofack, B. Y., Beeckman, H., Bourland, N., Belanganayi, B. L., Laurent, F., Ilondea, B. A., et al. (2024). Natural forest regeneration through fire protection is a less imminent threat for truly stable savannas than afforestation. Glob. Chang. Biol. 30. doi: 10.1111/gcb.17370
Dyderski, M. K., Gazda, A., Hachulka, M., Horodecki, P., Kalucka, I. L., Kamczyc, J., et al. (2018). Impacts of soil conditions and light availability on natural regeneration of Norway spruce Picea abies (L.) H. Karst. In low-elevation mountain forests. Ann. For. Sci. 75:91. doi: 10.1007/s13595-018-0775-x
Enríquez-de-Salamanca, A. (2022). Effects of climate change on Forest regeneration in Central Spain. Atmos. 13:1143. doi: 10.3390/atmos13071143
Eötvös, C. B., Fürjes-Mikó, Á., Paulin, M., Gáspár, C., Kárpáti, M., Hirka, A., et al. (2023). Enhanced natural regeneration potential of sessile oak in northern Hungary: role of artificially increased density of insectivorous birds. Forests 14:1548. doi: 10.3390/f14081548
Escandon, A. B., Paula, S., and Saldaa, A. (2020). Root suckering promotes recruitment in two temperate rainforest trees with contrasting shade tolerance. Perspect. Plant Ecol. Evol. Syst. 44:125531. doi: 10.1016/j.ppees.2020.125531
Facelli, J. M., and Facelli, E. (1993). Interactions after death: plant litter controls priority effects in a successional plant community. Oecologia 95, 277–282. doi: 10.1007/BF00323500
FAO (2024). The state of the world’s forests 2024: forest-sector innovations towards a more sustainable future. Rome, Italy: Food and agriculture Organization of the United Nations.
Feng, H. (2012). Study on sprout characteristics of 15 species of Evergreen broad-leaved trees in Jinyun Mountain of Chongqing : Southwest University.
Fernandes, P., Máguas, C., and Correia, O. (2017). Combined effects of climate, habitat, and disturbance on seedling establishment of Pinus pinaster and Eucalyptus globulus. Plant Ecol. 218, 501–515. doi: 10.1007/s11258-017-0706-1
Fiala, T., and Holusa, J. (2022). Polydrusus aeratus (Coleoptera: Curculionidae: Entiminae): a potential pest of young coniferous stands. Central Europ. Forest. J. 68, 36–42. doi: 10.2478/forj-2021-0014
Fisichelli, N., Wright, A., Rice, K., Mau, A., Buschena, C., and Reich, P. B. (2014). First-year seedlings and climate change: species-specific responses of 15 north American tree species. Oikos 123, 1331–1340. doi: 10.1111/oik.01349
Ganzhorn, J. U., Fietz, J., Rakotovao, E., Schwab, D., and Zinner, D. (1999). Lemurs and the regeneration of dry deciduous forest in Madagascar. Conserv. Biol. 13, 794–804. doi: 10.1046/j.1523-1739.1999.98245.x
Guariguata, M. R., and Pinard, M. A. (1998). Ecological knowledge of regeneration from seed in neotropical forest trees: implications for natural forest management. For. Ecol. Manag. 112, 87–99. doi: 10.1016/S0378-1127(98)00318-1
Harrington, C. A., and Gould, P. J. (2015). Tradeoffs between chilling and forcing in satisfying dormancy requirements for Pacific northwest tree species. Front. Plant 6. doi: 10.3389/fpls.2015.00120
He, X. Y., Ren, C. Y., Chen, L., Wang, Z. M., and Zheng, H. F. (2018). The Progress of Forest ecosystems monitoring with remote sensing techniques. Sci. Geogr. Sin. 38, 997–1011. doi: 10.13249/j.cnki.sgs.2018.07.001
He, Z. S., Wang, L. J., Jiang, L., Wang, Z., Liu, J. F., Xu, D. W., et al. (2019). Effect of microenvironment on species distribution patterns in the regeneration layer of Forest gaps and non-gaps in a subtropical natural Forest, China. Forests 10:90. doi: 10.3390/f10020090
Heimann, M., and Reichstein, M. (2008). Terrestrial ecosystem carbon dynamics and climate feedbacks. Nature 451, 289–292. doi: 10.1038/nature06591
Hishe, H., Giday, K., Fremout, T., Negussie, A., Aerts, R., and Muys, B. (2021). Environmental and anthropogenic factors affecting natural regeneration of degraded dry Afromontane forest. Restor. Ecol. 29:e13471. doi: 10.1111/rec.13471
Holl, K. D., and Aide, T. M. (2011). When and where to actively restore ecosystems? Forest Ecol. Manag. 261, 1558–1563. doi: 10.1016/j.foreco.2010.07.004
Horneck, D., Sullivan, D., Owen, J. Jr, and Hart, J., Soil test interpretation guide, Oregon State University extension, Environmental Science. (2011).
Howe, H. F., and Smallwood, J. (1982). Ecology of seed dispersal. Annu. Rev. Ecol. Syst. 13, 201–228. doi: 10.1146/annurev.es.13.110182.001221
Inbar, A., Trouvé, R., Benyon, R. G., Lane, P. N. J., and Sheridan, G. J. (2022). Long-term hydrological response emerges from forest self-thinning behaviour and tree sapwood allometry. Sci. Total Environ. 852:158410. doi: 10.1016/j.scitotenv.2022.158410
Jiang, X. Y., Wang, H. Y., Dong, J., and Zhou, Z. X. (2020). Study on the effect of compaction degree on hydrological effect of vegetation soil. Yellow River 42, 86–90.
Jones, M. W., Abatzoglou, J. T., Veraverbeke, S., Andela, N., Lasslop, G., Forkel, M., et al. (2022). Global and regional trends and drivers of fire under climate change. Rev. Geophys. 60. doi: 10.1029/2020RG000726
Juntunen, V., and Neuvonen, S. (2006). Natural regeneration of scots pine and Norway spruce close to the timberline in northern Finland. Silva Fennica 40, 443–458. doi: 10.14214/sf.329
Kassa, G., Molla, E., and Abiyu, A. (2020). Effects of Eucalyptus tree plantations on soil seed bank and soil physicochemical properties of Qimbaba forest. Cogent Food Agric. 5:1711297. doi: 10.1080/23311932.2019.1711297
Khaine, I., Woo, S. Y., Kwak, M., Lee, S. H., Je, S. M., You, H., et al. (2018). Factors affecting natural regeneration of tropical forests across a precipitation gradient in Myanmar. Forests 9. doi: 10.3390/f9030143
Kijowska-Oberc, J., Staszak, A. M., and Ratajczak, E. (2021). Climate change affects seed aging? Initiation mechanism and consequences of loss of forest tree seed viability. Trees-Struct. Funct. 35, 1099–1108. doi: 10.1007/s00468-020-02072-w
Kitao, M., Harayama, H., Furuya, N., Agathokleous, E., and Ishibashi, S. (2023). Regeneration of forest floor-grown seedlings of Sakhalin fir can be promoted through shading by shelter trees. J. For. Res. 29, 54–61. doi: 10.1080/13416979.2023.2246753
Kitao, M., Harayama, H., Yazaki, K., Tobita, H., Agathokleous, E., Furuya, N., et al. (2022). Photosynthetic and growth responses in a Pioneer tree (Japanese white birch) and competitive perennial weeds (Eupatorium sp.) grown under different regimes with limited water supply to waterlogging. Front. Plant Sci. 13:835068. doi: 10.3389/fpls.2022.835068
Kitayama, K., Majalap-Lee, N., and Aiba, S. (2000). Soil phosphorus fractionation and phosphorus-use efficiencies of tropical rainforests along altitudinal gradients of mount Kinabalu, Borneo. Oecologia 123, 342–349. doi: 10.1007/s004420051020
Klimesová, J., and Klime, L. (2007). Bud banks and their role in vegetative regeneration – a literature review and proposal for simple classification and assessment. Perspect. Plant Ecol. Evol. Syst. 8, 115–129. doi: 10.1016/j.ppees.2006.10.002
Knapp, B. O., Olson, M. G., and Dey, D. C. (2017). Early stump sprout development after two levels of harvest in a Midwestern bottomland hardwood Forest. For. Sci. 63, 377–387. doi: 10.5849/FS-2016-029R2
Kochkov, D., Yuval, J., Langmore, I., Norgaard, P., Smith, J., Mooers, G., et al. (2024). Neural general circulation models for weather and climate. Nature 632, 1060–1066. doi: 10.1038/s41586-024-07744-y
Koskela, J. (2008). Responses of gas exchange and growth in Merkus pine seedlings to expected climatic changes in Thailand. Glob. Chang. Biol. 7, 641–656. doi: 10.1046/j.1354-1013.2000.00394.x
Krawchuk, M. A., Moritz, M. A., Parisien, M. A., Van Dorn, J., and Hayhoe, K. (2009). Global Pyrogeography: the current and future distribution of wildfire. PLoS One 4. doi: 10.1371/journal.pone.0005102
Li, H.. (2018). Fractal dimension of soil particles under different plant communities in mountainous area, 2018 International Conference on Advanced Electronic Materials, Computers and Materials Engineering (AEMCME 2018). 439.
Li, J. W., Nie, S. Q., and An, B. H. (2005). Stump sprouting of the Main broad-leaved tree species of secondary Forestin eastern area of Norheast China. Sci. SilvaeI Sinicae 41, 72–77. doi: CNKI:SUN:LYKE.0.2005-06-010
Li, H. R., Wang, L. Z., Wei, C. L., Liu, X. S., and Hu, L. L. (2016). Effeet of medium strength burning on natural regeneration of Larix gmelinii plantation. Prot. Forest Sci. Technol. 9, 47–48.
Li, H. J., and Zhang, Z. B. (2001). Relationship between animals and plant regeneration by seed II. Seed predation dispersal and burial by animals and relationship bet ween animals and seedling establishment. Biodivers. Sci. 9, 25–37.
Liang, W. J., and Wei, X. (2020). Factors promoting the natural regeneration of Larix principis rupprechtii plantation in the Lvliang Mountains of Central China. Peerj 8:e9339. doi: 10.7717/peerj.9339
Lieffers, V. J., Benedik, J., Stadt, K., and Macdonald, S. E. (2024). Poor regeneration of pine after mountain pine beetle attack in colder boreal regions of Canada. Can. J. For. Res. 54, 168–191. doi: 10.1139/cjfr-2023-0113
Liu, J. B., Chen, J., Chen, G. S., Guo, J. F., and Li, Y. Q. (2020). Enzyme stoichiometry indicates the variation of microbial nutrient requirements at different soil depths in subtropical forests. PLoS One 15:e0220599. doi: 10.1371/journal.pone.0220599
Liu, Q. Q., Huang, Z. J., Guo, S., Wang, D. Y., Wang, C. H., Wang, Z. N., et al. (2019). Responses of seed germination and seedling growth of Cunninghamia lanceolata and Schima superba to different light intensities. Chin. J. Appl. Ecol. 30, 2955–2963. doi: 10.13287/j.1001-9332.201909.007
Liu, S., Luo, D., Cheng, R. M., Yang, H. G., Wu, J. M., and Shi, Z. M. (2020). Soil-atmosphere exchange of greenhouse gases from typical subalpine forests on the eastern Qinghai-Tibetan plateau: effects of forest regeneration patterns. Land Degrad. Dev. 31, 2019–2032. doi: 10.1002/ldr.3586
Liu, X. D., Wu, Q. X., and Zhao, H. Y. (1994). The vertical interception function of Forest vegetation and soil and water conservation. Res Soil Water Conserv., 8–13.
Liu, X. P., Zhang, W. J., Yang, F., Zhou, X., Liu, Z. J., Qu, F., et al. (2012). Changes in vegetation-environment relationships over long-term natural restoration process in middle Taihang Mountain of North China. Ecol. Eng. 49, 193–200. doi: 10.1016/j.ecoleng.2012.06.040
Loeb, R. E., and Mao, H. (2021). Natural Forest regeneration-soil relationships in a fire disturbed urban natural area Forest. Urban For. Urban Green. 59:126993. doi: 10.1016/j.ufug.2021.126993
Lu, X. H., Xu, N., Chen, Y., Li, Y., and Gan, X. H. (2021). Effects of light intensity and ground cover on seedling regeneration of Tetracentron sinense Oliv. J. Plant Growth Regul. 40, 736–748. doi: 10.1007/s00344-020-10137-w
Lucas Borja, M. E. (2014). Climate change and Forest natural regeneration in Mediterranean Mountain areas. For. Res. 3. doi: 10.4172/2168-9776.1000e108
Luo, W. J., Wang, Y. J., and Wang, L. H. (2023). Irradiation and nitrogen regulate growth and physiology in Horsfieldia hainanensis seedlings. Biol. Plant. 67, 19–27. doi: 10.32615/bp.2022.038
Ma, W., Liang, J., Cumming, J. R., Lee, E., Welsh, A. B., Watson, J. V., et al. (2016). Fundamental shifts of central hardwood forests under climate change. Ecol. Model. 332, 28–41. doi: 10.1016/j.ecolmodel.2016.03.021
Machado, D. L., Engel, V. L., Podadera, D. S., Sato, L. M., de Goede, R. G. M., de Moraes, L. F. D., et al. (2021). Site and plant community parameters drive the effect of vegetation on litterfall and nutrient inputs in restored tropical forests. Plant Soil 464, 405–421. doi: 10.1007/s11104-021-04964-3
Maciel-Nájera, J. F., Hernández-Velasco, J., Socorro González-Elizondo, M., Ciro Hernández-Díaz, J., López-Sánchez, C. A., Antúnez, P., et al. (2020). Unexpected spatial patterns of natural regeneration in typical uneven-aged mixed pine-oak forests in the Sierra Madre occidental, Mexico. Global Ecol. Conserv. 23:e01074. doi: 10.1016/j.gecco.2020.e01074
Markgraf, R., Doyon, F., and Kneeshaw, D. (2020). Forest landscape heterogeneity increases shrub diversity at the expense of tree seedling diversity in temperate Mixedwood forests. Forests 11. doi: 10.3390/f11020160
Martinez Pastur, G. J., Peri, P. L., Cellini, J. M., Lencinas, M. V., Barrera, M., and Ivancich, H. (2011). Canopy structure analysis for estimating forest regeneration dynamics and growth in Nothofagus pumilio forests. Ann. For. Sci. 68, 587–594. doi: 10.1007/s13595-011-0059-1
Massaccesi, L., De Feudis, M., Leccese, A., and Agnelli, A. (2020). Altitude and vegetation affect soil organic carbon, basal respiration and microbial biomass in Apennine Forest soils. Forests 11:710. doi: 10.3390/f11060710
Mc Carthy, R., Ekö, P. M., and Rytter, L. (2014). Reliability of stump sprouting as a regeneration method for poplars: clonal behavior in survival, sprout straightness and growth. Silva Fennica 48:1126. doi: 10.14214/sf.1126
Miles, L., and Kapos, V. (2008). Reducing greenhouse gas emissions from deforestation and forest degradation: global land-use implications. Science 320, 1454–1455. doi: 10.1126/science.1155358
Mueller-Dombois, D. (1992). Potential effects of the increase in carbon dioxide and climate change on the dynamics of vegetation. Water Air Soil Pollut. 64, 61–79. doi: 10.1007/BF00477096
Nakano, Y., and Sakio, H. (2018). The regeneration mechanisms of a Pterocarya rhoifolia population in a heavy snowfall region of Japan. Plant Ecol. 219, 1387–1398. doi: 10.1007/s11258-018-0888-1
Niemczyk, M., Thomas, B. R., and Jastrzebowski, S. (2023). Strategies for difficult times: physiological and morphological responses to drought stress in seedlings of central European tree species. Trees-Struct. Funct. 37, 1657–1669. doi: 10.1007/s00468-023-02450-0
Niu, Y. J., Liang, W. J., Wei, X., and Han, Y. Z. (2022). Factors affecting the natural regeneration of the Larix principis-rupprechtii Mayr plantations: evidence from the composition and co-occurrence network structure of soil bacterial communities. PRO 10:1771. doi: 10.3390/pr10091771
Nogueira, L. R., da Silva, C. F., Pereira, M. G., Gaia-Gomes, J. H., and Ribeiro da Silva, E. M. (2016). Biological properties and organic matter dynamics of soil in pasture and natural regeneration areas in the Atlantic Forest biome. Revista Brasileira De Ciencia Do Solo 40:e0150366. doi: 10.1590/18069657rbcs20150366
Ollerton, J., Winfree, R., and Tarrant, S. (2011). How many flowering plants are pollinated by animals? Oikos 120, 321–326. doi: 10.1111/j.1600-0706.2010.18644.x
Orman, O., Adamus, M., and Foremnik, K. (2023). Norway spruce sapling plasticity in their responses of architecture and growth to light gradient decreases with altitude in subalpine stands. For. Ecol. Manag. 536:120898. doi: 10.1016/j.foreco.2023.120898
Parra, A., and Moreno, J. M. (2018). Drought differentially affects the post-fire dynamics of seeders and resprouters in a Mediterranean shrubland. Sci. Total Environ. 626, 1219–1229. doi: 10.1016/j.scitotenv.2018.01.174
Pastur, G. M., Lencinas, M. V., Peri, P., and Cellini, J. M. (2008). Flowering and seeding patterns in unmanaged and managed Nothofagus pumilio forests with a silvicultural variable retention system. Forstarchiv 79, 60–65. doi: 10.23760300-4112-79-60
Petrou, P., and Milios, E. (2020). Investigation of the factors affecting artificial seed sowing success and seedling survival in Pinus brutia natural stands in middle elevations of Central Cyprus. Forests 11:1349. doi: 10.3390/f11121349
Pham, V. V., Ammer, C., Annighofer, P., and Heinrichs, S. (2022). Tree regeneration characteristics in limestone forests of the cat Ba National Park, Vietnam. Bmc Ecology and Evolution 22:6. doi: 10.1186/s12862-021-01957-9
Pietsch, S. A., Hasenauer, H., Kucera, J., and Cermák, J. (2003). Modeling effects of hydrological changes on the carbon and nitrogen balance of oak in floodplains. Tree Physiol. 23, 735–746
Pressler, Y., Zhou, J., He, Z., Van Nostrand, J. D., and Smith, A. P. (2020). Post-agricultural tropical forest regeneration shifts soil microbial functional potential for carbon and nutrient cycling. Soil Biol. Biochem. 145:107784. doi: 10.1016/j.soilbio.2020.107784
Principe, A., Matos, P., Sarris, D., Gaiola, G., do Rosario, L., Correia, O., et al. (2019). In Mediterranean drylands microclimate affects more tree seedlings than adult trees. Ecol. Indic. 106:105476. doi: 10.1016/j.ecolind.2019.105476
Qi, X., Treydte, K., Saurer, M., Fang, K. Y., An, W. L., Lehmann, M., et al. (2024). Contrasting water-use strategies to climate warming in white birch and larch in a boreal permafrost region. Tree Physiol. 44:tpae053. doi: 10.1093/treephys/tpae053
Qiu, T., Sharma, S., Woodall, C. W., and Clark, J. S. (2021). Niche shifts from trees to fecundity to recruitment that determine species response to climate change. Front. Ecol. Evol. 9:719141. doi: 10.3389/fevo.2021.719141
Regal, P. J. (1982). Pollination by wind and animals: ecology of geographic patterns. Annu. Rev. Ecol. Syst. 13, 497–524. doi: 10.1146/annurev.es.13.110182.002433
Ricklefs, R. E., Latham, R. E., and Qian, H. (1999). Global patterns of tree species richness in moist forests: distinguishing ecological influences and historical contingency. Oikos 86, 369–373. doi: 10.2307/3546454
Rodriguez-Garcia, E., Gratzer, G., and Bravo, F. (2011). Climatic variability and other site factor influences on natural regeneration of Pinus pinaster Ait. In Mediterranean forests. Ann. For. Sci. 68, 811–823. doi: 10.1007/s13595-011-0078-y
Ruan, D. Z., Chen, L., Zhang, W. J., Xu, B. D., Yang, D. J., and Wang, Q. H. (2011). Research Progress of Forest fire disturbance. For. Invent. Plan. 36, 63–66.
Ruggirello, M. J., Bustamante, G. N., and Soler, R. M. (2023). Nothofagus pumilio regeneration failure following wildfire in the sub-Antarctic forests of Tierra del Fuego, Argentina. Forestry 98, 40–49. doi: 10.1093/forestry/cpad028
Santelices-Moya, R., Promis, A., Peralta-Aguilera, M., and Cabrera Ariza, A. M. (2024). Effect of Silvicultural treatments on Nothofagus glauca forests regarding canopy structure patterns, Solar Radiation Transmission, and Tree Regeneration. Forests 15. doi: 10.3390/f15010134
Scariot, A. (2010). Seedling mortality by Litterfall in Amazonian Forest fragments 1. Biotropica 32, 662–669. doi: 10.1646/0006-3606(2000)032[0662:SMBLIA]2.0.CO;2
Schuele, M., Domes, G., Schwanitz, C., and Heinken, T. (2023). Early natural tree regeneration after wildfire in a central European scots pine forest: Forest management, fire severity and distance matters. For. Ecol. Manag. 539:120999. doi: 10.1016/j.foreco.2023.120999
See, C. R., Yanai, R. D., Fisk, M. C., Vadeboncoeur, M. A., Quintero, B. A., and Fahey, T. J. (2015). Soil nitrogen affects phosphorus recycling: foliar resorption and plant–soil feedbacks in a northern hardwood Forest. Ecology 96, 2488–2498. doi: 10.1890/15-0188.1
Sigdel, S. R., Zheng, X. Y., Babst, F., Camarero, J. J., Gao, S., Li, X. X., et al. (2024). Accelerated succession in Himalayan alpine treelines under climatic warming. Nat. Plants 10, 1909–1918. doi: 10.1038/s41477-024-01855-0
Sofo, A., Ciarfaglia, A., Scopa, A., Camele, I., Curci, M., Crecchio, C., et al. (2014). Soil microbial diversity and activity in a Mediterranean olive orchard using sustainable agricultural practices. Soil Use Manag. 30, 160–167. doi: 10.1111/sum.12097
Sofo, A., Nuzzo, V., Tataranni, G., Manfra, M., De Nisco, M., and Scopa, A. (2012). Berry morphology and composition in irrigated and non-irrigated grapevine (Vitis vinifera L.). J. Plant Physiol. 169, 1023–1031. doi: 10.1016/j.jplph.2012.03.007
Sun, C. Z., Huang, B. L., Chen, H. B., Liu, Z. W., and Wen, Z. M. (1998). Interaction between soil water conditions and different kinds of artificial plant cover in the loess plateau. J. Bijing Forest. Univ. 20, 7–14. doi: 10.1017/S0266078400010713
Tesfaye, K., Mamo, G., Debela, S., Tadesse, M., Bizuneh, A., Debele, B., et al. (2015). Ethiopian panel on climate change first assessment report, agriculture and food security (working group II). Geneva: Intergovernmental Panel on Climate Change.
Thrippleton, T., Bugmann, H., Kramer-Priewasser, K., and Snell, R. S. (2016). Herbaceous Understorey: An overlooked player in Forest landscape dynamics? Ecosystems 19, 1240–1254. doi: 10.1007/s10021-016-9999-5
Tiebel, K., Dahlmann, J., and Karge, A. (2023). Global warming could shorten the seed lifespan of Pioneer tree species and thus natural regeneration window of damaged areas. Eur. J. For. Res. 143, 437–450. doi: 10.1007/s10342-023-01633-1
Toledo, M., Poorter, L., Peña‐Claros, M., Alarcón, A., Balcázar, J., Leaño, C., et al. (2011). Climate is a stronger driver of tree and Forest growth rates than soil and disturbance RID C-8951-2009. J. Ecol. 99, 254–264. doi: 10.1111/j.1365-2745.2010.01741.x
Tredici, P. D. (2001). Sprouting in temperate trees: a morphological and ecological review. Bot. Rev. 67, 121–140. doi: 10.1007/BF02858075
Tuinenburg, O. A., Bosmans, J. H. C., and Staal, A. (2022). The global potential of Forest restoration for drought mitigation. Environ. Res. Lett. 17:034045. doi: 10.1088/1748-9326/ac55b8
Turczanski, K., Dyderski, M. K., Rutkowski, P., and Dieback, A. (2021). Soil and deer browsing influence natural regeneration of European ash (Fraxinus excelsior L.). Sci. Total Environ. 752:141787. doi: 10.1016/j.scitotenv.2020.141787
Voropay, N. N., Atutova, Z. V., and Shuklina, E. S. (2022). Long-term soil temperature dynamics in Pyrogenically transformed geosystems of the Tunka depression (southwestern Baikalia). Geogr. Nat. Resour. 43, 163–174. doi: 10.1134/S1875372822020123
Walters, M. B., Farinosi, E. J., and Willis, J. L. (2020). Deer browsing and shrub competition set sapling recruitment height and interact with light to shape recruitment niches for temperate Forest tree species. For. Ecol. Manag. 467:118134. doi: 10.1016/j.foreco.2020.118134
Wang, Y. F. (2015). Effect of temperature on the mechanism of plant seed germination. Protect. Forest Sci. Technol., 76–78. doi: 10.13601/j.issn.1005-5215.2015.06.029
Wang, X., Chen, Y., and Smith, M. L. (2022). Drought exacerbates the negative effects of high light intensity on seedling survival by amplifying nutrient limitations: a structural equation modeling approach. New Phytol. 235, 987–1002. doi: 10.1111/nph.18245
Wang, H. C. Y., Gao, Z. L., Cao, Y. F., Yu, W. T., Wang, Q. H., Zhou, R. L., et al. (2023). Response mechanism of Forest fire combustion products affecting plant seed germination. World Forest. Res. 36, 47–53. doi: 10.13348/j.cnki.sjlyyj.2023.0044.y
Wang, R. H., Hu, R. G., Wu, Y. P., Shaaban, M., Zhang, T., Pan, G., et al. (2024). Elevation-dependent natural regeneration of Abies georgei var. smithii Forest in southeastern Tibet. Forests 15:142. doi: 10.3390/f15010142
Wang, Z. Z., Qin, K. R., Fang, W., and Wang, H. Y. (2024). Neighborhood competition and understory-associated vegetation are important factors influencing the natural regeneration of Subtropical Mountain forests. Forests 15:1017. doi: 10.3390/f15061017
Wang, J. M., Wang, H. D., Cao, Y. G., Bai, Z. K., and Qin, Q. (2016). Effects of soil and topographic factors on vegetation restoration in opencast coal mine dumps located in a loess area. Sci. Rep. 6:22058. doi: 10.1038/srep22058
Wani, S. A., Mugloo, J. A., Pala, N. A., Malik, Z. A., Khan, P. A., Masoodi, T. H., et al. (2022). Regeneration status and soil site characteristics of spruce (Picea smithiana wall. Boiss) dominated Forest along altitudinal gradient in north Indian Himalaya. Acta Ecol. Sin. 43, 487–497. doi: 10.1016/j.chnaes.2022.03.005
Wardle, J. (1970). The ecology of Nothofagus solandri 3: regeneration. N. Z. J. Bot. 8, 571–608. doi: 10.1080/0028825X.1970.10430161
Wheeler, J. A., Hermanutz, L., and Marino, P. M. (2011). Feathermoss seedbeds facilitate black spruce seedling recruitment in the Forest-tundra ecotone (Labrador, Canada). Oikos 120, 1263–1271. doi: 10.1111/j.1600-0706.2010.18966.x
Willis, J. L., Schnake, D. K., DePerno, C. S., Lashley, M. A., Wetzstein, B., and Yow, J. (2021). Tree encroachment impacts on seed predator selection and seedling establishment in degraded pine woodlands. Appl. Veg. Sci. 24:e12570. doi: 10.1111/avsc.12570
Xiong, Z. Q., Sun, J. W., Zhong, P., Liang, L. X., Dang, H. X., and Wang, G. J. (2023). The impact of natural regeneration of Phoebe bournei in Anfu County, Jiangxi Province, on community diversity and soil nutrient characteristics. Forests 14:1783. doi: 10.3390/f14091783
Xu, X. L. (2007). Effect of cold stratification and temperature on seed germination of two types of Plant of the Alpine Meadow : Lanzhou University.
Xu, H. C., Wu, H., and Fu, L. Q. (2002). Study on integrated Forest Pest control. J. Zhejiang Forest. Sci. Technol. 22, 74–77. doi: 10.3969/j.issn.1001-3776.2002.02.020
Xu, C. H., Xiang, W. H., Gou, M. M., Chen, L., Lei, P. F., Fang, X., et al. (2018). Effects of Forest restoration on soil carbon, nitrogen, phosphorus, and their stoichiometry in Hunan, southern China. Sustain. For. 10:1874.
Xu, Q. W., Zhang, X. J., Li, J. X., Ren, J. R., Ren, L. L., and Luo, Y. Q. (2023). Pine wilt disease in northeast and Northwest China: a comprehensive risk review. Forests 14. doi: 10.3390/f14020174
Xue, G. Y., Wu, J. D., Zhou, B. J., Zhu, X. P., Zeng, J., Ma, Y., et al. (2023). Effects of shading on the growth and photosynthetic fluorescence characteristics of Castanopsis hystrix seedlings of top community-building species in southern subtropical China. Forests 14:1659. doi: 10.3390/f14081659
Yan, E. R., Wang, X. H., Shi, J. Y., Wang, X. B., and Wang, L. Y. (2005). Sprouting ecology of woody plants: a research review. Chin. J. Appl. Ecol. 16, 2459–2464.
Yan, X. Y., Zhang, G. Q., Zang, L. P., Liu, Q. F., Chen, D. M., and Sui, M. Z. (2024). Based on the 2012-2021 English literature metrology analysis of the Forest seedling research Progress update. J. Mount. Agric. Biol. 43, 23–30. doi: 10.15958/j.cnki.sdnyswxb.2024.04.004
Yang, R. Q., Fu, P. L., Fan, Z. X., Panthi, S., Gao, J., Niu, Y., et al. (2022). Growth-climate sensitivity of two pine species shows species-specific changes along temperature and moisture gradients in Southwest China. Agric. For. Meteorol. 318:108907. doi: 10.1016/j.agrformet.2022.108907
Zamfir, M. (2000). Effects of bryophytes and lichens on seedling emergence of Alvar plants: evidence from greenhouse experiments. Oikos 88, 603–611. doi: 10.1034/j.1600-0706.2000.880317.x
Zang, R. G., Zhang, W. Y., and Ding, Y. (2007). Seed dynamics in relation to gaps in a tropical montane rainforest of Hainan Island, South China: (I) seed rain. J. Integr. Plant Biol. 49, 1565–1572. doi: 10.1111/j.1774-7909.2007.00577.x
Zhang, J. F., Ge, J. R., Dayananda, B., and Li, J. Q. (2022). Effect of light intensities on the photosynthesis, growth and physiological performances of two maple species. Front. Plant Sci. 13:999026. doi: 10.3389/fpls.2022.999026
Zhang, H. D., Huang, W. L., Ma, X. M., Liu, Y. R., Li, Z. J., and Wng, Y. N. (2023). Study on the effect of abiotic factors on natural regeneration of seeds. J. Temp. Forest. Res. 6, 48–51. doi: 10.3969/j.issn.2096-4900.2023.03.008
Zhang, M., Wang, Z., Liu, X., and Yi, X. (2017). Seedling predation of Quercus mongolica by small rodents in response to forest gaps. New For. 48, 83–94. doi: 10.1007/s11056-016-9557-1
Zhang, W. C., and Zhang, J. P. (2004). Problems and countermeasures of planted forests. Prot. Forest Sci. Technol. 60, 45–46. doi: 10.13601/j.issn.1005-5215.2004.03.019
Zhang, H., Zhou, G. M., Wang, Y. X., Tang, C. X., and Cai, Y. J. (2021). Clear-cut and forest regeneration increase soil N2O emission in Cunninghamia lanceolata plantations. Geoderma 401:115238. doi: 10.1016/j.geoderma.2021.115238
Zhao, W. W., Sun, Y. J., and Gao, Y. F. (2023). Potential factors promoting the natural regeneration of Larix principis-rupprechtii in North China. Peerj 11:e15809. doi: 10.7717/peerj.15809
Zheng, X., Liu, Y. M., Wang, Q. X., Si, L. Q., Shu, L. F., Chen, F., et al. (2025). Research progress on effects of fire disturbance on standstructure and vegetation regeneration. J. Beijing Forest. Univ. 47, 156–162.
Zheng, R. S., and Zheng, R. (2013). Effects of shading treatment on active oxygen Metabolismand activities of antioxidant enzymes in tree Peony leaves. Shandong Agric. Sci. 45, 63–65. doi: 10.14083/j.issn.1001-4942.2013.04.037
Zhou, T., Geng, Y. J., Chen, J., Pan, J. J., Haase, D., and Lausch, A. (2020). High-resolution digital mapping of soil organic carbon and soil Total nitrogen using DEM derivatives, Sentinel-1 and Sentinel-2 data based on machine learning algorithms. Sci. Total Environ. 729:138244. doi: 10.1016/j.scitotenv.2020.138244
Zhou, Y., Huang, L. H., Wei, X. L., Zhou, H. Y., and Chen, X. (2017). Physiological, morphological, and anatomical changes in Rhododendron Agastum in response to shading. Plant Growth Regul. 81, 23–30. doi: 10.1007/s10725-016-0181-z
Keywords: environmental factors, natural regeneration, soil, moisture content, temperature
Citation: Liu J (2025) Progress in research on the effects of environmental factors on natural forest regeneration. Front. For. Glob. Change. 8:1525461. doi: 10.3389/ffgc.2025.1525461
Received: 09 November 2024; Accepted: 21 March 2025;
Published: 03 April 2025.
Edited by:
Alireza Rahemi, Morehead State University, United StatesReviewed by:
Timo Domisch, Natural Resources Institute Finland (Luke), FinlandCopyright © 2025 Liu. This is an open-access article distributed under the terms of the Creative Commons Attribution License (CC BY). The use, distribution or reproduction in other forums is permitted, provided the original author(s) and the copyright owner(s) are credited and that the original publication in this journal is cited, in accordance with accepted academic practice. No use, distribution or reproduction is permitted which does not comply with these terms.
*Correspondence: Jiabo Liu, MjAyMjE0MTI0MDEzNUBzdHUuc2N1LmVkdS5jbg==
Disclaimer: All claims expressed in this article are solely those of the authors and do not necessarily represent those of their affiliated organizations, or those of the publisher, the editors and the reviewers. Any product that may be evaluated in this article or claim that may be made by its manufacturer is not guaranteed or endorsed by the publisher.
Research integrity at Frontiers
Learn more about the work of our research integrity team to safeguard the quality of each article we publish.